- 1Department of Laboratory Medicine, the First Medical Centre, Chinese People's Liberation Army (PLA) General Hospital, Beijing, China
- 2Department of Respiratory and Critical Care, Beijing Shijitan Hospital, Capital Medical University, Peking University Ninth School of Clinical Medicine, Beijing, China
- 3School of Clinical Medicine, Weifang Medical University, Weifang, China
Cryptococcus is an invasive fungus that seriously endangers human life and health, with a complex and well-established immune-escaping mechanism that interferes with the function of the host immune system. Cryptococcus can attenuate the host’s correct recognition of the fungal antigen and escape the immune response mediated by host phagocytes, innate lymphoid cells, T lymphocytes, B lymphocytes with antibodies, and peripheral cytokines. In addition, the capsule, melanin, dormancy, Titan cells, biofilm, and other related structures of Cryptococcus are also involved in the process of escaping the host’s immunity, as well as enhancing the ability of Cryptococcus to infect the host.
1 Introduction
The Cryptococcus genus is affiliated with Basidiomycota, Agaricmycotina, Tremelloymcetes, and Tremellales (Heitman et al., 2010), and is a group of capsule-covered opportunistic pathogenic fungi. Of the 37 species known, Cryptococcus neoformans (Cn) and Cryptococcus gattii (Cg) are the main human pathogenic cryptococci (Franco-Paredes et al., 2015). Cn is widespread in nature, often originating from pigeon feces, and mainly infects immunocompromised individuals, such as the elderly, HIV/AIDS patients, and organ transplant recipients, as well as often causing central nervous system infections (NYu et al., 2021). It is estimated that 223,000 people with HIV/AIDS develop cryptococcal meningitis each year, of which 181,000 die (Rajasingham et al., 2017). Cg was initially thought to be transmitted only in tropical and subtropical eucalyptus areas; there has been a steady increase in the number of Cg infections in the world in temperate regions since the outbreak on Vancouver Island. Cg can infect both immunocompetent and immunosuppressed people, and has a mortality rate of nearly 33% (Jamil et al., 2020). Cryptococcus has become a lethal pathogen that poses a serious threat to public health safety (Jamil et al., 2020).
The host immune system has established a large and complex immune mechanism in response to invasion by Cryptococcus (reviewed in Hernández-Chávez et al., 2017). Cryptococci invade the host alveoli via the respiratory tract in the form of fungi or fungal spores, and are then exposed to the host immune response with phagocytes as the first barrier. The pattern recognition receptors (PRRs) of alveolar phagocytes recognize the conserved pathogen-associated molecular patterns (PAMPs) of Cryptococcus and mediate the formation of phagosomes by macrophages, dendritic cells, neutrophils, etc. (Hatinguais et al., 2020). In addition, phagocytes can secrete large amounts of cytokines and chemokines, and can act as antigen-presenting cells (APCs) to present antigens to T lymphocytes for the clearance of cryptococci through cellular and humoral immunity (reviewed in Li et al., 2019).
However, Cryptococcus has also evolved a targeted and well-established immune escape capability from being identified and killed (reviewed in Hernández-Chávez et al., 2017). In this review, we focus on and determine the mechanisms of Cryptococcus immune escaping, as well as the fungal structure associated with Cryptococcus escaping immunity.
2 Mechanisms by which Cryptococcus escapes host immunity
2.1 Immune escape of host cellular immunity
2.1.1 Immune escape of phagocytes
2.1.1.1 Immune escape of macrophages
Macrophages that infiltrate in large numbers into the alveoli are the first line of defense of the host immune system against cryptococci (Trevijano-Contador et al., 2016). The immune process of macrophages against Cryptococcus includes capsule polysaccharide recognition, phagocytosis, killing, cytokine and chemokine production, and antigen presentation (Barreto-Bergter and Figueiredo, 2014; Trevijano-Contador et al., 2016). Previous studies have suggested that the phagocytic process of Cryptococcus is mostly dependent on opsonin, including antibody and complement opsonization, such as antibody receptor in the Fc region against the capsule antigen component glucuronoxylomannan (GXM) in addition to the capsule-dependent C3-C3b complement system, which together complete the phagocytosis and internalization of Cryptococcus (reviewed in Rohatgi and Pirofski, 2015; Sun et al., 2019). However, recent studies have shown that macrophages can also phagocytose pathogens in a non-opsonic manner, i.e., through the direct recognition of fungal cell wall PAMPs by PRRs (Sun et al., 2019). In contrast to the “mannoprotein receptor-mannoprotein” recognition mode of other fungi, macrophages rely on the spleen tyrosine kinase pathway to recognize Cryptococcus, in which Cn can be recognized by both Dectin-1 and Dectin-2 receptors, whereas Cg is only recognized by Dectin-1 receptors, and the difference is determined by the different PAMPs in the cell walls of the two species (Lim et al., 2018) (Figure 1).
