- 1Department of Respiratory Medicine, The Second Xiangya Hospital of Central South University, Changsha, China
- 2Critical Care Medicine, The Second Xiangya Hospital of Central South University, Changsha, China
- 3Department of respiratory medicine, Hunan Provincial People’s Hospital, Changsha, China
Background: Nowadays, there is still no effective treatment developed for COVID-19, and early identification and supportive therapies are essential in reducing the morbidity and mortality of COVID-19. This is the first study to evaluate D-dimer to lymphocyte ratio (DLR) as a prognostic utility in patients with COVID-19.
Methods: We retrospectively analyzed 611 patients and separated them into groups of survivors and non-survivors. The area under the curve (AUC) of various predictors integrated into the prognosis of COVID-19 was compared using the receiver operating characteristic (ROC) curve. In order to ascertain the interaction between DLR and survival in COVID-19 patients, the Kaplan-Meier (KM) curve was chosen.
Results: Age (OR = 1.053; 95% CI, 1.022-1.086; P = 0.001), NLR (OR = 1.045; 95% CI, 1.001-1.091; P = 0.046), CRP (OR = 1.010; 95% CI, 1.005-1.016; P < 0.001), PT (OR = 1.184; 95% CI, 1.018-1.377; P = 0.029), and DLR (OR = 1.048; 95% CI, 1.018-1.078; P = 0.001) were the independent risk factors related with the mortality of COVID-19. DLR had the highest predictive value for COVID-19 mortality with the AUC of 0.924. Patients’ survival was lower when compared to those with lower DLR (Log Rank P <0.001).
Conclusion: DLR might indicate a risk factor in the mortality of patients with COVID-19.
Introduction
After being discovered for the first time in Wuhan, China in December 2019, the coronavirus disease 2019 (COVID-19) moved across the globe at an unprecedented speed. The World Health Organization (WHO) declared it to be a global pandemic on March 11, 2020, which marked the outbreak of COVID-19 in the world (Chen et al., 2021). The effects of COVID-19 on health and socio-economic development are still being felt and no viable remedy has yet been identified. Almost 5% of critically ill patients exhibit a rapid progression to acute respiratory distress syndrome (ARDS), which ultimately results in functional failure of important organs or even death with a mortality rate of 49% among critical cases, despite the fact that the majority of COVID-19 patients are asymptomatic or have mild symptoms (Wu and McGoogan, 2020). Early detection and supportive therapy can significantly lower morbidity and mortality. Therefore, timely establishment of reliable predictors associated with the prognosis of COVID-19 is of paramount importance.
The most sensitive coagulation measure, fibrin fragment D-dimer, which is formed when plasmin cleaves the insoluble fibrin, predicts higher thrombosis risk and frequently serves as a rule-out method for thrombosis with a high sensitivity of 80%−100% and negative predictive value (NPV) of up to 100% (Wells et al., 2001). Nonetheless, D-dimer has limited specificity for vascular embolism, and an increase in D-dimer level appears to occur when there is a production or breakdown of fibrin. According to reports, the D-dimer level is enhanced in several different circumstances, including infectious disorders, pregnancy, cancer, trauma, and surgery (Snijders et al., 2012; Lippi et al., 2014; Fruchter et al., 2015). The most common cause of an increase in D-dimer levels in adult patients receiving emergency care is infection, not venous thrombosis (Lippi et al., 2014). Coagulation and inflammation have a mutually beneficial relationship. The coagulation system can be activated by the released inflammatory mediators and, in turn, some coagulation components can intensify inflammatory responses (Marshall, 2001).
The immune system is maintained by lymphocytes and the subsets that include B cells, natural killer (NK) cells, CD4+ T cells, and CD8+ T cells. Mature naive T cells with antigen experience can transform into central and effector memory cells (van den Broek et al., 2018). The central memory cells can be activated by the contact of another antigen and developed into central or effector memory cells, while the important function of effector T lymphocytes is the clearance of virus-infected cells (Boldt et al., 2014), which is the primary task of T CD8+ cells. Mature CD4+ cells stimulate the development of B lymphocytes to produce specific antibodies and control the proliferation, activation, and differentiation of CD8+ cytotoxic T lymphocytes. Variable virus types have different numbers of total lymphocytes and subsets, indicating a possible connection between viral pathogenesis processes and changes in lymphocyte subsets (Li et al., 2004). Numerous studies have demonstrated that the majority of COVID-19 patients had peripheral blood lymphocyte counts and subsets that were significantly lower than normal, particularly in the severe cases (Wang et al., 2020), which were previously reported and confirmed in severe acute respiratory syndrome (SARS) and Middle East respiratory syndrome (MERS) (Huang et al., 2005; Chu et al., 2016).
However, there is currently no published literature examining the association of D-dimer to lymphocyte ratio (DLR) with the prognosis of COVID-19. Thus, the goal of the current study was to determine whether DLR might be used as a biomarker to predict COVID-19 prognosis.
Materials and methods
Study design and participants
The real-time polymerase chain reaction was used to diagnose 611 patients with COVID-19 who had been hospitalized by March 26, 2020, at Tongji Medical College of Huazhong University of Science and Technology and Public Health Treatment Center of Changsha, China. This was a retrospective study involving multiple centers. Patients aged 18 years or older were recruited for the study. With regard to survival status, we split the patients into two groups: survivors and non-survivors. The study was approved by the Ethics Committee of Second Xiangya Hospital of Central South University (No.2020001).
Data collection
On admission, routine blood test results (white blood cells [WBC], lymphocytes, neutrophils, neutrophils to lymphocytes ratio [NLR], platelet [PLT], C-reactive proteins [CRP], prothrombin time [PT], activated partial thromboplastin time [APTT], D-dimer, and DLR) were noted along with demographic information and symptoms of underlying disease.
