- 1State Key Laboratory Breeding Base of Green Pesticide and Agricultural Bioengineering, Key Laboratory of Green Pesticide and Agricultural Bioengineering, Ministry of Education, Guizhou University, Guiyang, China
- 2School of Biological Sciences, Guizhou Education University, Guiyang, China
To discover a lead compound for agricultural use, 34 novel chalcone derivatives containing an 1,2,4-oxadiazole moiety were designed and synthesized. Their nematocidal activities against Bursaphelenchus xylophilus, Aphelenchoides besseyi, and Ditylenchus dipsaci and their antiviral activities against tobacco mosaic virus (TMV), pepper mild mottle virus (PMMoV), and tomato spotted wilt virus (TSWV) were evaluated. Biological assay results indicate that compounds A13 and A14 showed good nematocidal activities against B. xylophilus, A. besseyi, and D. dipsaci, with LC50 values of 35.5, 44.7, and 30.2 μg/ml and 31.8, 47.4, and 36.5 μg/ml, respectively, which are better than tioxazafen, fosthiazate, and abamectin. Furthermore, compound A16 demonstrated excellent protective activity against TMV, PMMoV, and TSWV, with EC50 values of 210.4, 156.2, and 178.2 μg/ml, respectively, which are superior to ningnanmycin (242.6, 218.4, and 180.5 μg/ml).
Introduction
Plant-parasitic nematodes (PPNs) are a very important group of pests that include more than 60 regulated species. These pests are extremely difficult to prevent and cause annual global agricultural losses of roughly $157 billion (Abad, et al., 2008; Bernard, et al., 2017; Abd-Elgawad, 2020; Kantor, et al., 2022). At present, the application of chemical nematicides is the most reliable and effective method to control PPNs. However, these treatments are mainly based on highly toxic organophosphorus and carbamate nematocides, such as fosthiazate, cadusafos, fenamiphos, dazomet, aldicarb, oxamyl, and so on. The long-term use, overuse, and misuse of these nematicides have not only led to poor control effect and serious resistance but have also seriously harmed the environment (Ntalli and Caboni, 2012; Chen, et al., 2020). Meanwhile, plant virus disease, as a “plant cancer,” can lead to considerable crop loss (Chen, et al., 2016). Although Ribavirin is widely used to prevent plant virus disease, its inhibitory effect to a virus is less than 50% at 500 mg/L (Wang, et al., 2012). To date, we still lack effective and low toxicity nematicides and antiviral agents for use in agricultural production. In addition, a combined infection of PPNs and viruses will significantly increase the loss of agricultural production. Hence, the discovery of new, environmentally friendly, and efficient nematicides and antiviral agents is key to controlling plant nematode and virus diseases.
Natural products have often been the source of new drug discovery and have the benefits of low toxicity, easy decomposition, and are environmentally friendly (Leonard and Stephen, 2007; Qian, et al., 2010; Chen, et al., 2020). As one of the most important natural products, chalcone is widely found in plants (Du, et al., 2013) and has various biological activities, including anticancer (Kim, et al., 2013; Wang, et al., 2015), antibacterial (Wei, et al., 2016), antifungal (Lahtchev, et al., 2008) and antiviral (Park, et al., 2011) effects in medicine. In addition, chalcone and its derivatives have insecticidal (Thirunarayanan, et al., 2010), nematicidal (Attar, et al., 2011; Nunes, et al., 2013; Caboni, et al., 2016), antiviral (Du, et al., 2013), and other agricultural activities. In our previous work, we reported that chalcone derivatives containing 1,3,4-oxadiazole/thiadiazole, purine, and ferulic acid moieties have excellent antiviral activities (Gan, et al., 2017a; Gan, et al., 2017b).
As an important heterocyclic compound, 1,2,4-oxadiazole has been widely studied by pesticide scientists with a wide range of biological activities, such as herbicidal (Hang, et al., 2014), antibacterial (Karad, et al., 2017), antifungal (Yang, et al., 2021), and insecticidal activities (Fernandes, et al., 2020), among others. Tioxazafen, which is one of the 1,2,4-oxadiazole compounds, was designed by Monsanto as a new type of seed treatment agent to control nematodes in soybean, corn, and cotton (Slomczynska, et al., 2015). However, Tioxazafen has not formally been used on a large scale in agricultural production. To enhance the flexibility of the structure of Tioxazafen and discover the high activity 1,2,4-oxadiazole compound, some 1,2,4-oxadiazole derivatives containing 1,3,4-oxadiazole/thiadiazole and amide moieties with good nematocidal, antibacterial, and antifungal activities have been synthesized (Zhu, et al., 2020; Liu, et al., 2022).
Based on the biological activity of chalcone and 1,2,4-oxadiazole derivatives, the current study aims to further improve the nematocidal activity, and to extend the biological activity of chalcone and 1,2,4-oxadiazole moieties. In particular, a 1,2,4-oxadiazole fragment is introduced to the chalcone skeleton to obtain 34 novel chalcone derivatives containing 1,2,4-oxadiazole moiety (Figure 1). Their nematocidal activities against Bursaphelenchus xylophilus, Aphelenchoides besseyi, and Ditylenchus dipsaci and their antiviral activities to tobacco mosaic virus (TMV), pepper mild mottle virus (PMMoV), and tomato spotted wilt virus (TSWV) were then evaluated.
