- 1Key Laboratory of Agricultural Genetics and Breeding, The Biotechnology Research Institute, Shanghai Academy of Agricultural Sciences, Shanghai, China
- 2School of Life Science and Engineering, Lanzhou University of Technology, Lanzhou, China
- 3Crops Ecological Environment Security Inspection and Supervision Center (Shanghai), Ministry of Agriculture and Rural Affairs, Shanghai, China
- 4Institute of Applied Microbiology, Xinjiang Academy of Agricultural Sciences, Urumchi, China
Genetically modified (GM) crops containing phosphinothricin acetyltransferase (PAT) protein has been widely planted worldwide. The development of a rapid method for detecting PAT protein is of great importance to food supervision. In this study, a simple label-free electrochemical immunosensor for the ultrasensitive detection of PAT protein was constructed using thionine (Thi)/gold nanoparticles (AuNPs) as signal amplification molecules and electrochemically active substances. Under optimum conditions, the limits of detection of the sensor for soybean A2704-12 and maize BT-176 were 0.02% and 0.03%, respectively. The sensor could detect crops containing PAT protein and had no cross-reaction with other proteins. After storage at 4°C for 33 days, the sensor still retained 82.5% of the original signal, with a relative standard deviation (RSD) of 0.92%. The recoveries of the sensor for soybean A2704-12 and maize BT-176 were 85%–108% and 98%–113%, respectively. The developed PAT-target immunosensor with high sensitivity, specificity, and satisfactory reproducibility and accuracy will be a useful tool in the trace screening of GM crops. Moreover, this design concept can be extended to other proteins by simply changing the antibody.
1 Introduction
In recent years, the cultivation area of genetically modified (GM) crops has rapidly expanded and reached 1.904 billion hectares (James, 2019). Herbicide-resistant crops are one of the most important and widest-planted GM crops (Mathur et al., 2017) and include soybean, maize, cotton, and rapeseed. Phosphinothricin acetyltransferase (PAT) protein is encoded by the bar or the pat gene and makes crops resistant to the herbicide glyphosate (Hérouet et al., 2005). In China, GM crops and products are strictly subject to mandatory labeling by GM organic management; however, unauthorized GM crops or products still appear in the field and markets from time to time (Yue et al., 2019). Thus, it is necessary to find an effective and rapid method to strengthen the detection and supervision of GM crops and products.
At present, increasingly precise instruments and methods are being applied to detect GM crops, including polymerase chain reaction (PCR) assays and real-time immune-PCR (IPCR) (Liu et al., 2016; Geoffrey et al., 2018). As a DNA-based method, PCR can qualitatively and quantitatively analyze ingredients in GM crops. However, PCR methods depend on thermal cycler, which are complicated and time consuming, and fail to detect exogenous proteins (Grel et al., 2011). Methods based on protein-specific expression, such as enzyme-linked immunosorbent assay (ELISA), the test strip, and Western blot (Wang et al., 2015; Albright et al., 2016; Zeng et al., 2020), have also been applied to GM crops. While these methods are fast, they fail to sensitively and quantitatively detect the protein in GM crops. In addition, some new effective and rapid detection methods, including surface plasmon resonance, loop-mediated isothermal amplification (LAMP), and electrochemical immunosensors, are also being used for GM crop detection (Ming et al., 2015; Shen et al., 2016; Lu et al., 2018).
To construct a sensitive, selective, and stable immunosensor, many strategies have been used. Nanomaterials with a large surface area and good conductivity have been utilized as a substrate and to improve electron transfer capability (Lv et al., 2018). Functional molecules with active groups have been used to increase the effective binding sites. To date, various nanomaterials have been used to enhance the detection signal of immunosensor, such as gold nanoparticles (Choosang et al., 2021), quantum and carbon dots (Yang et al., 2020; Qin et al., 2019), and metal oxide nanomaterials (Dekany and Sebok and Daniel, 2015). Thionine (Thi) is a cationic phenothiazine dye that is usually used as an electrochemical indicator. As a functional monomer with two amino groups, it has exceptional electrochemical stability with good electron transfer ability (García-Mendiola et al., 2020). Because of these properties, Thi is widely used in the field of electrochemistry (Fan et al., 2019; Wang et al., 2020).
In this study, gold nanoparticles (AuNPs) were modified onto the electrode surface by electrodeposition to increase the electron transfer. Additionally, Thi was connected to the surface via Au–S bonds to dramatically enhance the electrochemical signal and was also used for conjunction with the monoclonal antibody (mAb). Based on this method, a label-free electrochemical immunosensor for PAT protein was constructed with high sensitivity, specificity, and reproducibility. Furthermore, this simple concept provides a useful approach for the detection of transgenic proteins and can also be applied in other field by changing the recognition elements.