Definition and endpoint
The analysis of the receiver operating characteristic (ROC) curve for the effect of DLR in predicting the mortality of COVID-19 defined high (or low) DLR as the ratio above or equal (or below) the cutoff value. Patient deaths while being treated in a hospital served as the endpoint.
Statistical analysis
Continuous variables were expressed as the median with interquartile range (IQR), and due to the non-normal distribution of all the data, the Mann-Whitney test was used to examine differences between survivors and non-survivors. For categorical variables, frequency and percentage were given, and Fisher’s exact test (χ2 test) was used to assess, as necessary. The risk factors linked to the mortality of COVID-19 patients were screened using a univariate and multivariate logistic regression. To determine the predictive value of biomarkers for the mortality of COVID-19, the ROC curve and the AUC were examined. Survival analysis with high and low levels of DLR was carried out utilizing the Kaplan-Meier (KM) curve and the log rank test. IBM®SPSS software, version 26 (Chicago, IL, USA) was used for all statistical analyses.
Results
A total of 611 COVID-19 patients were enrolled in the research, of whom 537 recovered and 74 died. Demographic characteristics, symptoms, and comorbidities of all patients are shown in Table 1. The median ages of the survivors and non-survivors are 54.50 years and 70 years, respectively. Comparing non-survivors with survivors, there were statistically significant variations in the age, gender, and cardiovascular disease comorbidity (p < 0.05).
Regarding laboratory findings on admission, the levels of white blood cells (WBC), neutrophils, neutrophils to lymphocytes ratio (NLR), C-reactive proteins (CRP), prothrombin time (PT), activated partial thromboplastin time (APTT), D-dimer, and DLR of the non-survivors were significantly higher compared with the survivors (p < 0.05), whilst the levels of lymphocytes, platelet, and albumin of the non-survivors were significantly lower than those of the survivors (p < 0.05) (Table 2).
The risk variables related to the mortality of patients with COVID-19 were age, gender, comorbidity with cardiovascular disease, WBC, lymphocytes, neutrophils, NLR, PLT, CRP, PT, APTT, D-dimer, and DLR (p < 0.05) (Table 3).
Age (OR = 1.053; 95% CI, 1.022−1.086; p = 0.001), NLR (OR = 1.045; 95% CI, 1.001−1.091; p = 0.046), CRP (OR = 1.010; 95% CI, 1.005−1.016; p < 0.001), PT (OR = 1.184; 95% CI, 1.018−1.377; p = 0.029), and DLR (OR = 1.048; 95% CI, 1.018−1.078; p = 0.001) were the independent risk factors related with the mortality of COVID-19 (Table 4).
ROC analysis indicated that lymphocytes, NLR, CRP, PT, D-dimer, and DLR were able to significantly predict the mortality of patients with COVID-19 (p < 0.001) with the AUCs being 0.850 (95% CI, 0.820−0.878), 0.896 (95% CI, 0.869−0.919), 0.870 (0.840−0.896), 0.858 (95% CI, 0.828−0.885), 0.902 (95% CI, 0.875−0.924), and 0.924 (95% CI, 0.900−0.943), respectively. The cutoff values for lymphocytes, NLR, CRP, PT, D-dimer, and DLR were 0.78 (sensitivity, 83.78; specificity, 75.98), 6.28 (sensitivity, 86.30; specificity, 81.94), 42.30 (sensitivity, 86.30; specificity, 76.00), 13.80 (sensitivity, 85.14; specificity, 71.08), 0.82 (sensitivity, 94.59; specificity, 72.20), and 1.29 (sensitivity, 93.24; specificity, 79.52), respectively (Table 5 and Figure 1).
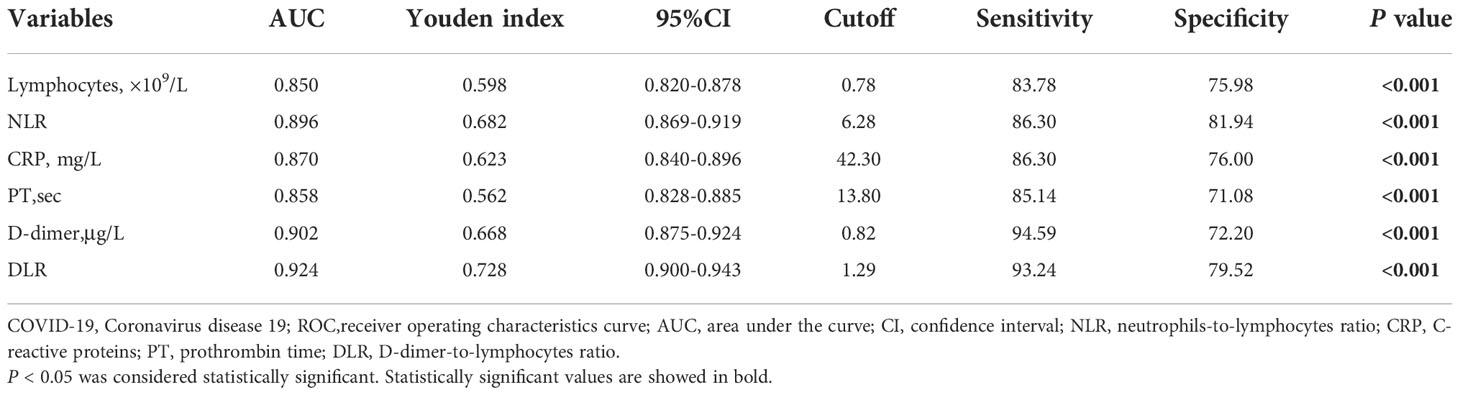
Table 5 Comparison of the biomarkers for predicting the prognosis of COVID-19 patients using ROC analysis.