Materials and Methods
General Information
The melting points of the compounds were determined on an X-4B microscope melting point apparatus and were uncorrected (Shanghai Electrophysics Optical Instrument Co., Ltd., Shanghai, China). The 1H NMR and 13C NMR spectra data of the compounds were recorded on a Bruker DPX-400 spectrometer (Bruker, Billerica, MA, United States), using DMSO-d6 as solvents and tetramethylsilane as an internal standard. The high-resolution mass spectrometer (HRMS) data of the compounds were obtained with a Thermo Scientific Q-Exactive (Thermo Scientific, Missouri, MOThermo, United States). Reactions were detected by thin-layer chromatography (TLC) and visualized under UV light at 254 nm. Chromatography was conducted on silica gel 200–300 mesh.
Synthesis
Preparation Procedure for Intermediates 2 and 6
As shown in Schemes 1 and 2, the chalcone intermediates 2 and 6 were obtained according to our previously reported methods (Gan, et al., 2017c). First, 0.2 M aqueous sodium hydroxide solution (22 mmol) was added to a solution of 4-hydroxyacetophenone or 4-hydroxybenzaldehyde (20 mmol) and various substituted benzaldehyde or acetophenone (20 mmol) in 20 ml ethanol, and then stirred at room temperature for 12 h. Second, upon reaction completion (monitored by TLC), the mixture was poured into ice-water and acidified to a pH value of 2‒3 by dropwise addition of aqueous HCl, filtered, washed, and dried to obtain intermediates 2 and 6.
Preparation Procedure for Intermediate 4
An aqueous solution of sodium hydroxide (50 mmol) was added to a solution of hydroxylamine hydrochloride (50 mmol) in 30 ml ethanol and stirred at room temperature. Various benzonitriles (50 mmol) were then added to the mixture. The mixture was heated to reflux and monitored with TLC. After completion of the reaction, the precipitated product was filtrated and the filtrate was concentrated under reduced pressure. The residue was dissolved with toluene and chloroacetyl chloride (50 mmol) was added dropwise into the mixture in an ice bath, and then refluxed for 10 h. The solvent was removed and the residue was dissolved with dichloromethane and washed with brine. The organic layer was then dried and further purified by column chromatography to afford intermediate 4.
Preparation Procedure for the Target Compounds A1−A21 and B1−B13
A solution of intermediate 4 (2.0 mmol) in 5 ml DMF was added to a solution of intermediate 2 or 6 (2.0 mmol), K2CO3 (2.2 mmol) in 5 ml N,N-dimethylformamide (DMF) and warmed to 40°C for 4 h. Upon completion of reaction, the mixture was poured into ice-water, filtered, and recrystallized from methanol to give the pure target compounds A1−A21 and B1−B13. The physical properties, 1HNMR, 13CNMR, and HRMS for title compounds are reported in the Supplementary Data. The spectral data of A1 and B1 are shown below.
(E)-3-(2,4-dichlorophenyl)-1-(4-((3-phenyl-1,2,4-oxadiazol-5-yl)methoxy)phenyl)prop-2-en-1-one (A1). White powder; m.p. 154–155°C; yield 89%; 1H NMR (400 MHz, DMSO-d6): δ 8.02 (d, J = 6.4 Hz, 2H), 7.79 (d, J = 8.8 Hz, 2H), 7.77 (d, J = 1.6 Hz, 1H), 7.59–7.58 (m, 5H), 7.40 (d, J = 16.0 Hz, 1H), 7.17 (d, J = 9.2 Hz, 2H), 7.16 (d, J = 15.6 Hz, 1H), 5.70 (s, 2H); 13C NMR (101 MHz, DMSO-d6): δ 192.83, 175.99, 168.85, 160.07, 147.02, 138.07, 135.90, 132.31, 131.63, 131.50, 131.50, 131.10, 130.12, 129.84, 129.84, 128.38, 128.10, 127.56, 127.56, 126.20, 124.95, 115.80, 115.80, 61.39. HRMS (ESI) m/z for C24H17O3N2Cl2 [M+H]+ calcd: 451.06107, found: 451.05978.
(E)-1-(2,4-dichlorophenyl)-3-(4-((3-phenyl-1,2,4-oxadiazol-5-yl)methoxy)phenyl)prop-2-en-1-one (B1). Faint yellow powder; m.p. 126–127°C; yield 56%; 1H NMR (400 MHz, DMSO-d6): δ 8.33–8.27 (m, 3H), 8.12–7.98 (m, 4H), 7.79 (d, J = 1.6 Hz, 1H), 7.67–7.59 (m, 4H), 7.33 (d, J = 8.8 Hz, 2H), 5.82 (s, 2H); 13C NMR (101 MHz, DMSO-d6): δ 187.54, 175.87, 168.27, 161.79, 137.19, 135.98, 135.57, 132.31, 131.92, 131.67, 131.67, 131.65, 130.30, 129.95, 129.84, 129.84, 128.39, 127.57, 127.57, 126.20, 125.72, 115.39, 115.39, 61.51. HRMS (ESI) m/z for C24H17O3N2Cl2 [M+H]+ calcd: 451.06107, found: 451.05972.
