- School of Engineering, Apadana Institute of Higher Education, Shiraz, Iran
A four-component domino Michael–Mannich cyclocondensation of amines, dialkyl acetylenedicarboxylaes, and formaldehyde was utilized to develop a green technique for sans metal combination of polyfunctionalized dihydro-2-oxypyrroles. It involves visible light as an environmentally friendly power source and acridine yellow G (AYG) as a photo-induced electron transfer (PET) photocatalyst. The motivation behind this examination was to expand the utilization of a non-metal dye that is both reasonable and broadly accessible. Photochemically catalyzed AYG flaunts exceptional returns, energy effectiveness, and natural agreeableness, as well as extraordinary iota economy, efficient highlights, and comfort of purpose. Key abilities consist of an easy experimental setup, big substrate tolerance, finance-friendly, clean painting-up strategies within the absence of tedious separation techniques, and minimized the quantity of waste for each organic transformation. The type of yields is pretty uniform (85–97%, average 92.09%), and the shape of reaction times might be very speedy (15–30 min, average 21.59 min), and the factor stated inside the dialogue is that the method tolerates quite a number electron-donating and electron-withdrawing functional groups, while, however, giving extremely good yields. The response within the reason is insensitive to the person of the substituents. Subsequently, many compounds and natural factors can be followed over the course of time. Shockingly, gram-scale cyclization is conceivable, proposing that the strategy could be utilized in industry.
Introduction
Because of retaining light in the noticeable scope of the electromagnetic range, photo-redox catalyst fosters their stable photoexcited states (Patel et al., 2021). Various flow reactors (Politano and Oksdath-Mansilla, 2018) including atom inexpensive, green, and efficient processes, have been constructed using visible light and dual photosensitized electrochemical methods (Verschueren and de Borggraeve, 2019), (Patel et al., 2021). Acridine yellow G (Figure 1) is a highly fluorescent dye that is widely used in cytology to stain cells (Tangelder et al., 1995; Teuber et al., 2001), DNA staining in chromatography (Vincent and Goldstein, 1981), fluorescent markers (Goryacheva et al., 2000), spectrophotometry to identify residues of impurities (Pérez-Ruiz et al., 1997; Pérez-Ruíz et al., 2003), and photocatalysis (Takemura, 1962; Amat et al., 2007; Arques et al., 2009). AYG has been shown to have photosensitizing (Saint-Cricq et al., 2012) and photodynamic (antibacterial) (Webb et al., 1979) effects (Kostjukova et al., 2021).
As a result of its enormous energy holds, modest expense, and sustainable power sources, green physicists believe noticeable light illumination to be a dependable innovation for harmless to the ecosystem compound combination (Mohamadpour, 2020a; Mohamadpour, 2021a; Mohamadpour, 2021b).
The designs that make up pyrrole subordinates have aroused the curiosity of chemists because of their organic and pharmacological impacts (Lampe et al., 1993; Shiraki et al., 1995; Singh et al., 1995; Borthwick et al., 2002; Chen et al., 2005; Alp et al., 2010). There are numerous options to synthesize polyfunctionalized dihydro-2-oxypyrroles, including I2 (Khan et al., 2012), glycine (Mohamadpour, 2020b), AcOH (Zhu et al., 2009), Cu(OAc)2.H2O (Lv et al., 2013), Fe3O4@nano-cellulose–OPO3H (Salehi and Mirjalili, 2017), tartaric acid (Mohamadpour et al., 2017), nano-Fe3O4@SiO2/SnCl4 (Mirjalili et al., 2019), glutamic acid (Mohamadpour, 2019a), graphene oxide (Bavadi and Niknam, 2018), caffeine (Mohamadpour, 2019b), 2,6-pyridinedicarboxylic acid (Khan et al., 2016), saccharin (Mohamadpour et al., 2016), BiFeO3 nanoparticles (Singh and Rajput, 2018), and CoFe2O4@SiO2@IRMOF-3 (Zhang et al., 2017). In order to manufacture heterocyclic compounds, we investigated photocatalysts (Mohamadpour, 2021c; Mohamadpour, 2021d; Mohamadpour, 2021e; Mohamadpour, 2022) in the green medium. This concentrate likewise tells the best way to utilize a photo-redox catalyst that is modest and broadly accessible. The photochemical mechanism by which AYG works as a photo-induced electron transfer (PET) photocatalyst has already been reported (Sengupta et al., 2018). Visible light facilitates the Michael–Mannich cyclocondensation of amines, dialkyl acetylenedicarboxylaes, and formaldehyde in ethanol at rt. This reaction was carried out at high speed and yielded.
