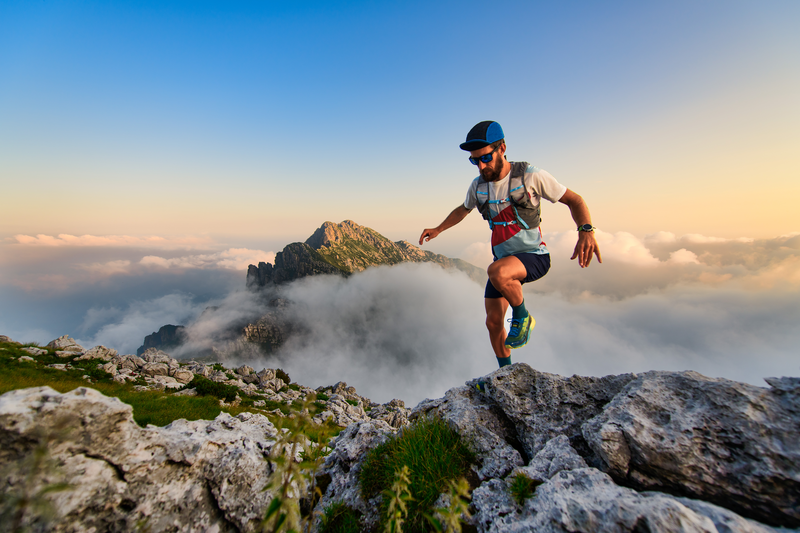
95% of researchers rate our articles as excellent or good
Learn more about the work of our research integrity team to safeguard the quality of each article we publish.
Find out more
REVIEW article
Front. Cell. Infect. Microbiol. , 14 January 2025
Sec. Extra-intestinal Microbiome
Volume 14 - 2024 | https://doi.org/10.3389/fcimb.2024.1518811
This article is part of the Research Topic Skin Microbiome: Microbiological, Immunological and Cellular aspects for therapies to control Antimicrobial Resistance and Skin Repair View all articles
Atopic dermatitis (AD) is a chronic and inflammatory skin disorder characterized by impaired barrier function and imbalanced immunity. Recent advances have revealed that dysbiosis of skin microbiota plays important roles in the pathogenesis and development of AD. Meanwhile, endogenous and external factors contribute to the dysbiosis of skin microbiota in AD. Additionally, various treatments, including topical treatments, phototherapy, and systemic biologics, have demonstrated positive impacts on the clinical outcomes, alongside with the modulations of cutaneous microbiota in AD patients. Importantly, therapeutics or products regulating skin microbiota homeostasis have demonstrated potential for AD treatment in early clinical studies. In this review, we underline changes of the skin microbiota correlated with AD. Meanwhile, we provide an overview of the skin microbiota regarding its roles in the pathogenesis and development of AD. Finally, we summarize therapeutic strategies restoring the skin microbial homeostasis in AD management.
Atopic dermatitis (AD) is a long-lasting and inflammatory skin disease. It usually appears during infancy and childhood; however, people of all ages can get this skin condition (Wollenberg et al., 2016; Weidinger et al., 2018). Symptoms of AD range from excessively dry, extremely itchy skin to painful skin, which can flare in multiple areas of the body sites, severely affecting the life quality of patients worldwide (Sidbury et al., 2014; Silverberg et al., 2015; Vakharia et al., 2017; Silverberg et al., 2019). Although it is clearly that genetics, immune system, and environmental factors play roles in AD pathogenesis, the precise mechanism that causes atopic dermatitis remains elusive (Weidinger and Novak, 2016; Weidinger et al., 2018; Langan et al., 2020). Recently, increasing evidence revealed that dysbiosis of skin microbiota contributes to AD development (Williams and Gallo, 2015; Bjerre et al., 2017; Tao et al., 2022).
The skin microbiota are mainly composed of numerous bacterial species, as well as fungi and virus (Flowers and Grice, 2020; Whiting et al., 2024). Homeostasis of the skin microbiota benefits the host by providing support to the skin barrier function and inhibiting pathogen colonization (Prescott et al., 2017; Parlet et al., 2019; Swaney and Kalan, 2021; Hammond et al., 2022). Meanwhile, the skin microbiota can modulate the host innate and adaptive immunity (Coates et al., 2019; Paller et al., 2019; Boxberger et al., 2021), both of which are core components of the skin immune system (Richmond and Harris, 2014; Coates et al., 2018; Ong, 2022). Thus, the skin microbiota homeostasis is apparently important in maintaining healthy skin, while its dysbiosis contributes to skin pathology.
Given that skin microbiota dysbiosis is increasingly implicated as a contributor to the pathogenesis of AD, the effects and molecular mechanisms of cutaneous microbiota on the development of AD cannot be overlooked (Figure 1).
Figure 1. The regulatory roles of cutaneous microbiota on the pathogenesis and development of AD. Dysbiosis of the skin microbiota contributes to the pathogenesis and development of AD. Firstly, the S. aureus colonization promotes the disease progression through inducing the skin inflammation, triggering the skin barrier damage, and mediating the Th2 immune response. Secondly, the commensals, including the S. epidermidis and the CoNS species, contribute to skin homeostasis via inhibiting the S. aureus overgrowth and maintaining the skin’s barrier function. Thirdly, the commensal fungi, especially the over-presentation of Malassezia, selectively induce Th17 activation and subsequent skin inflammation.
S. aureus on the skin is positively correlated with disease severity and represents one of the main triggers for the worsening of skin lesions in AD patients (Tauber et al., 2016; Totté et al., 2016). In the mouse model of AD, epicutaneous exposure of skin to S. aureus induces inflammation (Liu et al., 2017). Mechanistically, phenol-soluble modulin α (PSMα) secreted by S. aureus contributes to skin inflammation (Liu et al., 2017). Additionally, S. aureus-expressed PSMα induces release of pro-inflammatory cytokines/chemokines from keratinocytes, as well as subsequent IL-17 production from immune cells, leading to cutaneous inflammation in a murine infection model (Syed et al., 2015; Nakagawa et al., 2017). Consistently, Williams et al. reported that the proteases and PSMα secreted by S. aureus lead to epidermal proteolysis and skin barrier damage (Williams et al., 2019), further confirming that S. aureus contributes to AD pathogenesis.
Upon incubation with keratinocytes, heat killed S. aureus from AD patients are strongly agglutinated inside the cytoplasm, where they are located in lysosomes and promote the secretion of IL-1α (Moriwaki et al., 2019). In addition, the inoculation with S. aureus increased IL-1β and IL-18 production, whereas silencing of NLRP1 decreased the secretion of these cytokines in keratinocytes, suggesting that S. aureus may contribute to the pathogenesis of AD through NLRP1 inflammasome/IL-1β and IL-18 axis (Vaher et al., 2023). Moreover, S. aureus enhances the release of thymic stromal lymphopoietin (TSLP) in human keratinocytes, and mediates the Th2-type inflammation (Vu et al., 2010), providing further clue for the association between S. aureus colonization and AD disease progression.
Using shotgun metagenomic sequence analysis, Byrd et al. revealed that AD flares exhibited greater S. aureus predominance in patients with severe disease (Byrd et al., 2017). Specifically, S. aureus from AD patients with more severe flares induced epidermal thickening and expansion of cutaneous Th2 and Th17 cells in the murine models (Byrd et al., 2017), indicating its contributory role to AD development.
S. aureus is unique among staphylococcal species, as it’s able to induce rapid release of IL-33 from keratinocytes, identifying it as a dominant type 2 immunity trigger underlying AD pathogenesis (Al Kindi et al., 2021). Interestingly, Th2 cytokine exposure increases the sensitivity to S. aureus alpha toxin-induced cell lethality in keratinocytes, which is more commonly observed in skins from AD patients compared with healthy individuals (Brauweiler et al., 2014). Furthermore, Th2 cytokines decrease levels of sphingomyelinase (SMase) and production of lamellar bodies, which are critical for cleaving alpha toxin receptor and keeping epidermal barrier formation, respectively. Finally, SMase prevents Th2-mediated cell death (Brauweiler et al., 2014), uncovering a mechanism that Th2 cytokine exacerbating S. aureus-induced AD pathogenesis.
In fact, in addition to S. aureus alpha toxin, several other staphylococcal exotoxins also play crucial roles in the pathogenesis of AD. Nakamura et al. found that δ-toxin produced by S. aureus potently facilitated the degranulation of mast cells (Nakamura et al., 2013). Further studies demonstrated that the skin colonization by S. aureus enhanced the production of immunoglobulin-E (IgE) and IL-4, along with skin inflammation, in a δ-toxin-dependent manner. Interestingly, IgE could amplify the δ-toxin-induced mast cell degranulation in the absence of antigens. Moreover, mast cell deficiency and subsequent reconstitution could either abrogate or restore the production of IgE induced by δ-toxin, respectively, in a dermatitis mouse model. Clinically, S. aureus isolated from AD patients produced large amounts of δ-toxin (Nakamura et al., 2013). Therefore, the S. aureus/δ-toxin/IgE/mast cell axis represents a novel mechanism in the pathogenesis of AD.
Strikingly, the skin inflammation leads to a rapid recruitment of neutrophils, which correlates with enhanced S. aureus colonization. On the contrary, depletion of neutrophils reduces the skin colonization of S. aureus (Bitschar et al., 2020). Meanwhile, the interaction of neutrophil extracellular traps (NETs, released by infiltrating neutrophils) with keratinocytes are responsible for increased S. aureus colonization (Bitschar et al., 2020), suggesting that inflammatory environment contributes to enhanced S. aureus colonization in AD pathogenesis.
Moreover, monocyte-derived Langerhans cells stimulated by AD associated-S. aureus induce rapid proliferation of T cells (Iwamoto et al., 2017), demonstrating that S. aureus can skew T cell responses in AD pathogenesis. Additionally, exposure of S. aureus secretomes to monocyte-derived dendritic cells (moDC) promotes the release of pro-inflammatory IFN-γ (Laborel-Préneron et al., 2015). Meanwhile, allogeneic moDC exposed to S. aureus secretome induces CD4+ T cell proliferation. Furthermore, the S. aureus secretome inhibits Treg activity (Laborel-Préneron 9et al., 2015). Therefore, colonization of S. aureus on AD skins regulates cutaneous inflammation through multiple different pathways.
S. aureus is known to exacerbate AD, whereas Staphylococcus epidermidis (S. epidermidis) has been considered as a beneficial commensal organism. Interestingly, S. epidermidis activates and upregulates Perforin-2 (P-2), while the upregulation of P-2 correlates with increased killing of intracellular S. aureus in skin cells, thereby protecting the host from skin infections (Strbo et al., 2019; Pastar et al., 2020). Moreover, S. epidermidis contributes to skin’s physical integrity by secreting SMase (Zheng et al., 2022). In mouse models, S. epidermidis increases the skin ceramide levels and prevents skin water loss in a SMase-dependent way (Zheng et al., 2022), demonstrating its crucial role in maintaining the skin’s barrier function. S. epidermidis also plays important roles in keeping the proper differentiation and repairment of the epidermal barrier, which are partially mediated by aryl hydrocarbon receptor (AHR) signaling in keratinocytes (Uberoi et al., 2021). Interestingly, S. epidermidis strains produce strong cysteine protease activity when grow at high density, which is able to degrade desmoglein-1 and LL-37 in vitro, and disrupt the physical barrier and induce skin inflammation in vivo (Cau et al., 2021). Clinically, the abundance of S. epidermidis is increased on the skin of some patients with AD, which is correlated with disease severity (Cau et al., 2021). Mechanistically, phenol-soluble modulin (PSM) peptides produced by S. epidermidis induce host defense and are cytotoxic to human keratinocytes. Moreover, the expression of PSMδ from S. epidermidis is positively correlated with disease severity in AD patients (Williams et al., 2023). Thus, the over-presence of S. epidermidis found on some AD patients can act similarly to S. aureus and contribute to AD pathogenesis.
As discussed in “2.1 Section“, S. aureus secretes PSMα and contributes to skin inflammation and barrier dysfunction. Interestingly, coagulase-negative staphylococci (CoNS) species residing on normal skin, inhibit S. aureus growth and subsequently lead to decreased PSMα expression (Williams et al., 2019), showing a concept that normal skin microbiota contributes to epithelial barrier homeostasis. Meanwhile, the CoNS strains with antimicrobial activity are common on the healthy skins but rare on the AD skins. Moreover, application of these CoNS strains to mice or reintroduction of them to AD patients confirms their defense against S. aureus (Nakatsuji et al., 2017). Further, spent media from the CoNS species can inhibit quorum sensing by S. aureus (Paharik et al., 2017). Specifically, the autoinducing peptide of CoNS species is responsible for the reduction of quorum sensing, and it dramatically reduces the cutaneous bacterial burden in a murine model of infection (Paharik et al., 2017). Thus, the skin commensals protect against skin dysbiosis, especially the S. aureus outgrowth, and impact disease outcomes in AD.
