- 1School of Biological Sciences & Engineering, Yachay Tech University, San Miguel de Urcuquí, Ecuador
- 2Centro Universitário ENIAC, São Paulo, Brazil
- 3INTI International University, Persiaran Perdana BBN, Nilai, Negeri Sembilan, Malaysia
- 4Department of Microbiology and Immunology, Wake Forest School of Medicine, Winston Salem, NC, United States
- 5Dogs & Cats Hospital Veterinario, Ibarra, Imbabura, Ecuador
- 6One Health Brazilian Resistance Project (OneBR), São Paulo, Brazil
- 7Department of Microbiology, Institute of Biomedical Sciences, University of São Paulo, São Paulo, Brazil
- 8Department of Clinical Analysis, School of Pharmacy, University of São Paulo, São Paulo, Brazil
- 9Federal Institute of Espírito Santo, Vila Velha, Brazil
From a One Health perspective, dogs and cats have begun to be recognized as important reservoirs for clinically significant multidrug-resistant bacterial pathogens. In this study, we investigated the occurrence and genomic features of ESβL producing Enterobacterales isolated from dogs, in the province of Imbabura, Ecuador. We identified four isolates expressing ESβLs from healthy and diseased animals. In this regard, two Escherichia coli strains producing CTX-M-55-like or CTX-M-65 ESβLs belonged to the international ST10 and ST162, whereas two Klebsiella pneumoniae producing CTX-M-14 or CTX-M-27 belonged to ST35 and ST661. Phylogenomic analysis clustered (95-105 SNP differences) CTX-M-55/ST10 E. coli from companion animal with food and human E. coli strains of ST10 isolated in 2016, in Australia and Cambodia, respectively; whereas CTX-M-27-positive K. pneumoniae ST661 was clustered (201-216 SNP differences) with human strains identified in Italy, in 2013 and 2017, respectively. In summary, we report the presence and genomic data of global human-associated clones of CTX-M-producing E. coli and K. pneumoniae in dogs, in Ecuador. The implementation of a national epidemiological surveillance program is necessary to establish future strategies to control the dissemination of antibiotic-resistant priority pathogens using a One Health approach.
1 Introduction
Although Enterobacterales are natural inhabitants of the intestinal tract of mammals, some genus and species can cause infections of the respiratory and urinary systems, skin, ear, and soft tissue of human and non-human hosts (Zogg et al., 2018). In this regard, Escherichia coli and Klebsiella pneumoniae are leading causes of healthcare-associated infections worldwide (Pesesky et al., 2015), with carbapenem- and broad-spectrum cephalosporin-resistant lineages being categorized as critical priority pathogens by the World Health Organization (Tacconelli et al., 2018). Certainly, and even more worrying is the fact that extended-spectrum β-lactamase (ESβL)-producing strains are no longer restricted to hospital locations but also represents a serious problem involving pets, wildlife, and environmental and food safety (Lopes et al., 2021; Salgado-Caxito et al., 2021).
CTX-M enzymes have become the most prevalent type of ESβLs globally (Cantón & Coque, 2006; Pitout and Laupland, 2008). It is remarkable that the first report on the emergence of a CTX-M enzyme was in 1988, from a laboratory dog used in β-lactams research in Japan (Matsumoto et al., 1988), whereas E. coli producing blaCTX-M-1-type enzyme was first described in a healthy dog in Portugal. Since then, a significant occurrence of CTX-M-type ESβL-producing Enterobacterales has been documented in healthy and diseased dogs and cats from Asian, European and South American countries (Salgado-Caxito et al., 2021).
From a public health perspective, the rapid appearance of resistant bacterial populations among dogs and cats, and the close contact between household pets and people have favored the transmission of antibiotic-resistant bacteria from companion animals to humans (Damborg et al., 2016; Kawamura et al., 2017; Salgado-Caxito et al., 2021; Sellera et al., 2021). Transfer of resistant bacteria between humans and their dogs has been well documented (Albrechtova et al., 2012), as was illustrated by the identification of the same E. coli clone from a urinary tract infection in a dog, and from its household members (Johnson et al., 2008), although the direction of transfer is often difficult to prove (Pomba et al., 2017). In addition, the intensive use of antimicrobials in animals can be an important factor in the development of antimicrobial-resistant microorganisms (Caprioli et al., 2000; Umber and Bender, 2009; Marshall and Levy, 2011; Seiffert et al., 2013; Samanta et al., 2015). In this sense, companion animals might act as source of human contamination but may also be contaminated by human bacteria (Okubo et al., 2014; Fernandes et al., 2018; Melo et al., 2018). Furthermore, the role of companion animals as a source of AMR has, so far, been neglected (Ewers et al., 2012).
In South America, multidrug-resistant Enterobacterales are a major concern as the region exhibits some of the higher rates of antimicrobial resistance worldwide (Bonelli et al., 2014). The first report of ESβL in this region in companion animals was published in 2008 from E. coli isolates obtained from fecal samples of dogs and cats in Chile (Moreno et al., 2008). In that context, nosocomial infections caused by ESβL producing Enterobacterales have increased in the region more than others, since 2005 (Guzmán-Blanco et al., 2014). Several factors such living in crowded conditions, malnutrition, ineffective healthcare systems, deficient drug supply chain, massive use of antimicrobials in livestock and agriculture linked to lack of financial resources might be related to the greater prevalence of ESβLs in countries with lower economic resources (Villegas et al., 2008). In this study, we report the occurrence and genomic data of ESβL-producing E. coli and K. pneumoniae strains in dogs from Imbabura, Ecuador.
2 Materials and methods
2.1 Bacterial isolates and antibiotic susceptibility profile
During a microbiological and genomic surveillance study carried out in 2018, a total of 125 rectal swabs from dogs (64 healthy animals and 61 sick animals) were collected from the province of Imbabura in Ecuador, in order to monitor the presence of clinically significant drug-resistant Gram-negative bacteria in companion animals (Supplementary Table 1). Samples were collected between April and June and between October and December 2018; from a veterinary clinic located in Ibarra that attend the following counties in Imbabura: Antonio Ante, Cotacachi, Ibarra, Otavalo, Pimampiro, and San Miguel de Urcuquí (Figure S1).
The samples were cultured on blood and MacConkey agar plates supplemented with ceftriaxone (2 µg/mL) being incubated at 37°C overnight (Jacob et al., 2020). Bacteria were identified by conventional biochemical tests, whereas antimicrobial susceptibility testing was performed by the disk diffusion method on Mueller–Hinton agar plates (Clinical and Laboratory Standards Institute, 2023a; Clinical and Laboratory Standards Institute, 2023b). In addition, human and veterinary antibiotics including amoxicillin-clavulanic acid, ceftazidime, cefotaxime, ceftriaxone, ceftiofur, cefepime, cefoxitin, aztreonam, ertapenem, meropenem, imipenem, nalidixic acid, enrofloxacin, ciprofloxacin, trimethoprim/sulfamethoxazole, gentamicin, amikacin, and chloramphenicol, were tested (Supplementary Table 2). Additionally, minimum inhibitory concentration (MIC) of cefotaxime was determined by using ETEST® strips (bioMérieux). The results were interpreted according to Clinical and Laboratory Standards Institute (Clinical and Laboratory Standards Institute, 2023a; Clinical and Laboratory Standards Institute, 2023b). ESβL production was screened by the double disk synergy test (DDST) (Jarlier et al., 1988).
2.2 Whole genome sequencing analysis
Whole genomic DNA was extracted (PureLinkTM; Invitrogen) and used to prepare a library that was sequenced using the NextSeq550 platform (2 x 75-bp paired-end) (Illumina), and the de novo assembly method was the Unicycler v.0.4.8 with Phred20 as minimum score quality of reads. The contigs generated for all genomes were submitted to NCBI using the WGS submission and automatic annotation was performed by PGAP (Prokaryotic Genome Annotation Pipeline v.3.2.); CDSs, RNAs and pseudo genes are shown in Tables 1, 2. The genomes were analyzed by MLST 2.0, ResFinder 4.1, and PlasmidFinder 2.1 tools from the Center for Genomic Epidemiology (CGE). Additionally, antibiotic resistance and virulence genes were predicted using the Comprehensive Antibiotic Resistance Database (CARD) and the Virulence Factor Database (VFDB), respectively, whereas genes related with mercury, arsenic and disinfectant resistance (quaternary ammonium compounds) were screened using an in-house and the BIGSdb database. For phylotyping E. coli, the in silico Clermont phylotyper tool was used (https://ezclermont.hutton.ac.uk/).
