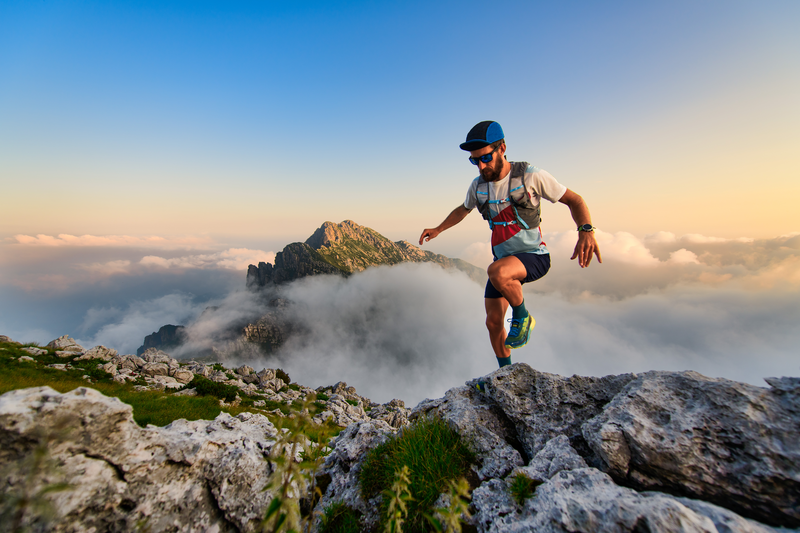
94% of researchers rate our articles as excellent or good
Learn more about the work of our research integrity team to safeguard the quality of each article we publish.
Find out more
ORIGINAL RESEARCH article
Front. Chem. , 25 November 2022
Sec. Organic Chemistry
Volume 10 - 2022 | https://doi.org/10.3389/fchem.2022.1089860
This article is part of the Research Topic Synthesis and Application of Organophosphorus Compounds View all 6 articles
Herein, we report an efficient and simple copper-catalyzed oxidative diarylthiolation of maleimides with sulfur powder and aryl boronic acids, in which S powder was used as a substrate and internal oxidant. The corresponding double C-S bonds coupling products were obtained in moderate to high yields under a simple catalytic system. Mechanistic studies indicated that copper-catalyzed radical thiolation of aryl boronic acids with S powder, and the resulting arylthiyl underwent radical addition with double bonds of maleimides.
From frequently-used chelating ligands and promising bioactive compounds to wonderful organic electrode materials, vicinal diarylthiolated alkenes all play extremely prominent roles in different scientific fields (Gerken et al., 2020; Ryu et al., 2007). Transition-metal catalyzed cis-addition of disulfides to terminal alkynes is a reliable and efficient method for the construction of these prevalent skeletons with the formation of double C-S bonds (Ananikov et al., 2004; Ananikov et al., 2008; Wei et al., 2020; Arisawa et al., 2001; Cai et al., 2007; Kuniyasu et al., 1991; Sartori et al., 2013; Gao et al., 2022; Yang, 2019i) (Scheme 1A). Recently, a three-component cascade diarylthiolation using the methods of inputting S elemental was developed. Jiang has demonstrated palladium-catalyzed cascade diarylthiolation of terminal alkynes, K2S and diaryliodonium salts (Li et al., 2018). Arylhydrazines could be converted into functionalized diarylthiolated alkenes by palladium-catalyzed oxidative coupling method and Na2S2O3 reagent was employed as a sulfur source (Lai et al., 2021). Furthermore, a facile metal-free preparation of 1,2-diarylthiolated styrenes by the reaction of thiocyanate with terminal alkynes has also been developed (Scheme 1B). Although a handful of strategies have been developed toward molecular diversification, however, expensive transition-metal catalysts, pre-preparation of raw materials and stoichiometric amounts of H2O2 oxidant are required (Li et al., 2013). To the best of our knowledge, oxidative diarylthiolation of internal alkenes is still an important but unresolved challenge (Kodama et al., 2007), which is due to the steric hindrance environment between internal C=C bonds.