Experimental
General procedure
A solution of amine 1 (1.0 mmol) and dialkyl acetylenedicarboxylate 2 (1.0 mmol) in EtOH (3 ml) was agitated for 15 min in the presence of AYG (1.5 mol%) under blue LED (12 W) irradiation at rt. The reaction mixture was then stirred at rt while the amine 3 (1.0 mmol) and formaldehyde 4 (1.5 mmol) were added. TLC was used to track the response. Thin layer chromatography (TLC) was carried out with silica gel as the stationary phase utilizing EtOAc/n-hexane (1:2) as an eluent. After the reaction, the resulting product was screened and washed with ethanol to produce the pure chemical without additional purification. Regardless of whether we could blend the previously mentioned synthetic substances utilizing gram-scale advancements, we needed to check whether we could increase to the level expected for drug process R&D. In one examination, 50 mmol aniline, 37.5 mmol formaldehyde, and 25 mmol diethyl acetylenedicarboxylate (DEAD) were used. The enormous scope response proceeded according to the plan, taking simply 20 min to complete, and then the item was accumulated utilizing standard filtration techniques. This substance’s 1HNMR range demonstrates that it is spectroscopically unadulterated. The items were ordered subsequently looking at spectroscopic information (1HNMR). For this composition, the spectroscopic information is given in the Supporting Information file.
Results and discussion
To start, the condensation of formaldehyde, aniline, and dimethyl acetylenedicarboxylate (DMAD) is investigated. The reaction was carried out at room temperature, in EtOH (3 ml), and utilizing LED light. In the absence of a photocatalyst, a trace amount of product was produced. Acridine yellow G, xanthene, riboflavin, 9H-xanthen-9-one, rhodamine B, alizarin, phenanthrenequinone, acenaphthenequinone, rose bengal, erythrosin B, and fluorescein were all attempted in the identical settings to improve the response. With yields ranging from 42 to 97%, this reaction generated the approved matching product 5a. As per the discoveries, AYG performed better in such a response. The yield was expanded to 97% utilizing 1.5 mol% AYG (Table 1, entry 3). H2O, DMF, DMSO, THF, DCM, toluene, and solvent-free conditions generally brought about lower item yields, as displayed in Table 2. In MeOH, EtOAc, and CH3CN, the yield and reaction rate improve. The reaction occurred in EtOH with a high rate and yield. Under indistinguishable circumstances, a yield of 97% was delivered, as displayed in Table 2. A variety of light sources were used to investigate how blue light influences yield. There was a minuscule measure of 5a without utilizing the light source, as per the test control. As per the discoveries, light and AYG are expected for fruitful amalgamation of item 5a. Blue LED intensity changes were also utilized to determine the ideal settings. The best outcomes, as indicated by the analysts, were obtained when blue LED (12 W) was utilized (Table 2, entry 10). Many substrates were tried under ideal circumstances (Table 3 and Scheme 1). It is vital to take note that the aniline substituent significantly affected the response’s result (Table 3). Both electron-donating and electron-withdrawing functional groups functioned admirably. The yield of all aliphatic and benzylic amines is incredibly high. The reaction patterns of dimethyl acetylenedicarboxylate (DMAD) and diethyl acetylenedicarboxylate (DEAD) were similar.
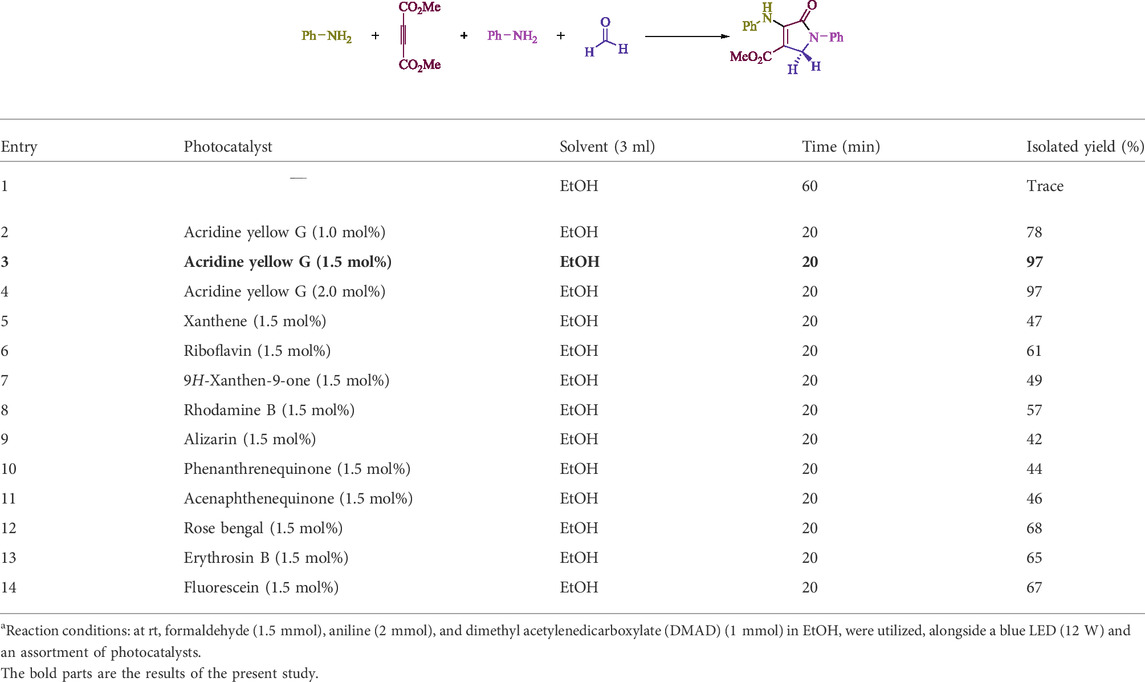
TABLE 1. Photocatalyst optimization table is supplied for 5a productiona.