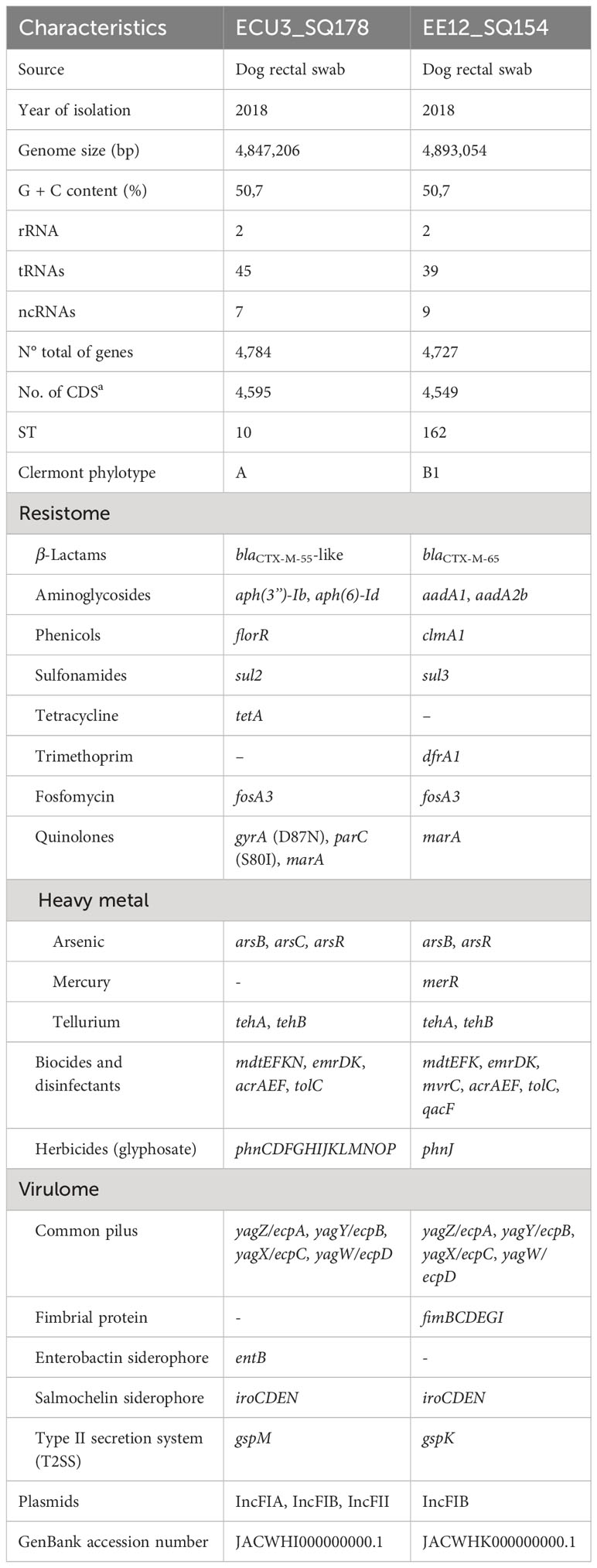
Table 1 Genomic characteristics of lineages of ESBL-producing Escherichia coli strains recovered from rectal swabs collected in dogs in Ecuador.
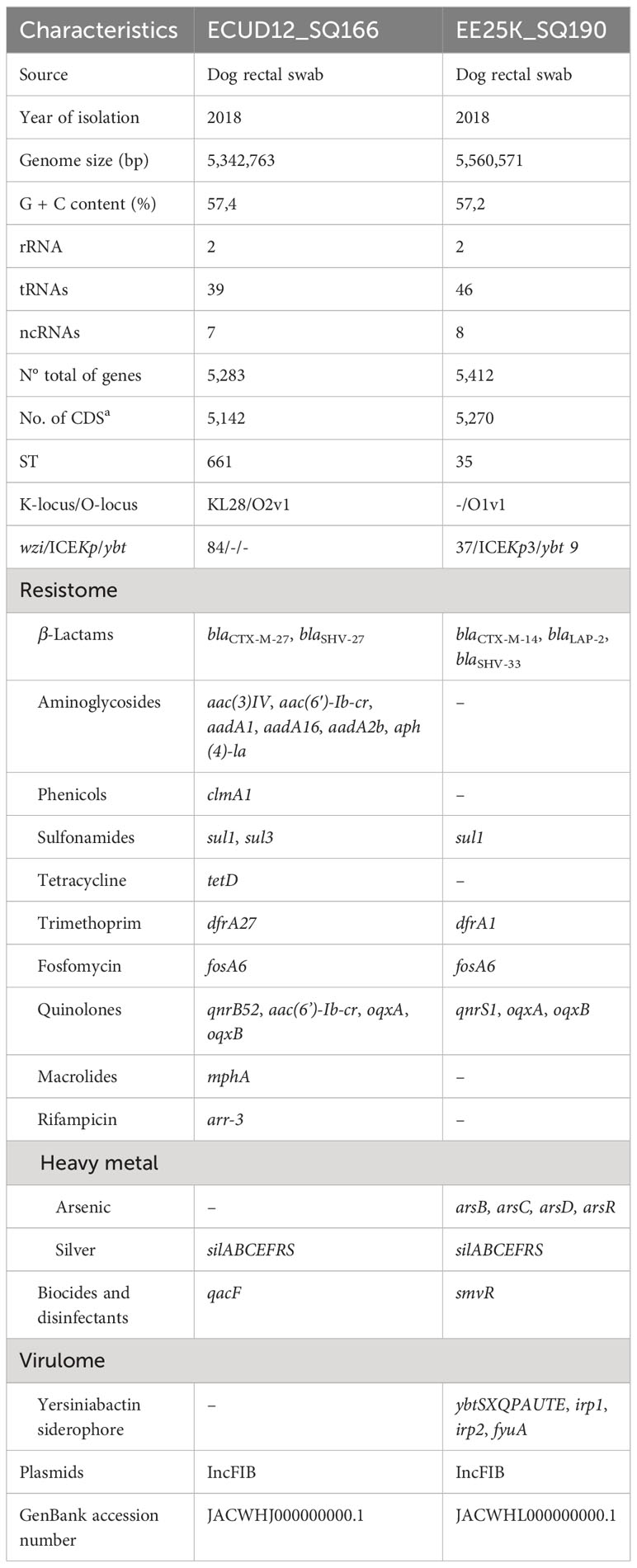
Table 2 Genomic characteristics of lineages of ESBL-producing Klebsiella pneumoniae strains recovered from rectal swabs collected in dogs in Ecuador.
2.3 Phylogenetic analysis
A search for genomic data of isolates for each sequence type identified was performed, in order to recruit genomes for phylogenetic comparison. Assemblies with no metadata for country, year and source of isolation were ignored. For E. coli strains, genomes were downloaded from Enterobase (3,572 assemblies of E. coli ST10 and 442 assemblies of ST162), while for K. pneumoniae strains, a search for each ST were performed on bacWGSTdb (http://bacdb.cn/BacWGSTdb/), and genomes were downloaded from NCBI GenBank (i.e., 60 assemblies of K. pneumoniae ST35 and 19 assemblies of ST661). With exception of ST661, which had only 19 assemblies downloaded, 30 genomes with highest average nucleotide identity (ANI) of each ST comparing with this work’s assemblies were performed using FastANIv1.32 (https://github.com/ParBLiSS/FastANI/). ANI values between downloaded and query genomes were ≥99.7625% for E. coli ST10, ≥99.7807% for E. coli ST162, ≥99.7631% for K. pneumoniae ST35 and ≥99.575% for K. pneumoniae ST661. CSI Phylogeny (https://cge.food.dtu.dk/services/CSIPhylogeny/) was used with default settings to generate approximate maximum-likelihood SNP-based trees. Chromosome sequences of SCU-118 (NZ_CP051716.1) and LD91-1 (NZ_CP042585.1) E. coli strains, and RJY9645 (NZ_CP041353.1) and F13 (NZ_CP026162.1) of K. pneumoniae strains were used as reference for E. coli ST10 and ST162, and K. pneumoniae ST35 and ST661, respectively. ABRicatev1.0.1 (https://github.com/tseemann/abricate) was used with ResFinder and PlasmidFinder databases to screen antimicrobial resistance genes and plasmids on each recruited genome. Identity and coverage limits were set to 98% and 100%, respectively. iTOLv6 (https://itol.embl.de/) was used to annotate the tree with data from Enterobase, bacWGSTdb and ABRicate.