Commensal fungi of the skin, such as those of the genus Malassezia, are also associated with AD pathogenesis. For instance, the presence of Malassezia, selectively induce IL-17 and related cytokines, leading to aggravated cutaneous inflammation. Moreover, AD patients show enhanced frequency of Th17 subsets than healthy individuals, which is Malassezia involved and specific (Sparber et al., 2019). Thus, Malassezia-induced Th17 response is pivotal in promoting skin inflammation.
The most common sebaceous skin commensal yeasts are Malassezia, while the dominant secreted Malassezia globosa protease is Malassezia globosa Secreted Aspartyl Protease 1 (MGSAP1), which can rapidly hydrolyze Staphylococcus aureus protein A and holds anti-biofilm properties against S. aureus (Li et al., 2018), indicating that Malassezia and its enzyme maybe beneficial for skin health. In AD patients, upregulated MGSAP1 is observed in lesional skins, as compared to healthy skins (Goh et al., 2022). Functional loss of MFSAP1 leads to reductions in the cell adhesion and dispersal in both cultured and a human 3D epidermis models. Furthermore, MGSAP1 contributes to inflammation in a murine model of Malassezia colonization (Goh et al., 2022). Thus, MGSAP1 plays crucial roles in enabling fungi (Malassezia) colonization and promoting the skin barrier disruption.
The overabundant colonization by S. aureus may trigger the aggravation of AD skins. Meanwhile, the initiation and progression of AD may require the adherence of S. aureus to the skin, the mechanism of which remains largely unknown. Interestingly, fibronectin and fibrinogen enhance the binding of S. aureus to the skins of AD patients (Cho et al., 2001). Meanwhile, the S. aureus itself stimulates keratinocytes to increase their trypsin activity, as well as degradation of desmoglein-1 and filaggrin (Williams et al., 2017), illustrating that S. aureus influences the skin barrier integrity by stimulating endogenous proteolytic activity.
Adhesion of S. aureus to corneocytes in the stratum corneum is also a key initial event in colonization. It was recently reported that S. aureus interacted with and took advantage of the host protein corneodesmosin, to facilitate its binding with AD corneocytes (Towell et al., 2021). The abnormalities of epidermal barrier in AD can also alter the entry of S. aureus into the dermis, while the dermal dysbiosis results in increased inflammation and exacerbated disease severity (Nakatsuji et al., 2016), defining a novel mechanism by which S. aureus contributes to AD development. Keratinocytes lacking JunB exhibit higher MyD88 levels, which promotes the colonization of S. aureus (Uluçkan et al., 2019). Additionally, the spontaneous S. aureus colonization in JunB deficient mice shows a large transcriptomic overlap with AD (Uluçkan et al., 2019). Thus, JunB works as an upstream regulator of microbiota-immune interaction in AD pathogenesis.
The metabolites of skin microbiota can also act as a regulator of AD inflammation (Li and Yosipovitch, 2020). For instance, indole-3-aldehyde (IAId), a skin microbiota-derived tryptophan metabolite, is significantly lower in the lesional skins of AD patients than that of healthy individuals (Yu et al., 2019). Moreover, IAId attenuates the skin inflammation in MC903-induced mice through inhibiting TSLP expression in keratinocytes (Yu et al., 2019), indicating a novel mechanism that skin microbial metabolites modulating AD pathogenesis. In the Langerhans cells (LCs), IAId increases the production of indoleamine 2,3-dioxygenase and IL-10 (Liu et al., 2020). Additionally, IAId induces a mature phenotype of LCs, leading to the inhibition of CD4+ T cell proliferation and IL-10 secretion (Liu et al., 2020), revealing its negative regulation of LC function in AD pathogenesis.
AD patients also demonstrates a dysregulated lipidome of sebum and aberrant lipid metabolism in sebaceous glands (Yin et al., 2023). Interestingly, the levels of sebum and its microbial metabolite, propionate, are lower on the skins of AD patients compared with those of healthy individuals (Qiu et al., 2022). Mice lacking sebum spontaneously develop AD-like dermatitis, which can be improved by topical propionate application (Qiu et al., 2022), pointing the sebum-propionate axis as a protector pattern in AD. A proof-of-concept clinical study further demonstrates the beneficial of topical propionate application in AD patients (Qiu et al., 2022), highlighting a possible therapeutic for AD treatment.
Accumulating clues have demonstrated that microbial dysbiosis can result in inflammatory skin diseases, such as atopic dermatitis (Williams and Gallo, 2015; Bjerre et al., 2017; Tao et al., 2022). The affected individuals are characteristically prone to colonized by different microorganisms, which may reflect the disease condition of patients. Specifically, the skin microbiota of AD patients shows increased Staphylococcus aureus (S. aureus) load, which is correlated with reduced bacterial diversity (Francuzik et al., 2018; Hui et al., 2020; Poh et al., 2022). Additionally, increased S. aureus abundance is positively related to exacerbated disease severity in AD (Edslev et al., 2021). Moreover, the intra-host genetic heterogeneity of the colonizing S. aureus provides evidence for within-host selection in AD patients (Harkins et al., 2018). In general, the distinct microbiotal colonization on AD skins are affected by endogenous and external factors (Shi et al., 2016). The endogenous factors are mainly composed of genetics, age, and skin sites, etc, while the exogenous factors are mainly composed of temperature, humidity, pressure, ultraviolet irradiation, and especially, various treatments (Figure 2).
Figure 2. Endogenous and exogenous factors that influence the dysbiosis of skin microbiota in AD patients. The distinct microbiotal colonization on the AD skins is affected by multiple endogenous and exogenous factors. The endogenous factors are mainly composed of genetics, age, and skin sites, etc, while the exogenous factors are mainly composed of temperature, humidity, pressure, ultraviolet irradiation, and especially, various treatments.
It is widely accepted that the genetic variations are closely associated with AD that shows high heritability. One of the main genes related to AD is the Filaggrin gene (FLG), the null mutation of which is a major risk factor for skin barrier dysfunction and tightly related to AD pathogenesis (Palmer et al., 2006; Margolis et al., 2012; Margolis et al., 2019; Hoyer et al., 2022). Interestingly, FLG mutation correlates with disease severity, as well as the skin microbiotal alteration in AD patients (Baurecht et al., 2018; Clausen et al., 2018; van Mierlo et al., 2022).
In pediatric patients with difficult-to-treat AD, the prevalence of FLG mutation is around 40%. Meanwhile, a significant effect of the FLG mutation on the overall skin microbiome is observed (van Mierlo et al., 2022). In adults, altered skin microbiota in AD patients is also detected, with microbial composition linked to the FLG mutation (Clausen et al., 2018). Importantly, the alpha diversity of microbiome shows inversely correlation with disease severity, and is lower in AD patients compared with healthy individuals (Clausen et al., 2018), proposing a possible correlation between skin microbiota and host genetics. Coincidentally, Baurecht et al. reported that AD displayed a distinct community structure, with the FLG-deficient skins showed a microbiota composition resembling the AD-related pattern (Baurecht et al., 2018). By incorporating the host genome and skin microbial composition into a computational model, Barnes et al. found that the bacterial communities varied markedly between AD patients without and with FLG mutations, further confirming the association between FLG mutation and the skin microbiotal diversity in AD (Barnes et al., 2022).
In Indian AD patients, the prevalence of FLG missense variants correlated with AD are significantly less than those reported in Europeans (Nath et al., 2020). Meanwhile, damaging missense single nucleotide polymorphisms of FLG gene are associated with the relative abundance of bacteria species (Nath et al., 2020). Specifically, increased S. aureus abundance in AD patients with FLG mutation is observed, suggesting that genetic differences are important for bacterial colonization on AD skins (Clausen et al., 2017). Mechanistically, FLG expression protects keratinocyte from staphylococcal alpha-toxin-induced death (Brauweiler et al., 2013). This partially explain why S. aureus α-toxin preferentially targets and destroys FLG-deficient keratinocytes, which also provides a mechanism for S. aureus-mediated exacerbation in AD pathogenesis.
The skin microbiomes differ significantly between children and adults, suggesting the possible role of age in altering the skin microbiota composition (Dominguez-Bello et al., 2010; Capone et al., 2011). For instance, Kennedy et al. demonstrated that skin microbiome, including the bacterial community and diversity, shift over time in infants (Kennedy et al., 2017). Interestingly, infantile AD patients do not have noticeably dysbiotic communities before disease flares and were not colonized by S. aureus before having AD. Moreover, reduced skin microbiome diversity is associated with an increased risk of AD in infancy (Halling et al., 2023). Additionally, commensal Staphylococci are less colonized in infants affected at month 12, suggesting their protective role against AD development (Kennedy et al., 2017). However, further studies are needed to confirm these observations.
In adult patients with AD, cutaneous S. aureus colonization positively correlates with skin barrier impairment, as well as the subsequent sensitization to antigens of skin-associated microorganisms (Jinnestål et al., 2014), illustrating its importance in eczema pathogenesis. Besides, S. aureus also play important roles in the pathophysiology of childhood AD (Hon et al., 2016). In infancy, S. aureus is more prevalent on the skin of infants who developed AD later on (Meylan et al., 2017). Further, infants positive for cutaneous S. aureus colonization are younger than uncolonized infants at AD onset (Meylan et al., 2017), suggesting that S. aureus colonization contributes to clinical AD onset at an early age, even in infancy.
In a large cohort study, Shi et al. systematically compared the skin microbiomes among different age groups to determine the effects of human physical developmental stages on AD (Shi et al., 2016). In the skin microbiome of healthy individuals, young children (age 2-12) demonstrated more diverse than adults (age 18-62), with distinct beta diversity and significant differences at both genus and specie levels. Similarly, differences between young children and adults and/or teenagers (age 13-17) were observed in the skin microbiome of AD patients (Shi et al., 2016). Since teenagers are in transition from young children to adults in physical development, their skin microbiomes are in transition as well, with a higher similarity to adults than young children, providing an explanation for the age differences in AD from a perspective of skin microbiome.
It is known that different sites in human skin are colonized by distinct microbial communities (Byrd et al., 2018; Manus et al., 2020; Boxberger et al., 2021). Moreover, the microbial heterogeneity varies between skin sites in AD lesions, which may reflect disease severity. For instance, although correlations exist between species in the microbiota of nose and skin, the nose and skin harbour distinct microbial communities in pediatric AD (Totté et al., 2019). Meanwhile, the skin and oral cavity of AD patients exhibit differential reductions in microbial diversity compared with healthy individuals, which are distinctly correlated with disease severity (Li et al., 2019). Additionally, the different habitats of AD patients exhibited site-specific alterations at the genus level, with many skin-specific microbes showing opposing directions of enrichment in oral cavity (Li et al., 2019). Specifically, the relative abundance of S. aureus is associated with disease severity in the posterior thigh, but not in the upper back of AD patients (Ottman et al., 2021). Though it is unclear what is the cause for this distinct microbial colonization, it shows that AD may select for specific microbes, such as S. aureus in different anatomical locations (Ottman et al., 2021). Above researches highlight the importance of considering the variability across skin sites when studying the microbial heterogeneity in AD.
Despite the growing understanding of the close correlation between endogenous factors and AD dysbiosis, various external factors—such as personal care products (including soaps and detergents), temperature and/or climate changes, environmental triggers like air pollution, ultraviolet radiation, and allergenic foods/diet—can also disrupt the balance of skin microbiota, leading to skin dysbiosis and contributing to the exacerbation of AD.
As we all know, skin barrier disruption is one of the contributing factors that promote the development of AD (Yue et al., 2021). Consequently, irritants or environmental factors that compromise the skin’s barrier function can increase the skin’s susceptibility to microbial colonization, even resulting in skin inflammation (Yue et al., 2021). For example, factors that determine water hardness, such as high domestic water calcium carbonate concentrations, positively correlated with the prevalence of infant AD (Perkin et al., 2016). Meanwhile, the effect of mineral content in tap water were greater in children carrying FLG mutations, who favor AD development and flares (Perkin et al., 2016; Jabbar-Lopez et al., 2020). Additionally, climate changes, such as dry climates and low humidity, can exacerbate AD symptoms by disrupting the skin barrier and altering the skin microbiota (Darlenski et al., 2021; Wrześniewska et al., 2024). Moreover, personal hygiene practices or hygiene habits, such as friction and the frequent use of alkaline or antimicrobial soaps, can disrupt the skin microbiome and facilitate the pathogenesis of AD (Jing et al., 2020; Skowron et al., 2021). Furthermore, there is growing evidence that diet and gut microbiota are closely associated with skin dysbiosis and the prevalence of AD (Tham et al., 2024). A clear link between gut microbiome imbalances and AD suggests the potential influence of the gut-skin axis on skin inflammation (Moniaga et al., 2022; Hoskinson et al., 2024; Jiang et al., 2024; Rios-Carlos et al., 2024).