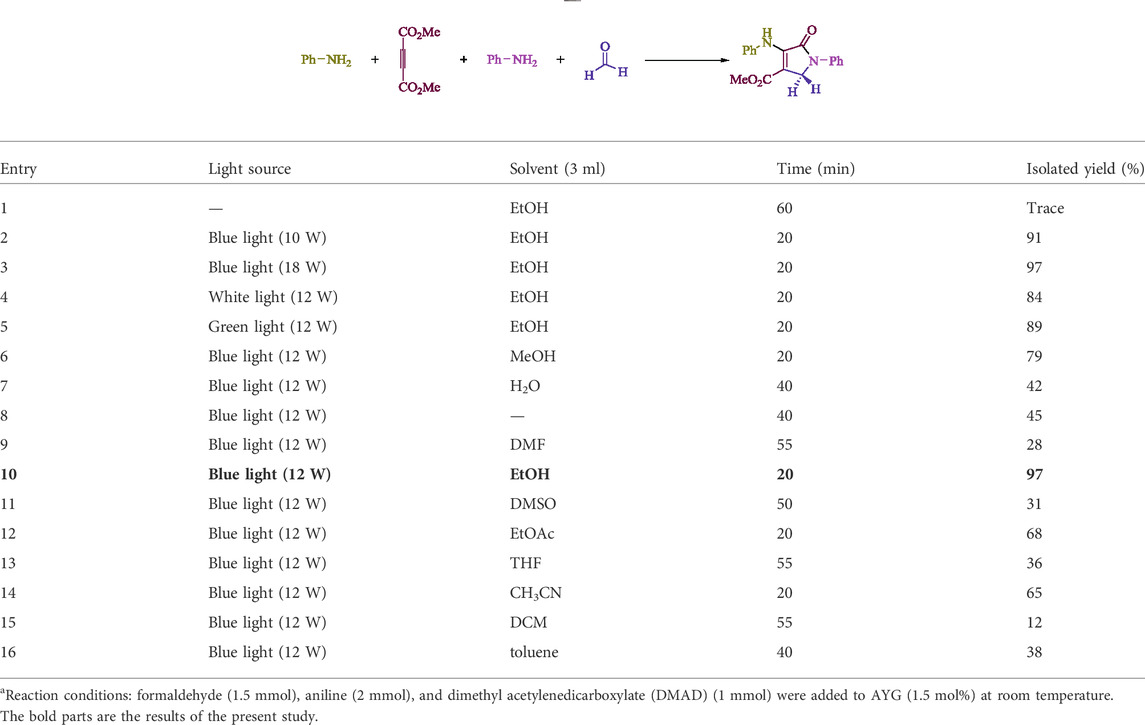
TABLE 2. Table of solvent and visible light optimization is provided for 5a synthesisa.
Table 4 additionally remembers information for turnover frequency (TOF) and turnover number (TON). The higher the TON and TOF mathematical qualities, the less the catalyst is utilized and the more prominent the yield gets, and as the worth ascents, the impetus turns out to be more viable.