3 Results and discussion
Forty-tree cephalosporin-resistant Gram-negative bacteria were isolated from 23 healthy dogs and 16 sick dogs (Supplementary Tables 1, 2). From the latter, eight dogs presented with gastrointestinal complications, four with metabolic syndrome, two with dermatological disease, one with respiratory problems, and another with cerebrovascular accident. Based on confirmation of ESβL phenotype, four bacterial isolates exhibiting a MDR profile (Magiorakos et al., 2012) were sequenced: i) E. coli strain ECU3_SQ178 (GenBank accession number: JACWHI000000000.1) isolated from a 6-month-old healthy female dog mixed breed, with no previous treatments reported. This strain presented resistance to ceftazidime, cefotaxime (MIC > 32 µg/mL), ceftriaxone, cefepime, aztreonam, nalidixic-acid, enrofloxacin, ciprofloxacin, and chloramphenicol, being susceptible to amoxicillin-clavulanic acid, cefoxitin, ertapenem, meropenem, imipenem, gentamicin, amikacin, trimethoprim-sulfamethoxazole (Supplementary Table 2). In this regard, WGS analysis predicted the presence of genes associated with resistance to β-lactams (blaCTX-M-55-like), phenicols (floR), tetracyclines (tetA), sulphonamides (sul2), aminoglycosides [aph(3”)-Ib, aph(6)-Id], fosfomycin (fosA3), and quinolones (gyrA-D87N and parC-S80I point mutations, marA). On the other hand, genes conferring tolerance to heavy metals [arsenic (arsBCR) and tellurium (tehAB)], herbicide [glyphosate (phnCDFGHIJKLMNOP)], biocides and disinfectants (mdtEFKN, emrDK, acrAEF and tolC) were also predicted (Table 1); ii) E. coli strain EE12_SQ154 (GenBank accession number: JACWHK000000000.1) isolated from a 4-years-old female Yorkshire terrier dog with a history of physical decline, cerebrovascular accident and shock. It was not reported by the private veterinary clinic the treatment received prior to the sample collection. Antimicrobial susceptibility testing revealed resistance to ceftazidime, cefotaxime (MIC > 32 µg/mL), ceftriaxone, cefepime, aztreonam, nalidixic-acid, enrofloxacin, ciprofloxacin, trimethoprim-sulfamethoxazole, chloramphenicol, and gentamicin, and susceptibility to amoxicillin-clavulanic acid, cefoxitin, ertapenem, meropenem, imipenem and amikacin (Supplementary Table 2). The antimicrobial resistome included genes conferring resistance to β-lactams (blaCTX-M-65), aminoglycosides (aadA1, aadA2b), fosfomycin (fosA3), phenicol (cmlA1), sulphonamides (sul3), trimethoprim (dfrA1), quinolones (marA), heavy metals [arsenic (arsBR), tellurium (tehAB) and mercury (merR)], herbicide [glyphosate (phnJ)], biocides and disinfectants (mdtEFK, emrDK, acrAEF, tolC, qacF and mvrC) (Table 1); iii) K. pneumoniae strain ECU12_SQ166 (GenBank accession number: JACWHJ000000000.1), isolated from a 12-year-old male English Shepherd dog admitted to a private veterinary clinic with signs of diarrhea, melena, vomiting, septicemia, and chronic kidney failure leading to death. Based on the anamnesis and initial physical examination, fluid therapy was established, as a stabilization measure (lactated ringer solution), and a not specified β-lactam antibiotic was administered. The strain exhibited resistance to amoxicillin-clavulanic acid, ceftazidime, cefotaxime (MIC > 32 µg/mL), ceftriaxone, cefepime, aztreonam, ceftiofur, trimethoprim-sulfamethoxazole, nalidixic-acid, enrofloxacin, ciprofloxacin, and gentamicin, being susceptible to cefoxitin, ertapenem, meropenem, imipenem, and amikacin (Supplementary Table 2). The resistome analysis predicted resistance genes to β-lactams (blaCTX-M-27, blaSHV-27), fosfomycin (fosA6), trimethoprim (dfrA27), rifampicin (arr-3), sulfonamides (sul1, sul3), aminoglycosides [aac(3)IV, aac(6′)-Ib-cr, aadA1, aadA16, aadA2b, aph(4)-la], macrolides (mphA), quinolones [aac(6’)-Ib-cr, oqxA, oqxB, qnrB52], phenicols (cmlA), tetracyclines (tetD), silver (silABCEFRS) and ammonium quaternary compounds (qacF) (Table 2); (iv) K. pneumoniae strain EE25K_SQ190 (GenBank accession number: JACWHL000000000.1) isolated from an 8-year-old male German shepherd dog, presenting with discomfort, anorexia and foreign body gingivitis. After clinical examination, the foreign body was removed and a combination of amoxicillin/clavulanic acid plus a non-steroidal anti-inflammatory was prescribed. Antimicrobial susceptibility testing revealed resistance to cefotaxime (MIC > 32 µg/mL), ceftriaxone, cefepime, nalidixic-acid, enrofloxacin, ciprofloxacin, and trimethoprim-sulfamethoxazole. This strain showed to be susceptible to amoxicillin-clavulanic acid, ceftazidime, cefoxitin, aztreonam, ertapenem, meropenem, imipenem, amikacin and gentamicin (Supplementary Table 2). Resistome encompass genes resistant to β-lactams (blaCTX-M-14, blaSHV-33, blaLAP2), fosfomycin (fosA6), trimethoprim (dfrA1), quinolones (oqxA, oqxB, qnrS1), and sulphonamides (sul1), silver (silABCEFRS), arsenic (arsBCDR) and chlorhexidine (smvR) (Table 2).
While CTX-M-55- and CTX-M-65-positive E. coli strains belonged to ST10 and ST162, K. pneumoniae producing CTX-M-27 and CTX-M-14 ESβLs belonged to ST661 and ST35, respectively. E. coli ST10 and ST162 have been previously associated with human infections (Coelho et al., 2011; Chen et al., 2014), being further identified in hospital sewage (Zhao et al., 2017), bovines (Umpiérrez et al., 2017), birds (Fuentes-Castillo et al., 2020), and dogs (Yasugi et al., 2021). The blaCTX-M-55 gene has been widely identified globally in E. coli isolates from various animal species (Kiratisin et al., 2007; Zhang et al., 2014; Birgy et al., 2018; Lupo et al., 2018). The remarkable prevalence of this gene, accompanied by a high propensity for horizontal gene transfer has facilitated its rapid and wide spread (Yang et al., 2023). In Ecuador blaCTX-M-55 has been the most prevalent allele of the blaCTX-M family in E. coli from poultry settings, followed by blaCTX-M-65 and blaCTX-M-2 (Ortega-Paredes et al., 2020a). On the other hand, according to Enterobase (https://enterobase.warwick.ac.uk/), ST10 has been identified in dogs from Germany, United States of America (USA), United Kingdom, South Korea, Canada and New Zealand, whereas in Ecuador ST10 has been identified in humans, wild animals, and environmental samples; confirming the One Health importance of this global lineage in this country. In fact, phylogenomic analysis showed that strain ECU3_SQ178 (CTX-M-55/ST10) clustered (95-105 SNP differences) with food and human E. coli strains of ST10 isolated in 2016, in Australia and Cambodia, respectively, whereas CTX-M-65-positive E. coli ST162 (strain EE12_SQ154) showed ubiquity, being clustered (207-265 SNP differences) with other four drug-resistant E. coli strains of ST162 isolated from livestock (USA, 2016), poultry (USA, 2020), human (Australia, 2014) and companion animal (USA, 2007) (Figure 1, Supplementary Table 3). Moreover, data retrieved from Enterobase confirm occurrence of this E. coli clone in companion animals from Germany, USA, and Canada. Interestingly, this is the first report of E. coli ST162 found in companion animal, in South America.