Therapeutics have shown great success on AD treatment in recent years. Meanwhile, different treatments may lead to varied skin microbiota shifts in AD patients (Guo et al., 2022). Herein, we summarized the recent progresses that different treatments influencing the skin microbiota alterations in AD (Table 1).
Topical emollients are considered as a first-line and mainstay therapy for AD treatment, which can reduce disease severity both in children and adults with AD (Tiplica et al., 2018; Pinter et al., 2019). Moreover, emollient therapy from birth represents an effective and safe approach for AD prevention (Simpson et al., 2014; Skjerven et al., 2020; Zhong et al., 2022). Further, emollients are used as monotherapy or adjuncts to soften and moisturize the skin (Hon et al., 2013; Giam et al., 2016; van Zuuren et al., 2017). However, the qualities and clinical effects of different emollients vary significantly, and only certain types are able to improve the skin’s barrier function and protect against irritants that trigger eczema (Danby et al., 2022; Elias, 2022). It was recently reported that one specific emollient associated with selected carbon material reduced the number and severity of AD flares. Concurrently, it normalized the skin microbiota compared with other emollients (Seité et al., 2017), suggesting that skin microbiota shifts could be used as biomarkers to evaluate the efficacy of different emollients during AD treatment.
Through clinical analysis and high-throughput sequencing of the skin microbiome, Seité et al. revealed that emollient improved the clinical symptoms in 72% of the AD patients, with the microbial communities in lesional skin more closely resembled unaffected skin after treatment, as indicated by increased overall diversity and decreased Staphylococcus species (Seite et al., 2014). Interestingly, the skin physiology, cutaneous microbiome, and AD severity were not affected by the pH of applied emollient (either pH 8.5 or pH 5.5). Instead, the skin pH tightly regulated by intrinsic factors limited the abundance of S. aureus in AD (Hülpüsch et al., 2020). Consistently, decreased skin pH, as well as increased S. salivarius population after emollient use, contribute to the preventative effects of emollient in infants at high-risk for developing AD (Glatz et al., 2018). Appropriate use of formulated emollient also increases the richness of skin microbiome, as well as the levels of ceramides and free fatty acids that play important roles in skin barrier integrity in infants, further underlining the predictive role of skin microbiota during the management of infant AD using emollient (Capone et al., 2023).
Emollient treatments also induce changes in fungal microbiome in AD, since the mycobiomes of pre-treatment and post-treatment samples cluster differently at all taxa levels by principal coordinate analysis (Chandra et al., 2018). Specifically, gram-negative Pseudomonas spp. significantly correlates with pathogenic fungal species (Aspergillus, Candida spp.) in lesional skins of pre-treatment group, but not in the post-treatment group (Chandra et al., 2018). Furthermore, lesional skins exhibit significant correlation between gram-positive bacteria (Corynebacterium kroppenstedtiian and Staphylococcus pettenkoferi) and pathogenic Candida species in the pre-treatment group, but not in the post-treatment group (Chandra et al., 2018). Thus, emollient induces beneficial changes in the skin mycobiome and modulates the microbe homeostasis in AD skin.
Patients with AD are prone to skin infections, most of which are suspected of contributing to pathogenesis. Thus, bleach baths could improve AD symptoms by reducing the skin microbial burden.
In a randomized, placebo-controlled, and single-blinded trial, skin samples from children with/without AD were examined at baseline and after 4 weeks’ treatment with topical corticosteroid (TCS) alone or TCS plus bleach bath. After TCS + bleach bath or TCS alone treatment, bacterial compositions on the lesional skins normalized, resembling non-lesional skin, with a tendency of restoring to the healthy skins (Gonzalez et al., 2016), indicating that TCS treatment alone is sufficient to normalize the cutaneous microbiota in AD. However, it does not mean that bleach baths have no additional impact on the skin microbiota, since 4 weeks’ time period may not be long enough for this kind of observation. Consistently, both standard treatment (emollient plus TCS) + dilute bleach bath (DBB) and only standard treatment improved disease severity in moderate-to-severe AD patients (Khadka et al., 2022). However, standard treatment with addition of DBB had significantly lower S. aureus burden than those who received only standard treatment over a three-month period (Khadka et al., 2022). Moreover, chronic use of DBB with intermittent application of mupirocin ointment decreased the disease severity of AD patients with clinical signs of secondary bacterial infections (Huang et al., 2009), further supporting its beneficial role of controlling bacteria load in AD therapy.
Apple cider vinegar has been shown to have antibacterial effects. Interestingly, no significant difference in cutaneous S. aureus presentation was observed in AD patients before and after 2 weeks’ treatment of 0.5% apple cider vinegar (Luu et al., 2021). However, additional studies are needed to explore the effects of higher concentrations and longer period of apple cider vinegar treatment on the skin microbiota in AD.
Upon topical coal tar treatment, a useful therapeutic for atopic hand and foot eczema (van den Bogaard et al., 2013; Wollenberg et al., 2020), Staphylococcus abundance decreased, whereas Propionibacterium abundance increased in AD skins (Smits et al., 2020), suggesting a shift of the microbiota composition toward that of healthy skin. Meanwhile, coal tar treatment restored the antimicrobial peptide levels in AD skins (Smits et al., 2020), confirming that coal tar can combat AD through targeting the skin microbiota and inflammation.
Ozone treatment improves AD conditions by decreasing the disease severity (Travagli et al., 2010; Zeng and Lu, 2018), which is accompanied by decreased S. aureus colonization in AD lesions (Zeng et al., 2020). Additionally, ozone therapy helps to restore the cutaneous microbiological diversity in AD patients (Zeng et al., 2020), revealing its role in modulating the skin microbiota in AD.
Tacrolimus, a calcineurin inhibitor, has been widely used as a maintenance therapy in AD (Cury Martins et al., 2015; Paller et al., 2020). Interestingly, tacrolimus positively affects the skin microbiota in AD, with increased commensal species observed following treatment (Wongpiyabovorn et al., 2019), underlining a novel mechanism that tacrolimus works as an useful strategy to alleviate AD.
Phototherapy is an effective treatment in dermatology, which has long been used for the management of various inflammatory skin diseases, such as AD (Ortiz-Salvador and Pérez-Ferriols, 2017; Kemény et al., 2019; Musters et al., 2021). For instance, narrow-band Ultraviolet B (NB-UVB) is widely used in AD treatment and can significantly improve the disease severity (Lossius et al., 2021). Interestingly, a drastic increase in the skin microbial diversity and decrease in the cutaneous S. aureus proportion are observed in AD patients following NB-UVB treatment (Kwon et al., 2019). Coincidentally, shift towards higher diversity in the microbiota of lesional skins after NB-UVB treatment is observed in AD patients, which is associated with disease improvement (Lossius et al., 2022), confirming the role of NB-UVB in regulating skin microbiota homeostasis.
The 308 nm excimer light is another effective treatment used in AD (Nisticò et al., 2008; Oh et al., 2016). It was recently reported that the 308 nm excimer light significantly changed the bacterial composition, as well as improved the skin barrier function in the lesional skins of AD (Kurosaki et al., 2020). In addition, the treatment decreased the relative abundance of S. aureus, which was correlated with improved clinical symptom in AD skins (Kurosaki et al., 2020), suggesting that alterations of the skin microbiota within excimer light treatment are partially involved in the improvement of AD severity. In dogs with canine AD, the 308-nm excimer light changed the composition and diversity of the skin microbiota, with increased abundance of phyla Actinobacteria and Cyanobacteria, and decreased abundance of S. pseudintermedius (Park et al., 2021). More importantly, it significantly alleviated the severity of canine AD without causing any serious side effects (Park et al., 2021), indicating that excimer light is also a suitable and safe therapy for canine AD.
Climatotherapy, including Dead Sea climatotherapy (DSC), alpine and moderate maritime climates, can improve the patient’s skin condition and have been widely used for the treatment of AD (Harari et al., 2000; Adler-Cohen et al., 2012; Fieten et al., 2015; Kudish et al., 2016; Heeringa et al., 2018). Notably, DSC treatment could partially attenuate the dysbiosis in the lesional skins of AD patients (Brandwein et al., 2019). Meanwhile, severe AD skins underwent the most significant community shifts after DSC treatment. Specifically, S. epidermidis, Streptococcus mitis, and Micrococcus luteus were significantly affected by DSC (Brandwein et al., 2019), showing new perspectives in the climatotherapy for AD. However, not all climate therapies affect skin microbiota in AD patients. The alpine climate treatment significantly changes, whereas moderate maritime climate treatment does not change the composition of skin microbiota on the lesional skins of AD patients (van Mierlo et al., 2019).
Dupilumab is a humanized antibody to IL-4 receptor α, which is effective in blocking IL-4 and IL-13 signaling, and reducing Th2 response (Gooderham et al., 2018). Currently, dupilumab is the only biologic medication approved by the US FDA for moderate-to-severe AD in adults and children, which can significantly improve symptoms and life quality of patients suffering from AD worldwide (Guttman-Yassky et al., 2019; Ariëns et al., 2020; Simpson et al., 2021; Zhao et al., 2022; Simpson et al., 2023). Interestingly, dupilumab rapidly reduces S. aureus abundance in subjects with moderate-severe AD, when compared with placebo. Importantly, patients with the greatest S. aureus reductions had the best clinical outcomes after dupilumab treatment (Pinter et al., 2019). Mechanistically, IL-4 receptor α blockade promotes S. aureus clearance partially by enhancing IL-17A expression from sites of allergic skin inflammation (Leyva-Castillo et al., 2023). The clinical improvement of AD mediated by dupilumab treatment is also positively correlated with increased microbial diversity (Callewaert et al., 2020; Olesen et al., 2021). Thus, dupilumab treatment changes the skin microbiome and ameliorates disease severity in AD. In consistent with this, recent data showed that dupilumab decreased the Eczema Area and Severity Index (EASI) as well as the load of S. aureus in AD patients (Lee et al., 2021). Concurrently, the microbial diversity and the abundance of Cutibacterium species increased (Lee et al., 2021), which were correlated with stratum corneum hydration levels and EASI improvement, suggesting that Th2 blockade-induced skin microbiotal normalization is associated with improved skin barrier properties in AD. Moreover, systemic dupilumab treatment tends to shift the skin microbiome of patients with moderate-to-severe AD toward a healthy skin flora, which is largely independent of the clinical response, indicating its direct regulation on the skin microbiome (Hartmann et al., 2023).
Alteration of the skin microbiota, especially the S. aureus colonization, is closely associated with disease severity in AD (Hendricks et al., 2019). Accordingly, commensal skin bacterium decolonizating S. aureus holds therapeutic benefits for AD patients (Table 2).
The culturable gram-negative bacteria from healthy people but not from AD patients are associated with enhanced barrier function, activated immunity, and controlled S. aureus colonization (Myles et al., 2016). Particularly, one commensal, Roseomonas mucosa (R. mucosa) from healthy skins, improves disease severity of AD in a mouse model, suggesting its therapeutic potential for AD treatment (Myles et al., 2016). Recently, a first-in-human topical microbiota transplantation using R. mucosa was conducted for AD treatment. In the open-labeled trial, AD patients topically treated with R. mucosa showed decreased S. aureus burden and disease severity, with no adverse events (Myles et al., 2018), supporting the efficacy and safety of R. mucosa therapy in AD treatment. More recently, a randomized and placebo-controlled trial of R. mucosa treatment in children with AD was conducted. In this trial, R. mucosa ameliorated disease severity, improved skin barrier function, decreased S. aureus abundance on the skin, and reduced topical steroid use without severe adverse events (Myles et al., 2020), further confirming that topical R. mucosa transplantation in AD patients is warranted.
To enhance the effects of R. mucosa transplantation long-termly, a living bacterial formulation that integrates R. mucosa with poly (vinyl pyrrolidone), poly (vinyl alcohol), and sodium alginate into a skin dressing was developed (Liu et al., 2022). Functionally, the skin dressing recovers skin barrier functions and alleviates AD-associated inflammation. Meanwhile, it serves as extrinsic culture harbors and nutrient suppliers to support R. mucosa survival (Liu et al., 2022), offering a clue that the combination of topical bacteria transplant with biomaterials represents an effective bacteriotherapy toward AD treatment.
As outlined in “2.2 Section”, the skin commensals, coagulase-negative staphylococci (CoNS) species residing on normal skins, inhibit S. aureus growth and development of AD (Nakatsuji et al., 2017; Paharik et al., 2017; Williams et al., 2019). Interestingly, S. aureus burden on the lesional skins of patients who received autologous antimicrobial-producing CoNS (CoNS-AM+) treatment was reduced by 99.2% compared with vehicle treatment, in a double-blind, vehicle-controlled, and single-center randomized trial (Nakatsuji et al., 2021a). Meanwhile, no serious adverse events were observed after treatment. Importantly, the autologous CoNS-AM+ treatment improved clinical outcomes in AD patients (Nakatsuji et al., 2021a), suggesting that autologous CoNS strains can safely decrease S. aureus colonization and improve disease severity.