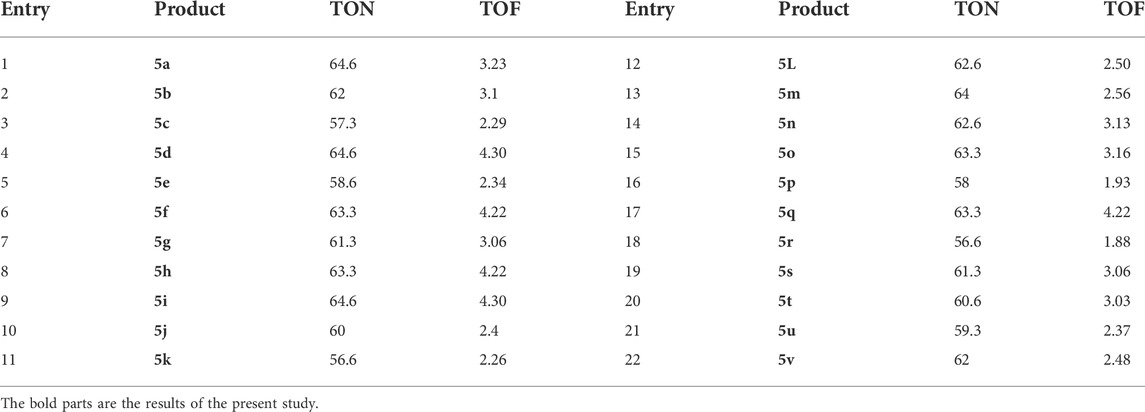
TABLE 4. For polyfunctionalized dihydro-2-oxypyrroles, the determined turnover number (TON) and the turnover frequency (TOF).
To acquire knowledge of the response system of this noticeable light-advanced four-component condensation, various control tests were performed. As displayed in Scheme 2, the condensation of aniline 3) with formaldehyde 4) was performed under standard circumstances (AYG in EtOH under blue LED) with the end of H2O to get the related imine (I). When dimethyl acetylenedicarboxylate (DMAD) 2) was responded with formaldehyde 4) under indistinguishable response conditions, no item was created. For the condensation of imine (I) and enamine radical (II), the yield for 5a was 97%. A trace of the corresponding product 5a was obtained when the reaction was carried out in the dark. Scheme 3 provides a possible reaction route in the presence of AYG after reviewing the findings of this experiment.
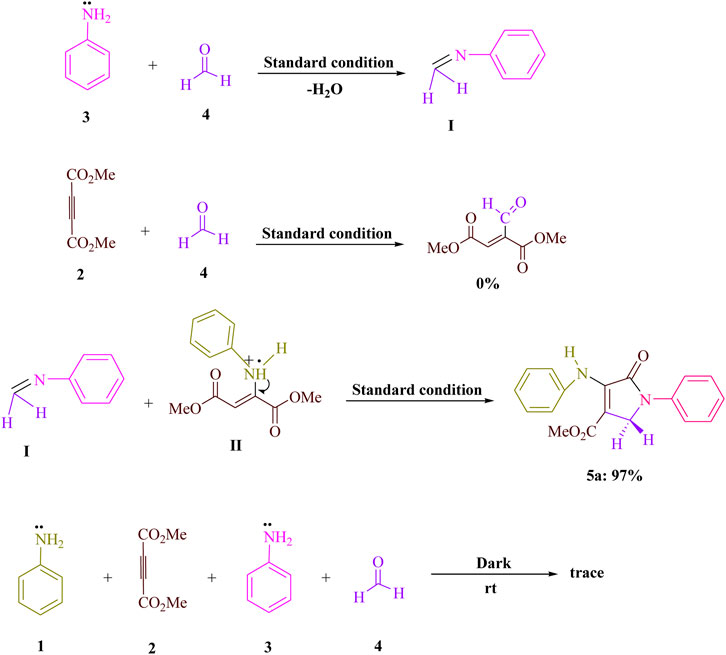
SCHEME 2. Significant control reads up for grasping the component of formaldehyde (4, 1.5 mmol), dimethyl acetylenedicarboxylate (DMAD) (2, 1 mmol), and aniline (1 and 3, 2 mmol) condensations.