2 Materials and Methods
2.1 Materials and Apparatus
The mAb against the PAT protein was purchased from Artron (Jinan, China). Glutaraldehyde, chloroauric acid, bovine serum albumin (BSA), and Thi were purchased from Sigma (St. Louis, MO, USA). Seed powder standards of GM crops (5%, 100%, and 1%) used for the immunosensor test were obtained from ERM (Geel, Belgium), AOCS (Urbana, IL, USA), and the Ministry of Agriculture (Beijing, China), as listed in Supplementary Table S1. All chemicals and solvents were of analytical grade.
All the electrochemical measurements used a three-electrode system on a computer-controlled CHI 660E electrochemical workstation (Chenhua, Shanghai, China). A glassy carbon electrode (GCE, d = 3 mm) was used as the working electrode, and the auxiliary and reference electrodes employed a platinum and Ag/AgCl electrode, respectively. The micromorphology of the materials coated on the GCE was verified by a scanning electron microscope (SEM, Hitachi, Japan).
2.2 Preparation of the Phosphinothricin Acetyltransferase Electrochemical Immunosensor
Prior to modification, the GCE was polished with 1 μm, 0.3 µm, and 0.05 µm aluminum powder sequentially on polishing paper, with sonication in double-distilled water between each polishing step. The cleaned GCE was immersed in HAuCl4 solution (1%) and electrodeposited on a cyclic voltammeter (CV) with measurements from−0.2 to +0.6 V (Zhang et al., 2011). A second layer was formed by dropping Thi solution (1.0 mg/ml, 5 μl) onto the surface of GCE and storing it at 4°C overnight. Afterward, the PAT-mAb was dropped onto the GCE and incubated at 37°C for 40 min to form the third layer. Finally, 5 μl of PBS (0.01 M, pH 7.4) containing 5% BSA was dropped onto the electrode to block remaining active sites. Each modified process was followed by washing with double-distilled water. The prepared electrode was stored at 4°C for subsequent use. The process of immunosensor preparation is illustrated in Scheme 1.
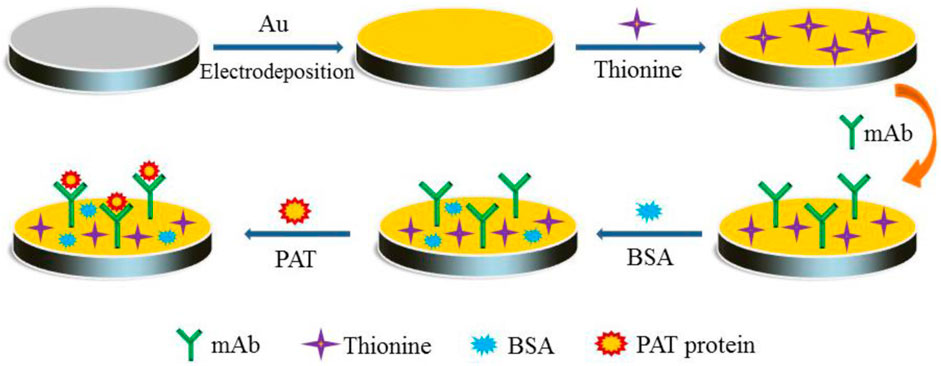
Scheme 1. Schematic diagram of the electrochemical immunosensor based on gold nanoparticles (AuNPs)/thionine (Thi) for the sensitive and rapid detection of phosphinothricin acetyltransferase (PAT) protein in genetically modified (GM) crops.
2.3 Electrochemical Measurements
The electrochemical measurement depends on the current changes before and after the antigen–antibody reaction. When the antigen–antibody immune complex is formed, the electron transfer is hindered and the peak current response is reduced. Differential pulse voltammetry (DPV) measurements were recorded from 0 to +0.4 V at a scan rate of 50 mV/s in 0.1 M PBS solution (pH 7.4) containing 5 mM [Fe(CN)6]3−/4− and 0.1 M KCl to detect the PAT protein. The amplitude, pulse width, sampling width, and quiet time were 0.05 V, 0.06 s, 0.02 s, and 2 s, respectively. The assembly process of the immunosensor was characterized step-by-step using CV measurements with [Fe(CN)6]3−/4− as the redox probe. All experimental measurements were performed at room temperature.
2.4 Sample Analysis
The PAT protein was extracted from different crop seed powder standards using PBS buffer (0.01 M, pH 7.4) at a mass volume ratio of 1:3. After adding the PBS into the seed powder, the mixture was shaken at room temperature for 5 min and centrifuged at 6,000 rpm for 5 min to remove the pellet. Crop supernatants were stored at 4°C for follow-up tests. Crop supernatants with different transgenic contents were diluted with corresponding blank crop supernatants. Five microliters of different samples were dropped onto the prepared working electrode incubation at 37°C for 40 min.
