- 1School of Chemical and Printing-Dyeing Engineering, Henan University of Engineering, Zhengzhou, China
- 2School of Chemical Engineering and Food Science, Zhengzhou Institute of Technology, Zhengzhou, China
Macrocyclic arenes-based fluorescent indicator displacement assays (F-IDAs) offer a unique and innovative approach to chemosensing, taking molecular recognition in host-guest chemistry to a higher level. Because of their special architecture and versatile host–guest binding properties, macrocyclic arenes, principally calix[n]arenes and, in recent years, pillar[n]arenes, in combination with various fluorophores, are widely used in F-IDAs for the specific and selective sensing of cationic, anionic, and neutral analytes. In this paper, we review recent progress in the development of F-IDAs based on macrocyclic arenes and outline the prospects and remaining challenges relating to macrocyclic arenes-based F-IDAs.
Introduction
Molecular sensors are considered a key component in the advancement of biological, environmental, and industrial sciences. Traditionally, the most widely used sensing assay has been the indicator spacer receptor approach (ISR), which involves the creation of covalent linkages between the indicator (fluorophore) and the receptor (Roberts, 1989). However, attaching the indicator to the receptor requires much synthetic work, and this is a major limitation of ISR. To avoid this problem, novel facile sensing assays through different non-covalent interactions have been developed (You et al., 2015; Mako et al., 2019; Hein et al., 2020), with indicator displacement assays (IDAs) being the most widely used, as well as offering a unique and innovative approach (Inouye et al., 1994; Nguyen et al., 2004). Displacement assays require that the indicator be able to reversibly bind to a receptor. The subsequent addition of a competitive analyte causes the displacement of the indicator from the receptor, regulating an optical signal if the indicator’s binding affinity to the receptor is smaller than that of the analyte.
Supramolecular chemistry, also known as host–guest chemistry, originated from the research of Pedersen, who was the first one to report the synthesis of crown ethers in 1967 (Pedersen, 1967). The field advanced rapidly, with an important development being the design of highly-selective macrocyclic hosts as receptors and their application in molecular sensing. The use of IDAs in supramolecular sensing was first proposed by Nguyen and Anslyn (2006), You and Anslyn (2012), You et al. (2015). Following these early efforts, the field has expanded to include colorimetric IDAs (C-IDAs) (Leontiev and Rudkevich, 2005), fluorescent IDAs (F-IDAs) (Martínez-Máñez and Sancenón, 2003), and metal complexing IDAs (M-IDAs) (Nguyen and Anslyn, 2006), etc. Of the different IDA approaches, F-IDAs provide the most convenient and efficient method to identify novel receptors able to bind to different target molecules (Bonizzoni, 2017). Calix[n]arenes, pillar[n]arenes, and their analogs—jointly referred to as “macrocyclic arenes”—are macrocyclic hosts formed by hydroxy-or alkoxy-substituted aromatic rings bridged by methylene or methenyl groups (Chen and Han, 2018). Macrocyclic arenes can provide the appropriate non-covalent interactions (such as hydrogen bonding, π-π or cation-π stacking, or hydrophobic or electrostatic interactions) to yield suitable complexation. F-IDAs have been created using synthetic macrocyclic arenes and a variety of fluorophores. The complexation of fluorophores by macrocyclic arenes causes readily detectable changes to their fluorescence properties, especially the intensity. The addition of an appropriate analyte can result in the displacement of the fluorophore from the complex to produce a detectable fluorescent response, converting the receptor-analyte binding event into an easily observable signal (Figure 1).
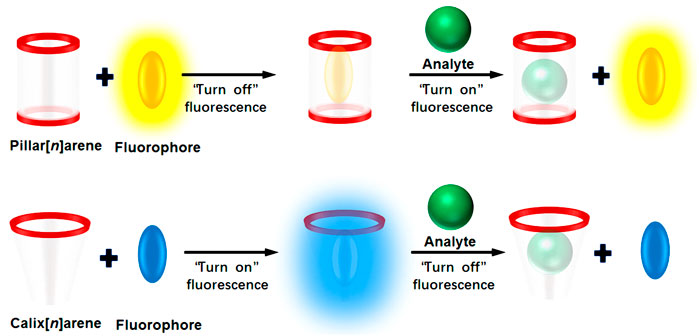
FIGURE 1. Cartoon depiction of an F-IDA which involves an integrated host-fluorophore sensor that disassembles in the presence of an analyte to produce a turn-on or turn-off fluorescence response.