Staphylococcus hominis A9 (ShA9), a bacterium isolated from healthy human skins, kills S. aureus on the skin of mice and inhibits PSMa expression from S. aureus (Nakatsuji et al., 2017; Nakatsuji et al., 2021b). Additionally, a first-in-human trial of topical ShA9 on the skins of adults with S. aureus-positive AD was conducted. The topical ShA9 treatment showed few adverse events on participants, while a significant decrease in S. aureus was observed after ShA9 treatment (Nakatsuji et al., 2021b). Although some S. aureus strains on participants were not directly killed by ShA9, mRNA expression of PSMa was inhibited in all strains. The post-hoc analysis revealed improvement in disease severity by ShA9 (Nakatsuji et al., 2021b), demonstrating the benefits of ShA9 bacteriotherapy for AD treatment.
S. epidermidis is a well-known commensal bacteria that selectively kills S. aureus (Nakatsuji et al., 2017), suggesting its protective role against AD. On the agar well diffusion assay, live planktonic S. epidermidis clearly inhibits S. aureus growth, but heat-killed cells do not show this effect (Jang et al., 2020), leading to the hypothesis that cytoplasmic bacteriocin from S. epidermidis could be a promising agent to inhibit S. aureus growth. In fact, a novel thermolabile cytoplasmic bacteriocin extracted from S. epidermidis exhibits selectively antimicrobial activity against S. aureus and methicillin-resistance S. aureus (Jang et al., 2020). However, more data should be obtained to confirm its potency and safety, before its clinical use for AD treatment.
Antibiotics specific for bacterial species including C. mastitidis, S. aureus, and C. bovis, almost completely reverse dysbiosis and eliminate the skin inflammation in mouse models (Kobayashi et al., 2015). However, it should be concerned that the use of antibiotics may trigger antibiotic resistance and more serious dysbiosis. ATx201 (niclosamide, a small molecule), was recently reported to reduce both S. aureus and methicillin-resistant S. aureus colonization, without affecting the skin commensals (Weiss et al., 2022). Additionally, in a phase 2 trial, ATx201 ointment effectively reduced S. aureus colonization and increased the Shannon diversity of skin microbiomes in patients with mild-to-severe AD (Weiss et al., 2022), revealing that small molecules could work as a decolonizing agent in AD treatment.
Probiotics are potent immune-modulators used in AD management (Dissanayake and Shimojo, 2016; Fanfaret et al., 2021; Herbert et al., 2023). A cosmetic lotion containing heat-treated Lactobacillus johnsonii NCC 533, was recently demonstrated to control S. aureus colonization as well as improve clinical outcomes in AD patients, supporting the development of topical lotion containing probiotics for AD treatments (Blanchet-Réthoré et al., 2017). More recently, ointment containing live Lactobacillus reuteri DSM 17938 was also shown to improve the local symptoms in adult AD patients (Butler et al., 2020). Most importantly, Lactobacillus reuteri DSM 17938 is cutaneously acceptable and safe, confirming that probiotics are safe and promising therapeutics in the management of AD.
Natural products represent new therapeutics combating against AD. Aquaphilus dolomiae (ADE) is an aqueous protein extract, which increases IL-10 expression in monocyte-derived dendritic cells (moDC) (Martin et al., 2016). Meanwhile, ADE-moDC counteracts the proliferative effect and mitogenic effect of S. aureus on CD4+ T cells (Martin et al., 2016). Thus, owing to the role of S. aureus in driving inflammation in AD, the immunosuppressive property of the ADE might be useful to reduce disease severity. Moreover, topical application of a Castanea sativa extract prevents the progression of AD, through increasing filaggrin, claudin-1, and loricrin expression, while decreasing S. aureus virulence (Cadau et al., 2022), further confirming that natural products hold great potential for AD treatment.
The dysbiosis of skin microbiota is widely accepted as important mechanisms in the pathogenesis of AD. Specifically, the decreased microbial diversity and increased S. aureus colonization are closed associated with disease severity in AD. Meanwhile, therapeutics targeting the cutaneous microbiota represent a novel strategy for AD treatment. Despite the significant potential of microbiota-based therapies for AD, the treatment of dysbiosis should be integrated with standard skincare practices, since AD is a complex skin disorder that requires a multifaceted approach, incorporating various skincare modalities and treatments for effective management. Although numerous studies, even several early phage clinical trials have shown that skin microbiota-based therapeutics hold great promise for the treatment of AD, the precise mechanisms remain to be elucidated, and many problems remain to be solved for their better clinical applications. For instance, current studies mainly focus on the role of skin microbiome in AD pathogenesis and its potential use in AD treatment. However, we cannot ignore that changes and dysregulations of skin microbiome can work as biomarkers for AD diagnosis, especially now that a variety of non-invasive sampling methods are developed (Elpa et al., 2021; Andersson et al., 2022; Shima et al., 2022). Meanwhile, we need to build/construct a systematic model, which comprehensively considers the skin microbiota and host as a whole that involved in the management of AD, since skin microbiota is affected by multiple internal and external factors during AD pathogenesis. The current researches regarding the interaction between skin microbiota and AD were mainly conducted on cellular or mouse models, some were conducted on preclinical or phase I/II clinical models, thus there is still a long way to go before these investigations can be truly applied to the clinic. However, we are confident about the application prospects of skin microbiota-based therapeutics and believe that theses therapeutics can be widely promoted for AD treatment in the near future.
CH: Conceptualization, Funding acquisition, Writing – original draft, Writing – review & editing. FZ: Writing – original draft, Writing – review & editing. YG: Funding acquisition, Writing – original draft, Writing – review & editing. SW: Writing – original draft, Writing – review & editing. KZ: Writing – original draft, Writing – review & editing. XL: Writing – original draft, Writing – review & editing. WD: Writing – original draft, Writing – review & editing. XD: Supervision, Writing – original draft, Writing – review & editing. BY: Funding acquisition, Supervision, Writing – original draft, Writing – review & editing.
The author(s) declare that financial support was received for the research, authorship, and/or publication of this article. This work was supported by grants from the National Natural Science Foundation of China (82103726 and 82103727), Shenzhen Science and Technology Program (JCYJ20210324110008023, JCYJ20230807095809019, and RCBS20210706092408008), Guangdong Basic and Applied Basic Research Foundation (2022A1515010957 and 2023A1515010575), Shenzhen Sanming Project (SZSM202311029), Shenzhen Key Medical Discipline Construction Fund (SZXK040), Shenzhen High-level Hospital Construction Fund and Peking University Shenzhen Hospital Scientific Research Fund (KYQD2024378).
The authors declare that the research was conducted in the absence of any commercial or financial relationships that could be construed as a potential conflict of interest.
The authors declare that no Generative AI was used in the creation of this manuscript.
All claims expressed in this article are solely those of the authors and do not necessarily represent those of their affiliated organizations, or those of the publisher, the editors and the reviewers. Any product that may be evaluated in this article, or claim that may be made by its manufacturer, is not guaranteed or endorsed by the publisher.
Adler-Cohen, C., Czarnowicki, T., Dreiher, J., Ruzicka, T., Ingber, A., Harari, M. (2012). Climatotherapy at the Dead Sea: an effective treatment modality for atopic dermatitis with significant positive impact on quality of life. Dermatitis 23, 75–80. doi: 10.1097/DER.0b013e31824a6141
Al Kindi, A., Williams, H., Matsuda, K., Alkahtani, A. M., Saville, C., Bennett, H., et al. (2021). Staphylococcus aureus second immunoglobulin-binding protein drives atopic dermatitis via IL-33. J. Allergy Clin. Immunol. 147, 1354–1368. doi: 10.1016/j.jaci.2020.09.023
Andersson, A. M., Sølberg, J., Koch, A., Skov, L., Jakasa, I., Kezic, S., et al. (2022). Assessment of biomarkers in pediatric atopic dermatitis by tape strips and skin biopsies. Allergy 77, 1499–1509. doi: 10.1111/all.15153
Ariëns, L. F. M., van der Schaft, J., Bakker, D. S., Balak, D., Romeijn, M. L. E., Kouwenhoven, T., et al. (2020). Dupilumab is very effective in a large cohort of difficult-to-treat adult atopic dermatitis patients: First clinical and biomarker results from the BioDay registry. Allergy 75, 116–126. doi: 10.1111/all.14080
Barnes, C. J., Clausen, M. L., Asplund, M., Rasmussen, L., Olesen, C. M., Yüsel, Y. T., et al. (2022). Temporal and spatial variation of the skin-associated bacteria from healthy participants and atopic dermatitis patients. mSphere 7, e0091721. doi: 10.1128/msphere.00917-21
Baurecht, H., Rühlemann, M. C., Rodríguez, E., Thielking, F., Harder, I., Erkens, A. S., et al. (2018). Epidermal lipid composition, barrier integrity, and eczematous inflammation are associated with skin microbiome configuration. J. Allergy Clin. Immunol. 141, 1668–1676. doi: 10.1016/j.jaci.2018.01.019
Bitschar, K., Staudenmaier, L., Klink, L., Focken, J., Sauer, B., Fehrenbacher, B., et al. (2020). Staphylococcus aureus skin colonization is enhanced by the interaction of neutrophil extracellular traps with keratinocytes. J. Invest. Dermatol. 140, 1054–1065. doi: 10.1016/j.jid.2019.10.017
Bjerre, R. D., Bandier, J., Skov, L., Engstrand, L., Johansen, J. D. (2017). The role of the skin microbiome in atopic dermatitis: a systematic review. Br. J. Dermatol. 177, 1272–1278. doi: 10.1111/bjd.15390
Blanchet-Réthoré, S., Bourdès, V., Mercenier, A., Haddar, C. H., Verhoeven, P. O., Andres, P. (2017). Effect of a lotion containing the heat-treated probiotic strain Lactobacillus johnsonii NCC 533 on Staphylococcus aureus colonization in atopic dermatitis. Clin. Cosmet. Investig. Dermatol. 10, 249–257. doi: 10.2147/CCID.S135529
Boxberger, M., Cenizo, V., Cassir, N., La Scola, B. (2021). Challenges in exploring and manipulating the human skin microbiome. Microbiome 9, 125. doi: 10.1186/s40168-021-01062-5
Brandwein, M., Fuks, G., Israel, A., Sabbah, F., Hodak, E., Szitenberg, A., et al. (2019). Skin microbiome compositional changes in atopic dermatitis accompany dead sea climatotherapy. Photochem. Photobiol. 95, 1446–1453. doi: 10.1111/php.13119
Brauweiler, A. M., Bin, L., Kim, B. E., Oyoshi, M. K., Geha, R. S., Goleva, E., et al. (2013). Filaggrin-dependent secretion of sphingomyelinase protects against staphylococcal alpha-toxin-induced keratinocyte death. J. Allergy Clin. Immunol. 131, 421–427. doi: 10.1016/j.jaci.2012.10.030
Brauweiler, A. M., Goleva, E., Leung, D. Y. M. (2014). Th2 cytokines increase Staphylococcus aureus alpha toxin-induced keratinocyte death through the signal transducer and activator of transcription 6 (STAT6). J. Invest. Dermatol. 134, 2114–2121. doi: 10.1038/jid.2014.43
Butler, É., Lundqvist, C., Axelsson, J. (2020). Lactobacillus reuteri DSM 17938 as a novel topical cosmetic ingredient: A proof of concept clinical study in adults with atopic dermatitis. Microorganisms 8, 1026. doi: 10.3390/microorganisms8071026
Byrd, A. L., Belkaid, Y., Segre, J. A. (2018). The human skin microbiome. Nat. Rev. Microbiol. 16, 143–155. doi: 10.1038/nrmicro.2017.157
Byrd, A. L., Deming, C., Cassidy, S. K. B., Harrison, O. J., Ng, W. I., Conlan, S., et al. (2017). Staphylococcus aureus and Staphylococcus epidermidis strain diversity underlying pediatric atopic dermatitis. Sci. Transl. Med. 9, eaal4651. doi: 10.1126/scitranslmed.aal4651
Cadau, S., Gault, M., Berthelemy, N., Hsu, C. Y., Danoux, L., Pelletier, N., et al. (2022). An inflamed and infected reconstructed human epidermis to study atopic dermatitis and skin care ingredients. Int. J. Mol. Sci. 23, 12880. doi: 10.3390/ijms232112880
Callewaert, C., Nakatsuji, T., Knight, R., Kosciolek, T., Vrbanac, A., Kotol, P., et al. (2020). IL-4Rα blockade by dupilumab decreases Staphylococcus aureus colonization and increases microbial diversity in atopic dermatitis. J. Invest. Dermatol. 140, 191–202. doi: 10.1016/j.jid.2019.05.024
Capone, K. A., Dowd, S. E., Stamatas, G. N., Nikolovski, J. (2011). Diversity of the human skin microbiome early in life. J. Invest. Dermatol. 131, 2026–2032. doi: 10.1038/jid.2011.168
Capone, K. A., Friscia, D. L., Nikolovski, J., Telofski, L. S., Stamatas, G. N. (2023). A randomized clinical study on the effects of emollient use on the developing infant skin microbiome and metabolome. Exp. Dermatol. 32, 75–77. doi: 10.1111/exd.14684
Cau, L., Williams, M. R., Butcher, A. M., Nakatsuji, T., Kavanaugh, J. S., Cheng, J. Y., et al. (2021). Staphylococcus epidermidis protease EcpA can be a deleterious component of the skin microbiome in atopic dermatitis. J. Allergy Clin. Immunol. 147, 955–966. doi: 10.1016/j.jaci.2020.06.024
Chandra, J., Retuerto, M., Seité, S., Martin, R., Kus, M., Ghannoum, M. A., et al. (2018). Effect of an emollient on the mycobiome of atopic dermatitis patients. J. Drugs Dermatol. 17, 1039–1048.
