- 1Laboratory of Bioorganic Chemistry, Department of Biochemistry and Biotechnology, University of Thessaly, Larissa, Greece
- 2Laboratory of Organic Chemistry, Department of Chemistry, Aristotle University of Thessaloniki, Thessaloniki, Greece
- 3Department of Microbiology and Immunology, Rega Institute for Medical Research, KU Leuven, Leuven, Belgium
We describe the synthesis of C8-alkynyl adenine pyranonucleosides 4, 5, and 8-phenylethynyl-adenine (II), via Sonogashira cross-coupling reaction under microwave irradiation. Compounds 4e and II were less cytostatic than 5-fluorouracil (almost an order of magnitude) against murine leukemia (L1210) and human cervix carcinoma (HeLa) cells, while the same compounds proved to be more active than 5-fluorouracil against human lymphocyte (CEM) cells.
Introduction
Synthetic nucleoside analogs with modified nucleobase moieties are of considerable importance in the search for promising lead candidates endowed with antiviral, anticancer, and antibacterial activities (Herdewijn, 2008; Manta et al., 2014). Among them, a number of purine and pyrimidine substituted nucleoside derivatives exhibited activity in both solid tumors and hematological malignancies, behaving as antimetabolites, competing with physiological nucleosides, and consequently, interacting with a large number of intracellular targets to induce cytotoxicity (Hatse et al., 1999).
Alkynyl-modified nucleosides and especially pyrimidine derivatives substituted at C5 and purine derivatives substituted at C8, have been shown to possess interesting biological properties (Lin et al., 1985; Meneni et al., 2007; Lee et al., 2009; Vivet-Boudou et al., 2011). Some representative examples include 5-ethynyl-2′-deoxyuridine, which exhibited antiproliferative activity against human breast cancer cells, exceeding that of cisplatin and 5-fluorouracil, while 5-bromoethynyluridine demonstrated significant anti-HCV properties (Escuret et al., 2005; Meneni et al., 2007). Although, little effort has been made toward the synthesis of C8-modified purine nucleosides, in some cases, interesting biological properties have been reported, such as some 8-alkynyl adenosines, which proved to be very selective ligands for the A3 adenosine receptor subtype behaving as adenosine antagonists (Volpini et al., 2001) and various C8-modified 2′-deoxy adenosines, which induced delayed chain termination in vitro and showed moderate anti HIV-1 activity in cell culture (Vivet-Boudou et al., 2011).
Considering all the progress made toward this direction, we have recently embarked on the synthesis of C5-substituted uracil and cytosine glucopyranonucleosides bearing a variety of alkyne substituents, such as linear alkyl chains and aromatic rings substituted with linear and branched alkyl groups (Dimopoulou et al., 2013), which effectively inhibited the proliferation of a variety of tumor cell lines and they also proved as some of the most potent inhibitors of the active site of glycogen phosphorylase (Kantsadi et al., 2012). Among these agents, the C5-phenylethynyluracil pyranonucleoside showed appreciable cytotoxic activity (IC50 of 5.2–6.2 μM), comparable to 5-fluorouracil (Dimopoulou et al., 2013).
As a continuation of our studies on the synthesis of base-modified pyranonucleosides and considering the interesting biological properties of substituted purines, it was speculated that the introduction of alkynyl modifications at the 8-position of either adenine pyranonucleosides or even adenine itself, could possibly lead to more efficacious therapeutic agents. This conjugation appeared to us as a challenge and the first biological results confirmed our hypothesis.
Experimental (General Methods)
Melting points were recorded in a Mel-Temp apparatus and are uncorrected. Thin layer chromatography (TLC) was performed on Merck precoated 60F254 plates. Reactions were monitored by TLC on silica gel, with detection by UV light (254 nm) or by charring with sulfuric acid. Flash column chromatography was performed using silica gel (240–400 mesh, Merck). 1H and 13C NMR spectra were obtained at room temperature with a Bruker 300 spectrometer at 300 and 75.5 MHz, respectively using chloroform-d (CDCl3) and dimethylsulfoxide-d6 (DMSO-d6) with internal tetramethylsilane (TMS). The 1H assignments were based on 1H -1H COSY experiments executed using standard Varian software. Chemical shifts (δ) were given in ppm measured downfield from TMS, and spin-spin coupling constants are in Hz. Mass spectra were obtained on a ThermoQuest Finnigan AQA Mass Spectrometer (electrospray ionization). Optical rotations were measured using an Autopol I polarimeter and UV-Vis spectra were recorded on a PG T70 UV-VIS spectrometer. Acetonitrile (CH3CN) was distilled from calcium hydride and stored over 3Å molecular sieves. N,N-Dimethylformamide (DMF) was stored over 3Å molecular sieves. All reactions sensitive to oxygen or moisture were carried out under nitrogen atmosphere using oven-dried glassware. All microwave irradiation experiments were carried out in a dedicated CEM-Explorer and CEM Discover monomode microwave apparatus, operating at a frequency of 2.45 GHz with continuous irradiation power from 0 to 300 W with utilization of the standard absorbance level of 300 W maximum power. The reactions were carried out in 10-mL glass tubes, sealed with a Teflon septum and placed in the microwave cavity. Initially, microwave irradiation of required watts was used, and the temperature was ramped from room temperature to the desired temperature. Once this was reached the reaction mixture was held at this temperature for the required time. The reaction mixture was continuously stirred during the reaction. The temperature was measured with an IR sensor on the outer surface of the process vial. After the irradiation period, gas jet cooling rapidly cooled the reaction vessel to ambient temperature.