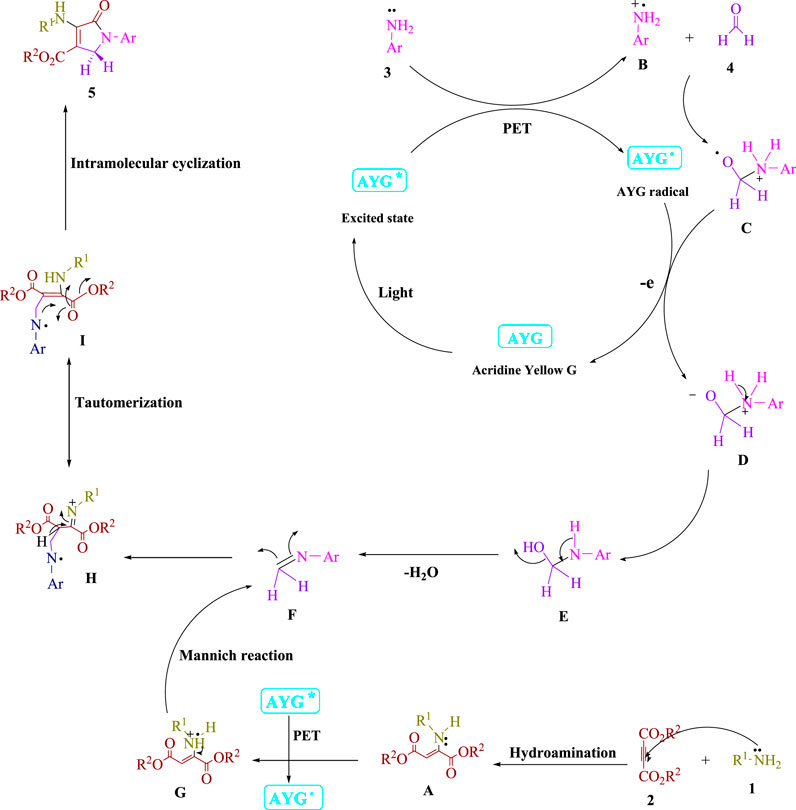
SCHEME 3. Manufacture of polyfunctionalized dihydro-2-oxypyrroles was described using a mechanistic process.
The recommended technique is depicted in Scheme 3. This broadly accessible AYG utilizes noticeable light as a wellspring of sustainable power to develop reactant frameworks that use the PET pathway. This process can be accelerated with visible light energy. Enamine (A) is produced through the Michael reaction between amine 1) and dialkyl acetylenedicarboxylate (2). To boost the visible-light-induced AYG*, the aniline radical (B) is produced utilizing a PET method and visible light irradiation. After that, the radical cation (B) reacts with formaldehyde 4) to create a radical cation (C). The electron transfer (ET) process between the radical adduct (C) and the AYG radical produces the intermediate (D) and ground-state AYG. Then, from (E), an H2O molecule is removed, leaving intermediate (F). To boost the visible-light-induced AYG*, the enamine radical (G) is produced utilizing a PET approach. A Mannich reaction occurs between an activated imine (F) and an enamine radical (G), resulting in an intermediate (H) that changes into a more stable tautomeric form (I). In the final phase, the intramolecular cyclization in intermediate (I) tautomerizes into polyfunctionalized dihydro-2-oxypyrroles (5).
Conclusion
A radical synthesis of polyfunctionalized dihydro-2-oxypyrroles utilizing AYG dye as a photo-induced electron transfer photocatalyst was studied. Visible light is used as a renewable energy source in an ethanol solution at room temperature in an air environment. The utilization of a minimal amount of photocatalyst, brilliant yields, a response with high proficiency, stable response conditions, and a renewable energy source, and a fast methodology without the utilization of harmful solvents or impetuses are the clearest benefits of this green convention. Chromatographic purging was not needed. As per a multigram scale response of model substrates, this response can be increased without compromising the result. Therefore, this innovation offers colossal advantages regarding both gathering modern requirements and settling ecological worries.
Data availability statement
The datasets presented in this study can be found in online repositories. The names of the repository/repositories and accession number(s) can be found in the article/Supplementary Material.
Author contributions
The author confirms being the sole contributor of this work and has approved it for publication.
Conflict of interest
The author declares that the research was conducted in the absence of any commercial or financial relationships that could be construed as a potential conflict of interest.
Publisher’s note
All claims expressed in this article are solely those of the authors and do not necessarily represent those of their affiliated organizations, or those of the publisher, the editors, and the reviewers. Any product that may be evaluated in this article, or claim that may be made by its manufacturer, is not guaranteed or endorsed by the publisher.