Nematocidal Activity Test
B. xylophilus, A. besseyi, and D. dipsaci were bred with potato dextrose agar–Botrytis cinerea provided from the Fine Chemical Research and Development Center of Guizhou University (Guizhou, China). The nematocidal bioassays of these target compounds was tested based on the previous reported methods with minor modification (Wei, et al., 2021). The compound was dissolved with 50 μl DMF, and was then diluted with 1% Tween-80 to obtain 50 and 10 μg/ml concentrations. Meanwhile, fosthiazate and tioxazafen were used as positive controls at the same concentrations and without compounds solution as a negative control group. Then, 10 µl of nematode suspension with 50 nematodes and 300 µl of the solution were added to the corresponding hole of 48-well plates, each treatment was repeated three times, and they were then placed in a biochemical incubator at 27°C for dark light culture. After 48 h, the dead nematodes were counted and the corrected mortality was calculated with the following formula:
Antiviral Activity Test
Nicotiana tabacum cv. K326, Nicotiana benthamiana, and Nicotiana glutinosa L. plants were cultivated in a greenhouse. N. tabacum cv. K326 was used to determine systemic TMV infection, and N. benthamiana was used to determine systemic PMMoV and TSWV infection. N. glutinosa L. was used as a local lesion host to evaluate the antiviral activity against TMV, PMMoV, and TSWV when the plants grew to 5–6 leaf stages. TMV, PMMoV, and TSWV were purified by the Gooding method (Gooding and Hebert, 1967) and the curative, protective activities of compounds were performed with the reported methods at 500 μg/ml (Song, et al., 2005; Zan, et al., 2021; Shi, et al., 2022). The EC50 values of the antiviral activity at concentrations of 500, 250, 125, 62.5, and 31.25 μg/ml were then calculated. The positive controls included ribavirin and ningnanmycin. Measurements were performed in triplicates.
Results and Discussion
Chemistry
The influence of the catalyst, temperature, and solvent for preparation compound A1 was tested and evaluated to obtain the facile, high efficiency, and yield synthetic method of the target compound; the results are given in Table 1. The results indicate that the yield of compound A1 was affected by the catalyst, solvent, and temperature. The optimum synthesis condition is catalyst as K2CO3/KI, DMF as solvent, and reaction for 6 h at 80°C. Under this condition, the yield of compound A1 achieved 89%. The other compounds were then prepared with the same condition. The structures of all of the compounds were identified with 1H NMR, 13C NMR, and HRMS. Two doublets appear in the 1H NMR data of compound A1, 7.40 (J = 16.0 Hz) ppm and 7.16 (J = 15.6 Hz) ppm, which indicate the presence of the HC=CH group. The proton of CH2 appears as a singlet at 5.70 ppm. Meanwhile, the 192.83 ppm peak of the 13C NMR data indicates the presence of the C=O group, and the 170.32 and 167.66 ppm peaks indicate the presence of C proton in the 1,2,4-oxadiazol group. The 61.39 ppm peak indicates the presence of the C proton of the CH2 group. Furthermore, compound A1 was confirmed correctly with HRMS data of the [M+H]+ as 451.05978, the calculated value was 451.06107.
Nematocidal Activity Test
The results of nematocidal activities of compounds are given in Table 2. As shown in Table 2, compounds A13, A14, and B3 exhibited higher nematocidal activity against B. xylophilus at 50 μg/ml, the corrected mortalities were 100%, 100%, and 51.8%, respectively, which are superior to those of tioxazafen (34.3%), fosthiazate (43.9%), and abamectin (49.4%). Meanwhile, compounds A13, A14, B6, and B12 showed good nematocidal activity against A. besseyi at 50 μg/ml, with corrected mortalities of 100%, 100%, 70.8%, and 59.6%, which are better than tioxazafen (40.0%) and abamectin (42.3%). In addition, compounds A13, A14, and B11 possessed desired nematocidal activity against D. dipsaci, with corrected mortalities of 100%, 100%, and 41.0%, respectively, which are superior to tioxazafen (29.0%), fosthiazate (33.3%), and abamectin (33.6%). However, there was dissatisfactory nematocidal activity of all compounds against B. xylophilus, A. besseyi, and D. dipsaci at 10 μg/ml.