9-(2′,3′,4′,6′-Tetra-O-Acetyl-β-D-Glucopyranosyl)-N6-Benzoyl Adenine (2)
A mixture of N6-benzoyladenine (797 mg, 3.33 mmol, 1.3 equiv), hexamethyldisilazane (HMDS) (871 μL, 4.13 mmol, 1.24 equiv) and saccharine (27 mg, 0.15 mmol, 0.046 equiv) in anhydrous CH3CN (14 mL) was refluxed for 1 h under nitrogen. 1,2,3,4,6-Penta-O-acetyl-D-glucopyranose (1) (1 g, 2.56 mmol) and tin(IV) chloride (SnCl4) (419 μL, 3.58 mmol, 1.4 equiv) were then added and the reaction mixture was stirring under reflux for 2 more h, cooled, neutralized with aqueous sodium bicarbonate, and extracted with ethyl acetate (1000 mL). The organic extract was dried over anhydrous sodium sulfate, filtered, and evaporated to dryness. The residue was purified by flash column chromatography (EtOAc/ hexane 7:3) to give compound 2, as a white solid (814 mg, 56%); mp 169–171°C; [α]22D = −6 (c 0.2, CHCl3); Rf = 0.47 (EtOAc); λ max 280 nm (ε 20557); 1H NMR (CDCl3,300 MHz): δ 8.83 (s, 1H, H-2), 8.22 (s, 1H, H-8), 8.04-7.51 (m, 5H, Bz), 5.98 (d, 1H, J1′,2′ = 9.4 Hz, H-1′), 5.65 (t, 1H, J = 9.3 Hz, H-3′), 5.50 (t, 1H, J = 9.4 Hz, H-2′), 5.32 (t, 1H, J = 9.7 Hz, H-4′), 4.34 (dd, 1H, J5′,6a′ = 4.1 Hz, J6a′,6b′ = 12.8 Hz, H-6a′), 4.24-4.14 (m, 1H, H-6b′), 4.11-4.02 (m, 1H, H-5′), 2.08, 2.07, 2.04, 1.79 (4s, 12H, 4OAc);13C NMR (CDCl3, 75.5 MHz): δ 170.4, 169.8, 169.3, 169.0, 164.5, 152.0, 151.9, 149.5, 140.9, 133.1, 133.0, 128.9, 128.1, 122.2, 80.6, 75.2, 72.7, 70.3, 67.7, 61.5, 20.6, 20.5, 20.4, 20.1; Mass (M+H)+: 570.16; Anal. Calcd. for C26H27N5O10: C, 54.83; H, 4.78; N, 12.30%. Found: C, 55.16; H, 4.66; N, 12.53%.
9-(2′,3′,4′,6′-Tetra-O-Acetyl-β-D-Glucopyranosyl)- 8-Bromo-N6-Benzoyl Adenine (3)
To a solution of 9-(2′,3′,4′,6′-tetra-O-acetyl-β-D-glucopyranosyl)-N6-benzoyl adenine (2) (250 mg, 0.44 mmol), and sodium acetate (172 mg, 2.1 mmol) in 1.6 mL of glacial acetic acid, 75 μL, 1.45 mmol of bromine were added. The reaction was left to stir at room temperature until completion (14 h). The whole was extracted with ethyl acetate and satured Na2S2O3. The organic layer was washed with water and brine, dried over anhydrous sodium sulfate, filtered, and evaporated to dryness. The residue was purified by flash column chromatography (EtOAc/ hexane 7:3) to give compound 3, (171 mg, 60%); [α]22D = −3 (c 0.2, CHCl3); Rf = 0.45 (EtOAc/ hexane 7:3); λmax 282 nm (ε 21223); 1H NMR (CDCl3,300 MHz): δ 8.82 (s, 1H, H-2), 8.04-7.49 (m, 5H, Bz), 6.21 (t, 1H, J = 9.6 Hz, H-2′), 5.96 (d, 1H, J1′,2′ = 9.6 Hz, H-1′), 5.47 (t, 1H, J = 9.4 Hz, H-3′), 5.35 (t, 1H, J = 9.9 Hz, H-4′), 4.26-4.25 (m, 2H, H-6a′, H-6b′), 4.06-3.96 (m, 1H, H-5′), 2.09, 2.07, 2.04, 1.79 (4s, 12H, 4OAc);13C NMR (CDCl3, 75.5 MHz): δ 170.5, 170.0, 169.9, 169.3, 164.7, 153.3, 152.9, 149.2, 133.3, 131.0, 128.9, 128.6, 128.2, 122.3, 78.2, 72.9, 68.8, 67.6, 67.5, 61.5, 20.7, 20.6, 20.5, 20.2; Mass (M+H)+: 648.08; Anal. Calcd. for C26H26BrN5O10: C, 48.16; H, 4.04; Br, 12.32; N, 10.80%. Found: C, 48.44; H, 4.15; Br, 12.11; N, 10.99%.