Supplementary material
The Supplementary Material for this article can be found online at: https://www.frontiersin.org/articles/10.3389/fchem.2022.1015330/full#supplementary-material
References
Alp, C., Ekinci, D., Gültekin, M. S., Şentürk, M., Şahin, E., and Küfrevioğlu, Ö. İ. (2010). A novel and one-pot synthesis of new 1-tosyl pyrrol-2-one derivatives and analysis of carbonic anhydrase inhibitory potencies. Bioorg. Med. Chem. 18, 4468–4474. doi:10.1016/j.bmc.2010.04.072
Amat, A. M., Arques, A., Galindo, F., Miranda, M. A., Santos-Juanes, L., Vercher, R. F., et al. (2007). Acridine yellow as solar photocatalyst for enhancing biodegradability and eliminating ferulic acid as model pollutant. Appl. Catal. B Environ. 73, 220–226. doi:10.1016/j.apcatb.2006.12.003
Arques, A., Amat, A. M., Santos-Juanes, L., Vercher, R. F., Marin, M. L., and Miranda, M. A. (2009). Abatement of methidathion and carbaryl from aqueous solutions using organic photocatalysts. Catal. Today 144, 106–111. doi:10.1016/j.cattod.2008.11.013
Bavadi, M., and Niknam, K. (2018). Synthesis of functionalized dihydro-2-oxopyrroles using graphene oxide as heterogeneous catalyst. Mol. Divers. 22, 561–573. doi:10.1007/s11030-017-9809-9
Borthwick, A. D., Crame, A. J., Ertl, P. F., Exall, A. M., Haley, T. M., Hart, G. J., et al. (2002). Design and synthesis of pyrrolidine-5, 5-trans-lactams (5-oxohexahydropyrrolo [3, 2-b] pyrroles) as novel mechanism-based inhibitors of human cytomegalovirus protease. 2. Potency and chirality. J. Med. Chem. 45, 1–18. doi:10.1021/jm0102203
Chen, Y., Zeng, D. X., Xie, N., and Dang, Y. Z. (2005). Study on photochromism of diarylethenes with a 2, 5-dihydropyrrole bridging unit: A convenient preparation of 3, 4-diarylpyrroles from 3, 4-diaryl-2, 5-dihydropyrroles. J. Org. Chem. 70, 5001–5005. doi:10.1021/jo050236r
Goryacheva, I. Y., Mel’nikov, G. V., and Shtykov, S. N. (2000). Acridine dyes in the triplet state as reagents for the selective luminescence determination of polycyclic aromatic hydrocarbons. J. Anal. Chem. 55, 874–878. doi:10.1007/BF02757853
Khan, A. T., Ghosh, A., and Khan, M. M. (2012). One-pot four-component domino reaction for the synthesis of substituted dihydro-2-oxypyrrole catalyzed by molecular iodine. Tetrahedron Lett. 53, 2622–2626. doi:10.1016/j.tetlet.2012.03.046
Khan, M. M., Khan, S., Iqbal, S., and Yousuf, R. (2016). Synthesis of functionalized dihydro-2-oxypyrroles and tetrahydropyridines using 2, 6-pyridinedicarboxylic acid as an efficient and mild organocatalyst. New J. Chem. 40, 7504–7512. doi:10.1039/C6NJ01170E
Kostjukova, L. O., Leontieva, S. V., and Kostjukov, V. V. (2021). The vibronic absorption spectra and electronic states of acridine yellow in aqueous solution. J. Mol. Liq. 326, 115312. doi:10.1016/j.molliq.2021.115312
Lampe, J. W., Chou, Y. L., Hanna, R. G., Di Meo, S. V., Erhardt, P. W., Hagedorn, A. A., et al. (1993). (Imidazolylphenyl) pyrrol-2-one inhibitors of cardiac cAMP phosphodiesterase. J. Med. Chem. 36, 1041–1047. doi:10.1021/jm00060a012
Lv, L., Zheng, S., Cai, X., Chen, Z., Zhu, Q., and Liu, S. (2013). Development of four-component synthesis of tetra-and pentasubstituted polyfunctional dihydropyrroles: Free permutation and combination of aromatic and aliphatic amines. ACS Comb. Sci. 15, 183–192. doi:10.1021/co300148c
Mirjalili, B. B., Araqi, R., and Mohajeri, S. A. (2019). A simple and green approach for the synthesis of substituted dihydro-2-oxypyrroles catalyzed by nano-Fe3O4@SiO2/SnCl4 superparamagnetic nanoparticles. Iran. J. Catal. 9, 11–20.