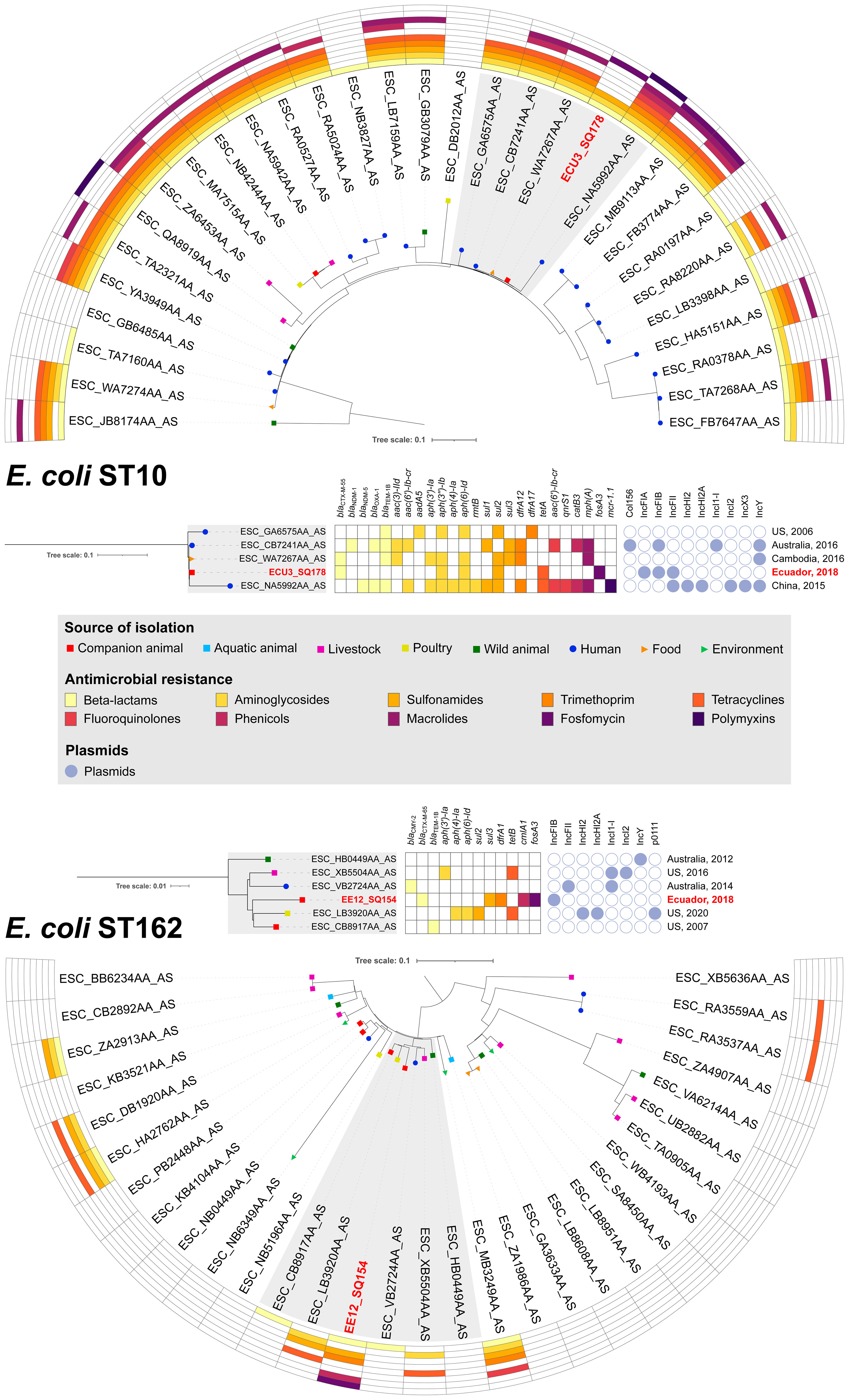
Figure 1 Phylogenetic trees of E. coli strains. SNP-based phylogenetic trees of E. coli ST10 and ST162, and heatmap showing presence/absence of antibiotic resistance genes for 10 antibiotics, and their source of isolation. Details on resistome, plasmidome and origin are showed for clusters formed with CTX-M-55-producing E. coli ECU3_SQ178 and CTX-M-65 E. coli EE12_SQ154 strains, isolated from dogs, in Ecuador.
In the case of K. pneumoniae ST661 and ST35 clones, they have been previously isolated from nosocomial pneumonia in humans (Zhao et al., 2019), rectal swabs from pigs and fecal human samples (Leangapichart et al., 2021). Moreover, ST661 has been recovered from aquatic environments (Furlan et al., 2020), hospitalized patients (Piazza et al., 2019), being recently reported as responsible for outbreaks in Europe (Martin et al., 2017); whereas ST35 has been identified among ESβL-producing K. pneumoniae strains in hospital settings (Marcade et al., 2013; Frenk et al., 2020), being lately recognized as a multidrug-resistant clone with worldwide distribution (Shen et al., 2020).
For CTX-M-27-positive K. pneumoniae ST661 (ECU12_SQ166), phylogenomic analysis revealed relationship (201-216 SNP differences) with human strains identified in Italy, in 2013 and 2017, respectively (Figure 2, Supplementary Table 3). Strikingly, all the three isolates within the clade carried an IncFIB-type plasmid. Moreover, ECU12_SQ166 and the human strain isolated in 2017 exhibited an identical MDR profile, sharing blaSHV-27, sul1 and mph(A) resistance genes. In brief, K. pneumoniae ST661 is other global clone identified in Italy, China, England, Brazil, Tunisia, Thailand, Uruguay, Mexico and Taiwan (Yan et al., 2015; Ku et al., 2017; Martin et al., 2017; Patil et al., 2019; Piazza et al., 2019; Sghaier et al., 2019; Furlan et al., 2020; Hassen et al., 2020; Ludden et al., 2020; Leangapichart et al., 2021; Papa-Ezdra et al., 2021; Toledano-Tableros et al., 2021).
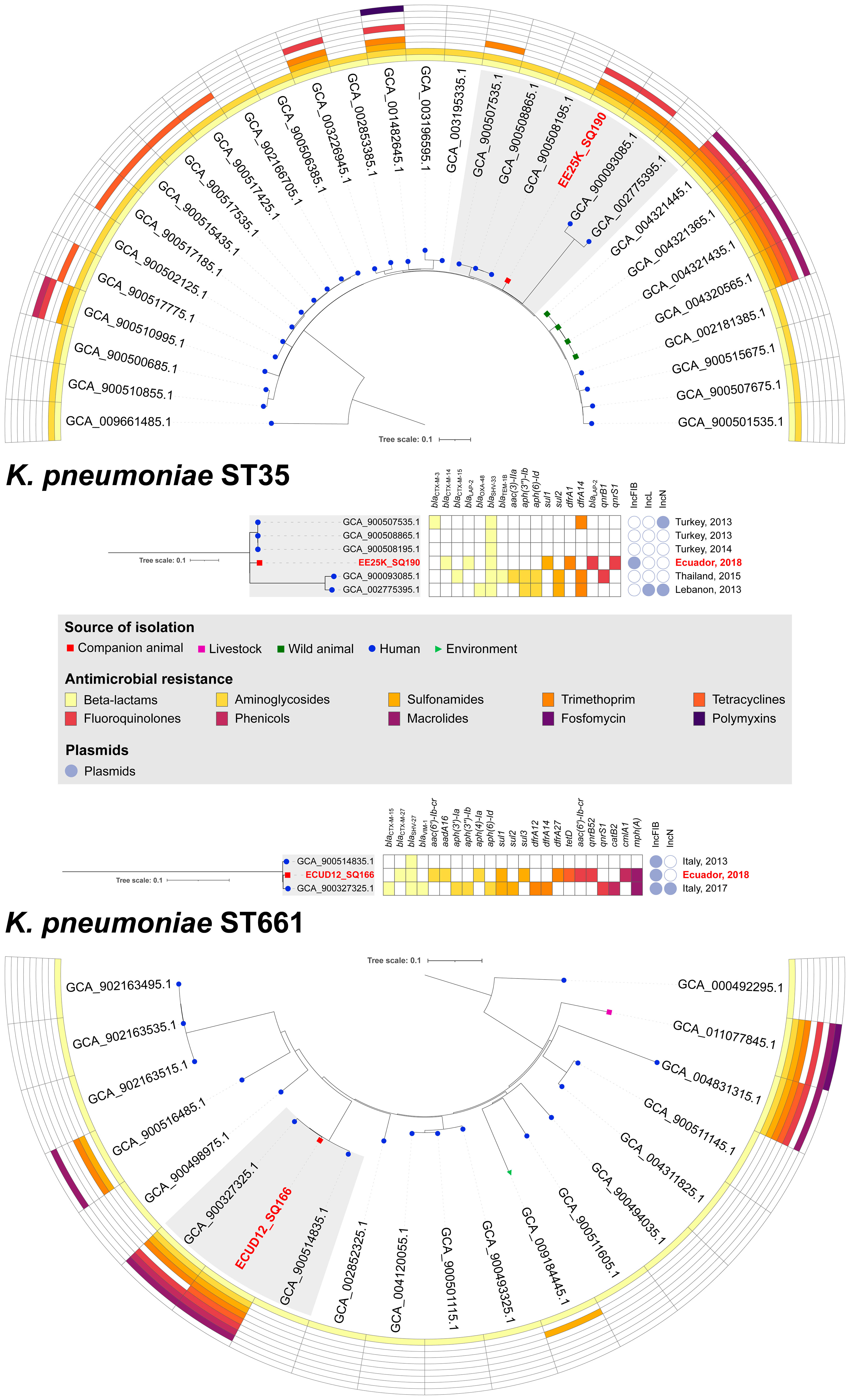
Figure 2 Phylogenetic trees of K. pneumoniae strains. SNP-based phylogenetic trees of K. pneumoniae ST35 and ST661, and heatmap showing presence/absence of antibiotic resistance genes for 10 antibiotics, and their source of isolation. Details on resistome, plasmidome and origin are showed for clusters formed with CTX-M-14-producing K. pneumoniae EE25K_SQ190 and CTX-M-27-producing K. pneumoniae ECU12_SQ166 strains, isolated from dogs in Ecuador.