3 Results
3.1 Characterization of the Modified Process
The stepwise assembly process of the immunosensor was detected by CV in PBS (0.1 M, pH 7.4) containing 5 mM [Fe(CN)6]3−/4− and 0.1 M KCl at a scan rate of 50 mV/s, as shown in Figure 1A. The bare GCE had a low-level peak current value, while the electrodeposited AuNPs could largely increase the surface area of electrode and promote electron transfer. When the Thi was bound to the electrode surface, the peak current increased significantly. As the antibody and BSA hindered the electron transport, the current gradually decreased when the antibody and BSA were coupled to the electrode. Finally, the peak current continued to decrease followed by incubation with PAT. The change in current on the electrode surface was obvious, indicating that the immunosensor had been successfully modified. Furthermore, the enhancement of the current by AuNPs/Thi was sufficient.
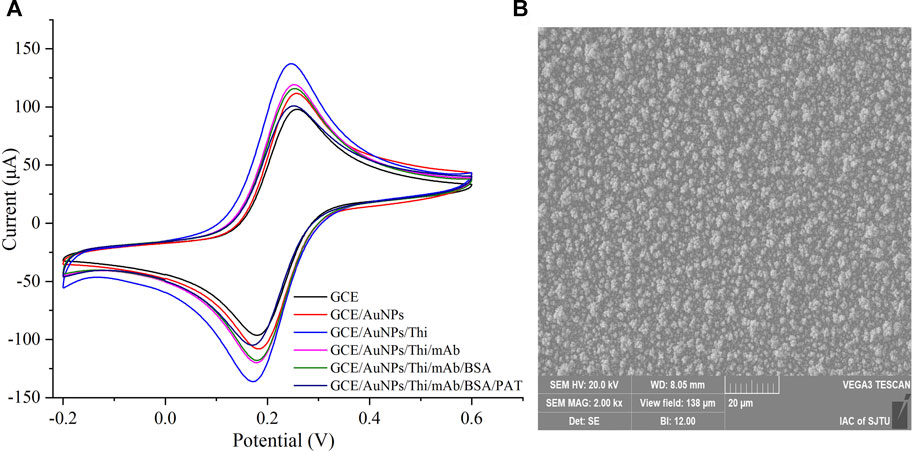
FIGURE 1. Characterization of the electrochemical immunosensor. (A) The stepwise assembly process of the immunosensor was detected by cyclic voltammeter measurements. (B) The scanning electron microscope (SEM) image of gold nanoparticles (AuNPs) deposited on the electrode surface.
AuNPs, as a low-dimensional functional material, has been reported to increase the surface area of sensors and the immobilization of antibodies (Zhang et al., 2011). Modification with AuNPs promoted the electron transfer between the oxidized compound and the sensors (Lu et al., 2018). In this study, AuNPs were electrodeposited onto the surface of GCE to promote the electronic transmission and amplify the sensor signals. The surface morphology of the modified electrode was investigated using SEM. As shown in Figure 1B, AuNPs formed a uniform film on the surface, which increased the surface area and greatly improved the loading capacity of the GCE.
3.2 Optimization of the Thionine Conditions
To obtain the best sensor response, the concentration and incubation time of Thi were optimized. As displayed in Figure 2A, with the increase in incubation time, the current signal gradually enhanced, and 4°C incubation overnight resulted in a maximum current response. Since there were no significant differences between 40 min and 1 h, the data overlapped in the figure. The influence of Thi concentration on current response is presented in Figure 2B. The peak current increased with the increase in Thi concentration. When the Thi concentration was higher than 1.0 mg/ml, the peak current decreased. This may be due to excessive Thi accumulation hindering the electron transfer on the electrode surface. Therefore, 1.0 mg/ml of Thi and an overnight incubation were selected as the optimal conditions for further testing.
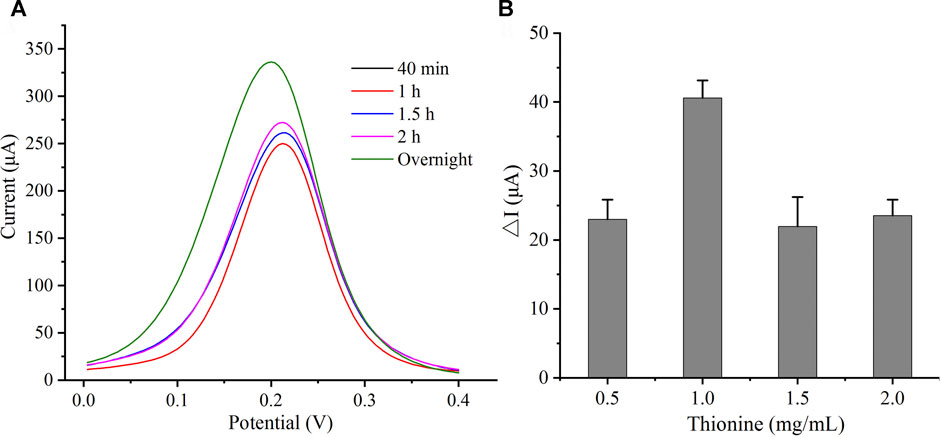
FIGURE 2. Condition optimization of Thi. (A) Optimization of time. (B) Optimization of Thi concentration.