This mini-review reports the progress of the development and application of macrocyclic arenes-based F-IDAs over the past 10 years, especially in the fields of analytical science and biological systems, focusing on two main types of macrocyclic arene, calix[n]arenes, and pillar[n]arenes. The structures of calix[n]arenes and pillar[n]arenes as synthetic receptors, fluorophores as indicators and analytes as competitors used in F-IDAs are shown in Figure 2. The objective of this review is to explore macrocyclic arenes-based F-IDAs in terms of their construction and detection mechanisms and major analytical applications.
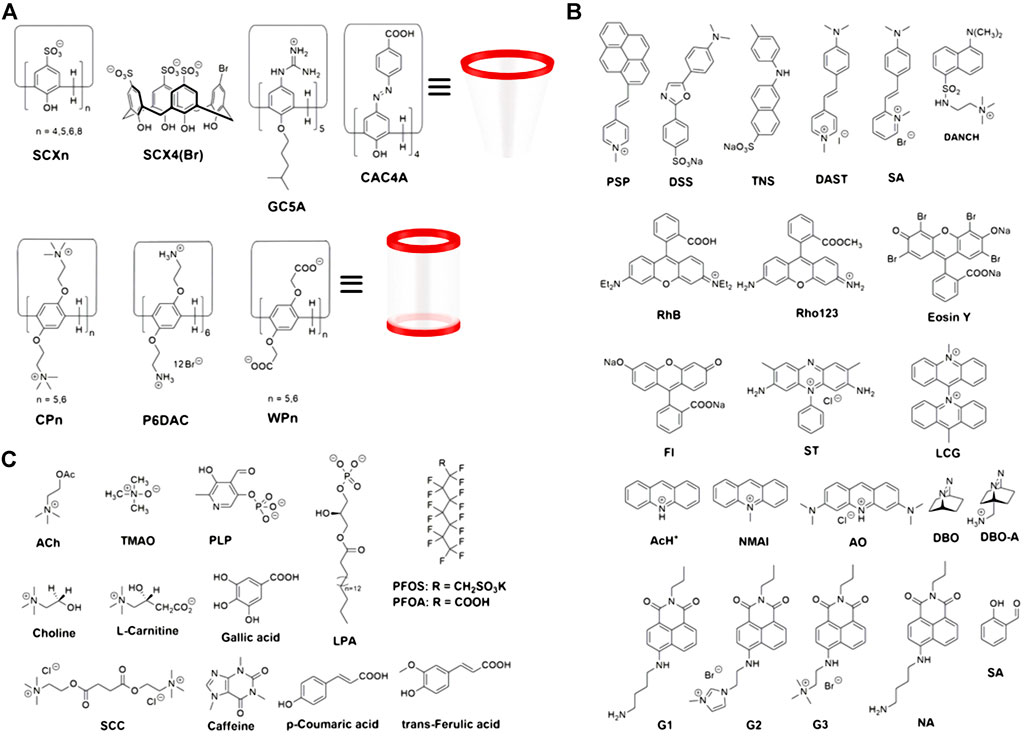
FIGURE 2. Chemical structures of (A) macrocyclic arenes as synthetic receptors, (B) fluorophores as indicators and (C) partial analytes detected as competitors.
Calix[n]arenes-based fluorescent indicator displacement assays
Calix[n]arenes, first effectively synthesized and named by Gutsche (1998) in the late 1970s, were the third class of macrocycles to be developed. The calix[n]arenes (n ≥ 4) especially, which are comprised of hydroxy-substituted aromatic rings bridged with methylene groups at the o-positions of phenolic hydroxy groups, possess tunable scaffolds and controllable conformations for the binding of cationic and neutral organic guests (Boehmer, 1995). Due to their ability to alter the photophysical properties of fluorophores (Dsouza et al., 2011), calix[n]arenes have been used to form a host–guest complex for the determination of analyte concentrations via F-IDA (Ghale and Nau, 2014).
