- 1BIOTEC-JGSEE Integrated Biorefinery Laboratory, National Center for Genetic Engineering and Biotechnology, Khlong Luang, Thailand
- 2Biorefinery and Bioproduct Technology Research Group, National Center for Genetic Engineering and Biotechnology, Khlong Luang, Thailand
- 3The Joint Graduate School of Energy and Environment, King Mongkut’s University of Technology Thonburi, Bangkok, Thailand
- 4National Metal and Materials Technology Center (MTEC), National Science and Technology Development Agency, Khlong-Nueng, Thailand
Organosolv lignin is an emerging bio-additive for creating functional properties in various products with its advantages in high-purity, sulfur-free, biocompatibility, and solubility in green solvents. In this study, effects of thermal and physical modification on alterations of functional properties and particle size distribution of isolated organosolv lignin from sugarcane bagasse (OLB) were studied. Thermal treatment of OLB at increasing temperatures from 170 to 230°C in 70%w/w aqueous ethanol led to alteration of phenolic hydroxyl content, while ultrasonication resulted in homogeneous size distribution of the modified OLB according to laser diffraction and scanning electron micrograph. The highest ultraviolet light absorbance and antioxidant activities were obtained at 190°C treatment which were correlated to the highest phenolic group content. Application of the modified OLB at 3% w/w in a base cream formulation resulted in enhancement of the anti-UV activity to exceed SPF 50 with increasing antioxidant activity in the product. The work provides basis on modification of organosolv lignin for application as a potent functional additive in cosmeceutical products.
1 Introduction
Biorefinery is a promising industry in which lignocellulosic biomass is converted into diverse biobased products through multidisciplinary technologies (Vu et al., 2020). Typically, three major components found in lignocelluloses are cellulose, hemicellulose, and lignin. The polysaccharide constituents (cellulose and hemicellulose) have been used for a wide range of applications such as for production of biofuels, commodity chemicals, and biomaterials through different biological and chemical processes. In contrast, the use of lignin is more challenging due to its heterogeneous and recalcitrant structure. Currently, less than 2% of lignin is used for production of value-added products besides its conventional use in heat and energy generation (Cao et al., 2018).
Lignin valorization has appeared as an essential requirement for a feasible biorefinery platform. Due to its structural complexity, a comprehensive study on lignin chemical structure and their relevant properties is crucial for an effective lignin utilization scheme. Owing to the presence of phenolic groups in its structure, lignin contains free radical scavenging ability which led to an excellent antibacterial and antioxidant properties (Dong et al., 2011; Qian et al., 2015; Kaur et al., 2017; Lu et al., 2022). Moreover, there are abundant UV-absorbing functional groups found on lignin structure such as conjugated phenolics, intramolecular hydrogen bonds, quinoids, and ketones (Lin et al., 2021; Zhang and Naebe, 2021). This led to an increasing interest on application of lignin as a bio-additive for creating functional properties in various products.
The use of lignin as a natural UV-blocker in cosmeceutical products has been recently reviewed (Tran et al., 2021). Studies on cell cytotoxicity of different technical lignins proved their safety on human cells (Ugartondo et al., 2008; Tortora et al., 2014; Gil-Chávez et al., 2019). Several studies on sunscreen formulation using lignin as a functional additive for Sun light protection were reported (Qian et al., 2016; Zhang et al., 2018; Lee et al., 2019). Further modifications on lignin structure, especially on functional group modifications and micromorphology assemble for UV protection enhancement were reported (Qian et al., 2017; Zhang et al., 2019a; Wu et al., 2019; Widsten et al., 2020). In this study, organosolv lignin from ethanosolv processing of sugarcane bagasse obtained in a pre-pilot scale pulp production unit was thermally modified to enhance its UV-absorption and antioxidant properties. The influences of temperature on chemical structures (hydroxyl contents and molecular weights (Mw)) of the modified lignin and their relationship to functional properties were comprehensively studied. Ultrasonication was applied in the precipitation of modified lignin by an anti-solvent to recover a well-distributed microparticle lignin for sub-kilogram scale. The synthesized lignin was used as a functional additive and blended with a base cream for creating antioxidant and SPF enhancing properties. Sunscreen performance of the formulated creams were evaluated and compared with a commercial sunscreen product to demonstrate its potential application in cosmeticeutical products.
2 Methodology
2.1 Raw material
Sugarcane bagasse (BG), from sugarcane harvested in March 2021 (Petchaboon, Thailand), was obtained from Thai Roong Ruang Sugar Mill (Petchaboon, Thailand). The biomass was collected on-site, immediately oven dried at 60°C to achieve less than 8.0 wt% moisture, milled to less than 4 mm in particle size and kept in vacuum sealed packages prior to further experiments. The composition of raw BG was analyzed according to the standard NREL Laboratory Analytical Procedures (LABs) (Sluiter et al., 2010). 2,2-Diphenyl-1-picrylhydrazyl (DPPH), butylated hydroxytoluene (BHT), and 2-chloro-4,4,5,5-tetramethyl1,3,2-dioxaphospholane (TMDP) applied were purchased from Sigma-Aldrich. Analytical grade organic solvents (methanol, ethanol, and tetrahydrofuran) were purchased from RCI Labscan Ltd., Thailand.
2.2 Ethanosolv fractionation of BG
Lignin was isolated from BG by an ethanosolv process using aqueous ethanol (70%v/v) as the solvent in a 50-L high pressure batch reactor. The process parameters including (i) temperature (150–190°C) and (ii) acid catalyst concentration (1.0%–2.0% w/w) were investigated. The biomass was mixed with aqueous ethanol at 10% (w/v) solid loading and heated to the target temperature under an initial pressure of 10 bar by nitrogen gas for 30 min. Afterward, the fractionated BG pulp was separated from the liquid fraction by a stainless filter mesh (50 micron) and washed with distilled water until neutral pH obtained. The pulp was dried at 65°C for 36 h and left in a desiccator until constant weight was achieved. The composition of fractionated BG pulp was analyzed by the standard NREL method mentioned and calculated for mass fraction retained in the pretreated solid. The liquid fraction (black liquor) was collected for subsequent thermal modification and precipitation of lignin by addition of distilled water as an anti-solvent. The separated lignin was collected as powder using a filter press and air dried for further characterization.
2.3 Thermal modification of organosolv lignin
The liquid fraction from ethanosolv process of BG (4 L) was loaded to the 7 L high-pressure reactor (Parr Instruments, Moline, Illinois, United States). The reaction system was pressurized with 10 bars of nitrogen gas and heated to the desired temperature (190°C–230°C) for 1 h with stirring at 100 rpm. Subsequently, various ratios of distilled water were added to the modified lignin solution to precipitate the modified lignin with ultrasonication (690 W Sonication bath, 10–30 min). The lignin suspension obtained was divided into fractions for particle morphology analysis, sunscreen formulation, and filter pressed as powder for further characterization. An overview of the described scheme is shown in Figure 1. The lignin suspension obtained at different ethanol concentrations (distilled water ratio) and at different sonication time were sampled for lignin precipitation yields by vacuum filtration and air dried. The lignin precipitation yields were calculated according to total Klason lignin (Sluiter et al., 2008) in the applied black liquor.