Mohamadpour, F. (2021c10.1016/j.jphotochem.20211134). A new role for photoexcited Na2 eosin Y as direct hydrogen atom transfer (HAT) photocatalyst in photochemical synthesis of dihydropyrano[2, 3-c]pyrazole scaffolds promoted by visible light irradiation under air atmosphere. J. Photochem. Photobiol. A Chem. 418, 113428. doi:10.1016/j.jphotochem.2021.113428
Mohamadpour, F. (2019b). Caffeine as a naturally green and biodegradable catalyst promoted convenient and expedient synthetic route for the synthesis of polysubstituted dihydro-2 oxypyrroles. Bull. Chem. Soc. Ethiop. 33, 149–158. doi:10.4314/bcse.v33i1.15
Mohamadpour, F. (2019a). Glutamic acid as green and bio-based α-amino acid catalyst promoted one-pot access to polyfunctionalized dihydro-2-oxypyrroles. J. Serbian Chem. Soc. 84, 1083–1092. doi:10.2298/JSC180720006M
Mohamadpour, F., Maghsoodlou, M. T., Heydari, R., and Lashkari, M. (2016). Saccharin: A green, economical and efficient catalyst for the one-pot, multi-component synthesis of 3, 4-dihydropyrimidin-2-(1H)-one derivatives and 1H-pyrazolo[1, 2-b]phthalazine-5, 10-dione derivatives and substituted dihydro-2-oxypyrrole. J. Iran. Chem. Soc. 13, 1549–1560. doi:10.1007/s13738-016-0871-5
Mohamadpour, F., Maghsoodlou, M. T., Heydari, R., and Lashkari, M. (2017). Tartaric acid: A naturally green and efficient di-functional brønsted acid catalyst for the one-pot four-component synthesis of polysubstituted dihydropyrrol-2-ones at ambient temperature. Iran. J. Sci. Technol. Trans. Sci. 41, 843–849. doi:10.1007/s40995-016-0049-0
Mohamadpour, F. (2021d). New role for photoexcited organic dye, Na2 eosin Y via the direct hydrogen atom transfer (HAT) process in photochemical visible-light-induced synthesis of spiroacenaphthylenes and 1H-pyrazolo[1, 2-b]phthalazine-5, 10-diones under air atmosphere. Dyes Pigments 194, 109628. doi:10.1016/j.dyepig.2021.109628
Mohamadpour, F. (2021e). Photoexcited Na2 eosin Y as direct hydrogen atom transfer (HAT) photocatalyst promoted photochemical metal-free synthesis of tetrahydrobenzo[b]pyran scaffolds via visible light-mediated under air atmosphere. J. Taiwan Inst. Chem. Eng. 129, 52–63. doi:10.1016/j.jtice.2021.09.017
Mohamadpour, F. (2021b). Catalyst-free and solvent-free visible light irradiation-assisted Knoevenagel–Michael cyclocondensation of aryl aldehydes, malononitrile, and resorcinol at room temperature. Monatsh. Chem. 152, 507–512. doi:10.1007/s00706-021-02763-1
Mohamadpour, F. (2021a). Catalyst-free, visible light irradiation promoted synthesis of spiroacenaphthylenes and 1H-pyrazolo[1, 2-b]phthalazine-5, 10-diones in aqueous ethyl lactate. J. Photochem. Photobiol. A Chem. 407, 113041. doi:10.1016/j.jphotochem.2020.113041
Mohamadpour, F. (2020b). Imin-based synthesis of polyfunctionalized dihydro-2-oxypyrroles catalyzed by glycine amino acid via tandem Michael–Mannich cyclocondensation reaction under ambient temperature. Res. Chem. Intermed. 46, 1931–1940. doi:10.1007/s11164-019-04072-z
Mohamadpour, F. (2022). Methylene blue as a photo-redox catalyst: The development synthesis of tetrahydrobenzo[b]pyran scaffolds via a single-electron transfer/energy transfer. Front. Chem. 10, 934781. doi:10.3389/fchem.2022.934781
Mohamadpour, F. (2020a). Visible light irradiation promoted catalyst-free and solvent-free synthesis of pyrano[2,3-d]pyrimidine scaffolds at room temperature. J. Saudi Chem. Soc. 24, 636–641. doi:10.1016/j.jscs.2020.06.006
Patel, R. I., Sharma, A., Sharma, S., and Sharma, A. (2021). Visible light-mediated applications of methylene blue in organic synthesis. Org. Chem. Front. 8, 1694–1718. doi:10.1039/D0QO01182G
Pérez-Ruiz, T., Martínez-Lozano, C., Tomás, V., and Fenoll, J. (2003). Spectrofluorimetric determination of formaldehyde by a flow-injection method based on its catalytic effect on the acridine yellow-bromate reaction. Anal. Bioanal. Chem. 375, 661–665. doi:10.1007/s00216-003-1763-y
Pérez-Ruiz, T., Martı́nez-Lozano, C., Sanz, A., and San Miguel, M. T. (1997). Flow extraction spectrophotometric method for the determination of diclofenac sodium in pharmaceutical preparations. J. Pharm. Biomed. Analysis 16, 249–254. doi:10.1016/S0731-7085(97)00028-9