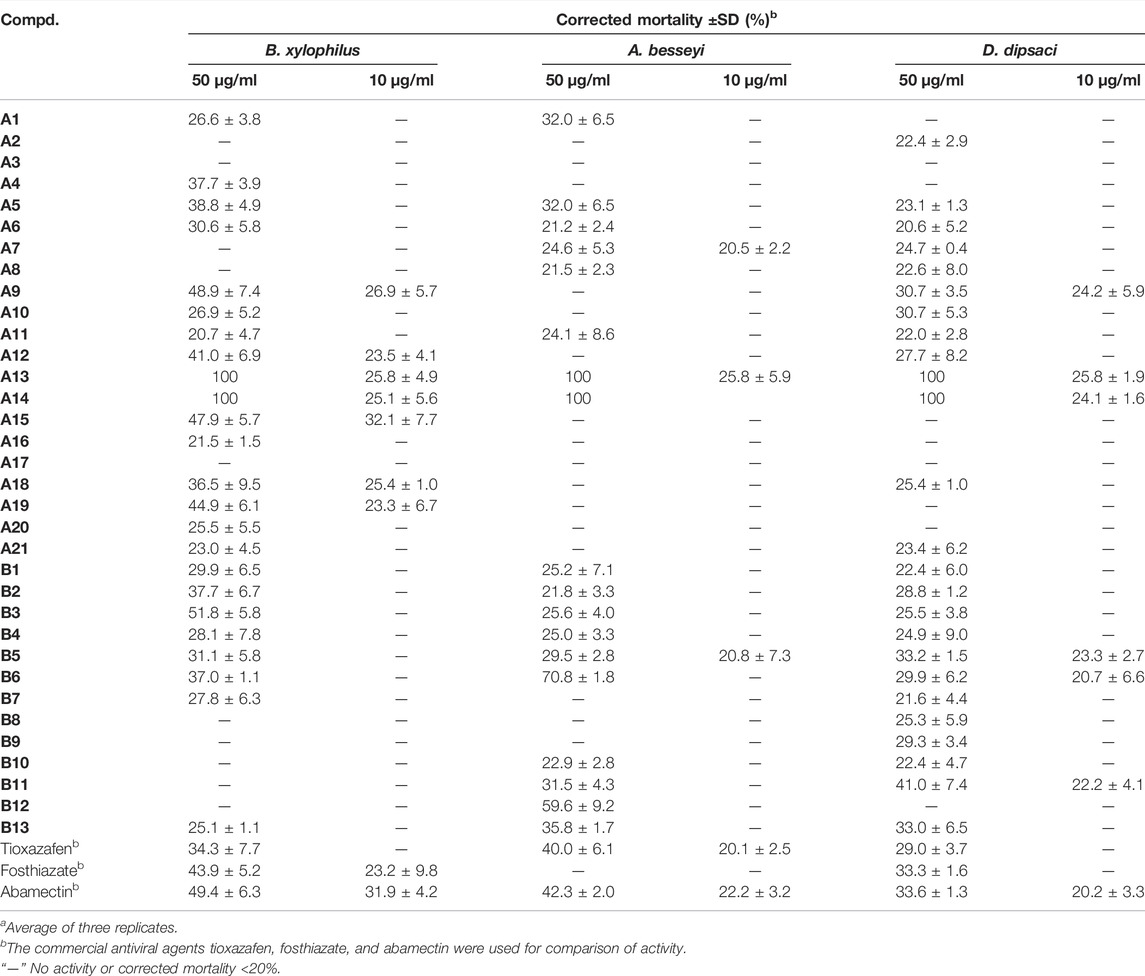
TABLE 2. Nematicidal activity of compounds A1−A21 and B1−B13.a
To further confirm their nematicidal activities of compounds A13 and A14, the LC50 values of compounds A13 and A14 against B. xylophilus, A. besseyi, and D. dipsaci were evaluated as tioxazafen, fosthiazate, and abamectin for positive controls; the results are given in Table 3. As shown in Table 3, compounds A13 and A14 had LC50 values of 35.5, 44.7, and 30.2 μg/ml and 31.8, 47.4, and 36.5 μg/ml against B. xylophilus, A. besseyi, and D. dipsaci, respectively, which are superior to tioxazafen, fosthiazate, and abamectin. In addition, the results indicate that A series compounds have better nematocidal activity than the B series compound. However, there is no obvious regularity between activity and structure.
Antiviral Activity Test
The antiviral activities of the target compounds were performed with the half leaf blight spot method and the results are given in Tables 4 and 5. As shown in Table 4, compounds A4, A11, A16, A18, and A20 exhibited better curative activity against TMV at 500 μg/ml, with values of 49.8%, 53.6%, 57.2%, 52.3%, and 51.2%, respectively, which are superior than those of ribavirin (39.9%) and ningnanmycin (49.8%). These compounds also showed good protective activity to TMV, the inhibitory was 64.5%, 67.9%, 68.6%, 65.2%, and 67.1%, respectively, which are better than those of ribavirin (51.2%) and ningnanmycin (61.3%). Meanwhile, compounds A4, A11, A16, A18, and B11 showed desirable curative action against PMMoV, the values were 52.3%, 53.6%, 56.5%, 55.6%, and 52.9%, which are better than those of ribavirin (31.6%) and ningnanmycin (51.8%). Furthermore, compounds A4, A11, A16, A18, A20, and B11 showed excellent protective activity against PMMoV, with values of 67.1%, 65.6%, 71.8%, 70.2%, 68.1%, and 63.7%, respectively, which are superior to ribavirin (48.8%) and ningnanmycin (63.3%). Unfortunately, the curative effect of compounds to TSWV was dissatisfactory. However, compounds A16 (69.5%) and A18 (65.6%) showed better protective activity against TSWV than ribavirin (46.2%) and ningnanmycin (65.1%). The results of the EC50 values (Table 5) indicate that compounds A16 and A18 showed excellent curative and protective activities against TMV and PMMoV, with EC50 values of 368.7 and 210.4 μg/ml, 310.8 and 156.2 μg/ml, 410.5 and 251.2 μg/ml, and 345.6 and178.2 μg/ml, respectively, which are superior to ningnanmycin (420.5 and 242.6 μg/ml, and 415.8 and 218.4 μg/ml, respectively). In addition, compound A16 (178.9 μg/ml) showed better protective activity against TSWV than ningnanmycin (180.5 μg/ml).