In 1996, Koh et al. (1996) proposed an artificial-signaling acetylcholine (ACh) receptor system that could easily illuminate the binding process of the cationic fluorophore to the p-sulfonatocalix[6]arene (SCX6) through a fluorescence intensity change. It was found that the fluorescence of the pyrene-modified pyridinium cation (PSP) is quenched upon binding to SCX6 but then returns following the displacement of SCX6 by ACh. In 2002, Zhang et al. (2002) also reported an F-IDA for determining ACh in a neutral aqueous medium based on SCX8 and Rhodamine B (RhB). The formation of the RhB⊂SCX8 complex brought about the fluorescence quenching of RhB, and the subsequent displacement of RhB by ACh caused an obvious increase in fluorescence emission. Later, Jin (2003) also developed an F-IDA to detect ACh in water based on SCX8 and dansylcholine (DANCh). The fluorescence intensity of DANCh in an aqueous solution was increased by the complexation with SCX8, and the subsequent replacement of DANCh in the DANCh⊂SCX8 complex by ACh greatly lowered the fluorescence intensity. This research provided a novel fluorometric approach to determine ACh (>10–4 M) with ATP and amino acids in a physiological salt solution.
In 2005, Bakirci et al. (2005) proposed a novel approach to detect inorganic cation binding via F-IDA based on the complexing of the receptor SCX4 with the fluorescent indicator 2,3-diazabicyclo [2.2.2]oct-2-ene (DBO). Their research showed that fluorescence regeneration occurred in different metal ions, and binding between monovalent cations (alkali and ammonium) and SCX4 was observed for the first time and quantitatively measured. Later in 2006, the same group also used the DBO and SCX4 combination to detect choline and carnitine derivatives through F-IDA (Bakirci and Nau, 2006). They observed that adding SCX4 to DBO solutions causes efficient fluorescence quenching, but adding choline and carnitine derivatives released DBO from the cavity of SCX4, leading to regeneration of its fluorescence. In 2007, SCX4 and aminomethyl-substituted DBO (DBO-A) were also used for monitoring cationic products in amino acid decarboxylase-catalyzed reactions through F-IDA (Hennig et al., 2007). Upon binding to SCX4, the fluorescence intensity of DBO-A is quenched, and the signal is turned on after displacement of DBO-A from the DBO-A⊂SCX4 complex by ammonium products. SCX4 shows higher affinities to cationic alkylammonium products than amino acids, so adding amino acids would not affect the F-IDA. The authors demonstrated that this system is an efficient approach to the evaluation of the inhibitor for enzymatic transformation.
In 2011, Guo et al. (2011) reported a system utilizing SCX4 and SCX5 for the determination of ACh through F-IDA applicable to the real-time monitoring of acetylcholinesterase. Their research shows that lower concentrations of ACh binds with the macrocycle, leading to the release of cationic fluorophore lucigenin (LCG) and a fluorescence turn-on response. Although the bound LCG exhibited weak fluorescence, its displacement by ACh significantly increased emission, and, therefore, the conversion of acetylcholine to choline significantly decreased the fluorescence intensity. Later in 2016, SCX4 and protonated cationic fluorophore acridine (AcH+) were also used as the reporter pair for the detection of ACh through F-IDA. It was found that the binding of AcH+ to SCX4 causes a dramatic fluorescence “turn OFF,” which can be switched to a strong fluorescence “turn ON” through ACh addition (Sayed et al., 2016).
In 2012, Minaker et al. (2012) reported a mix-and-match F-IDA toolkit for use in responding to different histone code analytes, such as methylated lysine. This F-IDA system comprised the cationic fluorophores LCG and N-alkyl-pyridinium (PSP) modified by pyrene, as well as various calixarene hosts [SCX4, SCX6, and SCX4(Br)]. With the addition of cationic peptides, cationic fluorophores were emitted, causing a fluorescence turn-on response. The authors used these ensembles to identify the unmethylated, mono-, di-, and trimethylated lysine of a single histone tail sequence. On the other hand, Norouzy et al. (2015) also used SCX4-based F-IDA in living cells to absorb various bioorganic analytes, including protamine, choline, and ACh. Their research showed that tight binding of the fluorescent indicator LCG to the receptor SCX4 caused fluorescence quenching of the LCG. The subsequent addition of organic analytes, such as choline, protamine, or ACh, could displace the indicator LCG from the receptor upon entry into living cells because of its high affinity with the receptor SCX4, thus, causing a fluorescence turn-on response. This research confirmed that F-IDA could be combined with other synthetic receptors to detect the uptake of bioorganic analytes in living cells.