Politano, F., and Oksdath-Mansilla, G. (2018). Light on the horizon: Current research and future perspectives in flow photochemistry. Org. Process Res. Dev. 22, 1045–1062. doi:10.1021/acs.oprd.8b00213
Saint-Cricq, P., Pigot, T., Blanc, S., and Lacombe, S. (2012). Selective oxidation with nanoporous silica supported sensitizers: An environment friendly process using air and visible light. J. Hazard. Mater. 211-212, 266–274. doi:10.1016/j.jhazmat.2011.09.066
Salehi, N., and Mirjalili, B. B. (2017). Synthesis of highly substituted dihydro-2-oxopyrroles using Fe3O4@nano-cellulose–OPO3H as a novel bio-based magnetic nanocatalyst. RSC Adv. 7, 30303–30309. doi:10.1039/C7RA04101B
Sengupta, C., Mitra, P., Chatterjee, S., Bhattacharjee, G., Satpati, B., and Basu, S. (2018). Photoinduced electronic interactions between acridine derivatives and small gold nanoparticles: A spectroscopic insight. J. Mol. Liq. 272, 198–208. doi:10.1016/j.molliq.2018.09.080
Shiraki, R., Sumino, A., Tadano, K. I., and Ogawa, S. (1995). Total synthesis of PI-091. Tetrahedron Lett. 36, 5551–5554. doi:10.1016/0040-4039(95)01049-N
Singh, H., and Rajput, J. K. (2018). Chelation and calcination promoted preparation of perovskite-structured BiFeO3 nanoparticles: A novel magnetic catalyst for the synthesis of dihydro-2-oxypyrroles. J. Mat. Sci. 53, 3163–3188. doi:10.1007/s10853-017-1790-2
Singh, S. B., Goetz, M. A., Jones, E. T., Bills, G. F., Giacobbe, R. A., Herranz, L., et al. (1995). Oteromycin: A novel antagonist of endothelin receptor. J. Org. Chem. 60, 7040–7042. doi:10.1021/jo00126a071
Takemura, F. (1962). Dye-sensitized photopolymerization of vinyl monomers. II. Photobleaching of acridine yellow in some vinyl monomers. Bull. Chem. Soc. Jpn. 35, 1078–1086. doi:10.1246/bcsj.35.1078
Tangelder, G. J., Janssens, C. J., Slaaf, D. W., Oude Egbrink, M. G., and Reneman, R. S. (1995). In vivo differentiation of leukocytes rolling in mesenteric postcapillary venules. Am. J. Physiology-Heart Circulatory Physiology 268, 909–915. doi:10.1152/ajpheart.1995.268.2.H909
Teuber, M., Rögner, M., and Berry, S. (2001). Fluorescent probes for non-invasive bioenergetic studies of whole cyanobacterial cells. Biochimica Biophysica Acta - Bioenergetics 1506, 31–46. doi:10.1016/S0005-2728(01)00178-5
Verschueren, R. H., and De Borggraeve, W. M. (2019). Electrochemistry and photoredox catalysis: A comparative evaluation in organic synthesis. Molecules 24, 2122–2160. doi:10.3390/molecules24112122
Vincent, W. S., and Goldstein, E. S. (1981). Rapid preparation of covalently closed circular DNA by acridine yellow affinity chromatography. Anal. Biochem. 110, 123–127. doi:10.1016/0003-2697(81)90121-4
Webb, R. B., Hass, B. S., and Kubitschek, H. E. (1979). Photodynamic effects of dyes on bacteria. Mutat. Research/Fundamental Mol. Mech. Mutagen. 59, 1–13. doi:10.1016/0027-5107(79)90190-8
Zhang, J. N., Yang, X. H., Guo, W. J., Wang, B., and Zhang, Z. H. (2017). Magnetic metal–organic framework CoFe2O4@SiO2@IRMOF-3 as an efficient catalyst for one-pot synthesis of functionalized dihydro-2-oxopyrroles. Synlett 28, 734–740. doi:10.1055/s-0036-1588924
Keywords: acridine yellow G (AYG), photo-induced electron transfer (PET), renewable energy source, polyfunctionalized dihydro-2-oxypyrroles, photochemical synthesis
Citation: Mohamadpour F (2022) Acridine yellow G (AYG) as a photo-induced electron transfer (PET) photocatalyst employed for the radical Michael–Mannich cyclocondensation of imines. Front. Chem. 10:1015330. doi: 10.3389/fchem.2022.1015330
Received: 18 August 2022; Accepted: 12 September 2022;
Published: 06 October 2022.
Edited by:
Guigen Li, Texas Tech University, United StatesReviewed by:
Changsheng Yao, Jiangsu Normal University, ChinaRajasekar Reddy Annapureddy, Ludwig Maximilian University of Munich, Germany
Copyright © 2022 Mohamadpour. This is an open-access article distributed under the terms of the Creative Commons Attribution License (CC BY). The use, distribution or reproduction in other forums is permitted, provided the original author(s) and the copyright owner(s) are credited and that the original publication in this journal is cited, in accordance with accepted academic practice. No use, distribution or reproduction is permitted which does not comply with these terms.
*Correspondence: Farzaneh Mohamadpour, bW9oYW1hZHBvdXIuZi43QGdtYWlsLmNvbQ==