3.3 Performance analysis of Phosphinothricin Acetyltransferase Immunosensor
3.3.1 Sensitivity of the Immunosensor
Under the optimal experimental parameters, the sensitivity of the developed PAT-targeted immunosensor was evaluated by DPV measurements. The current responses with different concentrations of soybean A2704-12 and maize BT-176 are shown in Figures 3A, B (from top to bottom, the concentrations of PAT in PBS buffer were in the range of 0.05%–1.5%). The peak current decreased as the concentration of GM crops increased, which was due to the hindrance of electron transfer with the increase of PAT protein on the electrode surface. The corresponding calibration curves for soybean A2704-12 and maize BT-176 are shown in Figures 3C, D. The linear regression equations were ΔI = 22.424x + 10.708 (R2 = 0.9936) and ΔI = 13.873x + 5.7094 (R2 = 0.9933), where ΔI was the current signal (μA) change and x was the PAT concentration. The limit of detection was 0.02% for A2704-12 and 0.03% for BT-176 samples (S/N = 3), which was the sensitivity of the developed immunosensor.
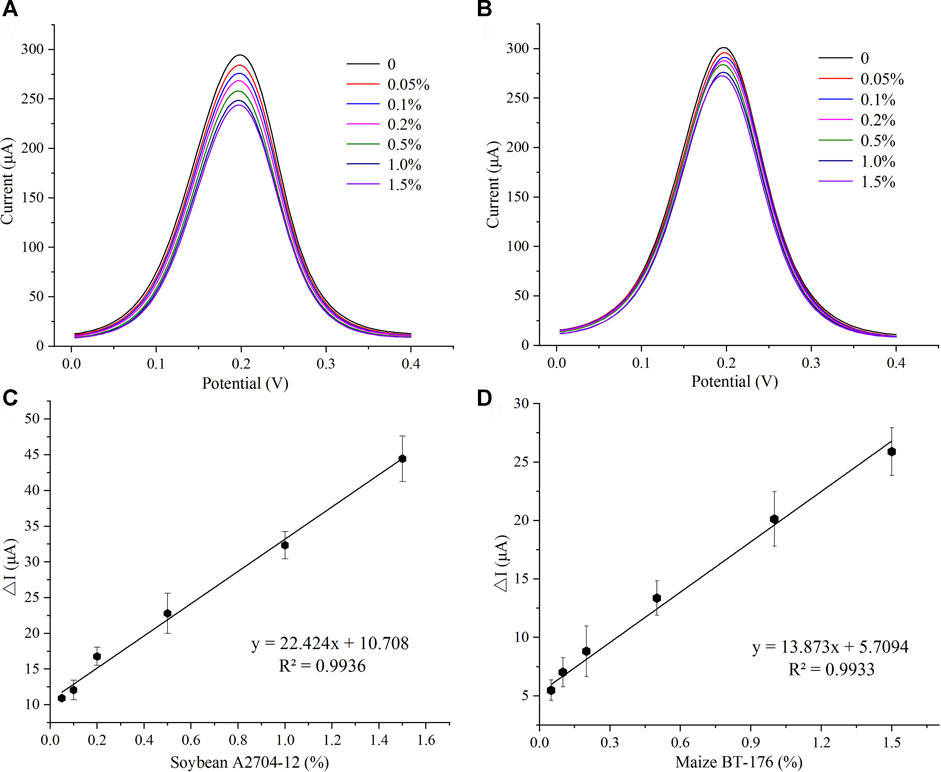
FIGURE 3. Sensitivity of the immunosensor. (A) Differential pulse voltammetry (DPV) peak current for different concentrations of soybean A2704-12. (B) DPV peak current for different concentrations of maize BT-176. (C) Calibration curve of the immunosensor toward soybean A2704-12 (n = 3). (D) Calibration curve of the immunosensor toward maize BT-176 (n = 3).