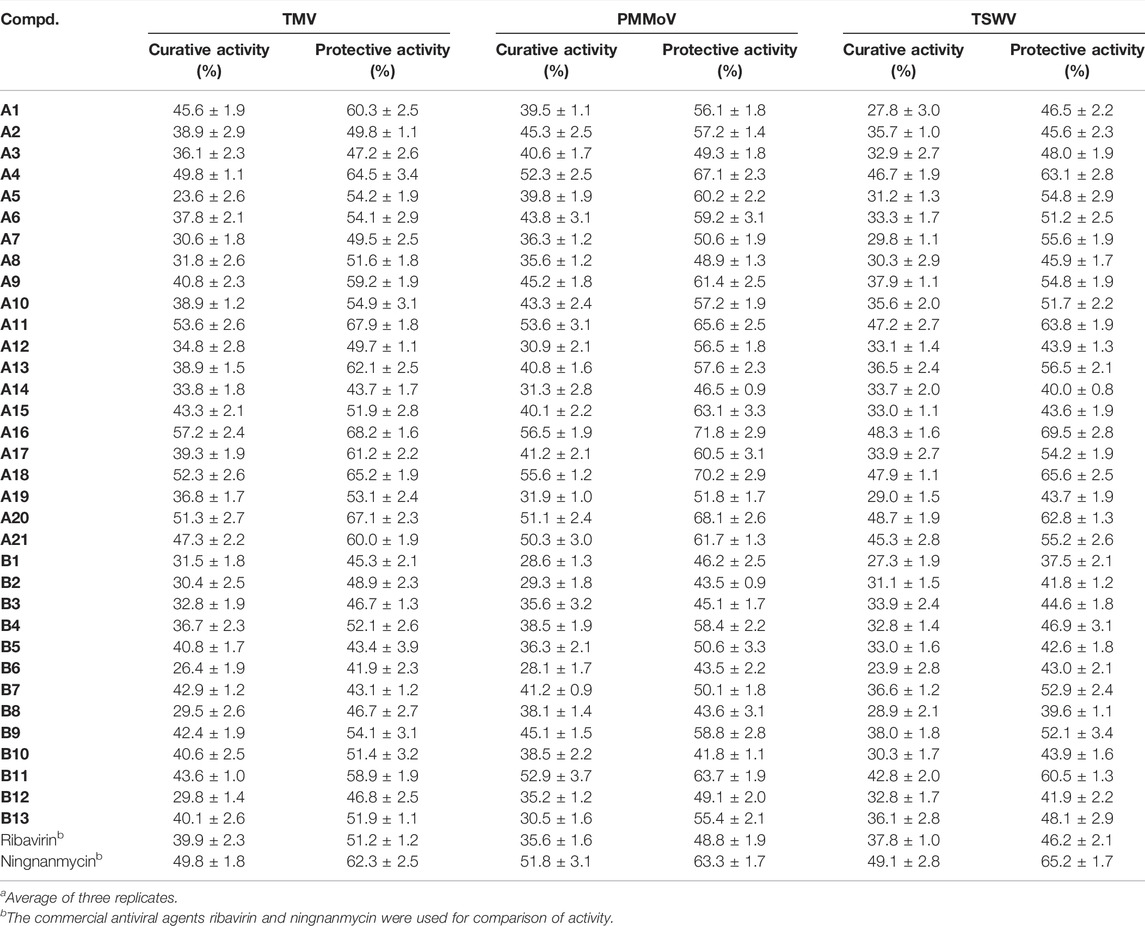
TABLE 4. Antiviral activities of compounds A1−A21and B1−B13 at 500 μg/ml.a
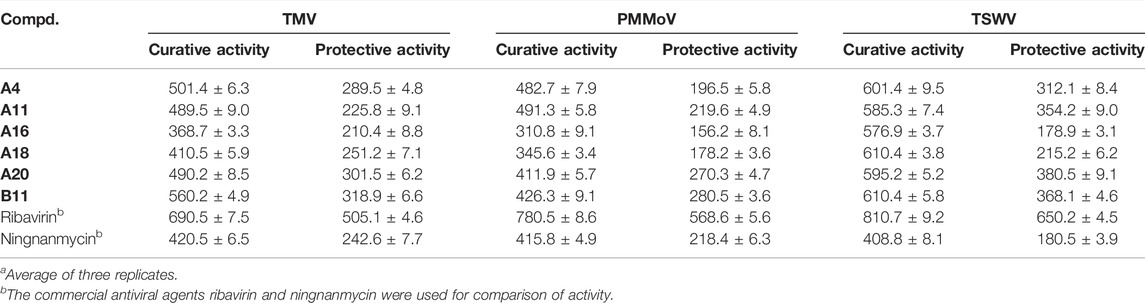
TABLE 5. The EC50 values of the compounds against TMV, PMMoV, and TSWVa.