In companion animals, ESβL production among Klebsiella isolates has been associated with CTX-M-14 and CTX-M-15 variants (Harada et al., 2016). In this study, CTX-M-14-positive K. pneumoniae EE25K_SQ190 belonged to ST35. Although this clone has been previously identified in China, Romania, Yemen, Israel, France, Spain and Thailand (Marcade et al., 2013; Cubero et al., 2016; Alsharapy et al., 2020; Frenk et al., 2020; Kong et al., 2020; Shen et al., 2020; Surleac et al., 2020; Zhong et al., 2020; Leangapichart et al., 2021), phylogenomic analysis clustered (353-354 SNP differences) EE25K_SQ190 with a human clone identified in Turkey in 2013 and 2014 (Figure 2, Supplementary Table 3).
Although, in Ecuador, occurrence of E. coli producing ESβL has been reported in pets, chicken, humans, food, vegetables, broiler farms, and river water samples, in Quito (Vinueza-Burgos et al., 2016; Chiluisa-Guacho et al., 2018; Ortega-Paredes et al., 2018; Ortega-Paredes et al., 2019; Vinueza-Burgos et al., 2019; Zurita et al., 2019; Ortega-Paredes et al., 2020a; Ortega-Paredes et al., 2020b; Zurita et al., 2020), and in other cities such as Guayaquil (Soria Segarra et al., 2018), Esmeraldas (Hedman et al., 2019), Loja (Delgado et al., 2016), and Cuenca (Zurita et al., 2013); as well as in the provinces of Tungurahua and Cotopaxi (Sánchez-Salazar et al., 2020), genomic data are scarce. Specifically, while CTX-M-55 and CTX-M-65-producing E. coli have been previously reported in dogs in central Ecuador, and in Quito (Ortega-Paredes et al., 2019; Albán et al., 2020; Salinas et al., 2021), CTX-M-producing K. pneumoniae have been isolated from human hosts in Cuenca (Nordberg et al., 2013), Quito, Guayaquil, and Azogues (Zurita et al., 2013), so far.
In summary, we report genomic data of global One Health-associated clones of CTX-M-55 and CTX-M-65-producing E. coli, and CTX-M-14 and CTX-M-15-producing K. pneumoniae in dogs from the province of Imbabura, in Ecuador. The implementation of a national epidemiological surveillance program is necessary to establish future strategies to control the dissemination of antibiotic-resistant priority pathogens using a One Health approach.
Data availability statement
The datasets presented in this study can be found in online repositories. The names of the repository/repositories and accession number(s) can be found in the article/supplementary material.
Ethics statement
The animal studies were approved by MSc. Elena Dorothea Balarezo Cisneros President of the Ethics Committee for Research Processes Yachay Tech University. The studies were conducted in accordance with the local legislation and institutional requirements. Written informed consent was obtained from the owners for the participation of their animals in this study.
Author contributions
FAG-Z: Conceptualization, Formal analysis, Project administration, Supervision, Writing – original draft, Writing – review & editing. JT: Formal analysis, Writing – review & editing. JV-B: Formal analysis, Methodology, Writing – original draft, Writing – review & editing. MV-B: Investigation, Methodology, Writing – review & editing. AC-A: Methodology, Writing – review & editing. FE: Formal analysis, Methodology, Software, Writing – review & editing. QM: Formal analysis, Methodology, Writing – review & editing. BF: Methodology, Writing – review & editing. ES: Formal analysis, Methodology, Software, Writing – review & editing. JGMP: Formal analysis, Methodology, Validation, Writing – review & editing. MJ: Investigation, Writing – review & editing. NL: Conceptualization, resources, Formal analysis, Writing – original draft, Writing – review & editing.
Funding
The author(s) declare financial support was received for the research, authorship, and/or publication of this article. This study was supported by the Fundação de Amparo à Pesquisa do Estado de São Paulo (FAPESP, 2020/08224-9 and 2019/15778-4) and Conselho Nacional de Desenvolvimento Científico e Tecnológico (CNPq 422984/2021-3 and 314336/2021-4). NL is a research fellow of CNPq (314336/2021-4). FE was a research fellow of FAPESP (2019/15778-4).
Acknowledgments
We are grateful to FAPESP and CNPq. We also thank Cefar Diagnóstica Ltda. (São Paulo, Brazil) and CEFAP-GENIAL facility for kindly supplying antibiotic discs for susceptibility testing and Illumina sequencing, respectively.
Conflict of interest
The authors declare that the research was conducted in the absence of any commercial or financial relationships that could be construed as a potential conflict of interest.
Publisher’s note
All claims expressed in this article are solely those of the authors and do not necessarily represent those of their affiliated organizations, or those of the publisher, the editors and the reviewers. Any product that may be evaluated in this article, or claim that may be made by its manufacturer, is not guaranteed or endorsed by the publisher.
Supplementary material
The Supplementary Material for this article can be found online at: https://www.frontiersin.org/articles/10.3389/fcimb.2023.1259764/full#supplementary-material
References
Albán, M. V., Núñez, E. J., Zurita, J., Villacís, J. E., Tamayo, R., Sevillano, G., et al. (2020). Canines with different pathologies as carriers of diverse lineages of Escherichia coli harbouring mcr-1 and clinically relevant β-lactamases in central Ecuador. J. Glob. Antimicrob. Resist. 22, 182–183. doi: 10.1016/j.jgar.2020.05.017
Albrechtova, K., Dolejska, M., Cizek, A., Tausova, D., Klimes, J., Bebora, L., et al. (2012). Dogs of nomadic pastoralists in Northern Kenya are reservoirs of plasmid-mediated cephalosporin-and quinolone-resistant Escherichia coli, including pandemic clone B2-O25-ST131. Antimicrob. Agents Chemother. 56, 4013–4017. doi: 10.1128/AAC.05859-11
Alsharapy, S. A., Gharout-Sait, A., Muggeo, A., Guillard, T., Cholley, P., Brasme, L., et al. (2020). Characterization of carbapenem-resistant Enterobacteriaceae clinical isolates in Al Thawra University Hospital, Sana’a, Yemen. Microb. Drug Resist. 26, 211–217. doi: 10.1089/mdr.2018.0443
Birgy, A., Madhi, F., Hogan, J., Doit, C., Gaschignard, J., Caseris, M., et al. (2018). CTX-M-55-, MCR-1-, and FosA-producing multidrug-resistant Escherichia coli infection in a child in France. Antimicrob. Agents Chemother. 62, e00127–e00118. doi: 10.1128/aac.00127-18
Bonelli, R. R., Moreira, B. M., Picão, R. C. (2014). Antimicrobial resistance among Enterobacteriaceae in South America: history, current dissemination status and associated socioeconomic factors. Drug Resist. Updat. 17, 24–36. doi: 10.1016/j.drup.2014.02.001
Cantón, R., Coque, T. M. (2006). The CTX-M β-lactamase pandemic. Curr. Opin. Microbiol. 9, 466–475. doi: 10.1016/j.mib.2006.08.011
Caprioli, A., Busani, L., Martel, J. L., Helmuth, R. (2000). Monitoring of antibiotic resistance in bacteria of animal origin: Epidemiological and microbiological methodologies. Int. J. Antimicrob. Agents. 14, 295–301. doi: 10.1016/S0924-8579(00)00140-0
Chen, Y., Chen, X., Zheng, S., Yu, F., Kong, H., Yang, Q., et al. (2014). Serotypes, genotypes and antimicrobial resistance patterns of human diarrhoeagenic Escherichia coli isolates circulating in southeastern China. Clin. Microbiol. Infect. 20, 52–58. doi: 10.1111/1469-0691.12188
Chiluisa-Guacho, C., Escobar-Perez, J., Dutra-Asensi, M. (2018). First detection of the CTX-M-15 producing Escherichia coli O25-ST131 pandemic clone in Ecuador. Pathogens. 7, 42. doi: 10.3390/pathogens7020042
CLSI (2023a). Performance standards for antimicrobial disk and dilution susceptibility tests for bacteria isolated from animals - 6th edition: VET01S (Clinical and Laboratory Standards Institute).
CLSI (2023b). Performance standards for antimicrobial susceptibility testing. 33rd (CLSI supplement M100. Clinical and Laboratory Standards Institute).