In 2018, Gao et al. (2018) realized the discrimination of highly similar Glycosaminoglycans (GAGs) via F-IDA (Zheng et al., 2018a). An F-IDA comprising four reporter pairs and the cationic fluorophore eosin Y was developed. In the sensing array, the guanidinium and quaternary ammonium derivatives of calixarene act as the receptors. The complexation of calixarenes quenches the fluorescence of eosin Y, and, thereby, the competitive complexation of the six GAG analytes leads to fluorescence regeneration through the displacement of eosin Y from the calixarene receptors, achieving turn-on sensing. By implementing F-IDA on four reporter pairs for selective and sensitive detection of six GAG analytes, the authors derived a unique fluorescence response pattern for each GAG. This calixarene-based F-IDA strategy easily targets analyte libraries to achieve different sensing patterns for each analyte. In the same year, Guo and co-workers also applied F-IDA to the ultrasensitive selective detection of a cancer biomarker, lysophosphatidic acid (LPA), at a nanomolar level by a turn-on fluorescence response with guanidinium-modified calix[5]arene (GC5A) as the receptor and fluorescein (FI) as the fluorescent indicator (Zheng et al., 2018b). They observed that FI fluorescence emission quenching occurred after the binding of FI with GC5A, and subsequent displacement of FI from GC5A through competitive binding by LPA, causing a turn-on fluorescence response. In another study, the same group also the GC5A-FI pair in the sensitive, selective, and label-free detection of bisphosphonates (BPs) in buffer solution and artificial urine via F-IDA, with the replacement of FI in the complex by BPs triggering a turn-on fluorescence response (Gao et al., 2018). This label-free sensing strategy shows application potential for the real-time monitoring of BPs concentration in urine and pharmacokinetic research. Later in 2019, the same group utilized the GC5A-FI pair for the sensitive and quantitative detection of PFOA and PFOS in contaminated water via F-IDA (Zheng et al., 2019). They also reported a sensing strategy suited to the turn-on fluorescence detection for metabolite trimethylamine N-oxide (TMAO) through F-IDA using GC5A and FI, demonstrating an inexpensive, convenient, label-free, and sensitive approach to detecting TMAO, which provides a new method for TMAO detection in clinical studies (Yu et al., 2019). The GC5A and FI reporter pair was also chosen to detect turn-on fluorescence in enzymatic substrate Pyridoxal-5′-phosphate (PLP) through F-IDA. (Yue et al., 2019).
Guo and co-workers proposed a simple F-IDA strategy to detect hypoxia in living cells based on an azocalix[4]arene (CAC4A) host and the fluorescent guest rhodamine 123 (Rho123) (Geng et al., 2019). Bioreductive enzymes capable of reducing various organic functionalities can be over-expressed in a hypoxic environment. Rho123 exhibited fluorescence quenching upon being located inside the cavity of CAC4A, and in a hypoxic environment, the azo groups of Rho123⊂CAC4A are reduced and transformed to amino groups, causing the release of Rho123 and a fluorescence turn-on response (Geng et al., 2019).
Pillar[n]arenes-based fluorescent indicator displacement assays
Pillar[n]arenes, also known as “pillararenes,” are new macrocyclic hosts, being studied for the first time by Ogoshi et al. (2008). These macrocyclic hosts are usually produced by the condensation of hydroquinone with paraformaldehyde (Ogoshi et al., 2016). They are widely studied because of their synthetic accessibility and pillar-shaped three-dimensional structures. Their symmetrical rigid pillar-shaped cavity, electron-rich cavity, easy modification, and special host-guest recognition characteristics have led researchers to favor them over traditional calix[n]arenes for building F-IDAs to detect different analytes.