Maleimide is widely employed to conjugate with thiol groups through S-Michael addition reactions. The resulting thiolated maleimide has unique biological activities and is commonly used as a useful handle in organic synthesis (Morais et al., 2017). Recently, Zhao has developed copper-catalyzed sulfenylation of maleimides with thiols to give 3-thiomaleimides with the addition of fluoroboric acid (Yang et al., 2016). However, this transformation could not be utilized to access 3,4-diarylthiolated maleimides, even in the presence of excess thiols or a step-by-step strategy. The main intrinsic reason is that the introduction of the thio group would dramatically reduce the electrophilicity of maleimide, thus preventing the further S-Michael addition with another nucleophilic thiol. Inspired by the free radical cyclization of maleimides (Zhu et al., 2019), we envisioned the radical cascade diarylthiolation, that is, the in-situ generated arylthiyl radical intermediates which initiated from the copper-catalyzed thiolation of aryl boronic acids with sulfur powder. Then, the resulting arylthiyl went through radical nucleophilic addition/radical-radical cross-coupling/oxidative dehydrogenation steps with maleimides to achieve alkene diarylthiolation (Scheme 1C). This three-component radical diarylthiolation protocol not only provides a concise pathway to important 3,4-diarylthiolated maleimides (Palanki et al., 2013; Jones et al., 2012), but also explores the chemical reactivity of S powder.
To test our assumptions, we set out to study the radical cascade reaction of N-phenyl maleimide with sulfur powder and phenylboronic acid (Table 1). After screening the common reaction parameters, when the reaction was stirred in the presence of 10% CuI in DMSO, at 100°C under air atmosphere, desired product 3a was obtained with exclusive diarylthiolation and promising chemoselectivity. In addition, no byproducts of mono-arylthiolated or S-Michael addition byproducts were observed (entry 1). Other copper salts such as CuCl and Cu(OAc)2 gave relatively lower yields (entries 2, 3). Next, we tried to explore the effect of ligands, which have been well demonstrated in copper-catalyzed radical reaction systems (Su et al., 2015). But it is regrettable that the use of nitrogen-based ligands such as phen and trans-(1R, 2R) N,N′-dimethyl-cyclohexane-1,2-diamine, or bidentate phosphine ligands such as XantPhos and Binap, maleimide diarylthiolation was completely inhibited (entries 4-7). Fortunately, in the case of PCy3 as a ligand, the yield of the diarylthiolation product increased (entry 8). Therefore, we examined other monodentate phosphines. To our delight, XPhos could give promising reactivity, while DavePhos and SPhos show slightly lower performance (entries 9-11). The wise choice of solvent had a significant influence on the transformation. Only DMSO could give the desired product. In contrast, the reactions were completely shut down when other solvents were used (entries 12-14). Notably, lowering the reaction temperature to 90°C dramatically reduced the yield of 3a (entry 15). Finally, when the reaction was exposed to O2 atmosphere, the reaction efficiency was improved, and 77% yield of 3a could be obtained (entry 16). It is worth noting that there’s no reaction at all under nitrogen atmosphere (entry 17).
TABLE 1. Reaction optimizationa.
Firstly, under the optimal reaction conditions, we synthesized various diarylthiolated maleimides with a variety of aryl boronic acids (Scheme 2). Generally, aryl boronic acids with different critical functional groups, whether electron-donating methyl (3b), phenoxyl (3n) and methoxyl (3o), or electron-deficient fluoro (3c), trifluoromethyl (3i) and ester (3j) were all competent substrates and facilely converted into the expected products. Surprisingly, the iodo and silicon functional groups (3h, 3L) frequently applied in copper-catalyzed cross-coupling reactions are also compatible with our standard reaction conditions, which provide a great opportunity for further derivations of the target product. Interestingly, mesityl boronic acid (3p) with large steric hindrance also successfully participated in multi-component reactions and gave the corresponding product. This experimental phenomenon is consistent with the tendency of chloro atom at different positions of aryl boronic acid (3d-3f).
SCHEME 2. Scope of arylboronic acidsa. a) Reaction conditions unless specified otherwise: 1a (0.2 mmol), sulfur powder (0.6 mmol), 2 (0.6 mmol), CuI (10 mol%) and XPhos (12 mol%) in DMSO (2.0 ml) were stirred at 100°C under O2 atmosphere for 24 h, isolated yields are given.