Structure-activity relationship analysis based on protective activity against three viruses indicates that A series compounds have better antiviral activity than B series compounds, which is consistent with the trend of nematicidal activity. Further structure-activity relationship analysis demonstrated that the compound with R1 as OCH3 showed better antiviral activity than that of the compounds with other groups, such as A13 (R1 = 4-OCH3, R2 = H) > A21 (R1 = 4-Br, R2 = H), A10 (R1 = 4-CH3, R2 = H), A5 (R1 = H, R2 = H), and A1 (R1 = 2,4-diCl, R2 = H). In particular, the compound with 2-OCH3 of R1 had the best antiviral activity; for example, A16 (R1 = 2-OCH3, R2 = H) > A13 (R1 = 4-OCH3, R2 = H) > A14 (R1 = 3-OCH3, R2 = H). Compared with the electron-donating group (CH3), the introduction of strong electron withdraw group (F) into R2 can favor antiviral activity, such as A18 (R1 = 2-OCH3, R2 = 4-F) > A17 (R1 = 2-OCH3, R2 = 4-CH3) and A11 (R1 = 4-OCH3, R2 = 4-F) > A12 (R1 = 4-OCH3, R2 = 4-CH3), A4 (R1 = 2,4-diCl, R2 = 4-F) > A2 (R1 = 2,4-diCl, R2 = 4-CH3).
Conclusion
In the present work, 34 novel chalcone derivatives containing an 1,2,4-oxadiazole moiety were synthesized and assessed for the nematocidal and antiviral activities of all of the compounds. The results show that compounds A13 and A14 have excellent nematocidal activities against B. xylophilus, A. besseyi, and D. dipsaci and are superior to tioxazafen, fosthiazate, and abamectin. Furthermore, compound A16 has better protective activity against TMV, PMMoV, and TSWV than that of ribavirin and ningnanmycin. Therefore, chalcone derivatives containing an 1,2,4-oxadiazole moiety can be considered as candidate leading structures for the development of new pesticides.
Data Availability Statement
The datasets presented in this study can be found in online repositories. The names of the repository/repositories and accession number(s) can be found in the article/Supplementary Material.
Author Contributions
LL and DL contributed to the synthesis, characterization, and activity research of all compounds. LL prepared the original manuscript. LL and SL analyzed the data. XG designed and supervised the research and revised the manuscript. All authors discussed, edited, and approved the final version.
Funding
This work was supported by the Outstanding Young Scientific and Technological Talents Project of Guizhou Province ([2019] 5646), the National Nature Science Foundation of China (32060622), the Construction Project of Key Laboratories from the Education Department of Guizhou Province (QJHKY [2018] 001), and Program of Introducing Talents to Chinese Universities (111 Program no. D20023).
Conflict of Interest
The authors declare that the research was conducted in the absence of any commercial or financial relationships that could be construed as a potential conflict of interest.
Publisher’s Note
All claims expressed in this article are solely those of the authors and do not necessarily represent those of their affiliated organizations, or those of the publisher, the editors, and the reviewers. Any product that may be evaluated in this article, or claim that may be made by its manufacturer, is not guaranteed or endorsed by the publisher.
Supplementary Material
The Supplementary Material for this article can be found online at: https://www.frontiersin.org/articles/10.3389/fchem.2022.943062/full#supplementary-material
References
Abad, P., Gouzy, J., Aury, J.-M., Castagnone-Sereno, P., Danchin, E. G. J., and Deleury, E. (2008). Genome Sequence of the Metazoan Plant-Parasitic Nematode Meloidogyne incognita. Nat. Biotechnol. 26, 909–915. doi:10.1038/nbt.1482
Abd-Elgawad, M. M. M. (2020). Biological Control Agents in the Integrated Nematode Management of Pepper in Egypt. Egypt. J. Biol. Pest Co. 30, 1–10. doi:10.1186/s41938-020-00273-6
Attar, S., O’Brien, Z., Alhaddad, H., Golden, M. L., and Calderón-Urrea, A. (2011). Ferrocenyl Chalcones versus Organic Chalcones: A Comparative Study of Their Nematocidal Activity. Bioorg. Med. Chem. 19, 2055–2073. doi:10.1016/j.bmc.2011.01.048