Cho, S. H., Strickland, I., Boguniewicz, M., Leung, D. Y. (2001). Fibronectin and fibrinogen contribute to the enhanced binding of Staphylococcus aureus to atopic skin. J. Allergy Clin. Immunol. 108, 269–274. doi: 10.1067/mai.2001.117455
Clausen, M. L., Agner, T., Lilje, B., Edslev, S. M., Johannesen, T. B., Andersen, P. S. (2018). Association of disease severity with skin microbiome and filaggrin gene mutations in adult atopic dermatitis. JAMA Dermatol. 154, 293–300. doi: 10.1001/jamadermatol.2017.5440
Clausen, M. L., Edslev, S. M., Andersen, P. S., Clemmensen, K., Krogfelt, K. A., Agner, T. (2017). Staphylococcus aureus colonization in atopic eczema and its association with filaggrin gene mutations. Br. J. Dermatol. 177, 1394–1400. doi: 10.1111/bjd.15470
Coates, M., Blanchard, S., MacLeod, A. S. (2018). Innate antimicrobial immunity in the skin: A protective barrier against bacteria, viruses, and fungi. PloS Pathog. 14, e1007353. doi: 10.1371/journal.ppat.1007353
Coates, M., Lee, M. J., Norton, D., MacLeod, A. S. (2019). The skin and intestinal microbiota and their specific innate immune systems. Front. Immunol. 10. doi: 10.3389/fimmu.2019.02950
Cury Martins, J., Martins, C., Aoki, V., Gois, A. F., Ishii, H. A., da Silva, E. M. (2015). Topical tacrolimus for atopic dermatitis. Cochrane Database Syst. Rev. 2015, CD009864. doi: 10.1002/14651858.CD009864.pub2
Danby, S. G., Andrew, P. V., Taylor, R. N., Kay, L. J., Chittock, J., Pinnock, A., et al. (2022). Different types of emollient cream exhibit diverse physiological effects on the skin barrier in adults with atopic dermatitis. Clin. Exp. Dermatol. 47, 1154–1164. doi: 10.1111/ced.15141
Darlenski, R., Kozyrskyj, A. L., Fluhr, J. W., Caraballo, L. (2021). Association between barrier impairment and skin microbiota in atopic dermatitis from a global perspective: Unmet needs and open questions. J. Allergy Clin. Immunol. 148, 1387–1393. doi: 10.1016/j.jaci.2021.10.002
Dissanayake, E., Shimojo, N. (2016). Probiotics and prebiotics in the prevention and treatment of atopic dermatitis. Pediatr. Allergy Immunol. Pulmonol. 29, 174–180. doi: 10.1089/ped.2016.0708
Dominguez-Bello, M. G., Costello, E. K., Contreras, M., Magris, M., Hidalgo, G., Fierer, N., et al. (2010). Delivery mode shapes the acquisition and structure of the initial microbiota across multiple body habitats in newborns. Proc. Natl. Acad. Sci. U.S.A. 107, 11971–11975. doi: 10.1073/pnas.1002601107
Edslev, S. M., Olesen, C. M., Nørreslet, L. B., Ingham, A. C., Iversen, S., Lilje, B., et al. (2021). Staphylococcal communities on skin are associated with atopic dermatitis and disease severity. Microorganisms 9, 432. doi: 10.3390/microorganisms9020432
Elias, P. M. (2022). Optimizing emollient therapy for skin barrier repair in atopic dermatitis. Ann. Allergy Asthma Immunol. 128, 505–511. doi: 10.1016/j.anai.2022.01.012
Elpa, D. P., Chiu, H. Y., Wu, S. P., Urban, P. L. (2021). Skin metabolomics. Trends Endocrinol. Metab. 32, 66–75. doi: 10.1016/j.tem.2020.11.009
Fanfaret, I. S., Boda, D., Ion, L. M., Hosseyni, D., Leru, P., Ali, S., et al. (2021). Probiotics and prebiotics in atopic dermatitis: Pros and cons (Review). Exp. Ther. Med. 22, 1376. doi: 10.3892/etm.2021.10811
Fieten, K. B., Weststrate, A. C., van Zuuren, E. J., Bruijnzeel-Koomen, C. A., Pasmans, S. G. (2015). Alpine climate treatment of atopic dermatitis: a systematic review. Allergy 70, 12–25. doi: 10.1111/all.12514
Flowers, L., Grice, E. A. (2020). The skin microbiota: balancing risk and reward. Cell Host Microbe 28, 190–200. doi: 10.1016/j.chom.2020.06.017
Francuzik, W., Franke, K., Schumann, R. R., Heine, G., Worm, M. (2018). Propionibacterium acnes Abundance Correlates Inversely with Staphylococcus aureus: Data from Atopic Dermatitis Skin Microbiome. Acta Derm. Venereol. 98, 490–495. doi: 10.2340/00015555-2896
Giam, Y. C., Hebert, A. A., Dizon, M. V., Van Bever, H., Tiongco-Recto, M., Kim, K. H., et al. (2016). A review on the role of moisturizers for atopic dermatitis. Asia Pac. Allergy 6, 120–128. doi: 10.5415/apallergy.2016.6.2.120
Glatz, M., Jo, J. H., Kennedy, E. A., Polley, E. C., Segre, J. A., Simpson, E. L., et al. (2018). Emollient use alters skin barrier and microbes in infants at risk for developing atopic dermatitis. PloS One 13, e0192443. doi: 10.1371/journal.pone.0192443
Goh, J. P. Z., Ruchti, F., Poh, S. E., Koh, W. L. C., Tan, K. Y., Lim, Y. T., et al. (2022). The human pathobiont Malassezia furfur secreted protease Mfsap1 regulates cell dispersal and exacerbates skin inflammation. Proc. Natl. Acad. Sci. U.S.A. 119, e2212533119. doi: 10.1073/pnas.2212533119
Gonzalez, M. E., Schaffer, J. V., Orlow, S. J., Gao, Z., Li, H., Alekseyenko, A. V., et al. (2016). Cutaneous microbiome effects of fluticasone propionate cream and adjunctive bleach baths in childhood atopic dermatitis. J. Am. Acad. Dermatol. 75, 481–493. doi: 10.1016/j.jaad.2016.04.066
Gooderham, M. J., Hong, H. C., Eshtiaghi, P., Papp, K. A. (2018). Dupilumab: A review of its use in the treatment of atopic dermatitis. J. Am. Acad. Dermatol. 78, 28–36. doi: 10.1016/j.jaad.2017.12.022
Guo, Y., Dou, X., Jian, X. L., Zhang, K. Y., Zheng, Y. J., Yu, B. (2022). Effect of treatments on skin microbiota in patients with atopic dermatitis: a protocol for systematic review. BMJ Open 12, e053488. doi: 10.1136/bmjopen-2021-053488
Guttman-Yassky, E., Bissonnette, R., Ungar, B., Suárez-Fariñas, M., Ardeleanu, M., Esaki, H., et al. (2019). Dupilumab progressively improves systemic and cutaneous abnormalities in patients with atopic dermatitis. J. Allergy Clin. Immunol. 143, 155–172. doi: 10.1016/j.jaci.2018.08.022
Halling, A. S., Fritz, B. G., Gerner, T., Rinnov, M. R., Bay, L., Knudgaard, M. H., et al. (2023). Reduced skin microbiome diversity in infancy is associated with increased risk of atopic dermatitis in high-risk children. J. Invest. Dermatol. 143, S0022–202X(23)01989-9. doi: 10.1016/j.jid.2023.03.1682
Hammond, M., Gamal, A., Mukherjee, P. K., Damiani, G., McCormick, T. S., Ghannoum, M. A., et al. (2022). Cutaneous dysbiosis may amplify barrier dysfunction in patients with atopic dermatitis. Front. Microbiol. 13. doi: 10.3389/fmicb.2022.944365
Harari, M., Shani, J., Seidl, V., Hristakieva, E. (2000). Climatotherapy of atopic dermatitis at the Dead Sea: demographic evaluation and cost-effectiveness. Int. J. Dermatol. 39, 59–69. doi: 10.1046/j.1365-4362.2000.00840.x
Harkins, C. P., Pettigrew, K. A., Oravcová, K., Gardner, J., Hearn, R. M. R., Rice, D., et al. (2018). The microevolution and epidemiology of Staphylococcus aureus colonization during atopic eczema disease flare. J. Invest. Dermatol. 138, 336–343. doi: 10.1016/j.jid.2017.09.023
Hartmann, J., Moitinho-Silva, L., Sander, N., Harder, I., Häsler, R., Rodriguez, E., et al. (2023). Dupilumab but not cyclosporine treatment shifts the microbiome toward a healthy skin flora in patients with moderate-to-severe atopic dermatitis. Allergy 78, 2290–2300. doi: 10.1111/all.15742
Heeringa, J. J., Fieten, K. B., Bruins, F. M., van Hoffen, E., Knol, E. F., Pasmans, S. G. M. A., et al. (2018). Treatment for moderate to severe atopic dermatitis in alpine and moderate maritime climates differentially affects helper T cells and memory B cells in children. Clin. Exp. Allergy 48, 679–690. doi: 10.1111/cea.13136
Hendricks, A. J., Mills, B. W., Shi, V. Y. (2019). Skin bacterial transplant in atopic dermatitis: Knowns, unknowns and emerging trends. J. Dermatol. Sci. 95, 56–61. doi: 10.1016/j.jdermsci.2019.07.001
Herbert, S., Haughton, R., Nava, J., Ji-Xu, A., Le, S. T., Maverakis, E. (2023). A review of topical probiotic therapy for atopic dermatitis. Clin. Exp. Dermatol. 48, 319–324. doi: 10.1093/ced/llac138
Hon, K. L., Leung, A. K., Barankin, B. (2013). Barrier repair therapy in atopic dermatitis: an overview. Am. J. Clin. Dermatol. 14, 389–399. doi: 10.1007/s40257-013-0033-9
Hon, K. L., Tsang, Y. C., Pong, N. H., Ng, C., Ip, M., Leung, T. F. (2016). Clinical features and Staphylococcus aureus colonization/infection in childhood atopic dermatitis. J. Dermatolog. Treat 27, 235–240. doi: 10.3109/09546634.2015.1093586
Hoskinson, C., Medeleanu, M. V., Reyna, M. E., Dai, D. L. Y., Chowdhury, B., Moraes, T. J., et al. (2024). Antibiotics taken within the first year of life are linked to infant gut microbiome disruption and elevated atopic dermatitis risk. J. Allergy Clin. Immunol. 154, 131–142. doi: 10.1016/j.jaci.2024.03.025
Hoyer, A., Rehbinder, E. M., Färdig, M., Asad, S., Lødrup Carlsen, K. C., Endre, K. M. A., et al. (2022). Filaggrin mutations in relation to skin barrier and atopic dermatitis in early infancy. Br. J. Dermatol. 186, 544–552. doi: 10.1111/bjd.20831
Huang, J. T., Abrams, M., Tlougan, B., Rademaker, A., Paller, A. S. (2009). Treatment of Staphylococcus aureus colonization in atopic dermatitis decreases disease severity. Pediatrics 123, 808–814. doi: 10.1542/peds.2008-2217
Hui, J. W., Goleva, E., Curran-Everett, D., Leung, D. Y. M. (2020). The atopic march and Staphylococcus aureus colonization are associated with fall birth. J. Allergy Clin. Immunol. Pract. 8, 3216–3218. doi: 10.1016/j.jaip.2020.05.014
Hülpüsch, C., Tremmel, K., Hammel, G., Bhattacharyya, M., de Tomassi, A., Nussbaumer, T., et al. (2020). Skin pH dependent Staphylococcus aureus abundance as predictor for increasing atopic. Allergy 75, 2888–2898. doi: 10.1111/all.14461
Iwamoto, K., Moriwaki, M., Niitsu, Y., Saino, M., Takahagi, S., Hisatsune, J., et al. (2017). Staphylococcus aureus from atopic dermatitis skin alters cytokine production triggered by monocyte-derived Langerhans cell. J. Dermatol. Sci. 88, 271–279. doi: 10.1016/j.jdermsci.2017.08.001
Jabbar-Lopez, Z. K., Craven, J., Logan, K., Greenblatt, D., Marrs, T., Radulovic, S., et al. (2020). Longitudinal analysis of the effect of water hardness on atopic eczema: evidence for gene-environment interaction. Br. J. Dermatol. 183, 285–293. doi: 10.1111/bjd.18597
Jang, I. T., Yang, M., Kim, H. J., Park, J. K. (2020). Novel Cytoplasmic Bacteriocin Compounds Derived from Staphylococcus epidermidis Selectively Kill Staphylococcus aureus, Including Methicillin-Resistant Staphylococcus aureus (MRSA). Pathogens 9, 87. doi: 10.3390/pathogens9020087