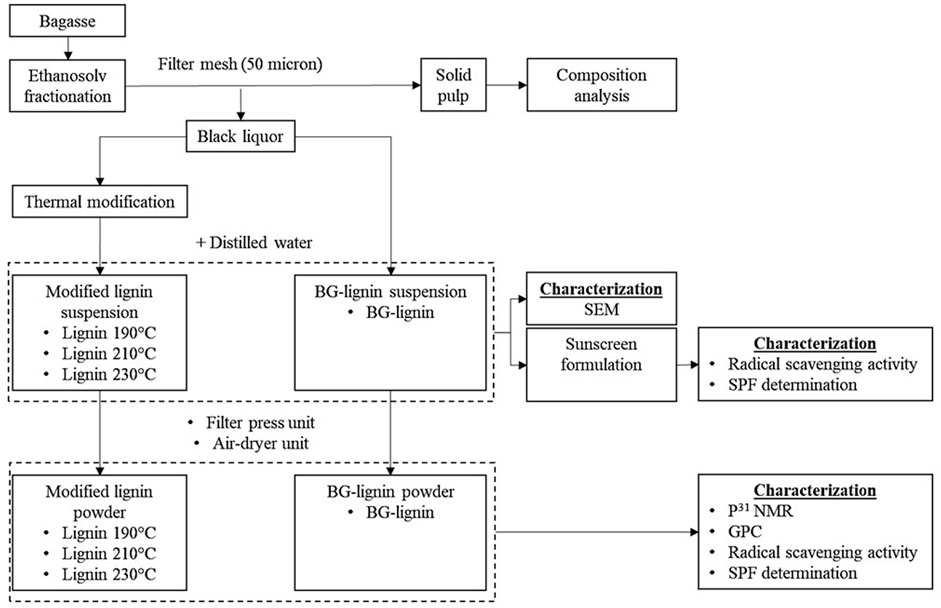
FIGURE 1. Organosolv fractionation and modification scheme os ligin for functional additive development.
2.4 Phosphorus-31 nuclear magnetic resonance analysis
31P-NMR analysis was applied for a quantitative analysis of lignin hydroxyl groups using Bruker Ascend™ 500 MHz spectrometer (Bruker, Zürich, Switzerland). The 31P-NMR solution was prepared according to Meng et al., 2019 (Meng et al., 2019). Endo-N-hydroxy-5-norbornene-2,3-dicarboximide (NHND) was used as internal standard. The internal standard solution was prepared by mixing 5.0 mg of chromium (III) acetylacetonate, 18.0 mg of NHND, and 1.0 mL of solvent mixture (anhydrous pyridine: deuterated chloroform (1.6:1 v/v)). Approximately 30 mg of oven-dried lignin samples were dissolved in 0.5 mL of the solvent mixture and stirred overnight at room temperature to fully dissolve the samples. 0.1 mL of the internal standard solution was then added to the sample solution. The mixed solution was reacted with 0.1 mL of a phosphitylation reagent (2-chloro-4,4,5,5-tetramethyl-1,3,2-dioxaphospholane (TMDP)) for 2 min with stirring. The phosphitylated lignin solution was transferred into a 5 mm NMR tube for 31P NMR acquisitions (inverse gated decoupling pulse sequence, 0.8 s acquisition time, 64 scans, and 25 s pulse delay). Bruker Topspin software was applied for spectra processing and analysis.
2.5 Scanning electron microscopy
The morphology of precipitated lignin (suspension) was analyzed using the S-3400 N Type II SEM (Hitachi, Tokyo, Japan). An electron beam at 10.0 kV energy voltage was applied for the scanning electron micrograph. The lignin suspension samples were dropped on glass slide and air dried prior to the gold coating process for the analysis.
2.6 Size exclusion chromatography
The recovered lignin powder samples were dissolved in tetrahydrofuran (THF) to a final concentration of 2.0 mg/mL and stirred overnight at room temperature to fully dissolve the samples. The dissolved samples were injected to the high-performance liquid chromatography model WATER e2695 (Waters, Milford, MA) through an Agilent PLgel 10 µm MIXED-B column for molecular weight determination. A standard calibration curve was prepared using sodium polystyrene sulfonate standards (1530, 4950, 16,600, and 34,700 g/mol) for weight average molecular weight (Mw) and number average molecular weight (Mn) determination of the acquired chromatograms.
2.7 Lignin blended sunscreen formulation
Lignin blended sunscreen creams were prepared by mixing base cream or cream SPF 30 (Watsons, Thailand) with the lignin suspensions to reach the target lignin concentration (1–5%w/v lignin) and the mixtures were then centrifuged at 1500 rpm for 10 min to remove the suspension supernatant. Subsequently, the lignin blended sunscreen creams were spread onto a 1 × 2 cm strip of 3M Transpore tape to form an even layer of 2.0 mg/cm2 sample and attached to a quartz plate for further SPF determination by UV transmittance analysis (Qian et al., 2015).
2.8 Determination of sun protection factor (SPF)
Lignin powder samples were redissolved in 70%v/v ethanol to reach a final concentration of 1–5%w/v and transferred into a quartz cuvette for UV transmittance analysis. The cuvette with 70%v/v ethanol was used to zero the UV spectrometer Shimadzu UV-3600 (Shimadzu, Kyoto, Japan). In case of the sunscreen cream samples, A quartz plate with 3M Transpore tape without sunscreen cream was used to zero the UV spectrometer. The UV transmittance was measured within wavelength 240–600 nm which cover UVB (290–320 nm) and UVA (320–400 nm) range. For each sample, five spots were scanned and measured for an average transmittance value. The SPF values were calculated according to the following equation:
Where Eλ represents CIE erythemal spectral effectiveness (McKinlay, 1987), Sλ represents solar spectral irradiance (Schmalwieser et al., 2002), and Tλ represents spectral transmittance of the sample.
2.9 Antioxidant activity evaluation
Antioxidant activity of the lignin samples were evaluated in vitro by the reported method using 2,2-diphenyl-1-picrylhydrazyl radicals (DPPH) (Dizhbite et al., 2004). A commercial antioxidant 2,6-Di-tert-butyl-4-methyl-phenol (BHT) was applied as a control antioxidant. One milliliter of sample solution (10–200 µg/mL) was mixed with 2.5 mL of DPPH methanolic solution (100 µg/mL) and was incubated at 25°C in the dark for 40 min. The absorbance of solutions were measured using microplate reader (Enspire® Perkin Elmer, Waltham, Massachusetts, United States) at wavelength 517 nm prior to the incubation (A0) and after 40 min of incubation (A40). All experiments were carried out in triplicate with relative deviations less than 5%. The radical scavenging activity (% RSA) was calculated according to the following equation:
The % RSA was plotted as a function of the sample concentration and determined for IC50 (sample concentration required to achieve 50% RSA).