Coelho, A., Mora, A., Mamani, R., López, C., González-López, J. J., Larrosa, M. N., et al. (2011). Spread of Escherichia coli O25b:H4-B2-ST131 producing CTX-M-15 and SHV-12 with high virulence gene content in Barcelona (Spain). J. Antimicrob. Chemother. 66, 517–526. doi: 10.1093/jac/dkq491
Cubero, M., Grau, I., Tubau, F., Pallarés, R., Dominguez, M. A., Liñares, J., et al. (2016). Hypervirulent Klebsiella pneumoniae clones causing bacteraemia in adults in a teaching hospital in Barcelona, Spain, (2007-2013). Clin. Microbiol. Infect. 22, 154–160. doi: 10.1016/j.cmi.2015.09.025
Damborg, P., Broens, E. M., Chomel, B. B., Guenther, S., Pasmans, F., Wagenaar, J. A., et al. (2016). Bacterial zoonoses transmitted by household pets: state-of-the-art and future perspectives for targeted research and policy actions. J. Comp. Pathol. 155, S27–S40. doi: 10.1016/j.jcpa.2015.03.004
Delgado, D. Y. C., Barrigas, Z. P. T., Astutillo, S. G. O., Jaramillo, A. P. A., Ausili, A. (2016). Detection and molecular characterization of β-lactamase genes in clinical isolates of Gram-negative bacteria in Southern Ecuador. Braz. J. Infect. Dis. 20, 627–630. doi: 10.1016/j.bjid.2016.07.001
Ewers, C., Bethe, A., Semmler, T., Guenther, S., Wieler, L. H. (2012). Extended-spectrum β-lactamase-producing and AmpC-producing Escherichia coli from livestock and companion animals, and their putative impact on public health: A global perspective. Clin. Microbiol. Infect. 18, 646–655. doi: 10.1111/j.1469-0691.2012.03850.x
Fernandes, M. R., Sellera, F. P., Moura, Q., Carvalho, M. P. N., Rosato, P. N., Cerdeira, L., et al. (2018). Zooanthroponotic transmission of drug-resistant Pseudomonas aeruginosa, Brazil. Emerg. Infect. Dis. 24, 1160–1162. doi: 10.3201/eid2406.180335
Frenk, S., Rakovitsky, N., Temkin, E., Schechner, V., Cohen, R., Kloyzner, B. S., et al. (2020). Investigation of outbreaks of extended-spectrum beta-lactamase-producing Klebsiella pneumoniae in three neonatal intensive care units using whole genome sequencing. Antibiotics. 9, 1–10. doi: 10.3390/antibiotics9100705
Fuentes-Castillo, D., Esposito, F., Cardoso, B., Dalazen, G., Moura, Q., Fuga, B., et al. (2020). Genomic data reveal international lineages of critical priority Escherichia coli harbouring wide resistome in Andean condors (Vulturgryphus Linnaeus 1978). Mol. Ecol. 29, 1919–1935. doi: 10.1111/mec.15455
Furlan, J. P. R., Savazzi, E. A., Stehling, E. G. (2020). Genomic insights into multidrug-resistant and hypervirulent Klebsiella pneumoniae co-harboring metal resistance genes in aquatic environments. Ecotox. Environ. Saf. 201, 110782. doi: 10.1016/j.ecoenv.2020.110782
Guzmán-Blanco, M., Labarca, J. A., Villegas, M. V., Gotuzzo, E. (2014). Extended spectrum β-lactamase producers among nosocomial Enterobacteriaceae in Latin America. Braz. J. Infect. Dis. 18, 421–433. doi: 10.1016/j.bjid.2013.10.005
Harada, K., Shimizu, T., Mukai, Y., Kuwajima, K., Sato, T., Usui, M., et al. (2016). Phenotypic and molecular characterization of antimicrobial resistance in Klebsiella spp. isolates from companion animals in Japan: clonal dissemination of multidrug-resistant extended-spectrum β-lactamase-producing Klebsiella pneumoniae. Front. Microbiol. 7. doi: 10.3389/fmicb.2016.01021
Hassen, B., Abbassi, M. S., Benlabidi, S., Ruiz-Ripa, L., Mama, O. M., Ibrahim, C., et al. (2020). Genetic characterization of ESBL-producing Escherichia coli and Klebsiella pneumoniae isolated from wastewater and river water in Tunisia: predominance of CTX-M-15 and high genetic diversity. Environ. Sci. pollut. Res. 27, 44368–44377. doi: 10.1007/s11356-020-10326-w
Hedman, H. D., Eisenberg, J. N. S., Vasco, K. A., Blair, C. N., Trueba, G., Berrocal, V. J., et al. (2019). High prevalence of extended-spectrum beta-lactamase CTX-M-producing Escherichia coli in small-scale poultry farming in rural Ecuador. Am. J. Trop. Med. Hyg. 100, 374–376. doi: 10.4269/ajtmh.18-0173
Jacob, M. E., Keelara, S., Aidara-Kane, A., Matheu Alvarez, J. R., Fedorka-Cray, P. J. (2020). Optimizing a screening protocol for potential extended-spectrum β-lactamase Escherichia coli on MacConkey agar for use in a global surveillance program. J. Clin. Microbiol. 58, e01039–e01019. doi: 10.1128/JCM.01039-19
Jarlier, V., Nicolas, M. H., Fournier, G., Philippon, A. (1988). Extended broad-spectrum β-lactamases conferring transferable resistance to newer β-lactam agents in Enterobacteriaceae: hospital prevalence and susceptibility patterns. Clin. Infect. Dis. 10, 867–878. doi: 10.1093/clinids/10.4.867
Johnson, J. R., Clabots, C., Kuskowski, M. A. (2008). Multiple-host sharing, long-term persistence, and virulence of Escherichia coli clones from human and animal household members. J. Clin. Microbiol. 46, 4078–4082. doi: 10.1128/JCM.00980-08
Kawamura, K., Sugawara, T., Matsuo, N., Hayashi, K., Norizuki, C., Tamai, K., et al. (2017). Spread of CTX-type extended-spectrum β-lactamase-producing Escherichia coli isolates of epidemic clone B2-O25-ST131 among dogs and cats in Japan. Microb. Drug Resist. 23, 1059–1066. doi: 10.1089/mdr.2016.0246
Kiratisin, P., Apisarnthanarak, A., Saifon, P., Laesripa, C., Kitphati, R., Mundy, L. M. (2007). The emergence of a novel ceftazidime-resistant CTX-M extended-spectrum β-lactamase, CTX-M-55, in both community-onset and hospital-acquired infections in Thailand. Diagn. Microbiol. Infect. Dis. 58, 349–355. doi: 10.1016/j.diagmicrobio.2007.02.005
Kong, Z., Liu, X., Li, C., Cheng, S., Xu, F., Gu, B. (2020). Clinical molecular epidemiology of carbapenem-resistant Klebsiella pneumoniae among pediatric patients in Jiangsu province, China. Infect. Drug Resist. 13, 4627–4635. doi: 10.2147/IDR.S293206
Ku, Y. H., Chuang, Y. C., Chen, C. C., Lee, M. F., Yang, Y. C., Tang, H. J., et al. (2017). Klebsiella pneumoniae isolates from meningitis: epidemiology, virulence and antibiotic resistance. Sci. Rep. 7, 1–10. doi: 10.1038/s41598-017-06878-6
Leangapichart, T., Lunha, K., Jiwakanon, J., Angkititrakul, S., Järhult, J. D., Magnusson, U., et al. (2021). Characterization of Klebsiella pneumoniae complex isolates from pigs and humans in farms in Thailand: population genomic structure, antibiotic resistance and virulence genes. J. Antimicrob. Chemother. 76, 2012–2016. doi: 10.1093/jac/dkab118
Lopes, R., Fuentes-Castillo, D., Fontana, H., Rodrigues, L., Dantas, K., Cerdeira, L., et al. (2021). Endophytic lifestyle of global clones of extended-spectrum β-lactamase-producing priority pathogens in fresh vegetables: a trojan horse strategy favoring human colonization? MSystems. 6, e01125–e01120. doi: 10.1128/msystems.01125-20
Ludden, C., Moradigaravand, D., Jamrozy, D., Gouliouris, T., Blane, B., Naydenova, P., et al. (2020). A one health study of the genetic relatedness of Klebsiella pneumoniae and their mobile elements in the east of England. Clin. Infect. Dis. 70, 219–226. doi: 10.1093/cid/ciz174
Lupo, A., Saras, E., Madec, J. Y., Haenni, M. (2018). Emergence of blaCTX-M-55 associated with fosA, rmtB and mcr gene variants in Escherichia coli from various animal species in France. J. Antimicrob. Chemother. 73, 867–872. doi: 10.1093/jac/dkx489
Magiorakos, A. P., Srinivasan, A., Carey, R. B., Carmeli, Y., Falagas, M. E., Giske, C. G., et al. (2012). Multidrug-resistant, extensively drug-resistant and pandrug-resistant bacteria: An international expert proposal for interim standard definitions for acquired resistance. Clin. Microbiol. Infect. 18, 268–281. doi: 10.1111/j.1469-0691.2011.03570.x
Marcade, G., Brisse, S., Bialek, S., Marcon, E., Leflon-Guibout, V., Passet, V., et al. (2013). The emergence of multidrug-resistant Klebsiella pneumoniae of international clones ST13, ST16, ST35, ST48 and ST101 in a teaching hospital in the Paris region. Epidemiol. Infect. 141, 1705–1712. doi: 10.1017/S0950268812002099