Then, significant efforts were dedicated to expand the range of maleimides with different structures (Scheme 3). On the whole, unprotected and N-benzylated maleimides effectively participated in this transformation and the desired products were accessed in good yields. Although the yield of 4a was slightly lower, it confirmed that maleimide was tolerant under current reaction conditions. The established reaction conditions do not influence usually important functional groups, thus leaving an important space for further late-stage functionalization through mature cross-coupling reactions. It was found that thiophene-substituted maleimide was feasible, and the expected product 4k could be isolated in good yield.
SCHEME 3. Scope of maleimidesa. a) Reaction conditions unless specified otherwise: 1 (0.2 mmol), sulfur powder (0.6 mmol), 2a (0.6 mmol), CuI (10 mol%) and XPhos (12 mol%) in DMSO (2.0 ml) were stirred at 100°C under O2 atmosphere for 24 h, isolated yields are given.
Unsurprisingly, multiple halogenated maleimide derivatives worked well using this protocol, providing 4L with 60% yield. Most importantly, the newly developed radical diarylthiolation strategy was well proved in two-fold reactions, 4m, 4n and 4o were provided in good yield. The above results demonstrated the distinctive advantage of our double C-S bonds formation protocol. Unfortunately, when we extended the substrates to other alkenes, such as styrene and methyl acrylate, no products were observed. We also screened copper catalysts, ligands, reaction solvents and reaction temperatures, but no beneficial results were obtained. These results in turn confirmed the unique chemical reactivity of maleimide.
Control experiments were performed to elucidate the detailed mechanism of copper-catalyzed diarylthiolation of maleimides (Scheme 4). As expected, the addition of TEMPO promptly extinguished the transformation (Eq. 1), which indicates that a multi-component reaction occurs through a radical reaction. The mixture of N-methyl maleimide with diphenyl disulfide did not provide the oxidative thiolated product (Eq. 2), which suggests that disulfides were not competent intermediate. In the reaction of sulfur powder and phenyl boronic acid under the optimized reaction conditions (Eq. 3), a mixture of diphenyl disulfide and biphenyl were detected by HRMS, this result showed that aryl and thiyl radical were formed during the reaction progress. Surprisingly, the reaction mentioned above was conducted in the absence of copper salt (Eq. 4), a large amount of biphenyl is still obtained and confirmed by HRMS. This reaction shows that phenylboronic acid was oxidized by sulfur powder to form phenyl radicals. In general, sulfide anions and high-valence sulfates could be formed by the disproportionation of sulfur powder under base conditions. However, our reaction conditions are almost neutral. When Na2S was used as a sulfur source in reaction mixture (Eq. 5), 3a product was not observed on TLC. Although the exact mechanism is not clear at the present research stage, S powder does play the role of substrate and internal oxidant in this transformation. Finally, the reaction did not occur when a mixture of 3-((4-fluorophenyl)thio)-1-phenyl-1H-pyrrole-2,5-dione, S power and phenylboronic acid (Eq. 6), the step-by-step oxidation thiolation of maleimides could be excluded. We also tried to use ArSH or ArSCu as substrate, however, no arylthiolation reaction was observed in both cases (Eqs 7, 8). According to this mechanistic investigation and previous related literature, a reasonable mechanism was proposed in Scheme 4. S powder undergoes disproportionation to produce sulfide and sulfate (Zhang et al., 2014), the former coordinated with copper salts to deliver sulfur copper species (Ravi et al., 2016), the latter oxidizes the aryl boronic acids to obtain aryl radicals (Dutta et al., 2020). The formed aryl radicals would preferentially react with sulfur copper to give arylthiyl radical (Jiang et al., 2009), and then nucleophilic addition with maleimide provided the carbon radical A. Subsequently, the highly selective capture reaction between A and arylthiyl radical afforded intermediate B, followed by copper-catalyzed oxidative dehydrogenation step to give the anticipated product.