In 2014, Wang et al. (2014) reported the first pillararene-based F-IDA, the water-soluble carboxylato-pillar[5]arene (WP5), as a macrocyclic host for the detection of paraquat via F-IDA. They used N-methylacridinium iodide (NMAI), a fluorophore, as the fluorescent indicator and found that, at pH 7.4, paraquat displaced the fluorophore from the WP5 cavity, resulting in a prominent emission increase. Later in 2015, Bojtár et al. (2015) reported a fluorescence spectroscopic study on the host-guest interaction of three different pyridinium-based stilbazolium dyes and WP5 in an aqueous solution. The resulting WP5 and 4-dimethylaminostyryl-N-methylpyridinium iodide (DAST) complex was successfully applied as an F-IDA-based probe for the sensitive detection of paraquat. In another study, Bojtár et al. (2016) presented a supramolecular sensory system consisting of WP5 and a 4-amino-1,8-naphthalimide cationic fluorophore having three anchors (G1, G2, and G3) that they used to selectively investigate basic amino acids via F-IDA. Later in 2019, based on their previous studies, Bojtár et al. (2016) also evaluated the complexation of three fluorescent indicators-a pyridinium-based stilbazolium dye (DAST) and two 4-amino-1,8-naphthalimide fluorophores with positively charged anchors (G2 and G3)-with carboxylato-pillar[6]arene, WP6. The stilbazolium dye gave turn-on, and the two naphthalimide derivatives turn-off fluorescence responses upon complexation. The two WP6-indicator supramolecular systems were used in the F-IDAs selective detection of histamine (Paudics et al., 2019). In another example, Hua et al. (2018) discovered a new WP6-based pillararene-indicator complex utilizing an aromatic fluorophore acridine orange (AO). Under the system, choline bound strongly to the WP6 cavity, displacing the already captured AO, causing emission enhancement. The constructed pillararene-indicator system was used to detect choline compounds and monitor enzymatic reactions. In the meantime, Pan et al. (2018) proposed a simple and rapid F-IDA method to detect Succinylcholine Chloride (SCC) based on naphthalimide dye (NA) or stilbazolium dye (SA) and the WP5 complex. In this F-IDA study, upon addition of SCC to the NA⊂WP5 or SA⊂WP5 solution, there was a recovery of the fluorescence intensity, bringing about the recognition of SCC due to competitive supramolecular displacement between SCC, NA, and SA for WP5. Recently, the authors of this mini-review proposed the use of F-IDA to perform selective detection of caffeine based on WP6 and the fluorophore safranine T (ST). The addition of caffeine to the ST⊂WP6 complex brings about ST displacement because of the higher binding affinity, with a turn-off fluorescence response (Duan et al., 2021).
Cationic water-soluble pillar[n]arenes containing an ammonium group have also been used to construct F-IDA to detect various analytes. In 2016, Hua et al. (2016) described a turn-on fluorescence switch based on the host-guest complexation between a water-soluble ammonium pillar[5]arene (CP5) and a salicylaldehyde (SA). CP5 encapsulated SA to promote deprotonation of SA and generate a highly fluorescent host-guest complex. SA deprotonation and deaggregation induced by complexation led to a significant fluorescence increase in the SA solution. This turn-on fluorescence switch was also used to detect F-IDA in phenols and chlorophenols. Later in 2017, Bojtár et al. (2017) employed the water-soluble ammonium pillar[6]arene (CP6) receptor with a larger cavity and a fluorescent indicator dapoxyl sodium sulfonate (DSS) for F-IDA. The complexation between CP6 and DSS produced a significant increase in fluorescence intensity. This system was selective for ATP detection over ADP/AMP/GTP through the ATP-induced displacement of the DSS on the CP6. This research was the first to demonstrate the importance of cationic pillararene-based F-IDA in detecting analytes with biological importance. Recently, the authors of this mini-review reported a novel fluorescence activation switch based on the host-guest complexation between a fluorescent indicator probe 6-p-toluidinylnaphthalene-2-sulfonate (TNS) and water-soluble pillar[6]arene dodecyl-ammonium chloride (P6DAC). The complexation remarkably increased the fluorescence intensity, and adding food additives (p-coumaric acid, trans-ferulic acid and gallic acid) to the TNS⊂P6DAC complex results in the displacement of TNS and thus causes a turn-off fluorescence response, which was employed for the F-IDA detection of phenolic food additives (Duan et al., 2022).
Conclusion and outlook
In this mini-review, we have attempted to highlight the recent advances and future prospects of F-IDAs using macrocyclic arenes as molecular recognition units. Direct sensing based on F-IDAs was explored for various analytes, such as cations, anions, small neutral molecules, or bioanalytes, using calix[n]arenes and pillar[n]arenes. Though great progress has been achieved in the development of macrocyclic arenes-based F-IDAs, there are still relatively few F-IDAs that can be truly applied to real-world environments. However, progress to date suggests that, through continued effort, more macrocyclic arenes and fluorescent indicators with better performance will be synthesized, and, in the future, F-IDAs based on macrocyclic arenes will become more reliable and versatile tools that address an ever-widening scope of real-world problems, such as the detection of diseases and providing environmental monitoring.
Author Contributions
QD supervised the project and mainly wrote the paper. All authors extensively reviewed the manuscript, and approved the final version of the manuscript to be submitted.
Funding
This work was supported by Henan Province Science and Technology Research Program (No. 222102320067).
Conflict of interest
The authors declare that the research was conducted in the absence of any commercial or financial relationships that could be construed as a potential conflict of interest.