General Experimental Procedure for the Preparation of Protected C8-Alkynyl Adenine Pyranonucleosides (4a–e)
The appropriate alkynes (3 equiv), Pd(PPh3)4 (43 mg, 0.1 equiv), CuI (7 mg, 0.1 equiv), triethylamine (103 μL, 2 equiv) and 9-(2′,3′,4′,6′-tetra-O-acetyl-β-D-glucopyranosyl)-8-bromo-N6-benzoyl adenine (3) (240 mg, 0.37 mmol), were irradiated under microwaves (200 W) in 1 mL of anhydrous DMF for 10 min at 120°C. The reaction mixture was concentrated under reduced pressure and the crude residue was purified by flash chromatography on silica gel. The purified material was dried in vacuo to afford the corresponding derivatives 4a–e, as colorless foams.
9-(2′,3′,4′,6′-Tetra-O-Acetyl-β-D-Glucopyranosyl)-8-Heptynyl-N6-Benzoyl Adenine (4a)
139 mg, 56%; [α]22D = −4 (c 0.4, CHCl3); Rf = 0.10 (EtOAc/ hexane 6:4); λmax 303 nm (ε 22594); 1H NMR (CDCl3,300 MHz): δ 8.99 (br s, 1H, NH), 8.85 (s, 1H, H-2), 8.03-7.41 (m, 5H, Bz), 6.28 (t, 1H, J = 9.4 Hz, H-2′), 5.95 (d, 1H, J1′,2′ = 9.2 Hz, H-1′), 5.46 (t, 1H, J = 9.3 Hz, H-3′), 5.35 (t, 1H, J = 9.3 Hz, H-4′), 4.28-4.19 (m, 2H, H-6a′, H-6b′), 4.04-3.87 (m, 1H, H-5′), 2.62 (t, 2H, J = 7.1 Hz, α-CH2), 2.09, 2.08, 2.05, 1.78 (4s, 12H, 4OAc), 1.56-1.35 (m, 6H, 3 × CH2), 0.96 (t, 3H, J = 7.2 Hz, CH3);13C NMR (CDCl3, 75.5 MHz): δ 170.8, 170.5, 170.0, 169.8, 165.0, 153.5, 152.2, 151.3, 148.8, 134.9, 133.0, 129.2, 127.9, 119.9, 97.7, 94.5, 82.9, 70.8, 70.1, 67.5, 67.2, 63.7, 31.5, 31.2, 29.6, 22.3, 21.5, 21.3, 21.0, 20.9, 15.2; Mass (M+H)+: 664.26; Anal. Calcd. for C33H37N5O10: C, 59.72; H, 5.62; N, 10.55%. Found: C, 59.96; H, 5.94; N, 10.65%.
9-(2′,3′,4′,6′-Tetra-O-Acetyl-β-D-Glucopyranosyl)-8-Phenylethynyl-N6-Benzoyl Adenine (4b)
161 mg, 66%; [α]22D = −6 (c 0.4, CHCl3); Rf = 0.33 (CH2Cl2/ MeOH 9.8:0.2); λmax 300 nm (ε 29706); 1H NMR (CDCl3, 300 MHz): δ 8.95 (br s, 1H, NH), 8.87 (s, 1H, H-2), 8.01-7.45 (m, 10H, Bz and Ph), 6.31 (t, 1H, J = 9.3 Hz, H-2′), 6.10 (d, 1H, J1′,2′ = 9.4 Hz, H-1′), 5.49 (t, 1H, J = 9.2 Hz, H-3′), 5.35 (t, 1H, J = 9.5 Hz, H-4′), 4.31-4.22 (m, 2H, H-6a′, H-6b′), 4.08-3.98 (m, 1H, H-5′), 2.09, 2.05, 1.90, 1.77 (4s, 12H, 4OAc);13C NMR (CDCl3, 75.5 MHz): δ 170.6, 170.3, 169.9, 169.7, 165.3, 153.1, 152.4, 150.5, 148.5, 134.5, 133.8, 133.5, 129.9, 129.5, 129.0, 128.3, 123.6, 119.3, 102.6, 98.7, 87.3, 83.6, 70.5, 67.9, 67.5, 63.5, 21.5, 21.3, 20.9, 20.5; Mass (M+H)+: 670.18; Anal. Calcd. for C34H31N5O10: C, 60.98; H, 4.67; N, 10.46%. Found: C, 61.23; H, 4.28; N, 10.80%.
9-(2′,3′,4′,6′-Tetra-O-Acetyl-β-D-Glucopyranosyl)-8-P-Tolylethynyl-N6-Benzoyl Adenine (4c)
157 mg, 61%; [α]22D = −10 (c 0.3, CHCl3); Rf = 0.37 (EtOAc/ hexane 8:2); λmax 325 nm (ε 27336); 1H NMR (CDCl3, 300 MHz): δ 8.98 (br s, 1H, NH), 8.86 (s, 1H, H-2), 8.02-7.29 (m, 9H, Bz and ArH), 6.31 (t, 1H, J = 9.4 Hz, H-2′), 6.08 (d, 1H, J1′,2′ = 9.3 Hz, H-1′), 5.48 (t, 1H, J = 9.4 Hz, H-3′), 5.37 (t, 1H,J = 9.7 Hz, H-4′), 4.29-4.22 (m, 2H, H-6a′, H-6b′), 4.07-3.98 (m, 1H, H-5′), 2.43 (s, 3H, CH3), 2.09, 2.05, 1.91, 1.77 (4s, 12H, 4OAc);13C NMR (CDCl3, 75.5 MHz): δ 170.3, 170.1, 169.7, 169.3, 165.1, 153.2, 152.3, 150.7, 148.7, 139.5, 135.8, 133.7, 133.4, 129.6, 129.2, 128.8, 120.3, 120.1, 102.4, 98.3, 87.6, 83.5, 70.7, 67.5, 67.1, 63.7, 22.5, 21.7, 21.5, 21.0, 20.2; Mass (M+H)+: 684.22; Anal. Calcd. for C35H33N5O10: C, 61.49; H, 4.87; N, 10.24%. Found: C, 61.84; H, 4.52; N, 10.02%.