In addition, we further determined the cytotoxicity of these 27 compounds on human lung cancer cells (Figure 1), H520 and H1299 were treated with these compounds at the same concentration (20 μM) for 48 h. As shown in Figure 1, 17 of these compounds can reduce cell viability of H520 cells to less than fifty percent. As for H1299 cells, there are 25 compounds can reduce cell viability to below 50%. Remarkably, 4c (H520 IC50 = 10.1μM; H1299 IC50 = 10.5 μM), 4a (H520 IC50 = 10.4μM; H1299 IC50 = 9.98 μM) and 4h (H520 IC50 = 10.2μM; H1299 IC50 = 11.1 μM) displayed potent cell growth inhibition activity of H520 and H1299. Then, we explored the relationship between toxicity and concentration of these three compounds. The results showed that treatment with these compounds caused a dose-dependent increase in cytotoxicity.
In conclusion, we have established a concise and feasible protocol to prepare diarylthiolated maleimides through copper-catalyzed radical cascade reaction of maleimides with S powder and aryl boronic acids. This novel transformation consists of single-electron oxidation of aryl boronic acids by sulfur powder, radical diarylthiolation and oxidative dehydrogenation steps. Sulfur powder is not only used as a substrate of multi-component thiolation reaction, but also work as an internal oxidant. The prepared 3,4-diarylthiolated maleimides, such as 3c, 4a and 4h showed excellent anticancer of human lung cancer cells (H520 and H1299).
Typan blue was purchased from Solarbio (Beijing, China), the human lung cancer H520 and H1299 cell lines were purchased from the Cell Bank of Type Culture Collection of the Chinese Academy of Sciences, Shanghai, China. The cells were routinely cultured in RPMI 1640 medium (Gibco, Eggenstein, Germany) containing 10% heat-inactivated fetal bovine serum. Cells were propagated in a humidified cell incubator with an atmosphere of 5% CO2 at 37°C.
H520 and H1299 cell lines were seeded in 6-well plates and incubated overnight in the incubator with an atmosphere of 5% CO2 at 37°C. The cells were then treated with different compounds for 48 h. After that, the cell viability was determined using trypan blue exclusion and then indicated the percentage of viable cells relative to DMSO-treated cells.
The cells were seeded in 6-well plates and incubated overnight in the incubator with an atmosphere of 5% CO2 at 37°C. After cultured for 1 week, the cells were washed with phosphate-buffered saline (PBS) twice when visible colonies were formed. After being fixed with 4% paraformaldehyde for 20 min, cells were washed with PBS and stained for 15 min. At last, cells were washed with PBS twice and the colonies were counted under ordinary optical microscope.
The original contributions presented in the study are included in the article/Supplementary Material, further inquiries can be directed to the corresponding author.
LW, ZL, ZM, KX, and WW performed this project. WY prepared and revised this manuscript. LW and ZL these authors contributed equally to this work.
Financial support from the Project of Jilin Provincial Health Commission (2020G009) is greatly appreciated.
The authors declare that they have no known competing financial interests or personal relationships that could have appeared to influence the work reported in this paper.
All claims expressed in this article are solely those of the authors and do not necessarily represent those of their affiliated organizations, or those of the publisher, the editors and the reviewers. Any product that may be evaluated in this article, or claim that may be made by its manufacturer, is not guaranteed or endorsed by the publisher.
The Supplementary Material for this article can be found online at: https://www.frontiersin.org/articles/10.3389/fchem.2022.1089860/full#supplementary-material