3.3.2 Selectivity and Stability of the Immunosensor
To estimate the selectivity of the immunosensor, common GM crops containing different GM proteins were tested. GM crops at contents of 5%, i.e., maize BT-176 (BT-Cry1Ac/PAT), maize MIR604 (BT-Cry3A), maize MON89034 (BT-Cry1A105/Cry2Ab), maize MON88017 (CP4-EPSPS/Cry3Bb1), soybean RRS (CP4-EPSPS), cotton MON88913 (CP4-EPSPS), and sugar beet H7-1 (CP4-EPSPS), were investigated. Additionally, the GM crops at contents of 1% including maize MON810 (BT-Cry1Ab), maize BT-11 (BT-Cry1Ab/PAT), soybean A2704-12 (PAT), and rapeseed T45 (PAT) were also examined. A blank signal was obtained from corresponding non-GM crops. As shown in Figure 4A, the peak current change in crops containing PAT protein was significantly higher than the others. The results indicated that the sensor could detect crops containing PAT protein, and the detection ability was not affected by other proteins such as CP4-EPSPS, BT-Cry1Ab, BT-Cry3A, and BT-Cry1A105/Cry2Ab.
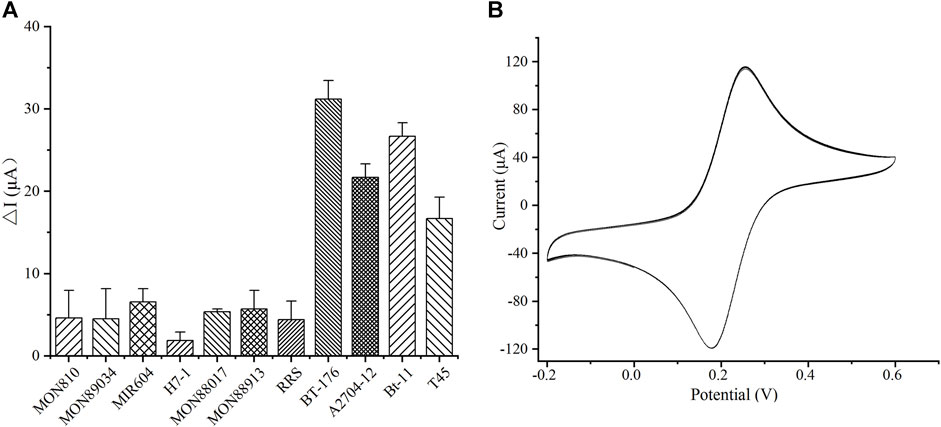
FIGURE 4. Specificity and stability of the developed immunosensor. (A) The specificity of the sensors. (B) The stability of the sensors by cyclic voltammeter (CV) measurements.
The stability of the developed sensor was evaluated. The constructed immunosensor still maintained 82.5% of the initial current value after storage at 4°C for 33 days. The sensor was then successively scanned under continuous CV for 15 cycles after storage for 33 days (Figure 4B), and the relative standard deviation (RSD) of the response was 0.92%. Thus, the constructed immunosensor demonstrated acceptable stability and satisfactory repeatability.
3.3.3 Recovery Experiment
The accuracy of the constructed immunosensor was evaluated using BT-176 and A2704-12 standards with five different concentrations (0.05%, 0.1%, 0.5%, and 1%). Each concentration had three parallel tests, and the results are shown in Table 1. The average recoveries of the maize and soybean samples were 98%–113% and 85%–108%, respectively. The RSDs were all less than 15.0%, which further confirmed that the electrochemical sensor was suitable for the detection of PAT protein and had good accuracy.
4 Discussion
According to previous reports, the immunosensor based on AuNPs/Thi/CMWCNTs (carboxylated multiwalled carbon nanotubes) for measuring interleukin-6 (IL-6) has been developed with high sensitivity (Wang et al., 2020). To the best of our knowledge, the AuNPs/Thi-modified electrochemical immunosensor for ultrasensitive detection of PAT protein has not been reported before. A brief summary of the different methods for the detection of GM crops is presented in Table 2. Each detection method has its own significance and value for the final determination of food quality and safety (Nazir et al., 2019). PCR (Cao et al., 2018) and real-time PCR methods (Yao et al., 2018) are used for detecting GM crops at nucleic acid levels. Immunochromatographic strip (ICS) testing can rapidly detect the targets with high specificity but generally low sensitivity. The previously reported electrochemiluminescence (ECL) method showed high sensitivity (Zhang et al., 2020); however, the materials used to modify the electrode were unfriendly to the environment and human health (Li et al., 2021). The main advantages of this study were as follows: 1) AuNPs were electrodeposited on the electrode surface without complicated procedures. 2) AuNPs and Thi were connected by an Au–S bond, which benefited the long-term stability and sensitivity of sensors (Xu et al., 2015). This simple modification scheme can enhance the conductivity of electrodes and enlarge the surface area to provide more binding sites.