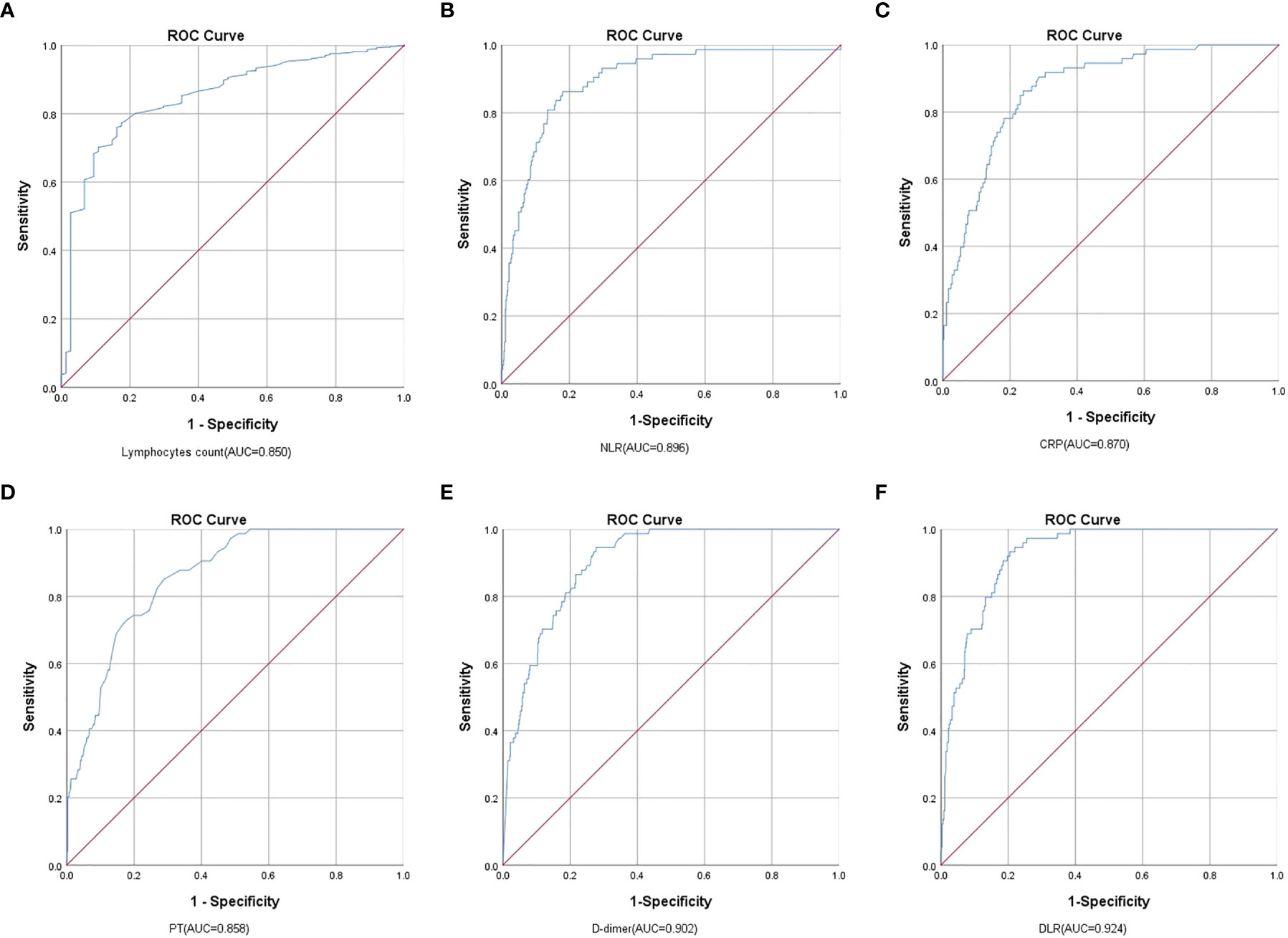
Figure 1 Analysis of the ROC curve of the (A) Lymphocytes, (B) NLR, (C) CRP, (D) PT, (E) D-dimer, and (F) DLR for the mortality of COVID-19. Comparing Lymphocyte, NLR, CRP, PT, D-dimer, and DLR, DLR had the highest AUC in predicting COVID-19 mortality.
By using the KM curve to investigate the relationship between DLR and survival, patients with high DLR showed a worse survival than those with low DLR (log rank p < 0.001) (Figure 2).
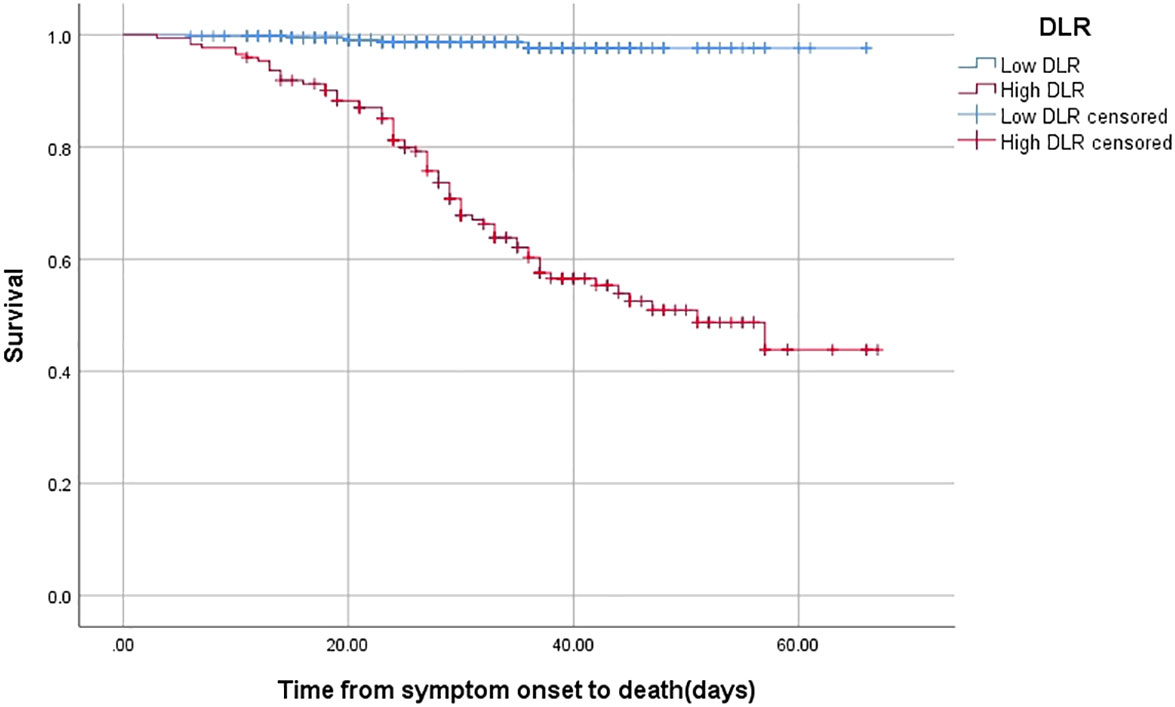
Figure 2 The time-dependent risk of death in COVID-19 patients with low and high DLR using Kaplan-Meier curve. Patients with high DLR showed a worse survival compared with those with low DLR (Log Rank P <0.001).