9-(2′,3′,4′,6′-Tetra-O-Acetyl-β-D-Glucopyranosyl)-8-(Pyridin-3-yl-Ethynyl)-N6-Benzoyl Adenine (4d)
141 mg, 58%; [α]22D = −12 (c 0.2, CHCl3); Rf = 0.23 (EtOAc/ hexane 6:4); λmax 320 nm (ε 16156); 1H NMR (CDCl3, 300 MHz): δ 8.99 (br s, 1H, NH), 8.87 (s, 1H, H-2), 8.72-7.39 (m, 9H, Bz and pyridine), 6.26 (t, 1H, J = 9.5 Hz, H-2′), 6.11 (d, 1H, J1′,2′ = 9.3 Hz, H-1′), 5.48 (t, 1H, J = 9.3 Hz, H-3′), 5.35 (t, 1H, J = 9.7 Hz, H-4′), 4.32 (dd, 1H,J5′,6a′ = 4.7 Hz, J6a′,6b′ = 12.5 Hz, H-6a′), 4.21 (dd, 1H,J5′,6b′ = 2.0 Hz, J 6a′,6b′ = 12.5 Hz, H-6b′), 4.09-4.00 (m, 1H, H-5′), 2.09, 2.05, 1.94, 1.78 (4s, 12H, 4OAc);13C NMR (CDCl3, 75.5 MHz): δ 170.4, 170.0, 169.6, 169.2, 165.4, 153.0, 152.7, 151.6, 150.7, 150.5, 148.8, 140.5, 135.4, 133.6, 129.5, 128.2, 124.6, 120.5, 117.1, 98.7, 95.6, 83.3, 75.7, 70.5, 67.5, 67.2, 63.5, 21.3, 21.0, 20.9, 20.7; Mass (M+H)+: 671.21; Anal. Calcd. for C33H30N6O10: C, 59.10; H, 4.51; N, 12.53%. Found: C, 59.42; H, 4.76; N, 12.22%.
9-(2′,3′,4′,6′-Tetra-O-Acetyl-β-D-Glucopyranosyl)-8-(Pyridin-2-yl-Ethynyl)-N6-Benzoyl Adenine (4e)
127 mg, 50%; [α]22D = −2 (c 0.1, CHCl3); Rf = 0.21 (EtOAc/ hexane 7:3); λmax 315 nm (ε 22103); 1H NMR (CDCl3, 300 MHz): δ 8.80 (br s, 2H, NH, H-2), 8.07-7.35 (m, 9H, Bz and pyridine), 6.31 (t, 1H,J = 9.4 Hz, H-2′), 6.12 (d, 1H, J1′,2′ = 9.3 Hz, H-1′), 5.71 (t, 1H, J = 9.5 Hz, H-3′), 5.48 (t, 1H, J = 9.5 Hz, H-4′), 4.29-4.25 (m, 2H, H-6a′, H-6b′), 4.10-4.02 (m, 1H, H-5′), 2.09, 2.03, 1.92, 1.75 (4s, 12H, 4OAc);13C NMR (CDCl3, 75.5 MHz): δ 170.6, 170.3, 169.7, 169.5, 164.3, 153.2, 152.8, 151.5, 149.9, 148.3, 145.2, 139.5, 134.6, 132.7, 128.6, 127.8, 127.6, 123.7, 119.7, 95.7, 92.5, 84.8, 82.7, 72.8, 69.5, 68.3, 65.7, 21.0, 20.7, 20.6, 20.5; Mass (M+H)+: 671.20; Anal. Calcd. for C33H30N6O10: C, 59.10; H, 4.51; N, 12.53%. Found: C, 59.40; H, 4.73; N, 12.34%.
General Procedure for the Preparation of Unprotected C8-Alkynyl Adenine Pyranonucleosides (5a–d)
The protected nucleosides 4a–e (0.5 mmol), were treated with methanolic ammonia (satured at 0°C, 27.9 mL). The solution was stirred overnight at room temperature and then evaporated under reduced pressure. The residue was purified by flash column chromatography to afford the unprotected derivatives 5a–d, in 60–72% yields, as yellowish and white foams.