Bernard, G. C., Egnin, M., and Bonsi, C. (2017). The Impact of Plant-Parasitic Nematodes on Agriculture and Methods of Control. Nematology 7, 122–151. doi:10.5772/intechopen.68958
Caboni, P., Aissani, N., Demurtas, M., Ntalli, N., and Onnis, V. (2016). Nematicidal Activity of Acetophenones and Chalcones against Meloidogyne incognita and Structure–Activity Considerations. Pest Manag. Sci. 72, 125–130. doi:10.1002/ps.3978
Chen, J. X., Li, Q. X., and Song, B. A. (2020). Chemical Nematicides: Recent Research Progress and Outlook. J. Agric. Food Chem. 68, 12175–12188. doi:10.1021/acs.jafc.0c02871
Chen, M. H., Li, P., Hu, D. Y., Zeng, S., Li, T. X., Jin, L., et al. (2016). Synthesis, Antiviral Activity, 3D-QSAR, and Interaction Mechanisms Study of Novel Malonate Derivatives Containing Quinazolin-4(3h)-One Moiety. Bioorg. Med. Chem. Lett. 26, 168–173. doi:10.1021/jf301337610.1016/j.bmcl.2015.11.006
Du, G., Han, J. M., Kong, W. S., Zhao, W., Yang, H. Y., Yang, G. Y., et al. (2013). Chalcones from the Flowers of Rosa Rugosa and Their Anti-tobacco Mosaic Virus Activities. Kor. Chem. Soc. 34, 1263–1265. doi:10.5012/bkcs.2013.34.4.1263
Fernandes, F. S., Santos, H., Lima, S. R., Conti, C., Rodrigues, M. T., Zeoly, L. A., et al. (2020). Discovery of Highly Potent and Selective Antiparasitic New Oxadiazole and Hydroxy-Oxindole Small Molecule Hybrids. Eur. J. Med. Chem. 201, 112418. doi:10.1016/j.ejmech.2020.112418
Gan, X. H., Hu, D. Y., Chen, Z., Wang, Y. J., and Song, B. A. (2017a). Synthesis and Antiviral Evaluation of Novel 1,3,4-Oxadiazole/Thiadiazole-Chalcone Conjugates. Bioorg. Med. Chem. 27, 4298–4301. doi:10.1016/j.bmcl.2017.08.038
Gan, X. H., Hu, D. Y., Wang, Y. J., Yu, L., and Song, B. (2017b). A. Novel Trans-ferulic Acid Derivatives Containing a Chalcone Moiety as Potential Activator for Plant Resistance Induction. J. Agric. Food Chem. 65, 4367–4377. doi:10.1021/acs.jafc.7b00958
Gan, X. H., Wang, Y. J., Hu, D. Y., and Song, B, A. (2017c). Design, Synthesis, and Antiviral Activity of Novel Chalcone Derivatives Containing a Purine Moiety. Chin. J. Chem. 35, 665–672. doi:10.1002/cjoc.201600568
Gooding, G. V. J., and Hebert, T. T. (1967). A Simple Technique for Purification of Tobacco Mosaic Virus in Large Quantities. Phytopathology 57, 1285–1287. doi:10.1098/rstl.1767.0047
Hang, T. H., Chen, H., Chen, J., and Zhang, A. D. (2014). Syntheses, Crystal Structures, and Biological Activities of Two 5-Pyrimidinyl-1,2,4-Oxadiazoles. Chin. J. Struc. Chem. 10, 1455–1459. doi:10.14102/j.cnki.0254-5861.2014.10.029
Kantor, M., Handoo, Z., Kantor, C., and Carta, L. (2022). Top Ten Most Important U.S.-Regulated and Emerging Plant-Parasitic Nematodes. Horticulturae 8, 208. doi:10.3390/horticulturae8030208
Karad, S. C., Purohit, V. B., Thummar, R. P., Vaghasiya, B. K., Kamani, R. D., Thakor, P., et al. (2017). Synthesis and Biological Screening of Novel 2-Morpholinoquinoline Nucleus Clubbed with 1,2,4-Oxadiazole Motifs. Eur. J. Med. Chem. 126, 894–909. doi:10.1016/j.ejmech.2016.12.016
Kim, S. H., Lee, E., Baek, K. H., Kwon, H. B., Woo, H., Lee, E. S., et al. (2013). Chalcones, Inhibitors for Topoisomerase I and Cathepsin B and L, as Potential Anti-cancer Agents. Bioorg. Med. Chem. Lett. 23, 3320–3324. doi:10.1016/j.bmcl.2013.03.106
Lahtchev, K. V., Batovska, D. I., Parushev, S. P., Ubiyvovk, V. M., and Sibirny, A. A. (2008). Antifungal Activity of Chalcones: A Mechanistic Study Using Various Yeast Strains. Eur. J. Med. Chem. 43, 2220–2228. doi:10.1016/j.ejmech.2007.12.027
Leonard, G. C., and Stephen, O. D. (2007). Natural Products that Have Been Used Commercially as Crop Protection Agents. Pest Manag. Sci. 63, 524–554. doi:10.1002/ps.1378
Liu, D., Luo, L., Wang, Z. X., Ma, X. Y., and Gan, X. H. (2022). Design, Synthesis and Antifungal/Nematicidal Activity of Novel 1,2,4-Oxadiazole Derivatives Containing Amide Fragments. Int. J. Mol. Sci. 23, 1596. doi:10.3390/ijms23031596
Ntalli, N. G., and Caboni, P. (2012). Botanical Nematicides: A Review. J. Agric. Food Chem. 60, 9929–9940. doi:10.1021/jf303107j