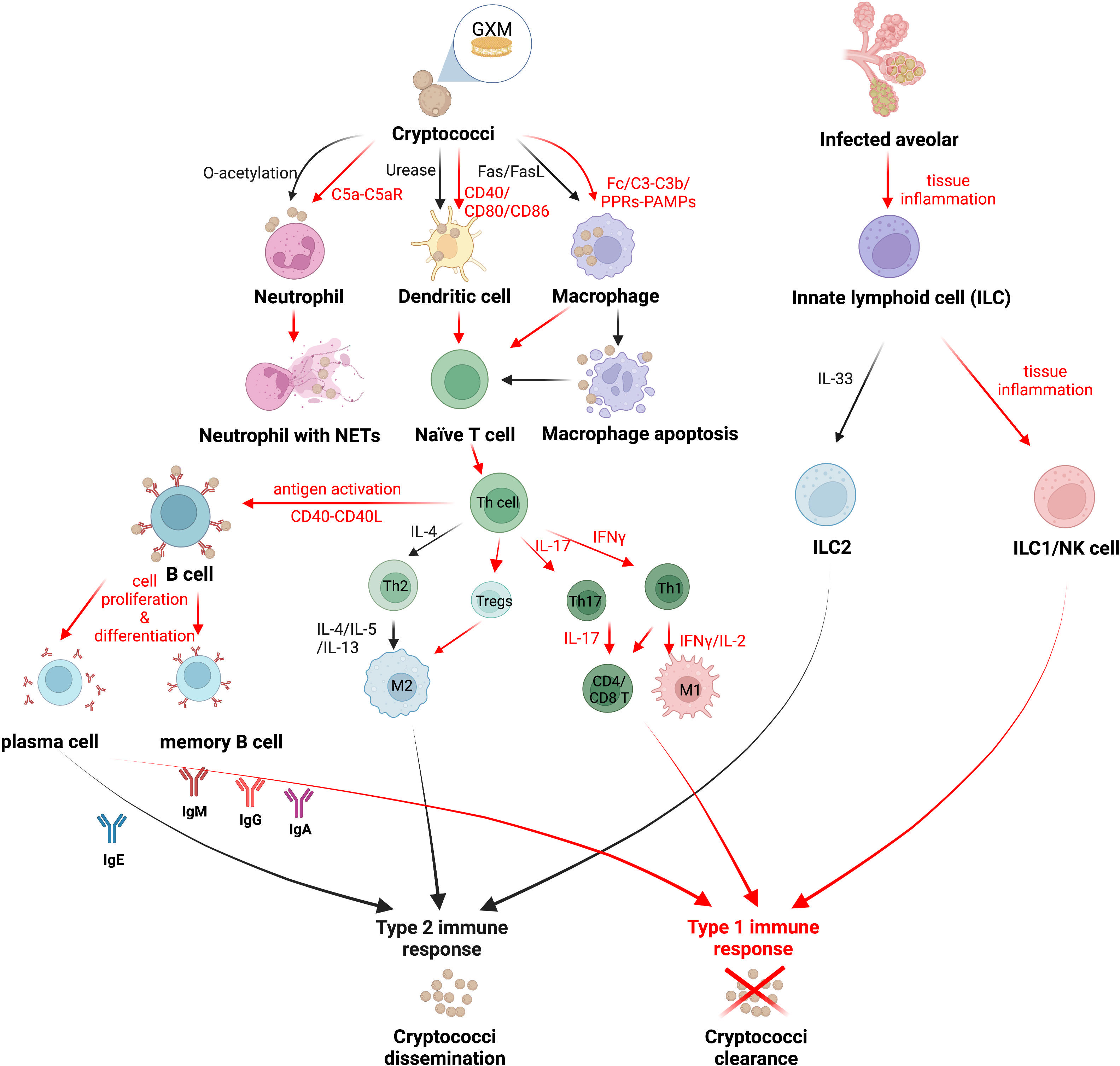
Figure 1 The strategy of Cryptococcus immune escaping. Cryptococcus can attenuate the host’s correct recognition of the fungal antigen components and escape the immune response mediated by host phagocytes. Red arrows indicate the mechanism by which the host immune system clears Cryptococcus, and black arrows indicate the mechanism by which Cryptococcus escapes host immunity. Created in BioRender.com.