Discussion
In the presentinvestigation, we discovered that DLR was a unique risk factor and had the most valuable prediction performance for mortality of in-hospital patients with COVID-19. Additionally, survival rates were worse for individuals with COVID-19 who had higher DLR than for those who had lower DLR.
Recent studies have uncovered that D-dimer was correlated with the severity and prognosis of COVID-19 (Gao et al., 2020; Zhou et al., 2020). Patients with COVID-19 who are hospitalized frequently have coagulopathy. Critically ill COVID-19 patients first exhibit high D-dimer values, which are then followed by an increase in partial thromboplastin times, aberrant prothrombin levels, and lastly low platelet counts (Connors and Levy, 2020). Diffuse alveolar damage, hyaline membrane formation, and pulmonary micro-emboli are highly reported in the autopsy of COVID-19 patients (Fox et al., 2020). In fact, severe acute respiratory syndrome coronavirus 2 (SARS-CoV-2)-induced endothelial dysfunction, platelet activation, excessive inflammation, and stasis can make patients more susceptible to thrombotic arterial and venous occlusions (Piazza et al., 2020). According to Tang et al. (Tang et al., 2020), inflammatory cytokines can disrupt the equilibrium between fibrinolysis and coagulation, and then activate the fibrinolysis system, which raises the level of D-dimer. D-dimer binding to “intracellular” adhesion molecule 1 (ICAM-1), an immunoglobulin-family adhesion molecule that is expressed on the membrane of leukocytes as well as endothelial cells, can increase vascular tone and impair blood circulation (Lominadze et al., 2005).
By increasing blood viscosity and activating signaling pathways dependent on hypoxia-inducible transcription factor, SARS-CoV-2 infection that results in organ damage and hypoxemia may promote the formation of thrombosis (Tang et al., 2020). Furthermore, patients with COVID-19 are more likely to have comorbid conditions, be immobile, and undergo invasive procedures, all of which raise the risk of developing thrombotic events (Zhang et al., 2020). It was reported that the level of D-dimer presented a heightened risk of cardiovascular and peripheral arterial diseases (Soomro et al., 2016). Mohamed et al. (Abu-Farha et al., 2020) genotyped the genes of COVID-19 patients for single nucleotide polymorphisms (SNPs) and found that the top three genetic associations with the elevated level of D-dimer were rs13109457-A, rs12029080-G, and rs6687813-A located in F5, FGG, and FGA, which could aid in identifying patients who are most at risk of thromboembolic complications. It is interesting to note that D-dimer may contribute to the pathophysiology in addition to serving as a biomarker. After administering the purified human fragment D to a rabbit, several physiological events take place. These include hypoxia, complement activation, neutropenia chemotaxis, enhanced pulmonary capillary leakage, platelet aggregation, and prostaglandin synthesis (Manwaring and Curreri, 1981; Manwaring and Curreri, 1982).
In most cases, the immunological response brought on by SARS-CoV-2 infection can be subdued through attracting monocytes and macrophages to the site of infection, releasing cytokines, and initiating adaptive T and B cell immune responses (Tay et al., 2020). However, a defective immune response can occur in specific circumstances, and lymphopenia is a prevalent clinical symptom. Together with profound lung lesions, autopsy results reveal cell degeneration, lymphopenia and necrosis in the spleen, lymphopenia and localized necrosis in the lymph nodes, and decreased myelopoiesis in the bone marrow (Yao et al., 2020).
There are multiple reasons that have been proposed to demonstrate the relationship between lymphopenia and COVID-19. Angiotensin-converting enzyme 2 (ACE2) is the necessary entry receptor for SARS-CoV-2, and the serine protease TMPRSS2 facilitates the interaction of the spike (S) glycoprotein of coronaviruses with receptor molecules (Hoffmann et al., 2020). It has been established that decreased levels of cytotoxic lymphocytes, such as CD8+ T cells and NK cells, had a striking link with elevated levels of ACE2 and TMPRSS2, inferring that patients who are more defenseless against SARS-CoV-2 infection may not have the lung capacity to generate a rapid antiviral cellular immune response (Duijf, 2020).