Jiang, S., Cai, M., Li, D., Chen, X., Chen, X., Huang, Q., et al. (2024). Association of breast milk-derived arachidonic acid-induced infant gut dysbiosis with the onset of atopic dermatitis. Gut 74, 45–57. doi: 10.1136/gutjnl-2024-332407
Jing, J. L. J., Pei Yi, T., Bose, R. J. C., McCarthy, J. R., Tharmalingam, N., Madheswaran, T. (2020). Hand sanitizers: A review on formulation aspects, adverse effects, and regulations. Int. J. Environ. Res. Public Health 17, 3326. doi: 10.3390/ijerph17093326
Jinnestål, C. L., Belfrage, E., Bäck, O., Schmidtchen, A., Sonesson, A. (2014). Skin barrier impairment correlates with cutaneous Staphylococcus aureus colonization and sensitization to skin-associated microbial antigens in adult patients with atopic dermatitis. Int. J. Dermatol. 53, 27–33. doi: 10.1111/ijd.12198
Kemény, L., Varga, E., Novak, Z. (2019). Advances in phototherapy for psoriasis and atopic dermatitis. Expert Rev. Clin. Immunol. 15, 1205–1214. doi: 10.1080/1744666X.2020.1672537
Kennedy, E. A., Connolly, J., Hourihane, J. O., Fallon, P. G., McLean, W. H. I., Murray, D., et al. (2017). Skin microbiome before development of atopic dermatitis: Early colonization with commensal staphylococci at 2 months is associated with a lower risk of atopic dermatitis at 1 year. J. Allergy Clin. Immunol. 139, 166–172. doi: 10.1016/j.jaci.2016.07.029
Khadka, V. D., Key, F. M., Romo-González, C., Martínez-Gayosso, A., Campos-Cabrera, B. L., Gerónimo-Gallegos, A., et al. (2022). The skin microbiome of patients with atopic dermatitis normalizes gradually during treatment. Front. Cell Infect. Microbiol. 11. doi: 10.3389/fcimb.2021.720674
Kobayashi, T., Glatz, M., Horiuchi, K., Kawasaki, H., Akiyama, H., Kaplan, D. H., et al. (2015). Dysbiosis and Staphyloccus aureus colonization drives inflammation in atopic dermatitis. Immunity 42, 756–766. doi: 10.1016/j.immuni.2015.03.014
Kudish, A., Marsakova, A., Jahn, I., Gkalpakiotis, S., Arenberger, P., Harari, M. (2016). Dead Sea ultraviolet climatotherapy for children with atopic dermatitis. Photodermatol. Photoimmunol. Photomed. 32, 254–261. doi: 10.1111/phpp.12250
Kurosaki, Y., Tsurumachi, M., Kamata, Y., Tominaga, M., Suga, Y., Takamori, K. (2020). Effects of 308 nm excimer light treatment on the skin microbiome of atopic dermatitis patients. Photodermatol. Photoimmunol. Photomed. 36, 185–191. doi: 10.1111/phpp.12531
Kwon, S., Choi, J. Y., Shin, J. W., Huh, C. H., Park, K. C., Du, M. H., et al. (2019). Changes in lesional and non-lesional skin microbiome during treatment of atopic dermatitis. Acta Derm. Venereol. 99, 284–290. doi: 10.2340/00015555-3089
Laborel-Préneron, E., Bianchi, P., Boralevi, F., Lehours, P., Fraysse, F., Morice-Picard, F., et al. (2015). Effects of the Staphylococcus aureus and Staphylococcus epidermidis Secretomes Isolated from the Skin Microbiota of Atopic Children on CD4+ T Cell Activation. PloS One 10, e0141067. doi: 10.1371/journal.pone.0141067
Langan, S. M., Irvine, A. D., Weidinger, S. (2020). Atopic dermatitis. Lancet 396, 345–360. doi: 10.1016/S0140-6736(20)31286-1
Lee, S. J., Kim, S. E., Shin, K. O., Park, K., Lee, S. E. (2021). Dupilumab therapy improves stratum corneum hydration and skin dysbiosis in patients with atopic dermatitis. Allergy Asthma Immunol. Res. 13, 762–775. doi: 10.4168/aair.2021.13.5.762
Leyva-Castillo, J. M., McGurk, A., Strakosha, M., Vega-Mendoza, D., Smith, S. E. M., Stafstrom, K., et al. (2023). IL-4 receptor alpha blockade dampens allergic inflammation and upregulates IL-17A expression to promote S. aureus clearance in antigen sensitized mouse skin. J. Allergy Clin. Immunol. 152, 907–915. doi: 10.1016/j.jaci.2023.05.025
Li, H., Goh, B. N., Teh, W. K., Jiang, Z., Goh, J. P. Z., Goh, A., et al. (2018). Skin Commensal Malassezia globosa Secreted Protease Attenuates Staphylococcus aureus Biofilm Formation. J. Invest. Dermatol. 138, 1137–1145. doi: 10.1016/j.jid.2017.11.034
Li, W., Xu, X., Wen, H., Wang, Z., Ding, C., Liu, X., et al. (2019). Inverse association between the skin and oral microbiota in atopic dermatitis. J. Invest. Dermatol. 139, 1779–1787. doi: 10.1016/j.jid.2019.02.009
Li, W., Yosipovitch, G. (2020). The role of the microbiome and microbiome−Derived metabolites in atopic dermatitis and non−Histaminergic itch. Am. J. Clin. Dermatol. 21, 44–50. doi: 10.1007/s40257-020-00538-8
Liu, H., Archer, N. K., Dillen, C. A., Wang, Y., Ashbaugh, A. G., Ortines, R. V., et al. (2017). Staphylococcus aureus Epicutaneous Exposure Drives Skin Inflammation via IL-36-Mediated T Cell Responses. Cell Host Microbe 22, 653–666. doi: 10.1016/j.chom.2017.10.006
Liu, X., Qin, Y., Dong, L., Han, Z., Liu, T., Tang, Y., et al. (2022). Living symbiotic bacteria-involved skin dressing to combat indigenous pathogens for microbiome-based biotherapy toward atopic dermatitis. Bioact. Mater. 21, 253–266. doi: 10.1016/j.bioactmat.2022.08.019
Liu, X., Zhang, X., Zhang, J., Luo, Y., Xu, B., Ling, S., et al. (2020). Activation of aryl hydrocarbon receptor in Langerhans cells by a microbial metabolite of tryptophan negatively regulates skin inflammation. J. Dermatol. Sci. 100, 192–200. doi: 10.1016/j.jdermsci.2020.10.004
Lossius, A. H., Berents, T. L., Saetre, F., Nilsen, H. R., Bradley, M., Asad, S., et al. (2021). Early transcriptional changes after UVB treatment in atopic dermatitis include inverse regulation of IL-36γ and IL-37. Exp. Dermatol. 30, 249–261. doi: 10.1111/exd.14217
Lossius, A. H., Sundnes, O., Ingham, A. C., Edslev, S. M., Bjørnholt, J. V., Lilje, B., et al. (2022). Shifts in the skin microbiota after UVB treatment in adult atopic dermatitis. Dermatology 238, 109–120. doi: 10.1159/000515236
Luu, L. A., Flowers, R. H., Gao, Y., Wu, M., Gasperino, S., Kellams, A. L., et al. (2021). Apple cider vinegar soaks do not alter the skin bacterial microbiome in atopic dermatitis. PloS One 16, e0252272. doi: 10.1371/journal.pone.0252272
Manus, M. B., Kuthyar, S., Perroni-Marañón, A. G., Núñez-de la Mora, A., Amato, K. R. (2020). Infant skin bacterial communities vary by skin site and infant age across populations in Mexico and the United States. mSystems 5, e00834–e00820. doi: 10.1128/mSystems.00834-20
Margolis, D. J., Apter, A. J., Gupta, J., Hoffstad, O., Papadopoulos, M., Campbell, L. E., et al. (2012). The persistence of atopic dermatitis and filaggrin (FLG) mutations in a US longitudinal cohort. J. Allergy Clin. Immunol. 130, 912–917. doi: 10.1016/j.jaci.2012.07.008
Margolis, D. J., Mitra, N., Wubbenhorst, B., D’Andrea, K., Kraya, A. A., Hoffstad, O., et al. (2019). Association of filaggrin loss-of-function variants with race in children with atopic dermatitis. JAMA Dermatol. 155, 1269–1276. doi: 10.1001/jamadermatol.2019.1946
Martin, H., Laborel-Préneron, E., Fraysse, F., Nguyen, T., Schmitt, A. M., Redoulès, D., et al. (2016). Aquaphilus dolomiae extract counteracts the effects of cutaneous S. aureus secretome isolated from atopic children on CD4+ T cell activation. Pharm. Biol. 54, 2782–2785. doi: 10.3109/13880209.2016.1173069
Meylan, P., Lang, C., Mermoud, S., Johannsen, A., Norrenberg, S., Hohl, D., et al. (2017). Skin colonization by Staphylococcus aureus precedes the clinical diagnosis of atopic dermatitis in infancy. J. Invest. Dermatol. 137, 2497–2504. doi: 10.1016/j.jid.2017.07.834
Moniaga, C. S., Tominaga, M., Takamori, K. (2022). An altered skin and gut microbiota are involved in the modulation of itch in atopic dermatitis. Cells 11, 3930. doi: 10.3390/cells11233930
Moriwaki, M., Iwamoto, K., Niitsu, Y., Matsushima, A., Yanase, Y., Hisatsune, J., et al. (2019). Staphylococcus aureus from atopic dermatitis skin accumulates in the lysosomes of keratinocytes with induction of IL-1α secretion via TLR9. Allergy 74, 560–571. doi: 10.1111/all.13622
Musters, A. H., Mashayekhi, S., Harvey, J., Axon, E., Lax, S. J., Flohr, C., et al. (2021). Phototherapy for atopic eczema. Cochrane Database Syst. Rev. 10, CD013870. doi: 10.1002/14651858.CD013870.pub2
Myles, I. A., Castillo, C. R., Barbian, K. D., Kanakabandi, K., Virtaneva, K., Fitzmeyer, E., et al. (2020). Therapeutic responses to Roseomonas mucosa in atopic dermatitis may involve lipid-mediated TNF-related epithelial repair. Sci. Transl. Med. 12, eaaz8631. doi: 10.1126/scitranslmed.aaz8631
Myles, I. A., Earland, N. J., Anderson, E. D., Moore, I. N., Kieh, M. D., Williams, K. W., et al. (2018). First-in-human topical microbiome transplantation with Roseomonas mucosa for atopic dermatitis. JCI Insight 3, e120608. doi: 10.1172/jci.insight.120608
Myles, I. A., Williams, K. W., Reckhow, J. D., Jammeh, M. L., Pincus, N. B., Sastalla, I., et al. (2016). Transplantation of human skin microbiota in models of atopic dermatitis. JCI Insight 1, e86955. doi: 10.1172/jci.insight.86955
Nakagawa, S., Matsumoto, M., Katayama, Y., Oguma, R., Wakabayashi, S., Nygaard, T., et al. (2017). Staphylococcus aureus virulent PSMa peptides induce keratinocyte alarmin release to orchestrate IL-17-dependent skin inflammation. Cell Host Microbe 22, 667–677. doi: 10.1016/j.chom.2017.10.008
Nakamura, Y., Oscherwitz, J., Cease, K. B., Chan, S. M., Muñoz-Planillo, R., Hasegawa, M., et al. (2013). Staphylococcus δ-toxin induces allergic skin disease by activating mast cells. Nature 503, 397–401. doi: 10.1038/nature12655
Nakatsuji, T., Chen, T. H., Narala, S., Chun, K. A., Two, A. M., Yun, T., et al. (2017). Antimicrobials from human skin commensal bacteria protect against Staphylococcus aureus and are deficient in atopic dermatitis. Sci. Transl. Med. 9, eaah4680. doi: 10.1126/scitranslmed.aah4680
Nakatsuji, T., Chen, T. H., Two, A. M., Chun, K. A., Narala, S., Geha, R. S., et al. (2016). Staphylococcus aureus exploits epidermal barrier defects in atopic dermatitis to trigger cytokine expression. J. Invest. Dermatol. 136, 2192–2200. doi: 10.1016/j.jid.2016.05.127
Nakatsuji, T., Gallo, R. L., Shafiq, F., Tong, Y., Chun, K., Butcher, A. M., et al. (2021a). Use of autologous bacteriotherapy to treat Staphylococcus aureus in patients with atopic dermatitis: A randomized double-blind clinical trial. JAMA Dermatol. 157, 978–982. doi: 10.1001/jamadermatol.2021.1311
Nakatsuji, T., Hata, T. R., Tong, Y., Cheng, J. Y., Shafiq, F., Butcher, A. M., et al. (2021b). Development of a human skin commensal microbe for bacteriotherapy of atopic dermatitis and use in a phase 1 randomized clinical trial. Nat. Med. 27, 700–709. doi: 10.1038/s41591-021-01256-2