The activation state of macrophages includes the classically activated M1 type, which is protective of the host, and the alternatively activated M2 type, which is nonprotective. M1/M2 macrophages can be interconverted by immediate changes in cytokines in the immune microenvironment (Davis et al., 2013; Subramani et al., 2020). M1 macrophages are activated by IFN-γ produced by type 1 immune responses via the signal transduction and activator of transcription-1 (STAT1) signaling pathway, which in turn produces reactive oxygen species (ROS) and reactive nitrogen species (RNS) to kill pathogenic bacteria (Leopold Wager and Wormley, 2014). M2 macrophages, in turn, are activated by IL-4 and IL-13 of type 2 immune responses with the induction of Arg1 and CD206 (mannose receptor) expression (Leopold Wager and Wormley, 2014; Subramani et al., 2020). Furthermore, Müller U (Müller et al., 2012) et al. demonstrated that the interleukin-4 receptor (IL-4R) on T helper (Th) cells suppressed host resistance in pulmonary cryptococcosis with an enhancing type 2 immunity. Cryptococci cannot be eliminated by M2 macrophages; instead, cryptococci can grow, multiply, and spread within M2 macrophages or even go dormant in preparation for latent infection (Subramani et al., 2020). Interestingly, cryptococci were proved to weaken the fungicidal ability of inflammatory monocytes (IM) and aggravate the progression of infection, which is independent of lymphocyte priming, eosinophil recruitment, or downstream M2 macrophage polarization pathways (Heung and Hohl, 2019). Cryptococcus can escape from M1 macrophages by inducing the polarization of macrophages towards the M2 phenotype via the Hsp 70 homolog Ssa1 (Eastman et al., 2015a). Recent research has revealed that macrophages, while maximizing the killing of Cryptococcus, are also “accomplices” in facilitating the immune escape of Cryptococcus. Sabiiti W (Sabiiti et al., 2014) et al. found that macrophages provide an ecological niche for Cryptococcus to replicate intracellularly, which limits the effect of extracellular antifungal drugs, as well as the fact that Cryptococcus crosses the blood-brain barrier to enter the brain via macrophages as a Trojan horse transmission (Santiago-Tirado et al., 2017). Interventional reductions in host macrophages have even been shown to reduce the risk of Cryptococcus spreading in vivo (Charlier et al., 2009).
It has been reported that the phagocytosed cryptococcal capsule component GXM may induce the apoptosis of macrophages mediated by the Fas/FasL pathway and inhibite subsequent immune activation (Villena et al., 2008). At the same time, Cryptococcus has evolved a way of escaping macrophages via vesicle encapsulation, known as nonlytic exocytosis, or “vomocytosis”, which not only assists Cryptococcus in invading the central nervous system but also protects Cryptococcus from the immune system during the incubation period (Santiago-Tirado et al., 2017).
2.1.1.2 Immune escape of dendritic cells
DCs are densely distributed in the host’s respiratory mucosa and serve as a bridge between innate and adaptive immune responses, with their main function being the uptake and presentation of pathogenic antigens (Trombetta and Mellman, 2005; reviewed in Mukaremera and Nielsen, 2017). Similar to macrophages, opsonization by antibodies or complements in the immune environment enhances phagocytosis and the killing of cryptococci by DCs (Campuzano and Wormley, 2018). DCs are induced to mature by costimulatory molecules (CD40, CD80, and CD86, etc.) and migrate to T-cell-rich lymphoid organs, where the major histocompatibility complex class II (MHC-II) on their surface presents the processed antigenic component to the initial T cells, namely mannoprotein (MP), initiating the subsequent T lymphocyte immune response. DCs engulf cryptococci and recruit lysosomes to fuse with them, which are independently segregated within the phagocytosed lysosomes and killed by oxidative and nonoxidative mechanisms, while cathepsin B forms pores in the cryptococci cell wall and accelerates cryptococci lysis (Nelson et al., 2021).