Immunosenescence is the age-associated functional deterioration of the immune system which includes decreased naïve lymphocytes production, an increase in memory lymphocytes, lower proliferative capacity and impaired function of effector lymphocytes, lymph node fibrosis, and dysregulated cytokine production (Pera et al., 2015). Patients with this condition demonstrate a decreased response to vaccination and are more prone to infection as a result, making the disease more contagious. Likewise, due to underlying comorbidities, aged people with COVID-19 have a higher prevalence of serious diseases and a worse prognosis (Qin et al., 2020). Tumor necrosis factor receptor superfamily member Fas (CD95), which is primarily expressed on effector and memory T cells following interaction with antigen but not on naive T cells, mediates apoptosis via death receptor pathway to maintain immunological homeostasis. Bellesi et al. (Bellesi et al., 2020), using flow cytometry, discovered that COVID-19 patients had greater levels of CD95 than controls, and that increased CD95 expression in CD4+ cells was related with decreased CD4+ counts, revealing a further mechanism for lymphopenia by which CD95 triggered apoptosis in COVID-19 patients. Local or systemic cytokine release is typical with COVID-19 and interleukin (IL)-6, IL-1β, and tumor necrosis factor-α (TNF-α) play key roles in the progression and exacerbation pathogenesis of COVID-19. Autophagy, an evolutionarily conserved lysosomal degradation system, has been implicated in regulating maturation and survival of T and B lymphocytes (Vomero et al., 2020). Recently, IL-6, IL-1β, and TNF-α have been described to be involved in autophagy regulation. It has been shown that IL-6 could activate STAT3 through Janus-activated kinases (Jak) (Wang et al., 2013), and rheumatoid arthritis patients treated with anti-Jak inhibitors in vitro saw an inhibition of lymphocyte autophagy (Vomero et al., 2020). Tumor necrosis factor-α can induce autophagy, further activate lymphocytes, and attract lymphocytes causing excessive inflammation of typical COVID-19 infection (Vomero et al., 2020). It is widely known that IL-1β has a direct effect on lymphocytes. Inflammasome promotes IL-1β secretion via the activation of caspase-1, which medicates autophagy in the regulation of inflammation (Vomero et al., 2020). A novel therapeutic approach for COVID-19 is drugs that target autophagy. Moreover, TNF-α combining with TNFR1 to promote T cell apoptosis is a characteristic of aged T cells (Gupta et al., 2005), IL-2 is an essential cytokine for the proliferation, differentiation, and function of T cells (Ross and Cantrell, 2018), and secretion of cytokines can draw T lymphocytes from blood to the site of infection, which may explain lymphopenia in patients with COVID-19 (Xu et al., 2020). It was observed that after two years of recovery, coronavirus-specific memory T cells could be discovered (Yang et al., 2006).
Hemostasis intricately interacts with the immune system. Neutrophils, platelets, and the coagulation cascade work together to increase the host’s ability to defend itself against pathogenic assaults in immunothrombosis, which is a type of physiological thrombosis (Massberg et al., 2010). The microvasculature is primarily affected by immunothrombotic dysregulation, which can occur in some specific inflammatory situations and upset the natural immunothrombosis equilibrium. Patients with COVID-19 are thought to have this important mechanism (Nicolai et al., 2020). Nicolai et al. (Nicolai et al., 2020) investigated the contribution of immunothrombosis in COVID-19 using immunofluorescence and confocal microscopy and discovered that neutrophils embedded in the fibrin clot in the microthrombi, and numerous intravascular neutrophils were intimately affiliated to platelets and fibrin.
Indeed, circulating platelet-leukocyte complexes can trigger the onset of neutrophil extracellular traps (NETs), which enable the development of immunothrombosis (Clark et al., 2007). Brinkmann et al. (Brinkmann et al., 2004) first described neutrophil cell death in 2004, positing that it could operate as a primitive defense to trap and kill microbial invaders and implement a procoagulant and prothrombotic scaffold (Fuchs et al., 2010). Multiple researchers have revealed that NETs were crucial components of microvascular and macrovascular thrombosis (Blasco et al., 2020) and bronchoalveolar lavage fluid in COVID-19 patients (Ouwendijk et al., 2021). Compared with healthy controls, there was a 50-fold increase in NETs release by neutrophils of COVID-19 patients, and healthy neutrophils might also develop NETs when exposed to plasma from COVID-19 patients (Middleton et al., 2020). In COVID-19, immunothrombotic occlusions with NETs were four times more common than influenza pneumonia (Nicolai et al., 2021). Dexamethasone and colchicine—NETs inhibitors—were recommended to lower COVID-19 patient mortality (Deftereos et al., 2020; Tomazini et al., 2020), and DNase1 could degrade NETs to reduce thrombosis in immunothrombotic models (Jimenez-Alcazar et al., 2017), highlighting that NETs might just be a focus of attention for COVID-19 treatment.
In the study, DLR had a greater AUC than D-dimer or lymphocytes separately for COVID-19 death, which suggested that the combination of D-dimer and lymphocytes might be superior in reflecting the status of COVID-19 patients. We speculated that DLRmight be concerned in the immunothrombosis of COVID-19. To pinpoint the precise mechanism through which DLR contributes to immunothrombosis, more study is necessary.
The study includes several limitations. First, because it was a retrospective study, only the initial level of DLR upon admission was noted and the association between time-dependent changes in DLR and prognostic relevance is yet unknown. Second, the sample size is relatively small, which could have influenced the statistical findings. Third, there was no D-dimer and lymphocyte values prior to COVID-19. For the value of underlying comorbidities with elevated D-dimer levels, this is an important factor to consider. Finally, the study cohort was entirely Chinese, therefore extrapolating the findings to other racial or ethnic groups might be limited.
Taken together, DLR might be quite important in forecasting the outcome of COVID-19 when taken as a whole. To identify viable strategies for treating COVID-19, the levels of DLR should, if necessary, be monitored, and the reason for the change in DLR should be examined.
Data availability statement
The raw data supporting the conclusions of this article will be made available by the authors, without undue reservation.
Author contributions
YZ and SW contributed to the conception, study design, implementation, and critical revision. FP contributed to methodology, software, and writing original draft preparation. CL, QY, JD and QZ collected and interpreted the data. MX and CW contributed to the conception and study design. All authors contributed to the article and approved the submitted version.