9-(β-D-Glucopyranosyl)-8-Heptynyl-Adenine (5a)
117 mg, 60%; [α]22D = −2 (c 0.2, MeOH); Rf = 0.17 (EtOAc/ MeOH 8:2) λmax 292 nm (ε 19971); 1H NMR (DMSO-d6, 300 MHz): δ 8.15 (s, 1H, H-2), 7.24 (s, 2H, NH2), 5.43 (d, 1H, J1′,2′ = 9.5 Hz, H-1′), 5.16, 5.09, 5.00 (3br s, 3H, 3OH), 4.60-4.45 (m, 2H, OH, H-2′), 3.81-3.34 (m, 5H, H-3′, H-4′, H-5′, H-6a′, H-6b′), 1.78-1.76 (m, 2H, β-CH2), 1.63 (t, 2H, J = 7.2 Hz, α-CH2), 1.51-1.30 (m, 4H, 2 × CH2), 0.93 (t, 3H, J = 7.2 Hz, CH3). 13C NMR (DMSO-d6, 75.5 MHz): δ 156.8, 152.7, 150.1, 148.3, 119.9, 96.2, 93.9, 83.2, 78.8, 73.6, 70.5, 66.7, 65.4, 30.9, 29.2, 22.3, 19.0, 14.5; Mass (M+H)+: 392.20; Anal. Calcd. for C18H25N5O5: C, 55.23; H, 6.44; N, 17.89%. Found: C, 54.90; H, 6.73; N, 17.72%.
9-(β-D-Glucopyranosyl)-8-Phenylethynyl-Adenine (5b)
143 mg, 72%; [α]22D = -6 (c 0.1, MeOH); Rf = 0.30 (EtOAc/ MeOH 9:1); λmax 313 nm (ε 14209); 1H NMR (DMSO-d6, 300 MHz): δ 8.19 (s, 1H, H-2), 7.66 (br s, 2H, NH2), 7.61-7.47 (m, 5H, Ph), 5.55 (d, 1H, J1′,2′ = 9.2 Hz, H-1′), 5.40 (d, 1H, J = 5.3 Hz, OH), 5.34 (d, 1H, J = 4.1 Hz, OH), 5.21 (d, 1H, J = 5.1 Hz, OH), 4.70 (t, 1H, J= 4.4 Hz, OH), 4.54-4.40 (m, 1H, H-2′), 3.81-3.39 (m, 5H, H-3′, H-4′, H-5′, H-6a′, H-6b′). 13C NMR (DMSO-d6, 75.5 MHz): δ 156.3, 152.6, 150.0, 148.1, 132.7, 128.9, 128.7, 123.0, 119.7, 101.9, 96.3, 86.8, 83.3, 78.1, 73.4, 70.8, 65.8; Mass (M+H)+: 398.15; Anal. Calcd. for C19H19N5O5: C, 57.43; H, 4.82; N, 17.62%. Found: C, 57.81; H, 4.51; N, 17.90%.
9-(β-D-Glucopyranosyl)-8-p-Tolylethynyl-Adenine (5c)
140 mg, 67%; [α]22D = −4 (c 0.1, MeOH); Rf = 0.21 (EtOAc/ MeOH 8:2); λmax 316 nm (ε 12871); 1H NMR (DMSO-d6, 300 MHz): δ 8.18 (s, 1H, H-2), 7.55 (d, 2H, J = 8.1 Hz, ArH), 7.37 (br s, 2H, NH2), 7.32 (d, 2H, J = 8.0 Hz, ArH), 5.55 (d, 1H, J1′,2′ = 9.3 Hz, H-1′), 5.25 (d, 1H, J= 4.1 Hz, OH), 5.15, 5.04 (2br s, 2H, 2OH), 4.58-4.47 (m, 2H, OH, H-2′), 3.81-3.34 (m, 5H, H-3′, H-4′, H-5′, H-6a′, H-6b′), 2.38 (s, 3H, CH3). 13C NMR (DMSO-d6, 75.5 MHz): δ 156.4, 152.1, 150.4, 148.5, 138.5, 132.5, 129.0, 122.3, 120.2, 101.8, 96.0, 86.9, 83.0, 78.9, 73.5, 70.7, 65.6, 24.6; Mass (M+H)+: 412.17; Anal. Calcd. for C20H21N5O5: C, 58.39; H, 5.14; N, 17.02%. Found: C, 58.72; H, 5.45; N, 17.27%.
9-(β-D-Glucopyranosyl)-8-(Pyridin-3-yl-Ethynyl)-Adenine (5d)
139 mg, 70%; [α]22D = +2 (c 0.2, MeOH); Rf = 0.16 (CH2Cl2/ MeOH 7:3); λmax 306 nm (ε 7353); 1H NMR (DMSO-d6, 300 MHz): δ 8.89-8.68 (m, 2H, pyridine), 8.21 (s, 1H, H-2), 8.10-7.52 (m, 2H, pyridine), 7.44 (br s, 2H, NH2), 5.58 (d, 1H, J1′,2′ = 9.3 Hz, H-1′), 5.28, 5.07, 4.56 (3 br s, 3H, 3OH), 4.45 (t, 1H,J = 8.1 Hz, OH), 3.81-3.40 (m, 6H, H-2′, H-3′, H-4′, H-5′, H-6a′, H-6b′). 13C NMR (DMSO-d6, 75.5 MHz): δ 156.9, 152.5, 152.3, 150.5, 149.8, 148.5, 139.9, 123.8, 119.8, 116.8, 96.0, 95.7, 83.1, 78.7, 74.1, 73.9, 70.2, 65.2; Mass (M+H)+: 399.15; Anal. Calcd. for C18H18N6O5: C, 54.27; H, 4.55; N, 21.10%. Found: C, 54.62; H, 4.74; N, 21.38%.