5 Conclusion
In this study, a simple label-free electrochemical immunosensor for the ultrasensitive detection of PAT protein in GM crops was successfully constructed using AuNPs and Thi as signal amplification molecules. Using the label-free strategy, PAT protein could be detected based on the current changes caused by the immunoreaction on the electrode surface. Under optimal conditions, the limits of detection for soybean A2704-12 and maize BT-176 were as low as 0.02% and 0.03%, respectively. Following storage at 4°C for 33 days, the sensor still maintained 82.5% of the initial signal, with an RSD of 0.92%, exhibiting high selectivity, acceptable stability, high sensitivity, and good reproducibility. This method can provide an effective tool for the trace detection of GM crops. Moreover, research is ongoing for more targets to realize high-throughput analysis.
Data Availability Statement
The original contributions presented in the study are included in the article/Supplementary Material. Further inquiries can be directed to the corresponding authors.
Author Contributions
QY handled the writing of the original draft, data curation, and formal analysis. HL and YW validated and investigated the study. HB took part in the formal analysis and validation. XL also handled the formal analysis, validation, and investigation. JW was in charge of the investigation, data curation, and funding acquisition. HZ was responsible for conceptualization, methodology, supervision, funding acquisition, and writing, reviewing, and editing manuscript.
Funding
This study was supported by the Natural Science Foundation of Shanghai No. 19ZR1436800, the Shanghai Sailing Program No. 20YF1443000, the Shanghai Agricultural Science and Technology Innovation Action Plan No. 21N31900800, the Shanghai Science and Technology Commission, the Belt and Road Project (20310750500), the SAAS Project on Agricultural Science and Technology Innovation Supporting Area (SAAS Application Basic Study 2021 (09)), and the SAAS Project on Agricultural Science and Technology Innovation Supporting Area (SAAS Platform 2021(08)) and SAAS Program for Excellent Research Team No. 2017 (B-07) and No. 2022 (B-16).
Conflict of Interest
The authors declare that the research was conducted in the absence of any commercial or financial relationships that could be construed as a potential conflict of interest.
Publisher’s Note
All claims expressed in this article are solely those of the authors and do not necessarily represent those of their affiliated organizations, or those of the publisher, the editors, and the reviewers. Any product that may be evaluated in this article, or claim that may be made by its manufacturer, is not guaranteed or endorsed by the publisher.
Supplementary Material
The Supplementary Material for this article can be found online at: https://www.frontiersin.org/articles/10.3389/fchem.2021.770584/full#supplementary-material
References
Albright, V. C., Hellmich, R. L., and Coats, J. R. (2016). A Review of Cry Protein Detection with Enzyme-Linked Immunosorbent Assays. J. Agric. Food Chem. 64, 2175–2189. doi:10.1021/acs.jafc.5b03766
Cao, J., Yao, Y., Fan, K., Tan, G., Xiang, W., Xia, X., et al. (2018). Harnessing a Previously Unidentified Capability of Bacterial Allosteric Transcription Factors for Sensing Diverse Small Molecules In Vitro. Sci. Adv. 4 (12), eaau4602. doi:10.1126/sciadv.aau4602
Choosang, J., Khumngern, S., Thavarungkul, P., Kanatharana, P., and Numnuam, A. (2021). An Ultrasensitive Label-free Electrochemical Immunosensor Based on 3D Porous Chitosan-Graphene-Ionic Liquid-Ferrocene Nanocomposite Cryogel Decorated with Gold Nanoparticles for Prostate-specific Antigen. Talanta 224, 121787. doi:10.1016/j.talanta.2020.121787
Cottenet, G., Blancpain, C., Sonnard, V., and Chuah, P. F. (2019). Two FAST Multiplex Real-Time PCR Reactions to Assess the Presence of Genetically Modified Organisms in Food. Food Chem. 274, 760–765. doi:10.1016/j.foodchem.2018.09.050
Fan, Y., Shi, S., Ma, J., and Guo, Y. (2019). A Paper-Based Electrochemical Immunosensor with Reduced Graphene Oxide/thionine/gold Nanoparticles Nanocomposites Modification for the Detection of Cancer Antigen 125. Biosens. Bioelectron. 135, 1–7. doi:10.1016/j.bios.2019.03.063
Gao, H., Wen, L., Wu, Y., Fu, Z., and Wu, G. (2017). An Ultrasensitive Label-Free Electrochemiluminescent Immunosensor for Measuring Cry1Ab Level and Genetically Modified Crops Content. Biosens. Bioelectron. 97, 122–127.
Gao, H., Wen, L., Wu, Y., Yan, X., Li, J., Li, X., et al. (2018). Sensitive and Facile Electrochemiluminescent Immunoassay for Detecting Genetically Modified Rapeseed Based on Novel Carbon Nanoparticles. J. Agric. Food Chem. 66 (20), 5247–5253.