Nath, S., Kumari, N., Bandyopadhyay, D., Sinha, N., Majumder, P. P., Mitra, R., et al. (2020). Dysbiotic lesional microbiome with filaggrin missense variants associate with atopic dermatitis in India. Front. Cell Infect. Microbiol. 10. doi: 10.3389/fcimb.2020.570423
Nisticò, S. P., Saraceno, R., Capriotti, E., Felice, C. D., Chimenti, S. (2008). Efficacy of monochromatic excimer light (308 nm) in the treatment of atopic dermatitis in adults and children. Photomed. Laser Surg. 26, 14–18. doi: 10.1089/pho.2007.2116
Oh, C. T., Kwon, T. R., Seok, J., Choi, E. J., Kim, S. R., Jang, Y. J., et al. (2016). Effect of a 308-nm excimer laser on atopic dermatitis-like skin lesions in NC/Nga mice. Lasers Surg. Med. 48, 629–637. doi: 10.1002/lsm.22524
Olesen, C. M., Ingham, A. C., Thomsen, S. F., Clausen, M. L., Andersen, P. S., Edslev, S. M., et al. (2021). Changes in skin and nasal microbiome and staphylococcal species following treatment of atopic dermatitis with dupilumab. Microorganisms 9, 1487. doi: 10.3390/microorganisms9071487
Ong, P. Y. (2022). Atopic dermatitis: Is innate or adaptive immunity in control? A clinical perspective. Front. Immunol. 13. doi: 10.3389/fimmu.2022.943640
Ortiz-Salvador, J. M., Pérez-Ferriols, A. (2017). Phototherapy in atopic dermatitis. Adv. Exp. Med. Biol. 996, 279–286. doi: 10.1007/978-3-319-56017-5_23
Ottman, N., Barrientos-Somarribas, M., Fyhrquist, N., Alexander, H., Wisgrill, L., Olah, P., et al. (2021). Microbial and transcriptional differences elucidate atopic dermatitis heterogeneity across skin sites. Allergy 76, 1173–1187. doi: 10.1111/all.14606
Paharik, A. E., Parlet, C. P., Chung, N., Todd, D. A., Rodriguez, E. I., Van Dyke, M. J., et al. (2017). Coagulase-negative staphylococcal strain prevents Staphylococcus aureus colonization and skin infection by blocking quorum sensing. Cell Host Microbe 22, 746–756. doi: 10.1016/j.chom.2017.11.001
Paller, A. S., Fölster-Holst, R., Chen, S. C., Diepgen, T. L., Elmets, C., Margolis, D. J., et al. (2020). No evidence of increased cancer incidence in children using topical tacrolimus for atopic dermatitis. J. Am. Acad. Dermatol. 83, 375–381. doi: 10.1016/j.jaad.2020.03.075
Paller, A. S., Kong, H. H., Seed, P., Naik, S., Scharschmidt, T. C., Gallo, R. L., et al. (2019). The microbiome in patients with atopic dermatitis. J. Allergy Clin. Immunol. 143, 26–35. doi: 10.1016/j.jaci.2018.11.015
Palmer, C. N., Irvine, A. D., Terron-Kwiatkowski, A., Zhao, Y., Liao, H., Lee, S. P., et al. (2006). Common loss of-function variants of the epidermal barrier protein filaggrin are a major predisposing factor for atopic dermatitis. Nat. Genet. 38, 441–446. doi: 10.1038/ng1767
Park, J. Y., Kim, S. M., Kim, J. H. (2021). Efficacy of phototherapy with 308-nm excimer light for skin microbiome dysbiosis and skin barrier dysfunction in canine atopic dermatitis. Front. Vet. Sci. 8. doi: 10.3389/fvets.2021.762961
Parlet, C. P., Brown, M. M., Horswill, A. R. (2019). Commensal staphylococci influence Staphylococcus aureus skin colonization and disease. Trends Microbiol. 27, 497–507. doi: 10.1016/j.tim.2019.01.008
Pastar, I., O’Neill, K., Padula, L., Head, C. R., Burgess, J. L., Chen, V., et al. (2020). Staphylococcus epidermidis boosts innate immune response by activation of gamma delta T cells and induction of perforin-2 in human skin. Front. Immunol. 11. doi: 10.3389/fimmu.2020.550946
Perkin, M. R., Craven, J., Logan, K., Strachan, D., Marrs, T., Radulovic, S., et al. (2016). Association between domestic water hardness, chlorine, and atopic dermatitis risk in early life: A population-based cross-sectional study. J. Allergy Clin. Immunol. 138, 509–516. doi: 10.1016/j.jaci.2016.03.031
Pinter, A., Thouvenin, M. D., Bacquey, A., Rossi, A. B., Nocera, T. (2019). Tolerability and efficacy of a medical device repairing emollient cream in children and adults with mild to moderate atopic dermatitis. Dermatol. Ther. (Heidelb) 9, 309–319. doi: 10.1007/s13555-019-0295-0
Poh, S. E., Koh, W. L. C., Lim, S. Y. D., Wang, E. C. E., Yew, Y. W., Common, J. E. A., et al. (2022). Expression of Staphylococcus aureus virulence factors in atopic dermatitis. JID Innov. 2, 100130. doi: 10.1016/j.xjidi.2022.100130
Prescott, S. L., Larcombe, D. L., Logan, A. C., West, C., Burks, W., Caraballo, L., et al. (2017). The skin microbiome: impact of modern environments on skin ecology, barrier integrity, and systemic immune programming. World Allergy Organ J. 10, 29. doi: 10.1186/s40413-017-0160-5
Qiu, Z., Zhu, Z., Liu, X., Chen, B., Yin, H., Gu, C., et al. (2022). A dysregulated sebum-microbial metabolite-IL-33 axis initiates skin inflammation in atopic dermatitis. J. Exp. Med. 219, e20212397. doi: 10.1084/jem.20212397
Richmond, J. M., Harris, J. E. (2014). Immunology and skin in health and disease. Cold Spring Harb. Perspect. Med. 4, a015339. doi: 10.1101/cshperspect.a015339
Rios-Carlos, M., Cervantes-García, D., Córdova-Dávalos, L. E., Bermúdez-Humarán, L. G., Salinas, E. (2024). Unraveling the gut-skin axis in atopic dermatitis: exploiting insights for therapeutic strategies. Gut Microbes 16, 2430420. doi: 10.1080/19490976.2024.2430420
Seite, S., Flores, G. E., Henley, J. B., Martin, R., Zelenkova, H., Aguilar, L., et al. (2014). Microbiome of affected and unaffected skin of patients with atopic dermatitis before and after emollient treatment. J. Drugs Dermatol. 13, 1365–1372.
Seité, S., Zelenkova, H., Martin, R. (2017). Clinical efficacy of emollients in atopic dermatitis patients-relationship with the skin microbiota modification. Clin. Cosmet. Investig. Dermatol. 10, 25–33. doi: 10.2147/CCID.S121910
Shi, B., Bangayan, N. J., Curd, E., Taylor, P. A., Gallo, R. L., Leung, D. Y. M., et al. (2016). The skin microbiome is different in pediatric versus adult atopic dermatitis. J. Allergy Clin. Immunol. 138, 1233–1236. doi: 10.1016/j.jaci.2016.04.053
Shima, K., Inoue, T., Uehara, Y., Iwamura, M., Fukagawa, S., Kuwano, T., et al. (2022). Non-invasive transcriptomic analysis using mRNAs in skin surface lipids obtained from children with mild-to-moderate atopic dermatitis. J. Eur. Acad. Dermatol. Venereol. 36, 1477–1485. doi: 10.1111/jdv.18173
Sidbury, R., Tom, W. L., Bergman, J. N., Cooper, K. D., Silverman, R. A., Berger, T. G., et al. (2014). Guidelines of care for the management of atopic dermatitis: section 4. Prevention of disease flares and use of adjunctive therapies and approaches. J. Am. Acad. Dermatol. 71, 1218–1233. doi: 10.1016/j.jaad.2014.08.038
Silverberg, J. I., Garg, N. K., Paller, A. S., Fishbein, A. B., Zee, P. C. (2015). Sleep disturbances in adults with eczema are associated with impaired overall health: a US population-based study. J. Invest. Dermatol. 135, 56–66. doi: 10.1038/jid.2014.325
Silverberg, J. I., Gelfand, J. M., Margolis, D. J., Boguniewicz, M., Fonacier, L., Grayson, M. H., et al. (2019). Pain is a common and burdensome symptom of atopic dermatitis in United States adults. J. Allergy Clin. Immunol. Pract. 7, 2699–2706. doi: 10.1016/j.jaip.2019.05.055
Simpson, E. L., Chalmers, J. R., Hanifin, J. M., Thomas, K. S., Cork, M. J., McLean, W. H., et al. (2014). Emollient enhancement of the skin barrier from birth offers effective atopic dermatitis prevention. J. Allergy Clin. Immunol. 134, 818–823. doi: 10.1016/j.jaci.2014.08.005
Simpson, E. L., Paller, A. S., Siegfried, E. C., Thaçi, D., Wollenberg, A., Cork, M. J., et al. (2021). Dupilumab demonstrates rapid and consistent improvement in extent and signs of atopic dermatitis across all anatomical regions in pediatric patients 6 years of age and older. Dermatol. Ther. (Heidelb) 11, 1643–1656. doi: 10.1007/s13555-021-00568-y
Simpson, E. L., Schlievert, P. M., Yoshida, T., Lussier, S., Boguniewicz, M., Hata, T., et al. (2023). Rapid reduction in Staphylococcus aureus in atopic dermatitis subjects following dupilumab treatment. J. Allergy Clin. Immunol. 152, 1179–1195. doi: 10.1016/j.jaci.2023.05.026
Skjerven, H. O., Rehbinder, E. M., Vettukattil, R., LeBlanc, M., Granum, B., Haugen, G., et al. (2020). Skin emollient and early complementary feeding to prevent infant atopic dermatitis (PreventADALL): a factorial, multicentre, cluster-randomised trial. Lancet 395, 951–961. doi: 10.1016/S0140-6736(19)32983-6
Skowron, K., Bauza-Kaszewska, J., Kraszewska, Z., Wiktorczyk-Kapischke, N., Grudlewska-Buda, K., Kwiecińska-Piróg, J., et al. (2021). Human skin microbiome: impact of intrinsic and extrinsic factors on skin microbiota. Microorganisms 9, 543. doi: 10.3390/microorganisms9030543
Smits, J. P. H., Ederveen, T. H. A., Rikken, G., van den Brink, N. J. M., van Vlijmen-Willems, I. M. J. J., Boekhorst, J., et al. (2020). Targeting the cutaneous microbiota in atopic dermatitis by coal tar via AHR-dependent induction of antimicrobial peptides. J. Invest. Dermatol. 140, 415–424. doi: 10.1016/j.jid.2019.06.142
Sparber, F., De Gregorio, C., Steckholzer, S., Ferreira, F. M., Dolowschiak, T., Ruchti, F., et al. (2019). The skin commensal yeast malassezia triggers a type 17 response that coordinates anti-fungal immunity and exacerbates skin inflammation. Cell Host Microbe 25, 389–403. doi: 10.1016/j.chom.2019.02.002
Strbo, N., Pastar, I., Romero, L., Chen, V., Vujanac, M., Sawaya, A. P., et al. (2019). Single cell analyses reveal specific distribution of anti-bacterial molecule Perforin-2 in human skin and its modulation by wounding and Staphylococcus aureus infection. Exp. Dermatol. 28, 225–232. doi: 10.1111/exd.13870
Swaney, M. H., Kalan, L. R. (2021). Living in your skin: microbes, molecules, and mechanisms. Infect. Immun. 89, e00695–e00620. doi: 10.1128/IAI.00695-20
Syed, A. K., Reed, T. J., Clark, K. L., Boles, B. R., Kahlenberg, J. M. (2015). Staphlyococcus aureus phenol-soluble modulins stimulate the release of proinflammatory cytokines from keratinocytes and are required for induction of skin inflammation. Infect. Immun. 83, 3428–3437. doi: 10.1128/IAI.00401-15
Tao, R., Li, R., Wang, R. (2022). Dysbiosis of skin mycobiome in atopic dermatitis. Mycoses 65, 285–293. doi: 10.1111/myc.13402