Funding
This work was supported by Key field research and development plan of science and technology department of Hunan province [No.2019SK2022], the Natural Science Foundation of Hunan Province, China [No.2021JJ40896, No.2022JJ40221], and Natural Science Foundation of China [No.81900056].
Acknowledgments
We acknowledge Key field research and development plan of science and technology department of Hunan province, the Natural Science Foundation of Hunan Province, and Natural Science Foundation of China for funding our project.
Conflict of interest
The authors declare that the research was conducted in the absence of any commercial or financial relationships that could be construed as a potential conflict of interest.
Publisher’s note
All claims expressed in this article are solely those of the authors and do not necessarily represent those of their affiliated organizations, or those of the publisher, the editors and the reviewers. Any product that may be evaluated in this article, or claim that may be made by its manufacturer, is not guaranteed or endorsed by the publisher.
References
Abu-Farha, M., Al-Sabah, S., Hammad, M. M., Hebbar, P., Channanath, A. M., John, S. E., et al. (2020). Prognostic genetic markers for thrombosis in COVID-19 patients: A focused analysis on d-dimer, homocysteine and thromboembolism. Front. Pharmacol. 11. doi: 10.3389/fphar.2020.587451
Bellesi, S., Metafuni, E., Hohaus, S., Maiolo, E., Marchionni, F., D'Innocenzo, S., et al. (2020). Increased CD95 (Fas) and PD-1 expression in peripheral blood T lymphocytes in COVID-19 patients. Br. J. Haematol. 191 (2), 207–211. doi: 10.1111/bjh.17034
Blasco, A., Coronado, M. J., Hernandez-Terciado, F., Martin, P., Royuela, A., Ramil, E., et al. (2020). Assessment of neutrophil extracellular traps in coronary thrombus of a case series of patients with COVID-19 and myocardial infarction. JAMA Cardiol. 6(4), 1–6. doi: 10.1001/jamacardio.2020.7308
Boldt, A., Borte, S., Fricke, S., Kentouche, K., Emmrich, F., Borte, M., et al. (2014). Eight-color immunophenotyping of T-, b-, and NK-cell subpopulations for characterization of chronic immunodeficiencies. Cytom. B Clin. Cytom. 86 (3), 191–206. doi: 10.1002/cyto.b.21162
Brinkmann, V., Reichard, U., Goosmann, C., Fauler, B., Uhlemann, Y., Weiss, D. S., et al. (2004). Neutrophil extracellular traps kill bacteria. Science 303 (5663), 1532–1535. doi: 10.1126/science.1092385
Chen, Y., Klein, S. L., Garibaldi, B. T., Li, H., Wu, C., Osevala, N. M., et al. (2021). Aging in COVID-19: Vulnerability, immunity and intervention. Ageing Res. Rev. 65, 101205. doi: 10.1016/j.arr.2020.101205
Chu, H., Zhou, J., Wong, B. H., Li, C., Chan, J. F., Cheng, Z. S., et al. (2016). Middle East respiratory syndrome coronavirus efficiently infects human primary T lymphocytes and activates the extrinsic and intrinsic apoptosis pathways. J. Infect. Dis. 213 (6), 904–914. doi: 10.1093/infdis/jiv380
Clark, S. R., Ma, A. C., Tavener, S. A., McDonald, B., Goodarzi, Z., Kelly, M. M., et al. (2007). Platelet TLR4 activates neutrophil extracellular traps to ensnare bacteria in septic blood. Nat. Med. 13 (4), 463–469. doi: 10.1038/nm1565
Connors, J. M., Levy, J. H. (2020). COVID-19 and its implications for thrombosis and anticoagulation. Blood 135 (23), 2033–2040. doi: 10.1182/blood.2020006000
Deftereos, S. G., Giannopoulos, G., Vrachatis, D. A., Siasos, G. D., Giotaki, S. G., Gargalianos, P., et al. (2020). Effect of colchicine vs standard care on cardiac and inflammatory biomarkers and clinical outcomes in patients hospitalized with coronavirus disease 2019: The GRECCO-19 randomized clinical trial. JAMA Netw. Open 3 (6), e2013136. doi: 10.1001/jamanetworkopen.2020.13136
Duijf, P. H. G. (2020). Low baseline pulmonary levels of cytotoxic lymphocytes as a predisposing risk factor for severe COVID-19. mSystems 5 (5), e00741-20. doi: 10.1128/mSystems.00741-20
Fox, S. E., Akmatbekov, A., Harbert, J. L., Li, G., Quincy Brown, J., Vander Heide, R. S. (2020). Pulmonary and cardiac pathology in African American patients with COVID-19: an autopsy series from new Orleans. Lancet Respir. Med. 8 (7), 681–686. doi: 10.1016/S2213-2600(20)30243-5
Fruchter, O., Yigla, M., Kramer, M. R. (2015). D-dimer as a prognostic biomarker for mortality in chronic obstructive pulmonary disease exacerbation. Am. J. Med. Sci. 349 (1), 29–35. doi: 10.1097/MAJ.0000000000000332
Fuchs, T. A., Brill, A., Duerschmied, D., Schatzberg, D., Monestier, M., Myers, D. D., Jr., et al. (2010). Extracellular DNA traps promote thrombosis. Proc. Natl. Acad. Sci. U.S.A. 107 (36), 15880–15885. doi: 10.1073/pnas.1005743107
Gao, Y., Li, T., Han, M., Li, X., Wu, D., Xu, Y., et al. (2020). Diagnostic utility of clinical laboratory data determinations for patients with the severe COVID-19. J. Med. Virol. 92 (7), 791–796. doi: 10.1002/jmv.25770