Marshall, B. M., Levy, S. B. (2011). Food animals and antimicrobials: Impacts on human health. Clin. Microbiol. Rev. 24, 718–733. doi: 10.1128/CMR.00002-11
Martin, J., Phan, H. T. T., Findlay, J., Stoesser, N., Pankhurst, L., Navickaite, I., et al. (2017). Covert dissemination of carbapenemase-producing Klebsiella pneumoniae (KPC) in a successfully controlled outbreak: long- and short-read whole-genome sequencing demonstrate multiple genetic modes of transmission. J. Antimicrob. Chemother. 72, 3025–3034. doi: 10.1093/jac/dkx264
Matsumoto, Y., Ikeda, F., Kamimura, T., Yokota, Y., Mine, Y. (1988). Novel plasmid-mediated β-lactamase from Escherichia coli that inactivates oxyimino-cephalosporins. Antimicrob. Agents Chemother. 32, 1243–1246. doi: 10.1128/AAC.32.8.1243
Melo, L. C., Oresco, C., Leigue, L., Netto, H. M., Melville, P. A., Benites, N. R., et al. (2018). Prevalence and molecular features of ESBL/pAmpC-producing Enterobacteriaceae in healthy and diseased companion animals in Brazil. Vet. Microbiol. 221, 59–66. doi: 10.1016/j.vetmic.2018.05.017
Moreno, A., Bello, H., Guggiana, D., Domínguez, M., González, G. (2008). Extended-spectrum β-lactamases belonging to CTX-M group produced by Escherichia coli strains isolated from companion animals treated with enrofloxacin. Vet. Microbiol. 129, 203–208. doi: 10.1016/j.vetmic.2007.11.011
Nordberg, V., Quizhpe Peralta, A., Galindo, T., Turlej-Rogacka, A., Iversen, A., Giske, C. G., et al. (2013). High Proportion of intestinal colonization with successful epidemic clones of ESBL-producing Enterobacteriaceae in a neonatal intensive care unit in Ecuador. PloS One 8, e76597. doi: 10.1371/journal.pone.0076597
Okubo, T., Sato, T., Yokota, S. I., Usui, M., Tamura, Y. (2014). Comparison of broad-spectrum cephalosporin-resistant Escherichia coli isolated from dogs and humans in Hokkaido, Japan. J. Infect. Chemother. 20, 243–249. doi: 10.1016/j.jiac.2013.12.003
Ortega-Paredes, D., Barba, P., Mena-López, S., Espinel, N., Crespo, V., Zurita, J. (2020b). High quantities of multidrug-resistant Escherichia coli are present in the Machángara urban river in Quito, Ecuador. J. Water Health 18, 67–76. doi: 10.2166/wh.2019.195
Ortega-Paredes, D., Barba, P., Mena-López, S., Espinel, N., Zurita, J. (2018). Escherichia coli hyperepidemic clone ST410-A harboring blaCTX-M-15 isolated from fresh vegetables in a municipal market in Quito-Ecuador. Int. J. Food Microbiol. 280, 41–45. doi: 10.1016/j.ijfoodmicro.2018.04.037
Ortega-Paredes, D., de Janon, S., Villavicencio, F., Ruales, K. J., de la Torre, K., Villacís, J. E., et al. (2020a). Broiler farms and carcasses are an important reservoir of multi-drug resistant Escherichia coli in Ecuador. Front. Vet. Sci. 7. doi: 10.3389/fvets.2020.547843
Ortega-Paredes, D., Haro, M., Leoro-Garzón, P., Barba, P., Loaiza, K., Mora, F., et al. (2019). Multidrug-resistant Escherichia coli isolated from canine faeces in a public park in Quito, Ecuador. J. Glob. Antimicrob. Resist. 18, 263–268. doi: 10.1016/j.jgar.2019.04.002
Papa-Ezdra, R., Caiata, L., Palacio, R., Outeda, M., Cabezas, L., Bálsamo, A., et al. (2021). Prevalence and molecular characterisation of carbapenemase-producing Enterobacterales in an outbreak-free setting in a single hospital in Uruguay. J. Glob. Antimicrob. Resist. 24, 58–62. doi: 10.1016/j.jgar.2020.11.006
Patil, S., Chen, X., Wen, F. (2019). Exploring the phenotype and genotype of multi-drug resistant Klebsiella pneumoniae harbouring blaCTX-M group extended-spectrum β-lactamases recovered from paediatric clinical cases in Shenzhen, China. Ann. Clin. Microbiol. Antimicrob. 18, 1–6. doi: 10.1186/s12941-019-0331-z
Pesesky, M. W., Hussain, T., Wallace, M., Wang, B., Andleeb, S., Burnham, C. D., et al. (2015). KPC and NDM-1 genes in related Enterobacteriaceae strains and plasmids from Pakistan and the United States. Emerg. Infect. Dis. 21, 1034–1037. doi: 10.3201/eid2106.141504
Piazza, A., Comandatore, F., Romeri, F., Brilli, M., Dichirico, B., Ridolfo, A., et al. (2019). Identification of blaVIM-1 gene in ST307 and ST661 Klebsiella pneumoniae clones in Italy: old acquaintances for new combinations. Microb. Drug Resist. 25, 787–790. doi: 10.1089/mdr.2018.0327
Pitout, J. D., Laupland, K. B. (2008). Extended-spectrum β-lactamase-producing Enterobacteriaceae: an emerging public-health concern. Lancet Infect. Dis. 8, 159–166. doi: 10.1016/S1473-3099(08)70041-0
Pomba, C., Rantala, M., Greko, C., Baptiste, K. E., Catry, B., van Duijkeren, E., et al. (2017). Public health risk of antimicrobial resistance transfer from companion animals. J. Antimicrob. Chemother. 72, 957–968. doi: 10.1093/jac/dkw481
Salgado-Caxito, M., Benavides, J. A., Adell, A. D., Paes, A. C., Moreno-Switt, A. I. (2021). Global prevalence and molecular characterization of extended-spectrum β-lactamase producing-Escherichia coli in dogs and cats - a scoping review and meta-analysis. One Health 12, 100236. doi: 10.1016/j.onehlt.2021.100236
Salinas, L., Loayza, F., Cárdenas, P., Saraiva, C., Johnson, T. J., Amato, H., et al. (2021). Environmental spread of extended spectrum beta-lactamase (ESBL) producing Escherichia coli and ESBL genes among children and domestic animals in Ecuador. Environ. Health Perspect. 129, 27007. doi: 10.1289/EHP7729
Samanta, I., Joardar, S. N., Mahanti, A., Bandyopadhyay, S., Sar, T. K., Dutta, T. K. (2015). Approaches to characterize extended spectrum beta-lactamase/beta-lactamase producing Escherichia coli in healthy organized vis-a-vis backyard farmed pigs in India. Infect. Genet. Evol. 36, 224–230. doi: 10.1016/j.meegid.2015.09.021
Sánchez-Salazar, E., Gudiño, M. E., Sevillano, G., Zurita, J., Guerrero-López, R., Jaramillo, K., et al. (2020). Antibiotic resistance of Salmonella strains from layer poultry farms in central Ecuador. J. Appl. Microbiol. 128, 1347–1354. doi: 10.1111/jam.14562
Seiffert, S. N., Hilty, M., Perreten, V., Endimiani, A. (2013). Extended-spectrum cephalosporin-resistant gram-negative organisms in livestock: An emerging problem for human health? Drug Resist. Updat. 16, 22–45. doi: 10.1016/j.drup.2012.12.001
Sellera, F. P., Da Silva, L. C. B. A., Lincopan, N. (2021). Rapid spread of critical priority carbapenemase-producing pathogens in companion animals: a One Health challenge for a post-pandemic world. J. Antimicrob. Chemother. 76, 2225–2229. doi: 10.1093/jac/dkab169
Sghaier, S., Abbassi, M. S., Pascual, A., Serrano, L., Díaz-De-Alba, P., Said, M., et al. (2019). Extended-spectrum β-lactamase-producing Enterobacteriaceae from animal origin and wastewater in Tunisia: first detection of O25b-B23-CTX-M-27-ST131 Escherichia coli and CTX-M-15/OXA-204-producing Citrobacter freundii from wastewater. J. Glob. Antimicrob. Resist. 17, 189–194. doi: 10.1016/j.jgar.2019.01.002
Shen, Z., Gao, Q., Qin, J., Liu, Y., Li, M. (2020). Emergence of an NDM-5-producing hypervirulent Klebsiella pneumoniae sequence type 35 strain with chromosomal integration of an integrative and conjugative element, ICEKp1. Antimicrob. Agents Chemother. 64, e01675–e01619. doi: 10.1128/AAC.01675-19
Soria Segarra, C., Soria Baquero, E., Cartelle Gestal, M. (2018). High prevalence of CTX-M-1-like enzymes in urinary isolates of Escherichia coli in Guayaquil, Ecuador. Microb. Drug Resist. 24, 393–402. doi: 10.1089/mdr.2017.0325
Surleac, M., Barbu, I. C., Paraschiv, S., Popa, L. I., Gheorghe, I., Marutescu, L., et al. (2020). Whole genome sequencing snapshot of multidrug resistant Klebsiella pneumoniae strains from hospitals and receiving wastewater treatment plants in Southern Romania. PloS One 15, 1–17. doi: 10.1371/journal.pone.0228079
Tacconelli, E., Carrara, E., Savoldi, A., Harbarth, S., Mendelson, M., Monnet, D. L., et al. (2018). Discovery, research, and development of new antibiotics: the WHO priority list of antibiotic-resistant bacteria and tuberculosis. Lancet Infect. Dis. 18, 318–327. doi: 10.1016/S1473-3099(17)30753-3.