3 Results and discussion
3.1 Extraction of organosolv lignin from BG
Raw BG used as the starting material in this study contained 23.9%w/w lignin together with cellulose (34.8%w/w) and hemicellulose (33.8%w/w) as the major constituents with 5.0%w/w ash. Treatment of BG by the ethanosolv process at 150°C–175°C in the presence of H2SO4 as an acid catalyst led to an increasing trend of lignin and hemicellulose removal along with the effect on de-ashing, while the cellulose fraction was highly conserved (Figure 2A). Slightly higher lignin content in the solid residue at 190°C was observed which could be due to the repolymerization of lignin and/or saccharide derived compounds into insoluble polymeric compounds (i.e., humin) and these could be misanalysed as being insoluble lignin by the standard NREL method. During hydrothermal pretreatment, the carbonium ion intermediates of fragmented lignin could be repolymerized via C-C formation with other lignin derived molecules and/or saccharide derived furans (furfural and hydroxymethyl furfural) when severe pretreatment conditions were applied (Leschinsky et al., 2008). Addition of H2SO4 markedly resulted in an increasing efficiency of hemicellulose and lignin removal at 175°C compared to the reaction in the absence of acid catalyst (Figure 2B). The reaction condition at 175°C with 1% H2SO4 led to the highest lignin removal of 83.8%w/w as seen by the lowest lignin content in the solid residue. Under this condition, the majority of hemicellulose was solubilized, while 97.1%w/w of cellulose was retained in the solid fraction.
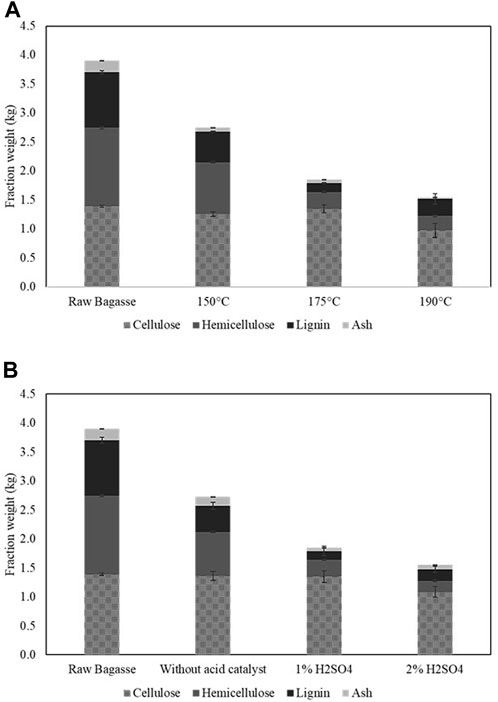
FIGURE 2. Composition of bagasse before and after ethanosolv fractionated at various temperatures (1%H2SO4,60 min) (A) and at various H2SO4 concentration (175°C, 60 min) (B).
Separation of lignin from the liquid phase by precipitation using water as an anti-solvent under this condition led to a lignin yield of 156.1 g/kg BG, equivalent to a recovery yield of 65.3%w/w compared to the lignin content in raw material. The obtained lignin was high in purity (97.5%) based on Klason lignin with a Mw of 2,976 g/mol (Table 1).

TABLE 1. Characteristics and recovery yield of organosolv lignin extracted from bagasse before and after thermal modification.
3.2 Thermal treatment of the extracted organosolv lignin
Since the major product for this organosolv fractionation scheme was paper pulp (cellulose enriched solid pulp, Figure 1) whereas organosolv lignin was considered as value-added product, the current pretreatment conditions were chosen to achieve the highest lignin removal from solid pulp with highest cellulose recovery in pretreated pulp possible. According to our previous studies (Chotirotsukon et al., 2019; Pongchaiphol et al., 2021), the optimized organosolv fractionation conditions for sugarcane-based pulp production were between 165°C and 175°C with pH adjusted to 2.0 using acid catalysts. Pretreatment of bagasse under milder conditions was insufficient to effectively extract lignin from the solid fraction whereas the harsher conditions resulted in excessive cellulose degradation and lignin repolymerization. Therefore, further thermal treatments of black liquor from the optimized organosolv extraction of BG (175°C, 1% H2SO4) at the elevated temperatures (190°C–230°C) were performed after the pretreatment and solid pulp recovery process to acquire both paper pulp and lignin functional additive for a feasible organosolv pulping pre-pilot unit. According to a preliminary analysis, production cost of the organosolv lignin between 6-9 USD/kg was estimated. This suggested potential on using the modified lignin as an additive in cosmetic products while relatively higher cost is acceptable compared to its use in commodity products. Thermal treatments of the organosolv lignin at elevated temperatures resulted in the isolated lignin with lower Mw with decreasing yield after precipitation (Table 1). Hydroxyl groups in the lignin samples were analyzed in detail by 31P-NMR. Aliphatic hydroxyl groups were most abundant in the isolated ethanosolv lignin prior to the thermal modification (BG-lignin) with the OH content of 1.43 mmol/g, followed by C-5 substituted (Syringyl OH, 5-5′, 4-O-5’, and β-5 linkages), p-hydroxyphenyl, and guaiacyl phenolic hydroxyl groups with the OH content of 1.06, 0.95, and 0.6 mmol/g, respectively. Carboxylic hydroxyl groups were found as a minor OH content of 0.24 mmol/g. Modification of lignin by treatment at 190°C–230°C led to decrease in aliphatic OH with remarkable increases in phenolic types of OH groups. Among them, C-5 substituted OH represented the most abundant OH group in the modified lignin obtained at all temperatures (Figure 3). The decreased aliphatic OH and increased phenolic OH content represents the depolymerization of lignin at the dominant lignin linkage (β-O-4 ether linkage) via acid-catalyzed route which results in aliphatic OH loss and phenolic OH augmented molecule (Li et al., 2018). The described hydroxyl group contents found in the thermally modified lignin were coupled with the decreasing trend of lignin molecular weight at the elevated temperature. Increasing treatment temperature led to decreasing trends on phenolic OH, while the carboxylic acid OH was relatively unchanged. This could also be explained by the condensation of lignin derived intermediates where the insoluble polymeric compounds were formed rather than the phenolic OH augmented molecules when severe conditions were applied. The repolymerized polymeric compounds are complex in structure and mostly separated as slurry from the treated black liquor prior to the lignin precipitation by anti-solvent. As a result, the modification of BG extracted lignin at 190°C provides modified lignin with the highest total phenolic OH content of 9.25 mmol/g. Theoretically, lignin polymers were made up of three different monomers including coumaryl-, coniferyl- and sinapyl alcohol which can be fragmented and fully degraded to phenols, guaiacol, and syringol, respectively. These monophenolic products were differently methoxylated thus contain different phenolic-OH content (phenol: 10.62 mmol/g, guaiacol: 8.05 mmol/g, and syringol: 6.49 mmol/g) (Kleinert and Barth, 2008). According to our previous study, lignin recovered from sugarcane bagasse using sulfuric acid catalyzed solvothermal process contain 32, 25, and 42% relative molar abundance of coumaryl-, coniferyl- and sinapyl alcohol, respectively (Imman et al., 2021). Therefore, the theoretical limit of phenolic-OH content for bagasse lignin fragmented mixture was 8.19 mmol/g. Demethylation of guaiacol and syringol to pyrocatechol (phenolic-OH content = 18.16 mmol/g) and pyrogallol (phenolic-OH content = 23.76 mmol/g) could be a possible approach to further increase the phenolic-OH content of bagasse lignin fragmented mixture to a theoretical limit of 18.23 mmol/g. In this study, thermal treatment was applied on organosolv lignin to fragment their polymer structure. At the optimized treatment condition, the modified lignin showed highest phenolic-OH content of 9.25 mmol/g which resulted in the highest antioxidation and UV absorption properties. The result assumes both fragmentation and partial demethylation on the modified lignin recovered. Demethylation of lignin derived compounds by acid catalysis approach have also been reported (Bomon et al., 2021).