Publisher’s note
All claims expressed in this article are solely those of the authors and do not necessarily represent those of their affiliated organizations, or those of the publisher, the editors and the reviewers. Any product that may be evaluated in this article, or claim that may be made by its manufacturer, is not guaranteed or endorsed by the publisher.
References
Bakirci, H., Koner, A. L., and Nau, W. M. (2005). Binding of inorganic cations by p-sulfonatocalix[4]arene monitored through competitive fluorophore displacement in aqueous solution. Chem. Commun. 43, 5411–5413. doi:10.1039/b510319c
Bakirci, H., and Nau, W. M. (2006). Fluorescence regeneration as a signaling principle for choline and carnitine binding: a refined supramolecular sensor system based on a fluorescent azoalkane. Adv. Funct. Mat. 16, 237–242. doi:10.1002/adfm.200500219
Boehmer, V. (1995). Calixarenes, macrocycles with (almost) unlimited possibilities. Angew. Chem. Int. Ed. Engl. 34, 713–745. doi:10.1002/anie.199507131
Bojtár, M., Kozma, J., Szakacs, Z., Hessz, D., Kubinyi, M., Bitter, I., et al. (2017). Pillararene-based fluorescent indicator displacement assay for the selective recognition of ATP. Sensors Actuators B Chem. 248, 305–310. doi:10.1016/j.snb.2017.03.163
Bojtár, M., Paudics, A., Hessz, D., Kubinyi, M., and Bitter, I. (2016). Amino acid recognition by fine tuning the association constants: Tailored naphthalimides in pillar [5] arene-based indicator displacement assays. RSC Adv. 6, 86269–86275. doi:10.1039/C6RA15003A
Bojtár, M., Szakács, Z., Hessz, D., Kubinyi, M., and Bitter, I. (2015). Optical spectroscopic studies on the complexation of stilbazolium dyes with a water soluble pillar[5]arene. RSC Adv. 5, 26504–26508. doi:10.1039/C4RA14809F
Bonizzoni, M. (2017). “Fluorescent sensors based on indicator displacement,” in Comprehensive supramolecular chemistry II. Editor J. L. Atwood (Amsterdam: Elsevier), 21–36.
Chen, C.-F., and Han, Y. (2018). Triptycene-derived macrocyclic arenes: from calixarenes to helicarenes. Acc. Chem. Res. 51, 2093–2106. doi:10.1021/acs.accounts.8b00268
Dsouza, R. N., Pischel, U., and Nau, W. M. (2011). Fluorescent dyes and their supramolecular host/guest complexes with macrocycles in aqueous solution. Chem. Rev. 111, 7941–7980. doi:10.1021/cr200213s
Duan, Q., Xing, Y., and Guo, K. (2021). Carboxylato-Pillar[6]arene-Based fluorescent indicator displacement assays for caffeine sensing. Front. Chem. 9, 816069. doi:10.3389/fchem.2021.816069
Duan, Q., Xing, Y., and Guo, K. (2022). The detection of food additives using a fluorescence indicator based on 6-p-toluidinylnaphthalence-2-sulfonateand cationic pillar[6]arene. Front. Chem. 10, 925881. doi:10.3389/fchem.2022.925881
Gao, J., Zheng, Z., Shi, L., Wu, S. Q., Sun, H., Guo, D. S., et al. (2018). Strong binding and fluorescence sensing of bisphosphonates by guanidinium-modified calix[5]arene. Beilstein J. Org. Chem. 14, 1840–1845. doi:10.3762/bjoc.14.157
Geng, W. C., Jia, S. R., Zheng, Z., Li, Z. H., Ding, D., Guo, D. S., et al. (2019). A noncovalent fluorescence turn-on strategy for hypoxia imaging. Angew. Chem. Int. Ed. 58, 2377–2381. doi:10.1002/anie.201813397
Ghale, G., and Nau, W. M. (2014). Dynamically analyte-responsive macrocyclic host-fluorophore systems. Acc. Chem. Res. 47, 2150–2159. doi:10.1021/ar500116d
Guo, D. S., Uzunova, V. D., Su, X., Liu, Y., and Nau, W. M. (2011). Operational calixarene-based fluorescent sensing systems for choline and acetylcholine and their application to enzymatic reactions. Chem. Sci. 2, 1722–1734. doi:10.1039/c1sc00231g
Gutsche, C. D. (1998). Calixarenes revisited; monographs in supramolecular chemistry. Cambridge: Royal Society of Chemistry.