8-Phenylethynyl-Adenine (II)
8-Bromoadenine (I) (43 mg, 0.2 mmol) was mixed with anhydrous DMF (1 mL), phenylacetylene (66 μL, 3 equiv), triethylamine (55 μL, 2 equiv), Pd(PPh3)4 (23 mg, 0.1 equiv), CuI (11 mg, 0.3 equiv) and irradiated with microwaves (200 W) for 6 min at 60°C. After removing volatiles in vacuo, the solid residue was purified by flash chromatography (CH2Cl2/MeOH, 9.5:0.5) to provide compound II, as yellowish foam. 19 mg, 40%; [α]22D = −2 (c 0.2, MeOH); Rf = 0.13 (CH2Cl2/ MeOH 8.5:1.5); λmax 308 nm (ε 9701); 1H NMR (DMSO-d6, 300 MHz): δ 13.38 (br s, 1H, NH), 8.15 (s, 1H, H-2), 7.65-7.49 (m, 5H, Ph), 7.27 (br s, 2H, NH2). 13C NMR (DMSO-d6, 75.5 MHz): δ 156.8, 154.2, 152.6, 148.1, 132.7, 129.1, 129.0, 123.0, 119.8, 101.8, 67.1; Mass (M+H)+: 236.08; Anal. Calcd. for C13H9N5: C, 66.37; H, 3.86; N, 29.77%. Found: C, 66.59; H, 4.01; N, 29.40%.
Antiproliferative Assay
Compounds 4e, 5a–d, and II, were evaluated for their cytostatic activity against human cervix carcinoma (HeLa) cells, human lymphocytes (CEM) as well as murine leukemia (L1210) cells. All assays were performed in 96-well microtiter plates. To each well (5–7.5) × 104 tumor cells were added and varying concentrations of the test compounds ranging from 250, 50, 10, 2, 0.4, to 0.08 μM. The tumor cells were allowed then to proliferate at 37°C in a humidified CO2-controlled atmosphere. To obtain their optimal growth curves this is for 2 days of the murine leukemia (L1210) cells and for 3 days for the human lymphocytic (CEM) cells and the human cervix carcinoma (HeLa) cells. At the end of the incubation period, the cells were counted in a Coulter counter. The IC50 (50% inhibitory concentration) was defined as the concentration of the compound that inhibited cell proliferation by 50%. Experiments were repeated at least three times and these data are presented in Table 1.
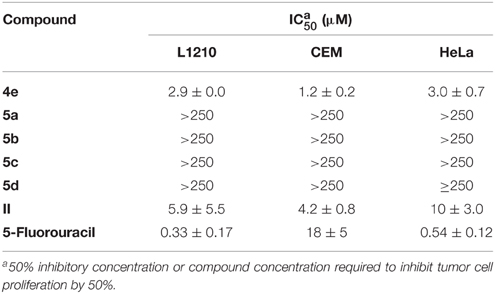
Table 1. Cytostatic activity of the compounds against tumor cell (L1210, CEM, and HeLa) proliferation.
Results and Discussion
Herein, we describe the synthesis of C8-alkynyl adenine pyranonucleosides and present their biological properties. The starting material of our synthesis was the commercially available per-O-acetylated D-glucose 1 which upon coupling, via two-step Vorbrüggen method (Vorbrüggen and Höfle, 1981), with N6-benzoyl adenine, gave selectively the N9-isomeric adenine nucleoside 2, under thermodynamically controlled conditions (SnCl4/CH3CN, reflux). Trans rule was followed (Baker, 1957) and the β-configured nucleoside 2 was solely obtained as deduced from 1H NMR vicinal coupling data (J1′,2′ = 9.4 Hz). Since halogenated C8-purine nucleosides have proven useful intermediates for the efficient preparation of their corresponding C8-alkynyl derivatives (Agrofoglio et al., 2003), the adenine nucleoside 2 was directly brominated after treatment with Br2/AcOH/AcONa (Holmes and Robins, 1964), affording the 8-bromo analog 3, in approximately 60% yield after aqueous work-up and flash chromatography. The position of substitution of the bromine atom was further confirmed by the absence of the characteristic sharp absorption peak at 8.22 ppm due to H-8 of nucleoside 2, while the syn conformation for adenine was induced due to the bulky bromo substituent at C8 position (Sarma et al., 1974). 8-Alkynyl adenine nucleosides 4 were accessed, through Sonogashira cross-coupling reaction of intermediate 3 with several terminal acetylenes, under microwave irradiation (200 W) (Figure 1). In a typical experiment, an effective catalyst (Pd(PPh3)4)/co-catalyst (CuI) combination proved to be (1:1) ratio (Meneni et al., 2007) affording 4a–e, in satisfactory yields (50–66%). To provide a detailed structure-activity relationship studies, diverse alkyne substituents R were selected which include linear alkyl chains (4a, R = n-pentyl), aromatic rings (4b, R = phenyl, 4c, R = p-tolyl) and pyridine moieties (4d, R = 3-pyridyl, 4e, R = 2-pyridyl). Finally, total deprotection of 4a–e by the action of saturated methanolic ammonia afforded only the target derivatives 5a–d, while attempts to remove all protecting groups from 4e either with sodium methoxide (Bozó et al., 1998) or potassium carbonate-methanol (Plattner et al., 1972) resulted in a mixture of intractable and inseparable materials.