García-Mendiola, T., Requena-Sanz, S., Martínez-Periñán, E., Bravo, I., Pariente, F., and Lorenzo, E. (2020). Influence of Carbon Nanodots on DNA-Thionine Interaction. Application to Breast Cancer Diagnosis. Electrochimica Acta 353, 136522. doi:10.1016/j.electacta.2020.136522
Gürel, F., Arican, E., Gzükirmizi, N., and Ar, A. (2011). Recent Molecular Tools for Detection Transgenic Events in Genetically Modified (GM) Crop Products. Scientific Res. essays 6, 5091–5099.
Hérouet, C., Esdaile, D. J., Mallyon, B. A., Debruyne, E., Schulz, A., Currier, T., et al. (2005). Safety Evaluation of the Phosphinothricin Acetyltransferase Proteins Encoded by the Pat and Bar Sequences that Confer Tolerance to Glufosinate-Ammonium Herbicide in Transgenic Plants. Regul. Toxicol. Pharmacol. 41, 134–149. doi:10.1016/j.yrtph.2004.11.002
James, C. (2019). Global Status of Commercialized Biotech/GM Crops in 2019: Biotech Crops. ISAAA Brief NO. 55.
Junichi, M., Shuko, H., Yasuaki, N., Satoshi, F., Reona, T., and Kazumi, K. (2019). Highly Sensitive GMO Detection Using Real-Time PCR With a Large Amount of DNA Template: Single-Laboratory Validation. J. Aoac Internat. 101 (2), 507–514.
Li, S., Ma, X., Pang, C., Wang, M., Yin, G., Xu, Z., et al. (2021). Novel Chloramphenicol Sensor Based on Aggregation-Induced Electrochemiluminescence and Nanozyme Amplification. Biosens. Bioelectron. 176, 112944. doi:10.1016/j.bios.2020.112944
Liu, Y., Jiang, D., Lu, X., Wang, W., Xu, Y., and He, Q. (2016). Phage-Mediated Immuno-PCR for Ultrasensitive Detection of Cry1Ac Protein Based on Nanobody. J. Agric. Food Chem. 64, 7882–7889. doi:10.1021/acs.jafc.6b02978
Lu, X., Jiang, D.-j., Yan, J.-x., Ma, Z.-e., Luo, X.-e., Wei, T.-l., et al. (2018). An Ultrasensitive Electrochemical Immunosensor for Cry1Ab Based on Phage Displayed Peptides. Talanta 179, 646–651. doi:10.1016/j.talanta.2017.11.032
Lv, M., Liu, Y., Geng, J., Kou, X., Xin, Z., and Yang, D. (2018). Engineering Nanomaterials-Based Biosensors for Food Safety Detection. Biosens. Bioelectron. 106, 122–128. doi:10.1016/j.bios.2018.01.049
Mathur, V., Javid, L., Kulshrestha, S., Mandal, A., and Reddy, A. A. (2017). World Cultivation of Genetically Modified Crops: Opportunities and Risks, 45, 87. doi:10.1007/978-3-319-58679-3_2
Ming, H., Wang, M., and Yin, H. (2015). Detection of Bacillus Thuringiensis Cry1Ab Protein Based on Surface Plasmon Resonance Immunosensor. Anal. Biochem. 468, 59–65. doi:10.1016/j.ab.2014.09.014
Nazir, S., Zaffar Iqbal, M., and Sajid-ur-Rahman., (2019). Molecular Identification of Genetically Modified Crops for Biosafety and Legitimacy of Transgenes. Gene Editing - Tech. Appl. doi:10.5772/intechopen.81079
Sebők, D., and Dékány, I. (2015). ZnO2 Nanohybrid Thin Film Sensor for the Detection of Ethanol Vapour at Room Temperature Using Reflectometric Interference Spectroscopy. Sensors Actuators B: Chem. 206, 435–442. doi:10.1016/j.snb.2014.09.087
Shen, P., Geng, F., Yu, Y., Zhang, Y., Wang, Z., Li, Z., et al. (2016). A Rapid Loop-Mediated Isothermal Amplification Method for Detection of the Modified GM cry1A Gene in Transgenic Insect-Resistant Cotton and rice. Food Control 62, 357–364. doi:10.1016/j.foodcont.2015.10.035
Takabatake, R., Kagiya, Y., Minegishi, Y., Yeasmin, S., Futo, S., Noguchi, A., et al. (2018). Development and Evaluation of Rapid Screening Detection Methods for Genetically Modified Crops Using Loop-Mediated Isothermal Amplification. Food Chem. 252, 390–396.