Toledano-Tableros, J. E., Gayosso-Vázquez, C., Jarillo-Quijada, M. D., Fernández-Vázquez, J. L., Morfin-Otero, R., Rodríguez-Noriega, E., et al. (2021). Dissemination of blaNDM–1 gene among several Klebsiella pneumoniae sequence types in Mexico associated with horizontal transfer mediated by IncF-like plasmids. Front. Microbiol. 12. doi: 10.3389/fmicb.2021.611274
Umber, J. K., Bender, J. B. (2009). Pets and antimicrobial resistance. Vet. Clin. N. Am. - Small Anim. Pract. 39, 279–292. doi: 10.1016/j.cvsm.2008.10.016
Umpiérrez, A., Bado, I., Oliver, M., Acquistapace, S., Etcheverría, A., Padola, N. L., et al. (2017). Zoonotic potential and antibiotic resistance of Escherichia coli in neonatal calves in Uruguay. Microbes Environ. 32, 275–282. doi: 10.1264/jsme2.ME17046
Villegas, M. V., Kattan, J. N., Quinteros, M. G., Casellas, J. M. (2008). Prevalence of extended-spectrum β-lactamases in South America. Clin. Microb. Infect. 14, 154–158. doi: 10.1111/j.1469-0691.2007.01869.x
Vinueza-Burgos, C., Cevallos, M., Ron-Garrido, L., Bertrand, S., De Zutter, L. (2016). Prevalence and diversity of Salmonella serotypes in Ecuadorian broilers at slaughter age. PloS One 11, 1–12. doi: 10.1371/journal.pone.0159567
Vinueza-Burgos, C., Ortega-Paredes, D., Narvaéz, C., De Zutter, L., Zurita, J. (2019). Characterization of cefotaxime resistant Escherichia coli isolated from broiler farms in Ecuador. PloS One 14, 1–14. doi: 10.1371/journal.pone.0207567
Yan, J. J., Wang, M. C., Zheng, P. X., Tsai, L. H., Wu, J. J. (2015). Associations of the major international high-risk resistant clones and virulent clones with specific ompK36 allele groups in Klebsiella pneumoniae in Taiwan. New Microbes New Infect. 5, 1–4. doi: 10.1016/j.nmni.2015.01.002
Yang, J.-T., Zhang, L.-J., Lu, Y., Zhang, R.-M., Jiang, H.-X. (2023). Genomic insights into global blaCTX-M-55-positive Escherichia coli epidemiology and transmission characteristics. Microbiol. Spectr. 11, e0108923. doi: 10.1128/spectrum.01089-23
Yasugi, M., Hatoya, S., Motooka, D., Matsumoto, Y., Shimamura, S., Tani, H., et al. (2021). Whole-genome analyses of extended-spectrum or AmpC β-lactamase-producing Escherichia coli isolates from companion dogs in Japan. PLoSONE. 16, e0246482. doi: 10.1371/journal.pone.0246482
Zhang, J., Zheng, B., Zhao, L., Wei, Z., Ji, J., Li, L., et al. (2014). Nationwide high prevalence of CTX-M and an increase of CTX-M-55 in Escherichia coli isolated from patients with community-onset infections in Chinese County Hospitals. BMC Infect. Dis. 14, 659. doi: 10.1186/s12879-014-0659-0
Zhao, F., Feng, Y., Lü, X., McNally, A., Zong, Z. (2017). Remarkable diversity of Escherichia coli carrying mcr-1 from hospital sewage with the identification of two new mcr-1 variants. Front. Microbiol. 8. doi: 10.3389/fmicb.2017.02094
Zhao, D., Zuo, Y., Wang, Z., Li, J. (2019). Characterize carbapenem-resistant Klebsiella pneumoniae isolates for nosocomial pneumonia and their Gram-negative bacteria neighbors in the respiratory tract. Mol. Biol. Rep. 46, 609–616. doi: 10.1007/s11033-018-4515-y
Zhong, X. S., Li, Y. Z., Ge, J., Xiao, G., Mo, Y., Wen, Y. Q., et al. (2020). Comparisons of microbiological characteristics and antibiotic resistance of Klebsiella pneumoniae isolates from urban rodents, shrews, and healthy people. BMC Microbiol. 20, 1–8. doi: 10.1186/s12866-020-1702-5
Zogg, A. L., Simmen, S., Zurfluh, K., Stephan, R., Schmitt, S. N., Nüesch-Inderbinen, M. (2018). High prevalence of extended-spectrum β-Lactamase producing Enterobacteriaceae among clinical isolates from cats and dogs admitted to a veterinary hospital in Switzerland. Front. Vet. Sci. 5. doi: 10.3389/fvets.2018.00062
Zurita, J., Alcocer, I., Ortega-Paredes, D., Barba, P., Yauri, F., Iñiguez, D., et al. (2013). Carbapenem-hydrolysing β-lactamase KPC-2 in Klebsiella pneumoniae isolated in Ecuadorian hospitals. J. Glob. Antimicrob. Resist. 1, 229–230. doi: 10.1016/j.jgar.2013.06.001
Zurita, J., Solís, M. B., Ortega-Paredes, D., Barba, P., Paz y Miño, A., Sevillano, G. (2019). High prevalence of B2-ST131 clonal group among extended-spectrum β-lactamase-producing Escherichia coli isolated from bloodstream infections in Quito, Ecuador. J. Glob. Antimicrob. Resist. 19, 216–221. doi: 10.1016/j.jgar.2019.04.019
Keywords: ESβL, gram-negative bacteria, Enterobacterales, antimicrobial resistance, One Health, veterinary medicine, genomic data
Citation: Gonzales-Zubiate FA, Tambor JHM, Valencia-Bacca J, Villota-Burbano MF, Cardenas-Arias A, Esposito F, Moura Q, Fuga B, Sano E, Pariona JGM, Jacome MPO and Lincopan N (2025) Pandemic one health clones of Escherichia coli and Klebsiella pneumoniae producing CTX-M-14, CTX-M-27, CTX-M-55 and CTX-M-65 ESβLs among companion animals in northern Ecuador. Front. Cell. Infect. Microbiol. 13:1259764. doi: 10.3389/fcimb.2023.1259764
Received: 16 July 2023; Accepted: 10 October 2023;
Published: 07 January 2025.
Edited by:
Margarita María De La Paz Arenas-Hernandez, Benemérita Universidad Autónoma de Puebla, MexicoReviewed by:
Edwin Barrios-Villa, Universidad de Sonora, Sonora, MexicoTahereh Navidifar, Shoushtar Faculty of Medical Sciences, Iran
Copyright © 2025 Gonzales-Zubiate, Tambor, Valencia-Bacca, Villota-Burbano, Cardenas-Arias, Esposito, Moura, Fuga, Sano, Pariona, Jacome and Lincopan. This is an open-access article distributed under the terms of the Creative Commons Attribution License (CC BY). The use, distribution or reproduction in other forums is permitted, provided the original author(s) and the copyright owner(s) are credited and that the original publication in this journal is cited, in accordance with accepted academic practice. No use, distribution or reproduction is permitted which does not comply with these terms.
*Correspondence: Fernando A. Gonzales-Zubiate, ZmdvbnphbGVzQHlhY2hheXRlY2guZWR1LmVj; Nilton Lincopan, bGluY29wYW5AdXNwLmJy