Hein, R., Beer, P. D., and Davis, J. J. (2020). Electrochemical anion sensing: supramolecular approaches. Chem. Rev. 120, 1888–1935. doi:10.1021/acs.chemrev.9b00624
Hennig, A., Bakirci, H., and Nau, W. M. (2007). Label-free continuous enzyme assays with macrocycle-fluorescent dye complexes. Nat. Methods 4, 629–632. doi:10.1038/nmeth1064
Hua, B., Shao, L., Yu, G., and Huang, F. (2016). Fluorescence indicator displacement detection based on pillar[5]arene-assisted dye deprotonation. Chem. Commun. 52, 10016–10019. doi:10.1039/c6cc04919b
Hua, B., Shao, L., Zhang, Z., Sun, J., and Yang, J. (2018). Pillar[6]arene/acridine orange host-guest complexes as colorimetric and fluorescence sensors for choline compounds and further application in monitoring enzymatic reactions. Sensors Actuators B Chem. 255, 1430–1435. doi:10.1016/j.snb.2017.08.141
Inouye, M., Hashimoto, K., and Isagawa, K. (1994). Nondestructive detection of acetylcholine in protic media: artificial-signaling acetylcholine receptors. J. Am. Chem. Soc. 116, 5517–5518. doi:10.1021/ja00091a085
Jin, T. (2003). A new fluorometric method for the detection of the neurotransmitter acetylcholine in water using a dansylcholine complex with p-sulfonated calix[8]arene. J. Incl. Phenom. Macrocycl. Chem. 45, 195–201. doi:10.1023/A:1024506714332
Koh, K. N., Araki, K., Ikeda, A., Otsuka, H., and Shinkai, S. (1996). Reinvestigation of calixarene-based artificial-signaling acetylcholine receptors useful in neutral aqueous (water/methanol) solution. J. Am. Chem. Soc. 118, 755–758. doi:10.1021/ja951488k
Leontiev, A. V., and Rudkevich, D. M. (2005). Revisiting noncovalent SO2-amine chemistry: an indicator-displacement assay for colorimetric detection of SO2. J. Am. Chem. Soc. 127, 14126–14127. doi:10.1021/ja053260v
Mako, T. L., Racicot, J. M., and Levine, M. (2019). Supramolecular luminescent sensors. Chem. Rev. 119, 322–477. doi:10.1021/acs.chemrev.8b00260
Martínez-Máñez, R., and Sancenón, F. (2003). Fluorogenic and chromogenic chemosensors and reagents for anions. Chem. Rev. 103, 4419–4476. doi:10.1021/cr010421e
Minaker, S. A., Daze, K. D., Ma, M. C. F., and Hof, F. (2012). Antibody-free reading of the histone code using a simple chemical sensor array. J. Am. Chem. Soc. 134, 11674–11680. doi:10.1021/ja303465x
Nguyen, B. T., and Anslyn, E. V. (2006). Indicator–displacement assays. Coord. Chem. Rev. 250, 3118–3127. doi:10.1016/j.ccr.2006.04.009
Nguyen, B. T., Wiskur, S. L., and Anslyn, E. V. (2004). Using indicator-displacement assays in test strips and to follow reaction kinetics. Org. Lett. 6, 2499–2501. doi:10.1021/ol0493599
Norouzy, A., Azizi, Z., and Nau, W. M. (2015). Indicator displacement assays inside live cells. Angew. Chem. Int. Ed. 54, 792–795. doi:10.1002/anie.201407808
Ogoshi, T., Kanai, S., Fujinami, S., Yamagishi, T. A., and Nakamoto, Y. (2008). para-Bridged symmetrical pillar[5]arenes: Their lewis acid catalyzed synthesis and host-guest property. J. Am. Chem. Soc. 130, 5022–5023. doi:10.1021/ja711260m
Ogoshi, T., Yamagishi, T.-a., and Nakamoto, Y. (2016). Pillar-shaped macrocyclic hosts pillar[n]arenes: New key players for supramolecular chemistry. Chem. Rev. 116, 7937–8002. doi:10.1021/acs.chemrev.5b00765
Pan, Q., He, Z., Xia, D., Gu, X., Wang, H., Sun, J., et al. (2018). Fluorescent determination of succinylcholine chloride by naphthalimide/stilbazolium dye ⊂ CP5A. J. Fluoresc. 28, 581–587. doi:10.1007/s10895-018-2219-2
Paudics, A., Kubinyi, M., Bitter, I., and Bojtár, M. (2019). Carboxylato-pillar[6]arene-Based fluorescent indicator displacement assays for the recognition of monoamine neurotransmitters. RSC Adv. 9, 16856–16862. doi:10.1039/c9ra03241j
Pedersen, C. J. (1967). Cyclic polyethers and their complexes with metal salts. J. Am. Chem. Soc. 89, 7017–7036. doi:10.1021/ja01002a035
Roberts, S. M. (1989). Molecular recognition: chemical and biochemical problems. Cambridge: Royal Society of Chemistry.