Wang, Q., Yin, Q., Fan, Y., Zhang, L., Xu, Y., Hu, O., et al. (2019). Double Quantum Dots-Nanoporphyrin Fluorescence-Visualized Paper-Based Sensors for Detecting Organophosphorus Pesticides. Talanta 199, 46–53. doi:10.1016/j.talanta.2019.02.023
Wang, X., Chen, X., Xu, J., Dai, C., and Shen, W. (2015). Degradation and Detection of Transgenic Bacillus Thuringiensis DNA and Proteins in Flour of Three Genetically Modified rice Events Submitted to a Set of thermal Processes. Food Chem. Toxicol. 84, 89–98. doi:10.1016/j.fct.2015.08.010
Wang, Z., Yang, S., Wang, Y., Feng, W., Li, B., Jiao, J., et al. (2020). A Novel Oriented Immunosensor Based on AuNPs-Thionine-CMWCNTs and Staphylococcal Protein A for Interleukin-6 Analysis in Complicated Biological Samples. Analytica Chim. Acta 1140, 145–152. doi:10.1016/j.aca.2020.10.025
Xu, W., He, J., Gao, L., Zhang, J., and Yu, C. (2015). Immunoassay for Netrin 1 via a Glassy Carbon Electrode Modified with Multi-Walled Carbon Nanotubes, Thionine and Gold Nanoparticles. Microchim Acta 182, 2115–2122. doi:10.1007/s00604-015-1551-3
Yang, X., Xu, J., Luo, N., Tang, F., Zhang, M., and Zhao, B. (2020). N,Cl Co-doped Fluorescent Carbon Dots as Nanoprobe for Detection of Tartrazine in Beverages. Food Chem. 310, 125832. doi:10.1016/j.foodchem.2019.125832
Yao, Y., Li, S., Cao, J., Liu, W., Qi, F., Xiang, W., et al. (2018). A Novel Signal Transduction System for Development of Uric Acid Biosensors. Appl. Microbiol. Biotechnol. 102, 7489–7497. doi:10.1007/s00253-018-9056-8
Yue, M., Geng, W., Wang, Y., and Jia, Y. (2019). Risk Analysis of Genetically Modified Ingredients in Soybeans and Soybean Products Sold in Tianjin. J. Prev. Med. Chin. People's Libearation Army, 37.
Zeng, H., Wang, J., Jia, J., Wu, G., Yang, Q., Liu, X., et al. (2020). Development of a Lateral Flow Test Strip for Simultaneous Detection of BT-Cry1Ab, BT-Cry1Ac and CP4 EPSPS Proteins in Genetically Modified Crops. Food Chem. 335, 127627. doi:10.1016/j.foodchem.2020.127627
Zhang, Q., Liu, Y., Nie, Y., and Ma, Q. (2020). Magnetic-plasmonic Yolk-Shell Nanostructure-Based Plasmon-Enhanced Electrochemiluminescence Sensor. Sensors Actuators B: Chem. 319, 128245. doi:10.1016/j.snb.2020.128245
Zhang, Q., Wang, W., Yang, Z., Wang, X., Xu, W., Huang, K., et al. (2021). A Portable 3D-Printed Biosensing Device for Rapid Detection of Genetically Modified maize Mon810. Sensors Actuators B: Chem. 349, 130748. doi:10.1016/j.snb.2021.130748
Keywords: immunosensor, thionine, gold nanoparticles, label free, genetically modified crops
Citation: Yang Q, Wang Y, Liu X, Liu H, Bao H, Wang J and Zeng H (2021) A Label-Free Immunosensor Based on Gold Nanoparticles/Thionine for Sensitive Detection of PAT Protein in Genetically Modified Crops. Front. Chem. 9:770584. doi: 10.3389/fchem.2021.770584
Received: 04 September 2021; Accepted: 29 October 2021;
Published: 07 December 2021.
Edited by:
Huan-Tsung Chang, National Taiwan University, TaiwanReviewed by:
Han Yih Lau, Malaysian Agricultural Research and Development Institute (MARDI), MalaysiaMaojun Jin, Chinese Academy of Agricultural Sciences (CAAS), China
Copyright © 2021 Yang, Wang, Liu, Liu, Bao, Wang and Zeng. This is an open-access article distributed under the terms of the Creative Commons Attribution License (CC BY). The use, distribution or reproduction in other forums is permitted, provided the original author(s) and the copyright owner(s) are credited and that the original publication in this journal is cited, in accordance with accepted academic practice. No use, distribution or reproduction is permitted which does not comply with these terms.
*Correspondence: Haijuan Zeng, zenghaijuan12@126.com; Jinbin Wang, wangjinbin2013@126.com
†These authors have contributed equally to this work