Based on the promising cytotoxic activity profile of our prior synthesized C5-phenylethynyl uracil glucopyranonucleoside (Dimopoulou et al., 2013) and in order to explore the impact of the glycosidic part on the biological activity as well as the potential inhibitory effects of adenine moiety, we sought to introduce phenylacetylene substituent in 8-position of adenine itself. Therefore, we investigated the development of the efficient Sonogashira alkynylation protocol for the cross-coupling of commercially available 8-bromoadenine (I) with phenylacetylene under microwave irradiation (200 W) (Figure 2). 8-Bromoadenine (I) was mixed with anhydrous DMF, phenylacetylene, triethylamine, Pd(PPh3)4, CuI, irradiated with microwaves for 6 min at 60°C and after removing volatiles in vacuo, the solid residue was purified by flash chromatography to provide compound II, in acceptable yield (40%).
Cytostatic Activity
The cytostatic activity of the novel C8-modified adenine pyranonucleosides 4e, 5a–d as well as 8-phenylethynyl-adenine (II) was determined against murine leukemia (L1210), human lymphocyte (CEM) and human cervix carcinoma (HeLa) cell cultures (Table 1). Compounds 4e and II were less cytostatic than 5-fluorouracil (almost an order of magnitude) against murine leukemia (L1210) and human cervix carcinoma (HeLa) cells, while the same compounds proved to be more active than 5-fluorouracil against human lymphocyte (CEM) cells.
Conclusion
In summary, we have prepared several novel C8-alkynyl adenine nucleosides as well as 8-phenylethynyl-adenine, via Sonogashira coupling conditions under microwave irradiation. Among the compound series tested, the protected adenine pyranonucleoside 4e, as well as phenylethynyl adenine (II) showed significant cytotoxicity (IC50 of 1.2–10.0 μM) against murine leukemia (L1210), human lymphocyte (CEM) and human cervix carcinoma (HeLa) cell cultures. Since the glucose derivative of phenylethynyl adenine, nucleoside 5b, showed no activity, it is clear that it is stable and not susceptible to hydrolysis. The replacement of glucose with ribo, arabino, and deoxyribose moieties as well as the introduction of functional substituents on the phenyl ring, such as halogens, nitro or amino and alkyl groups, could be explored in the future in an attempt to further increase the cytostatic potential of these lead compounds.
Conflict of Interest Statement
The authors declare that the research was conducted in the absence of any commercial or financial relationships that could be construed as a potential conflict of interest.
Acknowledgments
This work was supported in part by the Postgraduate Programmes “Biotechnology-Quality assessment in Nutrition and the Environment”, “Application of Molecular Biology-Molecular Genetics- Molecular Markers”, Department of Biochemistry and Biotechnology, University of Thessaly, and the KU Leuven (GOA 10/14). We thank Lizette van Berckelaer for her excellent technical assistance in the biological assays.
References
Agrofoglio, L. A., Gillaizeau, I., and Saito, Y. (2003). Palladium-assisted routes to nucleosides. Chem. Rev. 103, 1875–1916. doi: 10.1021/cr010374q
PubMed Abstract | Full Text | CrossRef Full Text | Google Scholar
Baker, B. R. (1957). “Stereochemistry of nucleoside synthesis,” in Ciba Foundation Symposium on the Chemistry and Biology of Purines, eds G. E. W. Wohlstenholme and C. M. O′Connor (London: John Wiley & Sons), 120–129.
Bozó, E., Boros, S., Kuszmann, J., Gács-Baitz, E., and Párkányi, L. (1998). An economic synthesis of 1,2,3,4-tetra-O-acetyl-5-thio-D-xylopyranose and its transformation into 4-substituted-phenyl 1,5-dithio-D-xylopyranosides possessing antithrombotic activity. Carbohydr. Res. 308, 297–310. doi: 10.1016/S0008-6215(98)00088-3
PubMed Abstract | Full Text | CrossRef Full Text | Google Scholar
Dimopoulou, A., Manta, S., Kiritsis, C., Gkaragkouni, D. N., Papasotiriou, I., Balzarini, J., et al. (2013). Rapid microwave-enhanced synthesis of C5-alkynyl pyranonucleosides as novel cytotoxic antitumor agents. Bioorg. Med. Chem. Lett. 23, 1330–1333 doi: 10.1016/j.bmcl.2012.12.092
PubMed Abstract | Full Text | CrossRef Full Text | Google Scholar
Escuret, V., Aucagne, V., Joubert, N., Durantel, D., Rapp, L. K., Schinazi, F. R., et al. (2005). Synthesis of 5-haloethynyl- and 5-(1,2-dihalo)vinyluracil nucleosides: antiviral activity and cellular toxicity. Bioorg. Med. Chem. 13, 6015–6024. doi: 10.1016/j.bmc.2005.06.021
PubMed Abstract | Full Text | CrossRef Full Text | Google Scholar
Hatse, S., De Clercq, E., and Balzarini, J. (1999). Role of antimetabolites of purine and pyrimidine nucleotide metabolism in tumor cell differentiation. Biochem. Pharmacol. 58, 539–555. doi: 10.1016/S0006-2952(99)00035-0
PubMed Abstract | Full Text | CrossRef Full Text | Google Scholar
Herdewijn, P. (2008). Modified Nucleosides in Biochemistry, Biotechnology and Medicine. Weinheim: Wiley-VCH Verlag GmbH & Co. KGaA.