Gupta, S., Bi, R., Kim, C., Chiplunkar, S., Yel, L., Gollapudi, S. (2005). Role of NF-kappaB signaling pathway in increased tumor necrosis factor-alpha-induced apoptosis of lymphocytes in aged humans. Cell Death Differ. 12 (2), 177–183. doi: 10.1038/sj.cdd.4401557
Hoffmann, M., Kleine-Weber, H., Schroeder, S., Kruger, N., Herrler, T., Erichsen, S., et al. (2020). SARS-CoV-2 cell entry depends on ACE2 and TMPRSS2 and is blocked by a clinically proven protease inhibitor. Cell 181 (2), 271–280 e278. doi: 10.1016/j.cell.2020.02.052
Huang, K. J., Su, I. J., Theron, M., Wu, Y. C., Lai, S. K., Liu, C. C., et al. (2005). An interferon-gamma-related cytokine storm in SARS patients. J. Med. Virol. 75 (2), 185–194. doi: 10.1002/jmv.20255
Jimenez-Alcazar, M., Rangaswamy, C., Panda, R., Bitterling, J., Simsek, Y. J., Long, A. T., et al. (2017). Host DNases prevent vascular occlusion by neutrophil extracellular traps. Science 358 (6367), 1202–1206. doi: 10.1126/science.aam8897
Lippi, G., Bonfanti, L., Saccenti, C., Cervellin, G. (2014). Causes of elevated d-dimer in patients admitted to a large urban emergency department. Eur. J. Intern. Med. 25 (1), 45–48. doi: 10.1016/j.ejim.2013.07.012
Li, T., Qiu, Z., Zhang, L., Han, Y., He, W., Liu, Z., et al. (2004). Significant changes of peripheral T lymphocyte subsets in patients with severe acute respiratory syndrome. J. Infect. Dis. 189 (4), 648–651. doi: 10.1086/381535
Lominadze, D., Tsakadze, N., Sen, U., Falcone, J. C., D'Souza, S. E. (2005). Fibrinogen and fragment d-induced vascular constriction. Am. J. Physiol. Heart Circ. Physiol. 288 (3), H1257–H1264. doi: 10.1152/ajpheart.00856.2004
Manwaring, D., Curreri, P. W. (1981). Cellular mediation of respiratory distress syndrome induced by fragment d. Ann. Chir. Gynaecol. 70 (6), 304–307.
Manwaring, D., Curreri, P. W. (1982). Platelet and neutrophil sequestration after fragment d-induced respiratory distress. Circ. Shock 9 (1), 75–80.
Marshall, J. C. (2001). Inflammation, coagulopathy, and the pathogenesis of multiple organ dysfunction syndrome. Crit. Care Med. 29 (7 Suppl), S99–106. doi: 10.1097/00003246-200107001-00032
Massberg, S., Grahl, L., von Bruehl, M. L., Manukyan, D., Pfeiler, S., Goosmann, C., et al. (2010). Reciprocal coupling of coagulation and innate immunity via neutrophil serine proteases. Nat. Med. 16 (8), 887–896. doi: 10.1038/nm.2184
Middleton, E. A., He, X. Y., Denorme, F., Campbell, R. A., Ng, D., Salvatore, S. P., et al. (2020). Neutrophil extracellular traps contribute to immunothrombosis in COVID-19 acute respiratory distress syndrome. Blood 136 (10), 1169–1179. doi: 10.1182/blood.2020007008
Nicolai, L., Leunig, A., Brambs, S., Kaiser, R., Joppich, M., Hoffknecht, M. L., et al. (2021). Vascular neutrophilic inflammation and immunothrombosis distinguish severe COVID-19 from influenza pneumonia. J. Thromb. Haemost. 19 (2), 574–581. doi: 10.1111/jth.15179
Nicolai, L., Leunig, A., Brambs, S., Kaiser, R., Weinberger, T., Weigand, M., et al. (2020). Immunothrombotic dysregulation in COVID-19 pneumonia is associated with respiratory failure and coagulopathy. Circulation 142 (12), 1176–1189. doi: 10.1161/CIRCULATIONAHA.120.048488
Ouwendijk, W. J. D., Raadsen, M. P., van Kampen, J. J. A., Verdijk, R. M., von der Thusen, J. H., Guo, L., et al. (2021). High levels of neutrophil extracellular traps persist in the lower respiratory tract of critically ill patients with coronavirus disease 2019. J. Infect. Dis. 223 (9), 1512–1521. doi: 10.1093/infdis/jiab050
Pera, A., Campos, C., Lopez, N., Hassouneh, F., Alonso, C., Tarazona, R., et al. (2015). Immunosenescence: Implications for response to infection and vaccination in older people. Maturitas 82 (1), 50–55. doi: 10.1016/j.maturitas.2015.05.004
Piazza, G., Campia, U., Hurwitz, S., Snyder, J. E., Rizzo, S. M., Pfeferman, M. B., et al. (2020). Registry of arterial and venous thromboembolic complications in patients with COVID-19. J. Am. Coll. Cardiol. 76 (18), 2060–2072. doi: 10.1016/j.jacc.2020.08.070
Qin, C., Zhou, L., Hu, Z., Zhang, S., Yang, S., Tao, Y., et al. (2020). Dysregulation of immune response in patients with coronavirus 2019 (COVID-19) in wuhan, China. Clin. Infect. Dis. 71 (15), 762–768. doi: 10.1093/cid/ciaa248
Ross, S. H., Cantrell, D. A. (2018). Signaling and function of interleukin-2 in T lymphocytes. Annu. Rev. Immunol. 36, 411–433. doi: 10.1146/annurev-immunol-042617-053352
Snijders, D., Schoorl, M., Schoorl, M., Bartels, P. C., van der Werf, T. S., Boersma, W. G. (2012). D-dimer levels in assessing severity and clinical outcome in patients with community-acquired pneumonia. a secondary analysis of a randomised clinical trial. Eur. J. Intern. Med. 23 (5), 436–441. doi: 10.1016/j.ejim.2011.10.019