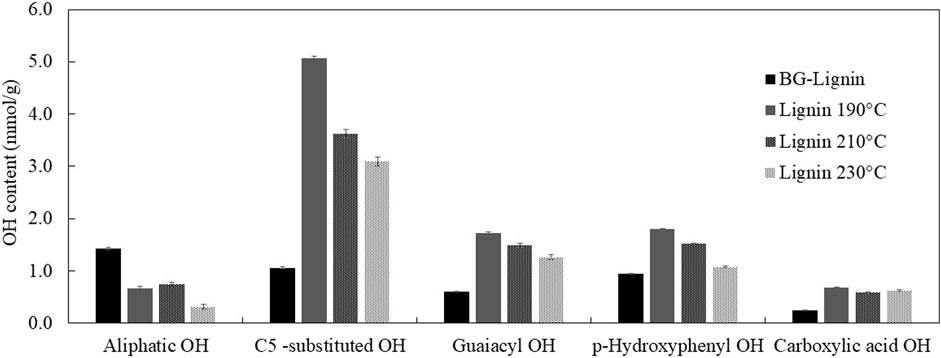
FIGURE 3. Aliphatic and phenolic hydroxyl content in organosolv lignin extracted from bagasse before and after thermal modification.
3.3 UV absorption and antioxidant performance of the extracted and modified organosolv lignin
Performance of the lignin samples prior and after the thermal modification on UV absorption was analyzed by redissolving the lignin sample powder in 70%v/v ethanol to the final concentration of 1% and 5%w/v for UV transmittance spectroscopy in the wavelength range of 240–390 nm (Figure 4). All lignin samples have relatively higher absorption for UVB (290–320 nm) than UVA (320–400 nm). The isolated BG-lignin has the lowest UV absorption efficiency under this condition as reflected by the highest UV transmittance. Increasing lignin concentration from 1%w/v to 5%w/v resulted in higher UV absorption. At both lignin concentrations, the modified lignin tended to show higher UV absorption efficiency compared to the BG lignin. The modified lignin obtained at 190°C showed the highest performance on UV absorption compared to those obtained from treatment at higher temperatures. Calculated from the spectral transmittance data, the lignin modified at 190°C provides highest SPF value of 44.55 which is 2.5 time higher than that of the unmodified one at the same concentration (5% w/v).
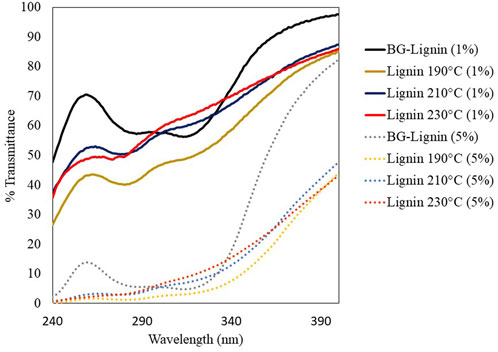
FIGURE 4. UV transmittance of solvent (70%v/v ethanol) containing 1%w/v and 5%w/v organosolv lignin extracted from bagasse prior and after thermal modification.
Antioxidation property of the lignin samples were tested using DPPH scavenging assay. Modification of lignin by treatment at 190°C led to a marked increase of the radical scavenging activity which represented in the lower IC50 of 10.96 μg/mL compared to that of the unmodified one (13.10 μg/mL). Decreasing radical scavenging activity was observed when the lignin was modified at 210 and 230°C. However, the antioxidant efficiency of all lignin sample provided in this study was higher compared to BHT (commercial antioxidant) used as the benchmark agent. The UV absorption and antioxidant activity of lignin sample modified at different temperatures were also corresponded with the altered phenolic OH content trend (Table 2). This emphasizes the potential of organosolv lignin from bench scale production for using as a functional additive for antioxidant and SPF enhancer properties. The increase in functional properties of the modified organosolv lignin is related to alteration in its structure. Several studies reported correlation between phenolic OH content and antioxidant performance of lignin due to radical scavenging ability of phenolic as hydrogen donor groups (Ugartondo et al., 2008; Vanderghem et al., 2011). Furthermore, pretreatment of lignin to increase its phenolic hydroxyl content for antioxidant property enhancement via homogenous acid approach (e.g., diluted H2SO4, HCl, and H3PO4) has been reported (do Nascimento Santos et al., 2020; Lourençon et al., 2021). The phenolic-OH content of lignin was increased from 69.7 to 303.6 mg/g when diluted H2SO4 pretreatment was applied which led to antioxidant capacity enhancement (IC50 decreased from 173.4 to 38.5 μg/mL) (You et al., 2022).
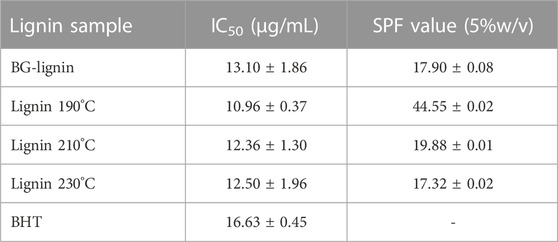
TABLE 2. Antioxidation property and SPF organosolv lignin extracted from bagasse before and after thermal modification.
3.4 Formulation and performance of lignin blended sunscreen cream
The formulation of lignin blended sunscreen cream was done by mixing the suspensions of lignin precipitated from BG extracted or modified black liquor with base cream. After lignin particles were blended well with the cream, the excess suspension supernatant was separated from the final product by low-speed centrifugation. The lignin particle size distribution had influences on the quality and homogeneity of the acquired lignin blended sunscreens which also affected their antioxidant and SPF properties. Typically, organosolv lignin can be precipitated from the extracted solvent by antisolvent approach as lignin solubility is greatly related to the solvent concentration. Organosolv lignin was found precipitated from ethanosolv liquor within pH 2-7 when ethanol concentration was diluted to less than 40wt% and the optimum precipitation condition was found when the ethanol concentration was within 17.9–19.9%wt. Although these optimum precipitation conditions gave the fastest and highest lignin precipitation yield, the particle size of lignin precipitate tended to be larger as the ethanol concentration was more diluted (Beisl et al., 2020). Therefore, this study applied sonication to assist the lignin precipitation by antisolvent approach at less dilute ethanol concentration to ensure an excellent homogeneity of lignin blended sunscreen result.
The effects of sonication on %yield of lignin were studied at varying times and ethanol concentrations (Figure 5). The black liquor from thermal modification process at 190°C was selected for the formulation of lignin blended sunscreen as the sample provides highest UV absorption and antioxidant activity. Prior to sonication, lignin precipitation yields by antisolvent at highest dilution (17.5%v/v ethanol) gave the highest lignin yield of 76.6%w/w. This result was comparable to our previous study on fractionation of bagasse by hydrothermal/ethanosolv process using a flow-through reactor (Pongchaiphol et al., 2021). In contrast, the lignin precipitation yields by antisolvent at lowest dilution (35.0%v/v ethanol) gave only 64.7%w/w lignin yield. However, the highest lignin yield of 86.6–89.9%w/w was obtained after the sonication was applied for 20 min with no significant difference (p > 0.05) in yield for the samples obtained at different ethanol concentrations. This led to 21.9%w/w improvement in the lignin yield compared with that obtained with no sonication at 35.0%v/v ethanol concentration. The morphologies of the lignin isolated from different ethanol concentrations are shown in Figure 6. Lignin precipitated at 17.5% ethanol concentration showed agglomerated morphology. In contrast, isolation of lignin from the suspension of 35.0%v/v ethanol showed homogenous spherical particle with the size range between 0.5 and 2.0 µm. The results thus indicate the effects of ethanol concentration on the morphological properties of lignin obtained from the precipitation step.