Nunes, A. S., Campos, V. P., Mascarello, A., Stumpf, T. R., Chiaradia-Delatorre, L. D., Machado, M. A. T., et al. (2013). Activity of Chalcones Derived from 2,4,5-Trimethoxybenzaldehyde against Meloidogyne Exigua and In Silico Interaction of One Chalcone with a Putative Caffeic Acid 3-O-Methyltransferase from Meloidogyne Incognita. Exp. Parasitol. 135, 661–668. doi:10.1016/j.exppara.2013.10.003
Park, J. Y., Jeong, H. J., Kim, Y. M., Park, S. J., Rho, M. C., Park, K. H., et al. (2011). Characteristic of Alkylated Chalcones from Angelica Keiskei on Influenza Virus Neuraminidase Inhibition. Bioorg. Med. Chem. Lett. 21, 5602–5604. doi:10.1016/j.bmcl.2011.06.130
Qian, X. H., Lee, P. W., and Cao, S. (2010). China: Forward to the Green Pesticides via a Basic Research Program. J. Agric. Food Chem. 58, 2613–2623. doi:10.1021/jf904098w
Shi, J., He, H. F., Hu, D. Y., and Song, B. A. (2022). Defense Mechanism of Capsicum Annuum L. Infected with Pepper Mild Mottle Virus Induced by Vanisulfane. J. Agric. Food. Chem. 70, 3618–3632. doi:10.1021/acs.jafc.2c00659
Slomczynska, U., South, M. S., Bunkers, G. J., Edgecomb, D., Wyse-Pester, D., Selness, S., et al. (2015). Tioxazafen: A New Broad-Spectrum Seed Treatment Nematicide. J. Am. Chem. Soc. 10, 129–147. doi:10.1021/bk-2015-1204.ch010
Song, B. A., Zhang, H. P., Wang, H., Yang, S., Jin, L. H., Hu, D. Y., et al. (2005). Synthesis and Antiviral Activity of Novel Chiral Cyanoacrylate Derivatives. J. Agric. Food. Chem. 53, 7886–7891. doi:10.1021/jf051050w
Thirunarayanan, G., Surya, S., Srinivasan, S., Vanangamudi, G., and Sathiyendiran, V. (2010). Synthesis and Insect Antifeedant Activities of Some Substituted Styryl 3, 4-Dichlorophenyl Ketones. Spectrochim. Acta A. 75, 152–156. doi:10.1016/j.saa.2009.10.003
Wang, H. M., Zhang, L., Liu, J., Yang, Z. L., Zhao, H. Y., Yang, Y., et al. (2015). Synthesis and Anti-cancer Activity Evaluation of Novel Prenylated and Geranylated Chalcone Natural Products and Their Analogs. Eur. J. Med. Chem. 92, 439–448. doi:10.1016/j.ejmech.2015.01.007
Wang, Z. W., Wang, L., Ma, S., Liu, Y. X., Wang, L. Z., and Wang, Q. M. (2012). Design, Synthesis, Antiviral Activity, and SARs of 1,4-Aminophenanthroindolizidines. J. Agric. Food Chem. 60, 5825–5831. doi:10.1021/jf3013376
Wei, C. Q., Huang, J. J., Luo, Y. Q., Wang, S. B., Wu, S. K., Xing, Z. F., et al. (2021). Novel Amide Derivatives Containing an Imidazo [1,2-α] Pyridine Moiety: Design, Synthesis as Potential Nematicidal and Antibacterial Agents. Pestic. Biochem. Physiol. 175, 104857. doi:10.1016/j.pestbp.2021.104857
Wei, Z. Y., Chi, K. Q., Yu, Z. K., Liu, H. Y., Sun, L. P., Zheng, C. J., et al. (2016). Synthesis and Biological Evaluation of Chalcone Derivatives Containing Aminoguanidine or Acylhydrazone Moieties. Bioorg. Med. Chem. Lett. 26, 5920–5925. doi:10.1016/j.bmcl.2016.11.001
Yang, S., Ren, C. L., Ma, T. Y., Zou, W. Q., Dai, L., Tian, X. Y., et al. (2021). 1,2,4-Oxadiazole-Based Bio-Isosteres of Benzamides: Synthesis, Biological Activity and Toxicity to Zebrafish Embryo. Int. J. Mol. Sci. 22, 2367. doi:10.3390/ijms22052367
Zan, N. N., Li, J., He, H. F., Hu, D. Y., and Song, B. A. (2021). Discovery of Novel Chromone Derivatives as Potential Anti-TSWV Agents. J. Agric. Food. Chem. 69, 10819–10829. doi:10.1021/acs.jafc.1c03626
Keywords: plant-parasitic nematodes, chalcone, 1, 2, 4-oxadiazole, nematocidal activity, antiviral activity
Citation: Luo L, Liu D, Lan S and Gan X (2022) Design, Synthesis, and Biological Activity of Novel Chalcone Derivatives Containing an 1,2,4-Oxadiazole Moiety. Front. Chem. 10:943062. doi: 10.3389/fchem.2022.943062
Received: 13 May 2022; Accepted: 23 May 2022;
Published: 22 July 2022.
Edited by:
Pei Li, Kaili University, ChinaCopyright © 2022 Luo, Liu, Lan and Gan. This is an open-access article distributed under the terms of the Creative Commons Attribution License (CC BY). The use, distribution or reproduction in other forums is permitted, provided the original author(s) and the copyright owner(s) are credited and that the original publication in this journal is cited, in accordance with accepted academic practice. No use, distribution or reproduction is permitted which does not comply with these terms.
*Correspondence: Xiuhai Gan, Z3hoMjAwNzE5QDE2My5jb20=