Sayed, M., Shinde, K., Shah, R., and Pal, H. (2016). pH-responsive indicator displacement assay of acetylcholine based on acridine-p-sulfonatocalix[4]arene supramolecular system: Fluorescence off/on switching and reversible pKa shift. ChemistrySelect 5, 989–999. doi:10.1002/slct.201600226
Wang, P., Yao, Y., and Xue, M. (2014). A novel fluorescent probe for detecting paraquat and cyanide in water based on pillar [5]arene/10-methylacridinium iodide molecular recognition. Chem. Commun. 50, 5064–5067. doi:10.1039/C4CC01403K
You, L., and Anslyn, E. V. (2012). “Competition experiments,” in Supramolecular chemistry. Editors P. Gale, and J. Steed (Chichester: John Wiley & Sons). doi:10.1002/9780470661345.smc010
You, L., Zha, D., and Anslyn, E. V. (2015). Recent advances in supramolecular analytical chemistry using optical sensing. Chem. Rev. 115, 7840–7892. doi:10.1021/cr5005524
Yu, H., Geng, W. C., Zheng, Z., Gao, J., Guo, D. S., Wang, Y., et al. (2019). Facile fluorescence monitoring of gut microbial metabolite trimethylamine N-oxide via molecular recognition of guanidinium-modified calixarene. Theranostics 9, 4624–4632. doi:10.7150/thno.33459
Yue, Y. X., Kong, Y., Yang, F., Zheng, Z., Hu, X. Y., Guo, D. S., et al. (2019). Supramolecular tandem assay for pyridoxal-5’-phosphate by the reporter pair of guanidinocalix[5]Arene and fluorescein. ChemistryOpen 8, 1437–1440. doi:10.1002/open.201900316
Zhang, Y. J., Cao, W.-X., and Xu, J. (2002). Interaction of sulfonated calix[n]arenes with rhodamine B and its application to determine acetylcholine in a real neutral aqueous medium. Chin. J. Chem. 20, 322–326. doi:10.1002/cjoc.20020200406
Zheng, Z., Geng, W. C., Gao, J., Mu, Y.-J., and Guo, D. S. (2018a). Differential calixarene receptors create patterns that discriminate glycosaminoglycans. Org. Chem. Front. 5, 2685–2691. doi:10.1039/C8QO00606G
Zheng, Z., Geng, W. C., Gao, J., Wang, Y. Y., Sun, H., Guo, D. S., et al. (2018b). Ultrasensitive and specific fluorescence detection of a cancer biomarker via nanomolar binding to a guanidinium-modified calixarene. Chem. Sci. 9, 2087–2091. doi:10.1039/C7SC04989G
Keywords: calix[n]arenes, pillar[n]arenes, host-guest complex, fluorescence, indicator displacement assay
Citation: Duan Q, Wang F and Lu K (2022) Recent advances in macrocyclic arenes-based fluorescent indicator displacement assays. Front. Chem. 10:973313. doi: 10.3389/fchem.2022.973313
Received: 20 June 2022; Accepted: 30 June 2022;
Published: 18 July 2022.
Edited by:
Tangxin Xiao, Changzhou University, ChinaReviewed by:
Bin Hua, ZJU-Hangzhou Global Scientific and Technological Innovation Center, ChinaCopyright © 2022 Duan, Wang and Lu. This is an open-access article distributed under the terms of the Creative Commons Attribution License (CC BY). The use, distribution or reproduction in other forums is permitted, provided the original author(s) and the copyright owner(s) are credited and that the original publication in this journal is cited, in accordance with accepted academic practice. No use, distribution or reproduction is permitted which does not comply with these terms.
*Correspondence: Qunpeng Duan, cXBkdWFuQGhhdWUuZWR1LmNu; Kui Lu, bHVja3lsdWtlQGhhdWUuZWR1LmNu