Ananikov, V. P., and Beletskaya, I. P. (2004a). Palladium-catalyzed addition of disulfides and diselenides to alkynes under solvent free conditionsElectronic supplementary information (ESI) available: Full experimental details of synthetic procedure, compound separation and purification, details of spectroscopic studies, kinetic measurements and compound characterization. See http://www.rsc.org/suppdata/ob/b3/b312471a/. Org. Biomol. Chem. 2, 284–287. doi:10.1039/B312471A
Ananikov, V. P., Gayduk, K. A., Beletskaya, I. P., Khrustalev, V. N., and Antipin, M. Y. (2008b). Remarkable ligand effect in Ni- and Pd-Catalyzed bisthiolation and bisselenation of terminal alkynes solving the problem of stereoselective dialkyldichalcogenide addition to the C≡C bond. Chem. Eur. J. 14, 2420–2434. doi:10.1002/chem.200701278
Arisawa, M., and Yamaguchi, M. (2001d). Addition reaction of dialkyl disulfides to terminal alkynes catalyzed by a rhodium complex and trifluoromethanesulfonic acid. Org. Lett. 3, 763–764. doi:10.1021/ol015521l
Cai, M., Wang, Y., and Hao, W. (2007e). Palladium-catalyzed addition of diaryl disulfides and diselenides to terminal alkynes in room temperature ionic liquids. Green Chem. 9, 1180–1184. doi:10.1039/B706320B
Dutta, N. B., Bhuyana, M., and Baishy, G. (2020). K2S2O8 mediated C-3 arylation of quinoxalin-2(1H)-ones under metal-photocatalyst- and light-free conditions. RSC Adv. 10, 3615–3624. doi:10.1039/D0RA00013B
Gao, B., Jiang, T., Yang, R., Yan, Q., Liu, X., Xie, Q., et al. (2022h). Palladium-catalyzed highly chemo- and stereoselective bisthiolation of terminal alkynes with allyl phenyl sulfides via C–S Bond cleavage. J. Org. Chem. 87, 7895–7904. doi:10.1021/acs.joc.2c00545
Gerken, J. B., Stamoulis, A., Suh, S.-E., Fischer, Nicholas D., Kim, Y. J., Guzei, I. A., et al. (2020a). Efficient electrochemical synthesis of robust, densely functionalized water soluble quinones. Chem. Commun. 56, 1199–1202. doi:10.1039/C9CC08878D
Jiang, Y., Qin, Y., Xie, S., Zhang, X., Dong, J., and Ma, D. (2009). A general and efficient approach to aryl thiols: CuI-Catalyzed coupling of aryl iodides with sulfur and subsequent reduction. Org. Lett. 11, 5250–5253. doi:10.1021/ol902186d
Jones, M. W., Strickland, R. A., Schumacher, F. F., Caddick, S., Baker, J. R., Gibson, M. I., et al. (2012b). Highly efficient disulfide bridging polymers for bioconjugates from radical-compatible dithiophenol maleimides. Chem. Commun. 48, 4064–4066. doi:10.1039/C2CC30259D
Kodama, S., Nishinaka, E., Nomoto, A., Sonoda, M., and Ogawa, A. (2007). A novel palladium(0)-catalyzed addition of diphenyl disulfide to allenes leading to vicinal disulfides and its application to carbonylation with carbon monoxide. Tetrahedron Lett. 48, 6312–6317. doi:10.1016/j.tetlet.2007.07.019
Kuniyasu, H., Ogawa, A., Miyazaki, S., Ryu, I., Kambe, N., and Sonoda, N. (1991f). Palladium-catalyzed addition and carbonylative addition of diaryl disulfides and diselenides to terminal acetylenes. J. Am. Chem. Soc. 113, 9796–9803. doi:10.1021/ja00026a013
Lai, Y.-L., Yan, S., He, D., Zhou, L.-Z., Chen, Z.-S., Dua, Y.-L., et al. (2021). Palladium-catalyzed bisthiolation of terminal alkynes for the assembly of diverse (Z)-1, 2-bis(arylthio)alkene derivatives. RSC Adv. 11, 28447–28451. doi:10.1039/D1RA05773A
Li, J., Huang, H., Liang, W., Gao, Q., and Duan, Z. (2013). Catalytic C-H and C-S bond activation of thiophenes. Org. Lett. 15, 282–285. doi:10.1021/ol303136x
Li, J., Li, C., Ouyang, L., Li, C., Yang, S., Wu, W., et al. (2018). Palladium-catalyzed regioselective three-component cascade bisthiolation of terminal alkynes. Adv. Synth. Catal. 360, 1138–1150. doi:10.1002/adsc.201701417
Morais, M., Nunes, J. P. M., Karu, K., Forte, N., Benni, I., Smith, M. E. B., et al. (2017). Optimisation of the dibromomaleimide (DBM) platform for native antibody conjugation by accelerated post-conjugation hydrolysis. Org. Biomol. Chem. 15, 2947–2952. doi:10.1039/C7OB00220C