Holmes, E. R., and Robins, K. R. (1964). Purine nucleosides. VII. Direct bromination of adenosine, deoxyadenosine, guanosine, and related purine nucleosides. J. Am. Chem. Soc. 86, 1242–1245. doi: 10.1021/ja01060a057
Kantsadi, A. L., Manta, S., Psara, A. M., Dimopoulou, A., Kiritsis, C., Parmenopoulou, V., et al. (2012). The binding of C5-alkynyl and alkylfurano[2,3-d]pyrimidine glucopyranonucleosides to glycogen phosphorylase b: synthesis, biochemical and biological assessment. Eur. J. Med. Chem. 54, 740–749. doi: 10.1016/j.ejmech.2012.06.029
PubMed Abstract | Full Text | CrossRef Full Text | Google Scholar
Lee, Y. S., Park, S. M., Kim, H. M., Park, S. K., Lee, K., Lee, C. W., et al. (2009). C5-Modified nucleosides exhibiting anticancer activity. Bioorg. Med. Chem. Lett. 19, 4688–4691. doi: 10.1016/j.bmcl.2009.06.072
PubMed Abstract | Full Text | CrossRef Full Text | Google Scholar
Lin, T. S., Cheng, J. C., Ishiguro, K., and Sartorelli, A. C. (1985). Purine and 8-substituted purine arabinofuranosyl and ribofuranosyl nucleoside derivatives as potential inducers of the differentiation of the Friend erythroleukemia. J. Med. Chem. 28, 1481–1485. doi: 10.1021/jm00148a018
PubMed Abstract | Full Text | CrossRef Full Text | Google Scholar
Manta, S., Kiritsis, C., Dimopoulou, A., Parmenopoulou, V., Kollatos, N., Tsotinis, A., et al. (2014). Unsaturation: an important structural feature to nucleosides′ antiviral activity. Anti Infect. Agents 12, 2–57. doi: 10.2174/22113525113119990106
Meneni, S., Ott, I., Sergeant, C. D., Sniady, A., Gust, R., and Dembinski, R. (2007). 5-Alkynyl-2′-deoxyuridines: chromatography-free synthesis and cytotoxicity evaluation against human breast cancer cells. Bioorg. Med. Chem. 15, 3082–3088. doi: 10.1016/j.bmc.2007.01.048
PubMed Abstract | Full Text | CrossRef Full Text | Google Scholar
Plattner, J. J., Gless, R. D., and Rapoport, H. (1972). Synthesis of some DE and CDE ring analogs of camptothecin. J. Am. Chem. Soc. 94, 8613–8615. doi: 10.1021/ja00779a072
PubMed Abstract | Full Text | CrossRef Full Text | Google Scholar
Sarma, H. R., Lee, C.-H., Evans, E. F., Yathindra, N., and Sundaralingam, M. (1974). Probing the interrelation between the glycosyl torsion, sugar pucker, and the backbone conformation in C(8) substituted adenine nucleotides by 1H and 1H-{31P} fast fourier transform nuclear magnetic resonance methods and conformational energy calculations. J. Am. Chem. Soc. 96, 7337–7348. doi: 10.1021/ja00830a028
PubMed Abstract | Full Text | CrossRef Full Text | Google Scholar
Vivet-Boudou, V., Isel, C., Sleiman, M., Smyth, R., Ben Gaied, N., Barhoum, P., et al. (2011). 8-Modified-2′-deoxyadenosine analogues induce delayed polymerization arrest during HIV-1 reverse transcription. PLoS ONE 6:e27456. doi: 10.1371/journal.pone.0027456
Volpini, R., Costanzi, S., Lambertucci, C., Vittori, S., Klotz, K., Lorenzen, A., et al. (2001). Introduction of alkynyl chains on C-8 of adenosine led to very selective antagonists of the A(3) adenosine receptor. Bioorg. Med. Chem. Lett. 11, 1931–1934. doi: 10.1016/S0960-894X(01)00347-X
PubMed Abstract | Full Text | CrossRef Full Text | Google Scholar
Keywords: Sonogashira coupling reaction, 8-bromoadenine, N6-benzoyladenine pyranonucleosides, microwave irradiation, cytotoxic activity
Citation: Dimopoulou A, Manta S, Parmenopoulou V, Kollatos N, Christidou O, Triantakonstanti VV, Schols D and Komiotis D (2015) An easy microwave-assisted synthesis of C8-alkynyl adenine pyranonucleosides as novel cytotoxic antitumor agents. Front. Chem. 3:21. doi: 10.3389/fchem.2015.00021
Received: 04 December 2014; Accepted: 05 March 2015;
Published: 23 March 2015.
Edited by:
Stefano D'Errico, Università degli Studi di Napoli ‘Federico II’, ItalyCopyright © 2015 Dimopoulou, Manta, Parmenopoulou, Kollatos, Christidou, Triantakonstanti, Schols and Komiotis. This is an open-access article distributed under the terms of the Creative Commons Attribution License (CC BY). The use, distribution or reproduction in other forums is permitted, provided the original author(s) or licensor are credited and that the original publication in this journal is cited, in accordance with accepted academic practice. No use, distribution or reproduction is permitted which does not comply with these terms.
*Correspondence: Dimitri Komiotis, Laboratory of Bioorganic Chemistry, Department of Biochemistry and Biotechnology, University of Thessaly, 26 Ploutonos Str., 41221 Larissa, Greece dkom@bio.uth.gr