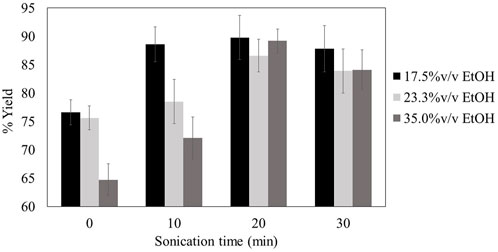
FIGURE 5. Organosolv lignin precipition yield at various solvent dilutions after assisted by sonication for different times.
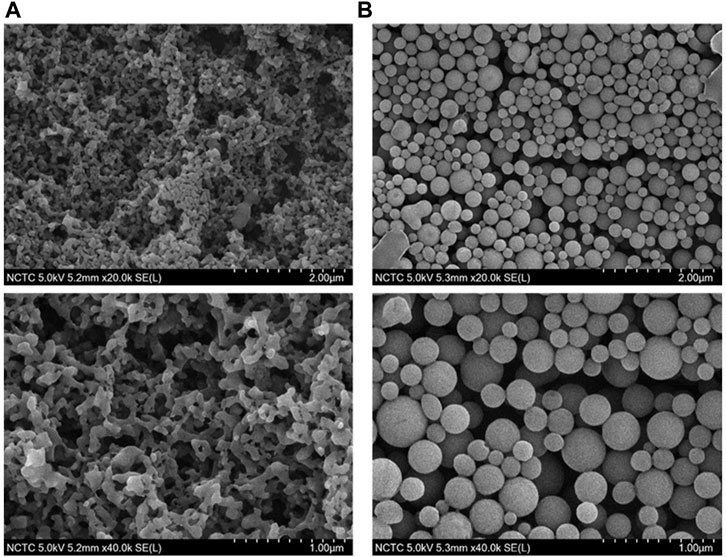
FIGURE 6. Morphology of organosolv lignin precipitated by solvent dilution at 17.5%v/v ethanol (A) and 35.0%v/v ethanol (B).
The obtained lignin precipitated at 17.5–35.0%v/v ethanol was used for sunscreen formulation with the base cream and tested for UV absorption efficiency (Figure 7). The base cream can only slightly absorb UV compared to the samples containing lignin. The sunscreen formulated using lignin precipitated at less diluted ethanol concentrations provided better UV absorption due to the superior homogeneity. This is represented by the increasing SPF trend of 7.4, 8.4, and 32.0 in the base cream blend with 1%w/v lignin precipitated at 17.5, 23.3, and 35.0%v/v ethanol suspensions, respectively. A well-dispersed micro/nanoparticles was reported to express 30% enhanced UV protection performance when compared with that of native state lignin (Li et al., 2016). Several studies on sunscreen formulation using micro/nanoparticle lignin as SPF enhancer showed outstanding performance due to the exceptionally high surface area and chromophores on the lignin micromorphology (Wang et al., 2018; Zhang et al., 2019b; Wu et al., 2019; Lee et al., 2020). Increasing lignin loading in the base cream led to increasing efficiency on UV absorption. At 3%w/v of the modified organosolv lignin (optimized in phenolic OH content and particle size) blended with the base cream, SPF value of the formulated sunscreen (55.1) exceed the SPF value of the commercial SPF30 cream (39.3). Furthermore, the lignin additives in this study were modified to concentrate their UV-absorbing functional groups (phenolic groups) which absorb a broader spectral range of UV from 250 to 400 nm (Sugiarto et al., 2022) whereas compound used in the commercial SPF-30 sunscreen (TiO2) provides 290–400 nm spectral range of UVA II and UVB protection due to its UV absorption and scattering properties (Serpone et al., 2007). The use of lignin additive could benefit the future property of cosmeceutical products for protection of UVC radiation (200–290 nm) which must be taken into account according to the depleting global ozone layer (Wang et al., 2010). Addition of the modified organosolv lignin at higher concentration further enhanced the SPF value of the formulated sunscreen (Table 3). Compared to other studies on sunscreen formulation using lignin as SPF enhancer, the modified lignin in this study offers comparable SPF improvement with lower concentration added. Typically, about 5–10%w/v of lignin was added to SPF 15 sunscreen or base cream and resulted in 8–70 boosted SPF value (Qian et al., 2015; Kai et al., 2016; Qian et al., 2016; Qian et al., 2017; Wang et al., 2018; Zhang et al., 2019a; Zhang et al., 2019b; Lee et al., 2019; Wu et al., 2019; de Araújo Padilha et al., 2020; Lee et al., 2020; Widsten et al., 2020) (Table 4). Similar trends were found for the antioxidant activity enhancement when the modified lignin was added to the base cream. Addition of lignin precipitated from all ethanol concentrations at 1% loading in our study led to marked increase in radical scavenging activity compared to the base cream and the standard SPF30 cream. At 1% lignin concentration, lignin precipitated at less diluted ethanol concentrations resulted in increasing %radical scavenging activity (at 100 µg/mL) from 75.61% to 82.86%. Further increase in lignin concentration from 2% to 5% led to a slight increasing trend on radical scavenging activity (at 100 µg/mL) from 86.65% to 89.83%. The antioxidant activity of the modified lignin can add on beneficial property to the cosmetic products (Arulselvan et al., 2016). The results thus indicate the advantages of using the modified lignin as the functional ingredient with dual activities in cream formulation.
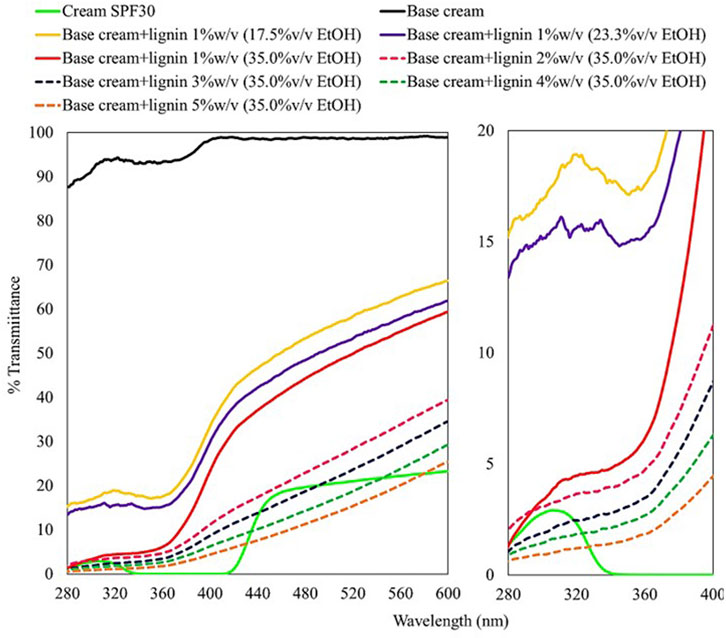
FIGURE 7. UV transmittance of cream SPF30 (commerical) and cream containing 1–5%w/v of thermal modified organosolv lignin (190°C) precipitated at various solvent dilutions.