Soomro, A. Y., Guerchicoff, A., Nichols, D. J., Suleman, J., Dangas, G. D. (2016). The current role and future prospects of d-dimer biomarker. Eur. Heart J. Cardiovasc. Pharmacother. 2 (3), 175–184. doi: 10.1093/ehjcvp/pvv039
Tang, N., Bai, H., Chen, X., Gong, J., Li, D., Sun, Z. (2020). Anticoagulant treatment is associated with decreased mortality in severe coronavirus disease 2019 patients with coagulopathy. J. Thromb. Haemost. 18 (5), 1094–1099. doi: 10.1111/jth.14817
Tay, M. Z., Poh, C. M., Renia, L., MacAry, P. A., Ng, L. F. P. (2020). The trinity of COVID-19: immunity, inflammation and intervention. Nat. Rev. Immunol. 20 (6), 363–374. doi: 10.1038/s41577-020-0311-8
Tomazini, B. M., Maia, I. S., Cavalcanti, A. B., Berwanger, O., Rosa, R. G., Veiga, V. C., et al. (2020). Effect of dexamethasone on days alive and ventilator-free in patients with moderate or severe acute respiratory distress syndrome and COVID-19: The CoDEX randomized clinical trial. JAMA 324 (13), 1307–1316. doi: 10.1001/jama.2020.17021
van den Broek, T., Borghans, J. A. M., van Wijk, F. (2018). The full spectrum of human naive T cells. Nat. Rev. Immunol. 18 (6), 363–373. doi: 10.1038/s41577-018-0001-y
Vomero, M., Barbati, C., Colasanti, T., Celia, A. I., Speziali, M., Ucci, F. M., et al. (2020). Autophagy modulation in lymphocytes from COVID-19 patients: New therapeutic target in SARS-COV-2 infection. Front. Pharmacol. 11. doi: 10.3389/fphar.2020.569849
Wang, F., Nie, J., Wang, H., Zhao, Q., Xiong, Y., Deng, L., et al. (2020). Characteristics of peripheral lymphocyte subset alteration in COVID-19 pneumonia. J. Infect. Dis. 221 (11), 1762–1769. doi: 10.1093/infdis/jiaa150
Wang, Y., van Boxel-Dezaire, A. H., Cheon, H., Yang, J., Stark, G. R. (2013). STAT3 activation in response to IL-6 is prolonged by the binding of IL-6 receptor to EGF receptor. Proc. Natl. Acad. Sci. U.S.A. 110 (42), 16975–16980. doi: 10.1073/pnas.1315862110
Wells, P. S., Anderson, D. R., Rodger, M., Stiell, I., Dreyer, J. F., Barnes, D., et al. (2001). Excluding pulmonary embolism at the bedside without diagnostic imaging: management of patients with suspected pulmonary embolism presenting to the emergency department by using a simple clinical model and d-dimer. Ann. Intern. Med. 135 (2), 98–107. doi: 10.7326/0003-4819-135-2-200107170-00010
Wu, Z., McGoogan, J. M. (2020). Characteristics of and important lessons from the coronavirus disease 2019 (COVID-19) outbreak in China: Summary of a report of 72314 cases from the Chinese center for disease control and prevention. JAMA 323 (13), 1239–1242. doi: 10.1001/jama.2020.2648
Xu, Z., Shi, L., Wang, Y., Zhang, J., Huang, L., Zhang, C., et al. (2020). Pathological findings of COVID-19 associated with acute respiratory distress syndrome. Lancet Respir. Med. 8 (4), 420–422. doi: 10.1016/S2213-2600(20)30076-X
Yang, L. T., Peng, H., Zhu, Z. L., Li, G., Huang, Z. T., Zhao, Z. X., et al. (2006). Long-lived effector/central memory T-cell responses to severe acute respiratory syndrome coronavirus (SARS-CoV) s antigen in recovered SARS patients. Clin. Immunol. 120 (2), 171–178. doi: 10.1016/j.clim.2006.05.002
Yao, X. H., Li, T. Y., He, Z. C., Ping, Y. F., Liu, H. W., Yu, S. C., et al. (2020). A pathological report of three COVID-19 cases by minimal invasive autopsies. Zhonghua Bing Li Xue Za Zhi 49 (5), 411–417. doi: 10.3760/cma.j.cn112151-20200312-00193
Zhang, L., Yan, X., Fan, Q., Liu, H., Liu, X., Liu, Z., et al. (2020). D-dimer levels on admission to predict in-hospital mortality in patients with covid-19. J. Thromb. Haemost. 18 (6), 1324–1329. doi: 10.1111/jth.14859
Keywords: COVID-19, D-dimer, lymphocyte, D-dimer to lymphocyte ratio, mortality, immunothrombosis
Citation: Peng F, Yi Q, Zhang Q, Deng J, Li C, Xu M, Wu C, Zhong Y and Wu S (2022) Performance of D-dimer to lymphocyte ratio in predicting the mortality of COVID-19 patients. Front. Cell. Infect. Microbiol. 12:1053039. doi: 10.3389/fcimb.2022.1053039
Received: 24 September 2022; Accepted: 28 November 2022;
Published: 15 December 2022.
Edited by:
Santiago J. Ballaz, Yachay Tech University, EcuadorReviewed by:
Manish Sharma, Emory University, United StatesAtakan Yilmaz, Pamukkale University, Turkey
Copyright © 2022 Peng, Yi, Zhang, Deng, Li, Xu, Wu, Zhong and Wu. This is an open-access article distributed under the terms of the Creative Commons Attribution License (CC BY). The use, distribution or reproduction in other forums is permitted, provided the original author(s) and the copyright owner(s) are credited and that the original publication in this journal is cited, in accordance with accepted academic practice. No use, distribution or reproduction is permitted which does not comply with these terms.
*Correspondence: Yanjun Zhong, emhvbmd5YW5qdW5AY3N1LmVkdS5jbg==; Shangjie Wu, d3VzaGFuZ2ppZUBjc3UuZWR1LmNu