Tauber, M., Balica, S., Hsu, C. Y., Jean-Decoster, C., Lauze, C., Redoules, D., et al. (2016). Staphylococcus aureus density on lesional and nonlesional skin is strongly associated with disease severity in atopic dermatitis. J. Allergy Clin. Immunol. 137, 1272–1274. doi: 10.1016/j.jaci.2015.07.052
Tham, E. H., Chia, M., Riggioni, C., Nagarajan, N., Common, J. E. A., Kong, H. H. (2024). The skin microbiome in pediatric atopic dermatitis and food allergy. Allergy 79, 1470–1484. doi: 10.1111/all.16044
Tiplica, G. S., Boralevi, F., Konno, P., Malinauskiene, L., Kaszuba, A., Laurens, C., et al. (2018). The regular use of an emollient improves symptoms of atopic dermatitis in children: a randomized controlled study. J. Eur. Acad. Dermatol. Venereol. 32, 1180–1187. doi: 10.1111/jdv.14849
Totté, J. E. E., Pardo, L. M., Fieten, K. B., Vos, M. C., van den Broek, T. J., Schuren, F. H. J., et al. (2019). Nasal and skin microbiomes are associated with disease severity in paediatric atopic dermatitis. Br. J. Dermatol. 181, 796–804. doi: 10.1111/bjd.17755
Totté, J. E., van der Feltz, W. T., Hennekam, M., van Belkum, A., van Zuuren, E. J., Pasmans, S. G. (2016). Prevalence and odds of Staphylococcus aureus carriage in atopic dermatitis: a systematic review and meta-analysis. Br. J. Dermatol. 175, 687–695. doi: 10.1111/bjd.14566
Towell, A. M., Feuillie, C., Vitry, P., Da Costa, T. M., Mathelié-Guinlet, M., Kezic, S., et al. (2021). Staphylococcus aureus binds to the N-terminal region of corneodesmosin to adhere to the stratum corneum in atopic dermatitis. Proc. Natl. Acad. Sci. U.S.A. 118, e2014444118. doi: 10.1073/pnas.2014444118
Travagli, V., Zanardi, I., Valacchi, G., Bocci, V. (2010). Ozone and ozonated oils in skin diseases: a review. Mediators Inflammation 2010, 610418. doi: 10.1155/2010/610418
Uberoi, A., Bartow-McKenney, C., Zheng, Q., Flowers, L., Campbell, A., Knight, S. A. B., et al. (2021). Commensal microbiota regulates skin barrier function and repair via signaling through the aryl hydrocarbon receptor. Cell Host Microbe 29, 1235–1248. doi: 10.1016/j.chom.2021.05.011
Uluçkan, Ö., Jiménez, M., Roediger, B., Schnabl, J., Díez-Córdova, L. T., Troulé, K., et al. (2019). Cutaneous immune cell-microbiota interactions are controlled by epidermal JunB/AP-1. Cell Rep. 29, 844–859. doi: 10.1016/j.celrep.2019.09.042
Vaher, H., Kingo, K., Kolberg, P., Pook, M., Raam, L., Laanesoo, A., et al. (2023). Skin colonization with S. aureus can lead to increased NLRP1 inflammasome activation in patients with atopic dermatitis. J. Invest. Dermatol. 143, 1268–1278. doi: 10.1016/j.jid.2023.01.013
Vakharia, P. P., Chopra, R., Sacotte, R., Patel, K. R., Singam, V., Patel, N., et al. (2017). Burden of skin pain in atopic dermatitis. Ann. Allergy Asthma Immunol. 119, 548–552. doi: 10.1016/j.anai.2017.09.076
van den Bogaard, E. H., Bergboer, J. G., Vonk-Bergers, M., van Vlijmen-Willems, I. M., Hato, S. V., van der Valk, P. G., et al. (2013). Coal tar induces AHR-dependent skin barrier repair in atopic dermatitis. J. Clin. Invest. 123, 917–927. doi: 10.1172/JCI65642
van Mierlo, M. M. F., Pardo, L. M., Fieten, K. B., van den Broek, T. J., Schuren, F. H. J., van Geel, M., et al. (2022). The skin and nose microbiome and its association with filaggrin gene mutations in pediatric atopic dermatitis. Dermatology 238, 928–938. doi: 10.1159/000520978
van Mierlo, M. M. F., Totté, J. E. E., Fieten, K. B., van den Broek, T. J., Schuren, F. H. J., Pardo, L. M., et al. (2019). The influence of treatment in alpine and moderate maritime climate on the composition of the skin microbiome in patients with difficult to treat atopic dermatitis. Clin. Exp. Allergy 49, 1437–1445. doi: 10.1111/cea.13492
van Zuuren, E. J., Fedorowicz, Z., Christensen, R., Lavrijsen, A., Arents, B. W. M. (2017). Emollients and moisturisers for eczema. Cochrane Database Syst. Rev. 2, CD012119. doi: 10.1002/14651858.CD012119.pub2
Vu, A. T., Baba, T., Chen, X., Le, T. A., Kinoshita, H., Xie, Y., et al. (2010). Staphylococcus aureus membrane and diacylated lipopeptide induce thymic stromal lymphopoietin in keratinocytes through the Toll-like receptor 2-Toll-like receptor 6 pathway. J. Allergy Clin. Immunol. 126, 985–993. doi: 10.1016/j.jaci.2010.09.002
Weidinger, S., Beck, L. A., Bieber, T., Kabashima, K., Irvine, A. D. (2018). Atopic dermatitis. Nat. Rev. Dis. Primers 4, 1. doi: 10.1038/s41572-018-0001-z
Weidinger, S., Novak, N. (2016). Atopic dermatitis. Lancet 387, 1109–1122. doi: 10.1016/S0140-6736(15)00149-X
Weiss, A., Delavenne, E., Matias, C., Lagler, H., Simon, D., Li, P., et al. (2022). Topical niclosamide (ATx201) reduces Staphylococcus aureus colonization and increases Shannon diversity of the skin microbiome in atopic dermatitis patients in a randomized, double-blind, placebo-controlled Phase 2 trial. Clin. Transl. Med. 12, e790. doi: 10.1002/ctm2.790
Whiting, C., Abdel Azim, S., Friedman, A. (2024). The skin microbiome and its significance for dermatologists. Am. J. Clin. Dermatol. 25, 169–177. doi: 10.1007/s40257-023-00842-z
Williams, M. R., Bagood, M. D., Enroth, T. J., Bunch, Z. L., Jiang, N., Liu, E., et al. (2023). Staphylococcus epidermidis activates keratinocyte cytokine expression and promotes skin inflammation through the production of phenol-soluble modulins. Cell Rep. 42, 113024. doi: 10.1016/j.celrep.2023.113024
Williams, M. R., Costa, S. K., Zaramela, L. S., Khalil, S., Todd, D. A., Winter, H. L., et al. (2019). Quorum sensing between bacterial species on the skin protects against epidermal injury in atopic dermatitis. Sci. Transl. Med. 11, eaat8329. doi: 10.1126/scitranslmed.aat8329
Williams, M. R., Gallo, R. L. (2015). The role of the skin microbiome in atopic dermatitis. Curr. Allergy Asthma Rep. 15, 65. doi: 10.1007/s11882-015-0567-4
Williams, M. R., Nakatsuji, T., Sanford, J. A., Vrbanac, A. F., Gallo, R. L. (2017). Staphylococcus aureus induces increased serine protease activity in keratinocytes. J. Invest. Dermatol. 137, 377–384. doi: 10.1016/j.jid.2016.10.008
Wollenberg, A., Christen-Zäch, S., Taieb, A., Paul, C., Thyssen, J. P., de Bruin-Weller, M., et al. (2020). ETFAD/EADV Eczema task force 2020 position paper on diagnosis and treatment of atopic dermatitis in adults and children. J. Eur. Acad. Dermatol. Venereol. 34, 2717–2744. doi: 10.1111/jdv.16892
Wollenberg, A., Oranje, A., Deleuran, M., Simon, D., Szalai, Z., Kunz, B., et al. (2016). ETFAD/EADV Eczema task force 2015 position paper on diagnosis and treatment of atopic dermatitis in adult and paediatric patients. J. Eur. Acad. Dermatol. Venereol. 30, 729–747. doi: 10.1111/jdv.13599
Wongpiyabovorn, J., Soonthornchai, W., Wilantho, A., Palasuk, M., Payungporn, S., Sodsai, P., et al. (2019). Effect of tacrolimus on skin microbiome in atopic dermatitis. Allergy 74, 1400–1406. doi: 10.1111/all.13743
Wrześniewska, M., Wołoszczak, J., Świrkosz, G., Szyller, H., Gomułka, K. (2024). The role of the microbiota in the pathogenesis and treatment of atopic dermatitis-A literature review. Int. J. Mol. Sci. 25, 6539. doi: 10.3390/ijms25126539
Yin, H., Qiu, Z., Zhu, R., Wang, S., Gu, C., Yao, X., et al. (2023). Dysregulated lipidome of sebum in patients with atopic dermatitis. Allergy 78, 1524–1537. doi: 10.1111/all.15569
Yu, J., Luo, Y., Zhu, Z., Zhou, Y., Sun, L., Gao, J., et al. (2019). A tryptophan metabolite of the skin microbiota attenuates inflammation in patients with atopic dermatitis through the aryl hydrocarbon receptor. J. Allergy Clin. Immunol. 143, 2108–2119. doi: 10.1016/j.jaci.2018.11.036
Yue, H., Umehara, Y., Trujillo-Paez, J. V., Peng, G., Nguyen, H. L. T., Chieosilapatham, P., et al. (2021). Exogenous factors in the pathogenesis of atopic dermatitis: Irritants and cutaneous infections. Clin. Exp. Allergy 51, 382–392. doi: 10.1111/cea.13820
Zeng, J., Dou, J., Gao, L., Xiang, Y., Huang, J., Ding, S., et al. (2020). Topical ozone therapy restores microbiome diversity in atopic dermatitis. Int. Immunopharmacol. 80, 106191. doi: 10.1016/j.intimp.2020.106191
Zeng, J., Lu, J. (2018). Mechanisms of action involved in ozone-therapy in skin diseases. Int. Immunopharmacol. 56, 235–241. doi: 10.1016/j.intimp.2018.01.040
Zhao, Y., Wu, L., Lu, Q., Gao, X., Zhu, X., Yao, X., et al. (2022). The efficacy and safety of dupilumab in Chinese patients with moderate-to-severe atopic dermatitis: a randomized, double-blind, placebo-controlled study. Br. J. Dermatol. 186, 633–641. doi: 10.1111/bjd.20690
Zheng, Y., Hunt, R. L., Villaruz, A. E., Fisher, E. L., Liu, R., Liu, Q., et al. (2022). Commensal Staphylococcus epidermidis contributes to skin barrier homeostasis by generating protective ceramides. Cell Host Microbe 30, 301–313. doi: 10.1016/j.chom.2022.01.004
Keywords: atopic dermatitis, skin microbiota, Staphylococcus aureus, pathogenesis, microbiota-based therapy
Citation: Huang C, Zhuo F, Guo Y, Wang S, Zhang K, Li X, Dai W, Dou X and Yu B (2025) Skin microbiota: pathogenic roles and implications in atopic dermatitis. Front. Cell. Infect. Microbiol. 14:1518811. doi: 10.3389/fcimb.2024.1518811
Received: 30 October 2024; Accepted: 27 December 2024;
Published: 14 January 2025.
Edited by:
Lucas De Paula Ramos, Université Claude Bernard Lyon 1, FranceReviewed by:
Tomoko Hanawa, Kyorin University, JapanCopyright © 2025 Huang, Zhuo, Guo, Wang, Zhang, Li, Dai, Dou and Yu. This is an open-access article distributed under the terms of the Creative Commons Attribution License (CC BY). The use, distribution or reproduction in other forums is permitted, provided the original author(s) and the copyright owner(s) are credited and that the original publication in this journal is cited, in accordance with accepted academic practice. No use, distribution or reproduction is permitted which does not comply with these terms.
*Correspondence: Xia Dou, ZHJkb3V4aWFAMTYzLmNvbQ==; Bo Yu, ZHJib3l1X2Rlcm1AMTI2LmNvbQ==
†These authors share first authorship
Disclaimer: All claims expressed in this article are solely those of the authors and do not necessarily represent those of their affiliated organizations, or those of the publisher, the editors and the reviewers. Any product that may be evaluated in this article or claim that may be made by its manufacturer is not guaranteed or endorsed by the publisher.
Research integrity at Frontiers
Learn more about the work of our research integrity team to safeguard the quality of each article we publish.