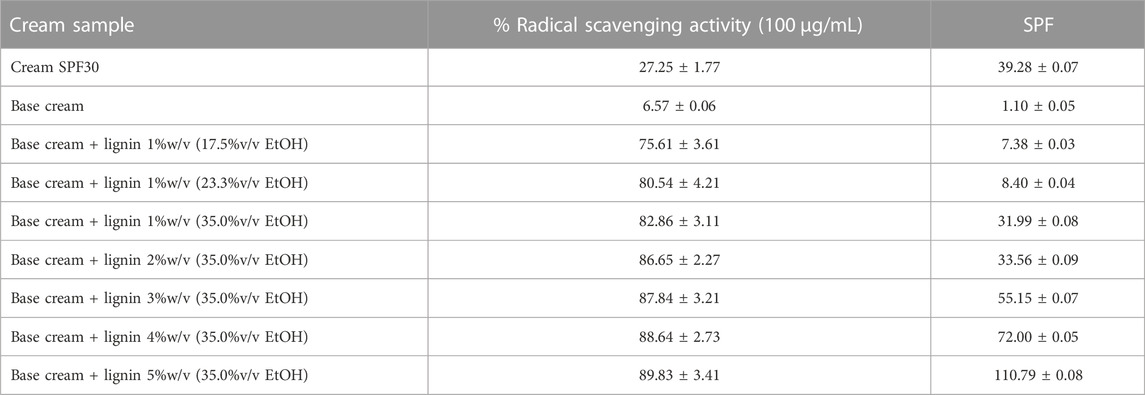
TABLE 3. Antioxidation property and SPF of cream SPF30 (commercial) and cream containing 1–5%w/v of thermal modified organosolv lignin (190°C) which precipitated at various solvent dilutions.
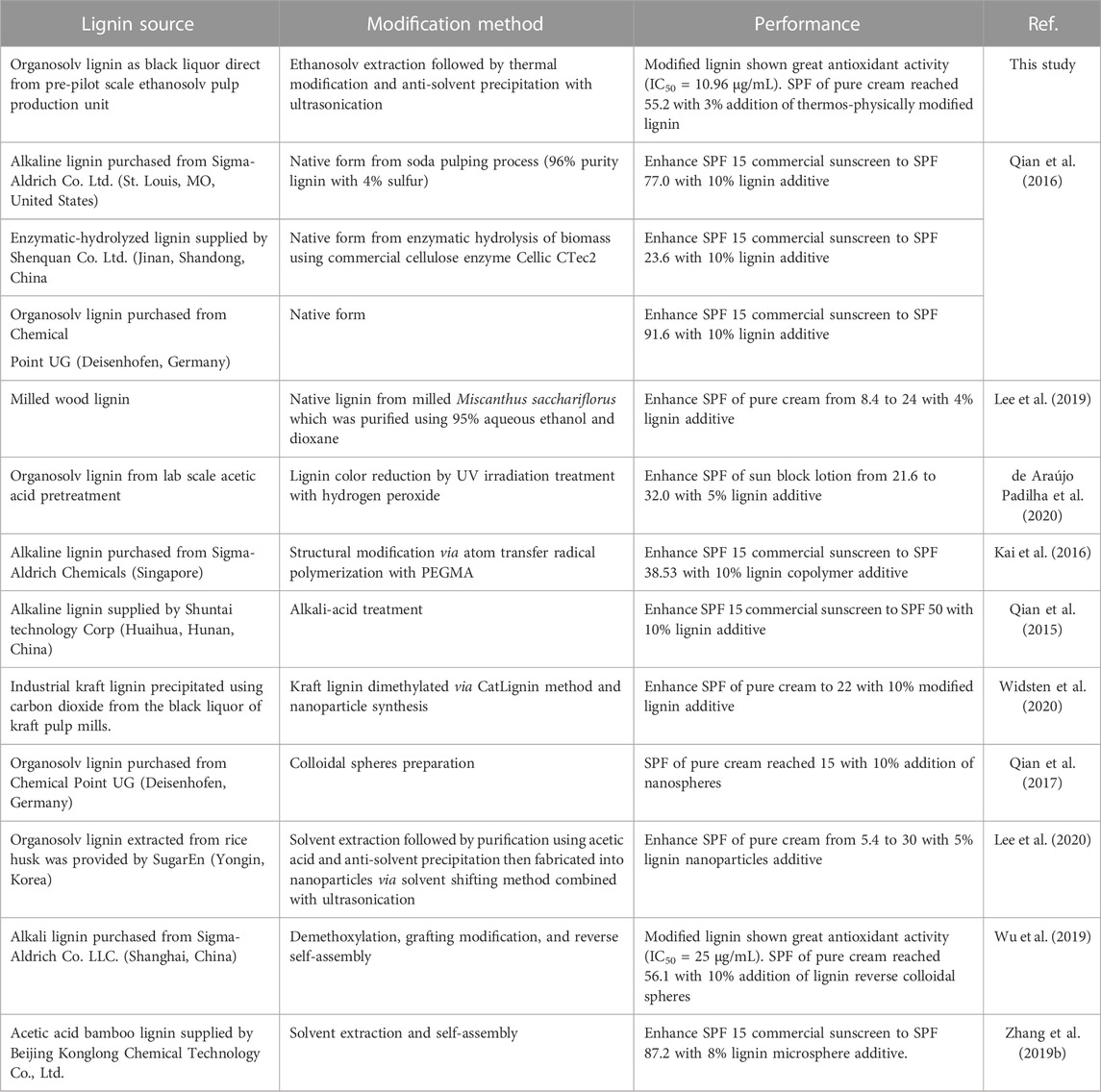
TABLE 4. Summary of current study and other recent research on production of lignin-based cosmeceutical products.
4 Conclusion
An efficient thermal and physical modification on organosolv lignin obtained from the bench-scale bagasse pulp production for improving the sunscreen and antioxidant properties of lignin using as functional bio-additive in cosmeceutical products was reported. The increased phenolic hydroxyl content affected by thermal treatment of black liquor boosted the UV absorption and free radical scavenging ability of the modified lignin. The ultra-sonication assisted solvent exchange method resulted in a well-distributed microsphere lignin colloidal which further enhanced the Sun protection and antioxidant performance of the formulated product. The work provides value addition strategy for lignin as co-product from green pulping production process in cosmeceutical application.
Data availability statement
The original contributions presented in the study are included in the article/supplementary material, further inquiries can be directed to the corresponding author.
Author contributions
All authors listed have made a substantial, direct, and intellectual contribution to the work and approved it for publication.
Funding
This project was financially supported by the Program Management Unit for Competitiveness (grant number C10F640106). CC has received funding support from the NSRF via the Program Management Unit for Human Resources & Institutional Development, Research and Innovation (PMU-B) [grant number B01F640033].
Conflict of interest
Authors CC, KJ, MR, SP, NL, and VC were employed by the company National Center for Genetic Engineering and Biotechnology.
The remaining author declares that the research was conducted in the absence of any commercial or financial relationships that could be construed as a potential conflict of interest.
Publisher’s note
All claims expressed in this article are solely those of the authors and do not necessarily represent those of their affiliated organizations, or those of the publisher, the editors and the reviewers. Any product that may be evaluated in this article, or claim that may be made by its manufacturer, is not guaranteed or endorsed by the publisher.