Palanki, M. S. S., Bhat, A., Bolanos, B., Brunel, F., Rosario, J. D., Dettling, D., et al. (2013a). Development of a long acting human growth hormone analog suitable for once a week dosing. Bioorg. Med. Chem. Lett. 23, 402–406. doi:10.1016/j.bmcl.2012.11.104
Ravi, C., Reddy, N. N. K., Pappula, V., Samanta, S., and Adimurthy, S. (2016). Copper-catalyzed three-component system for arylsulfenylation of imidazopyridines with elemental sulfur. J. Org. Chem. 81, 9964–9972. doi:10.1021/acs.joc.6b01715
Ryu, C.-K., Park, R.-E., Ma, M.-Y., and Nho, J.-H. (2007b). Synthesis and antifungal activity of 6-arylamino-phthalazine-5, 8-diones and 6, 7-bis(arylthio)-phthalazine-5, 8-diones. Bioorg. Med. Chem. Lett. 17, 2577–2580. doi:10.1016/j.bmcl.2007.02.003
Sartori, G., Neto, J. S. S., Pesarico, A. P., Back, D. F., Nogueira, C. W., and Zeni, G. (2013g). Bis-vinyl selenides obtained via iron(iii) catalyzed addition of PhSeSePh to alkynes: Synthesis and antinociceptive activity. Org. Biomol. Chem. 11, 1199–1208. doi:10.1039/C2OB27064A
Su, W., Gong, T.-J., Lu, X., Xu, M.-Y., Yu, C.-G., Xu, Z.-Y., et al. (2015). Ligand-controlled regiodivergent copper-catalyzed alkylboration of alkenes. Angew. Chem. Int. Ed. 54, 12957–12961. doi:10.1002/anie.201506713
Wei, C., He, Y., Wang, J., Ye, X., Wojtas, L., and Shi, X. (2020c). Hexafluoroisopropanol-promoted disulfidation and diselenation of alkyne, alkene, and allene. Org. Lett. 22, 5462–5465. doi:10.1021/acs.orglett.0c01834
Yang, L., Tian, Z.-Y., and Zhang, C.-P. (2019i). Transition-metal-free selective synthesis of (Z)-1, 2-diarylthio-1-arylalkenes, (2-arylethene-1, 1, 2-triyl)tris(arylsulfane)s and alkynyl sulfides from thiocyanates and terminal arylalkynes. ChemistrySelect 4, 311–315. doi:10.1002/slct.201803719
Yang, Z.-H., An, Y.-L., Chen, Y., Shao, Z.-Y., and Zhao, S.-Y. (2016). Copper (I) iodide-catalyzed sulfenylation of maleimides and related 3-indolylmaleimides with thiols. Adv. Synth. Catal. 358, 3869–3875. doi:10.1002/adsc.201600812
Zhang, G., Yi, H., Chen, H., Bian, C., Liu, C., and Lei, A. (2014). Trisulfur radical anion as the key intermediate for the synthesis of thiophene via the interaction between elemental sulfur and NaOtBu. Org. Lett. 16, 6156–6159. doi:10.1021/ol503015b
Keywords: radical thiolation, copper-catalyzed, sulfur powder, maleimides, bisthiolation
Citation: Wang L, Li Z, Ma Z, Xia K, Wang W and Yu W (2022) Anticancer-active 3,4-diarylthiolated maleimides synthesis via three-component radical diarylthiolation. Front. Chem. 10:1089860. doi: 10.3389/fchem.2022.1089860
Received: 04 November 2022; Accepted: 11 November 2022;
Published: 25 November 2022.
Edited by:
Ge Wu, Wenzhou Medical University, ChinaReviewed by:
Zu-Li Wang, Qingdao Agricultural University, ChinaCopyright © 2022 Wang, Li, Ma, Xia, Wang and Yu. This is an open-access article distributed under the terms of the Creative Commons Attribution License (CC BY). The use, distribution or reproduction in other forums is permitted, provided the original author(s) and the copyright owner(s) are credited and that the original publication in this journal is cited, in accordance with accepted academic practice. No use, distribution or reproduction is permitted which does not comply with these terms.
*Correspondence: Wenchang Yu, MTE1MTYxNDMyMkBxcS5jb20=
†These authors contributed equally to this work.
Disclaimer: All claims expressed in this article are solely those of the authors and do not necessarily represent those of their affiliated organizations, or those of the publisher, the editors and the reviewers. Any product that may be evaluated in this article or claim that may be made by its manufacturer is not guaranteed or endorsed by the publisher.
Research integrity at Frontiers
Learn more about the work of our research integrity team to safeguard the quality of each article we publish.