References
Arulselvan, P., Fard, M. T., Tan, W. S., Gothai, S., Fakurazi, S., Norhaizan, M. E., et al. (2016). Role of antioxidants and natural products in inflammation. Oxidative Med. Cell. Longev. 2016, 5276130. doi:10.1155/2016/5276130
Beisl, S., Adamcyk, J., and Friedl, A. (2020). Direct precipitation of lignin nanoparticles from wheat straw organosolv liquors using a static mixer. Molecules 25 (6), 1388. doi:10.3390/molecules25061388
Bomon, J., Bal, M., Achar, T. K., Sergeyev, S., Wu, X., Wambacq, B., et al. (2021). Efficient demethylation of aromatic methyl ethers with HCl in water. Green Chem. 23 (5), 1995–2009. doi:10.1039/d0gc04268d
Cao, L., Iris, K., Liu, Y., Ruan, X., Tsang, D. C., Hunt, A. J., et al. (2018). Lignin valorization for the production of renewable chemicals: State-of-the-art review and future prospects. Bioresour. Technol. 269, 465–475. doi:10.1016/j.biortech.2018.08.065
Chotirotsukon, C., Raita, M., Champreda, V., and Laosiripojana, N. (2019). Fractionation of sugarcane trash by oxalic-acid catalyzed glycerol-based organosolv followed by mild solvent delignification. Industrial Crops Prod. 141, 111753. doi:10.1016/j.indcrop.2019.111753
de Araújo Padilha, C. E., da Costa Nogueira, C., Oliveira Filho, M. A., de Santana Souza, D. F., de Oliveira, J. A., and dos Santos, E. S. (2020). Valorization of cashew apple bagasse using acetic acid pretreatment: Production of cellulosic ethanol and lignin for their use as sunscreen ingredients. Process Biochem. 91, 23–33. doi:10.1016/j.procbio.2019.11.029
Dizhbite, T., Telysheva, G., Jurkjane, V., and Viesturs, U. (2004). Characterization of the radical scavenging activity of lignins––natural antioxidants. Bioresour. Technol. 95 (3), 309–317. doi:10.1016/j.biortech.2004.02.024
do Nascimento Santos, D. K. D., da Silva Barros, B. R., de Souza Aguiar, L. M., da Cruz Filho, I. J., de Lorena, V. M. B., de Melo, C. M. L., et al. (2020). Immunostimulatory and antioxidant activities of a lignin isolated from Conocarpus erectus leaves. Int. J. Biol. Macromol. 150, 169–177. doi:10.1016/j.ijbiomac.2020.02.052
Dong, X., Dong, M., Lu, Y., Turley, A., Jin, T., and Wu, C. (2011). Antimicrobial and antioxidant activities of lignin from residue of corn stover to ethanol production. Industrial Crops Prod. 34 (3), 1629–1634. doi:10.1016/j.indcrop.2011.06.002
Gil-Chávez, G. J., Padhi, S. S. P., Pereira, C. V., Guerreiro, J. N., Matias, A. A., and Smirnova, I. (2019). Cytotoxicity and biological capacity of sulfur-free lignins obtained in novel biorefining process. Int. J. Biol. Macromol. 136, 697–703. doi:10.1016/j.ijbiomac.2019.06.021
Imman, S., Khongchamnan, P., Wanmolee, W., Laosiripojana, N., Kreetachat, T., Sakulthaew, C., et al. (2021). Fractionation and characterization of lignin from sugarcane bagasse using a sulfuric acid catalyzed solvothermal process. RSC Adv. 11 (43), 26773–26784. doi:10.1039/d1ra03237b
Kai, D., Chua, Y. K., Jiang, L., Owh, C., Chan, S. Y., and Loh, X. J. (2016). Dual functional anti-oxidant and SPF enhancing lignin-based copolymers as additives for personal and healthcare products. Rsc Adv. 6 (89), 86420–86427. doi:10.1039/c6ra21433a
Kaur, R., Uppal, S., and Sharma, P. (2017). Antioxidant and antibacterial activities of sugarcane bagasse lignin and chemically modified lignins. Sugar Tech. 19 (6), 675–680. doi:10.1007/s12355-017-0513-y
Kleinert, M., and Barth, T. (2008). Phenols from lignin. Ind. Chemistry-Plant Equipment-Process Engineering-Biotechnology 31 (5), 736–745. doi:10.1002/ceat.200800073
Lee, S. C., Tran, T. M. T., Choi, J. W., and Won, K. (2019). Lignin for white natural sunscreens. Int. J. Biol. Macromol. 122, 549–554. doi:10.1016/j.ijbiomac.2018.10.184
Lee, S. C., Yoo, E., Lee, S. H., and Won, K. (2020). Preparation and application of light-colored lignin nanoparticles for broad-spectrum sunscreens. Polymers 12 (3), 699. doi:10.3390/polym12030699
Leschinsky, M., Zuckerstätter, G., Weber, H. K., Patt, R., and Sixta, H. (2008). Effect of autohydrolysis of Eucalyptus globulus wood on lignin structure. Holzforschung 62 (6), 645–658.
Li, H., Deng, Y., Wu, H., Ren, Y., Qiu, X., Zheng, D., et al. (2016). Self-assembly of kraft lignin into nanospheres in dioxane-water mixtures. Holzforschung 70 (8), 725–731. doi:10.1515/hf-2015-0238
Li, N., Li, Y., Yoo, C. G., Yang, X., Lin, X., Ralph, J., et al. (2018). An uncondensed lignin depolymerized in the solid state and isolated from lignocellulosic biomass: A mechanistic study. Green Chem. 20 (18), 4224–4235. doi:10.1039/c8gc00953h
Lin, M., Yang, L., Zhang, H., Xia, Y., He, Y., Lan, W., et al. (2021). Revealing the structure-activity relationship between lignin and anti-UV radiation. Industrial Crops Prod. 174, 114212. doi:10.1016/j.indcrop.2021.114212
Lourençon, T. V., de Lima, G. G., Ribeiro, C. S., Hansel, F. A., Maciel, G. M., da Silva, K., et al. (2021). Antioxidant, antibacterial and antitumoural activities of kraft lignin from hardwood fractionated by acid precipitation. Int. J. Biol. Macromol. 166, 1535–1542. doi:10.1016/j.ijbiomac.2020.11.033
Lu, X., Gu, X., and Shi, Y. (2022). A review on lignin antioxidants: Their sources, isolations, antioxidant activities and various applications. Int. J. Biol. Macromol. 210, 716–741. doi:10.1016/j.ijbiomac.2022.04.228
McKinlay, A. (1987). A reference action spectrum for ultraviolet induced erythema in human skin. Cie. J. 6, 17–22.
Meng, X., Crestini, C., Ben, H., Hao, N., Pu, Y., Ragauskas, A. J., et al. (2019). Determination of hydroxyl groups in biorefinery resources via quantitative 31P NMR spectroscopy. Nat. Protoc. 14 (9), 2627–2647. doi:10.1038/s41596-019-0191-1
Pongchaiphol, S., Chotirotsukon, C., Raita, M., Champreda, V., and Laosiripojana, N. (2021). Two-stage fractionation of sugarcane bagasse by a flow-through hydrothermal/ethanosolv process. Industrial Eng. Chem. Res. 60 (34), 12629–12639. doi:10.1021/acs.iecr.1c00669
Qian, Y., Qiu, X., and Zhu, S. (2015). Lignin: A nature-inspired sun blocker for broad-spectrum sunscreens. Green Chem. 17 (1), 320–324. doi:10.1039/c4gc01333f
Qian, Y., Qiu, X., and Zhu, S. (2016). Sunscreen performance of lignin from different technical resources and their general synergistic effect with synthetic sunscreens. ACS Sustain. Chem. Eng. 4 (7), 4029–4035. doi:10.1021/acssuschemeng.6b00934
Qian, Y., Zhong, X., Li, Y., and Qiu, X. (2017). Fabrication of uniform lignin colloidal spheres for developing natural broad-spectrum sunscreens with high sun protection factor. Industrial Crops Prod. 101, 54–60. doi:10.1016/j.indcrop.2017.03.001
Schmalwieser, A. W., Wallisch, S., and Diffey, B. (2002). A library of action spectra for erythema and pigmentation. Photochem. Photobiological Sci. 1 (12), 251–268. doi:10.1039/c1pp05271c
Serpone, N., Dondi, D., and Albini, A. (2007). Inorganic and organic UV filters: Their role and efficacy in sunscreens and suncare products. Inorganica Chim. acta 360 (3), 794–802. doi:10.1016/j.ica.2005.12.057
Sluiter, A., Hames, B., Ruiz, R., Scarlata, C., Sluiter, J., Templeton, D., et al. (2008). Determination of structural carbohydrates and lignin in biomass. Lab. Anal. Proced. 1617, 1–16.
Sluiter, J. B., Ruiz, R. O., Scarlata, C. J., Sluiter, A. D., and Templeton, D. W. (2010). Compositional analysis of lignocellulosic feedstocks. 1. Review and description of methods. J. Agric. food Chem. 58 (16), 9043–9053. doi:10.1021/jf1008023
Sugiarto, S., Leow, Y., Tan, C. L., Wang, G., and Kai, D. (2022). How far is Lignin from being a biomedical material? Bioact. Mater. 8, 71–94. doi:10.1016/j.bioactmat.2021.06.023
Tortora, M., Cavalieri, F., Mosesso, P., Ciaffardini, F., Melone, F., and Crestini, C. (2014). Ultrasound driven assembly of lignin into microcapsules for storage and delivery of hydrophobic molecules. Biomacromolecules 15 (5), 1634–1643. doi:10.1021/bm500015j
Tran, M. H., Phan, D-P., and Lee, E. Y. (2021). Review on lignin modifications toward natural UV protection ingredient for lignin-based sunscreens. Green Chem. 23 (13), 4633–4646. doi:10.1039/d1gc01139a
Ugartondo, V., Mitjans, M., and Vinardell, M. P. (2008). Comparative antioxidant and cytotoxic effects of lignins from different sources. Bioresour. Technol. 99 (14), 6683–6687. doi:10.1016/j.biortech.2007.11.038
Vanderghem, C., Richel, A., Jacquet, N., Blecker, C., and Paquot, M. (2011). Impact of formic/acetic acid and ammonia pre-treatments on chemical structure and physico-chemical properties of Miscanthus x giganteus lignins. Polym. Degrad. Stab. 96 (10), 1761–1770. doi:10.1016/j.polymdegradstab.2011.07.022
Vu, H. P., Nguyen, L. N., Vu, M. T., Johir, M. A. H., McLaughlan, R., and Nghiem, L. D. (2020). A comprehensive review on the framework to valorise lignocellulosic biomass as biorefinery feedstocks. Sci. Total Environ. 743, 140630. doi:10.1016/j.scitotenv.2020.140630
Wang, B., Sun, D., Wang, H., Yuan, T., and Sun, R. (2018). Green and facile preparation of regular lignin nanoparticles with high yield and their natural broad-spectrum sunscreens. ACS Sustain. Chem. Eng. 7 (2), 2658–2666. doi:10.1021/acssuschemeng.8b05735
Wang, S. Q., Balagula, Y., and Osterwalder, U. (2010). Photoprotection: A review of the current and future technologies. Dermatol. Ther. 23 (1), 31–47. doi:10.1111/j.1529-8019.2009.01289.x
Widsten, P., Tamminen, T., and Liitiä, T. (2020). Natural sunscreens based on nanoparticles of modified kraft lignin (CatLignin). ACS omega 5 (22), 13438–13446. doi:10.1021/acsomega.0c01742
Wu, Y., Qian, Y., Lou, H., Yang, D., and Qiu, X. (2019). Enhancing the broad-spectrum adsorption of lignin through methoxyl activation, grafting modification, and reverse self-assembly. ACS Sustain. Chem. Eng. 7 (19), 15966–15973. doi:10.1021/acssuschemeng.9b02317
You, S., Xie, Y., Zhuang, X., Chen, H., Qin, Y., Cao, J., et al. (2022). Effect of high antioxidant activity on bacteriostasis of lignin from sugarcane bagasse. Biochem. Eng. J. 180, 108335. doi:10.1016/j.bej.2022.108335
Zhang, H., Chen, F., Liu, X., and Fu, S. (2018). Micromorphology influence on the color performance of lignin and its application in guiding the preparation of light-colored lignin sunscreen. ACS Sustain. Chem. Eng. 6 (9), 12532–12540. doi:10.1021/acssuschemeng.8b03464
Zhang, H., Liu, X., Fu, S., and Chen, Y. (2019). Fabrication of light-colored lignin microspheres for developing natural sunscreens with favorable UV absorbability and staining resistance. Industrial Eng. Chem. Res. 58 (31), 13858–13867. doi:10.1021/acs.iecr.9b02086
Zhang, H., Liu, X., Fu, S., and Chen, Y. (2019). High-value utilization of kraft lignin: Color reduction and evaluation as sunscreen ingredient. Int. J. Biol. Macromol. 133, 86–92. doi:10.1016/j.ijbiomac.2019.04.092
Keywords: organosol lignin, anti UV, Antio×idant, thermal modifcation, functional additive
Citation: Chotirotsukon C, Jirachavala K, Raita M, Pongchaiphol S, Hararak B, Laosiripojana N and Champreda V (2023) Effects of thermal and physical modification on functional properties of organosolv lignin from sugarcane bagasse and its application in cosmeceutical products. Front. Chem. Eng. 5:1099010. doi: 10.3389/fceng.2023.1099010
Received: 15 November 2022; Accepted: 09 February 2023;
Published: 22 February 2023.
Edited by:
Chang Geun Yoo, SUNY College of Environmental Science and Forestry, United StatesReviewed by:
Kenneth L. Sale, Sandia National Laboratories, United StatesDaehwan Kim, Hood College, United States
Copyright © 2023 Chotirotsukon, Jirachavala, Raita, Pongchaiphol, Hararak, Laosiripojana and Champreda. This is an open-access article distributed under the terms of the Creative Commons Attribution License (CC BY). The use, distribution or reproduction in other forums is permitted, provided the original author(s) and the copyright owner(s) are credited and that the original publication in this journal is cited, in accordance with accepted academic practice. No use, distribution or reproduction is permitted which does not comply with these terms.
*Correspondence: Verawat Champreda, dmVyYXdhdEBiaW90ZWMub3IudGg=
†These authors have contributed equally to this work
‡These authors share senior authorship