As a heterogeneous population, DCs comprise different subtypes, such as CD11b+ myeloid DCs, plasmacytoid DCs (pDCs), and CD103+ DCs (Condon et al., 2011; Nelson et al., 2020). During cryptococcal infection, CD11b+ DCs migrate to the lung and present antigens to cryptococcal-specific T cells, which is of significance for the clearance of cryptococci (Wozniak et al., 2006; Osterholzer et al., 2009a). The recognition and uptake of Cryptococcus by pDCs rely on the expressive level of Dectin-3 and the chemokine receptor CXCR3, and the fungicidal activity of pDCs depends on the production of ROS within the lysosome (Hole et al., 2016). CD103+ DCs infiltrate into the lung mucosa and distribute along the lung vascular wall; however, the CD103+ DCs population occupied a very small proportion of the total DCs (Eastman et al., 2015b; Nelson et al., 2020). Studies on CD103+ DCs in response to pulmonary cryptococcosis deserve more attention (Nelson et al., 2020).
Activated by IL-12, a protective Th1-type cytokine produced by mature DCs in the presence of IFN-γ, Th1 cells possess a positive interrelationship with DCs, together activate the inflammatory response against Cryptococcus and enhance host protection (Vieira et al., 2000; reviewed in Mukaremera and Nielsen, 2017), whereas the nonprotective DCs expressing the costimulatory molecules CD86 and OX40L induced Th2 cells to secrete IL-4, IL-5, and IL-13, and recruit eosinophils to participate in the anti-inflammatory response, enhancing the immune escape of Cryptococcus from the host (Ohshima et al., 1998; reviewed in Mukaremera and Nielsen, 2017). Cryptococci were found to evade immune surveillance by DCs through the virulence factor urease, which impedes the maturation of DCs and inhibits their antigen presentation role (Osterholzer et al., 2009b). While the O-acetyl groups in the GXM of the capsule assist DCs in recognizing cryptococci, the deacetylation of an O-acetyl group in the GXM structure of Cg further limits the immune recognition function of DCs compared to Cn, making Cg more immune-evasive from host clearance (Urai et al., 2016) (Figure 1).
In addition, Cg phagocytosed by DCs forms a persistent actin cage at the periphery of the phagosome, a cage-like structure that spatially and functionally effectively prevents the fusion of phagosomes with lysosomes as well as inhibiting the formation of phagolysosomes and the further immune activation of DCs, leading to the immune paralysis of Cg by DCs. This unique immune evasion mechanism may be an important reason for the highly virulent phenotype of Cg, and partially explains its ease of infection of immunocompetent hosts (Jamil et al., 2020).
2.1.1.3 Immune escape of neutrophils
Neutrophils are one of the key immune mechanisms for host resistance to cryptococcal infection, and can be rapidly recruited to the site of infection in response to chemokines released by the pathogen or host cells. The augmentation of neutrophil fungicidal activity by stimulation of granulocyte colony stimulating factor (G-CSF) and granulocyte-macrophage colony-stimulating factor (GM-CSF) remarkably reduced the fungal burden and prolonged the survival time (Chiller et al., 2002). The recruitment of neutrophils to Cryptococcus requires the activation of the complement C5a-C5aR pathway, as well as the activation of the mitogen-activated protein kinase (MAPK) system of extracellular signal-regulated kinases (ERK) and p38, resulting in the production of large amounts of proinflammatory cytokines, including high concentrations of IL-4, IL-10, IL-12, and TNF-α (Sun et al., 2015). Leukotriene B4 (LTB4), a kind of eicosanoid produced by the expression of complement C3 and CD11b, was quickly released by neutrophils during interactions with cryptococci, motivating large amounts of neutrophils to the site of infection, swarming and engulfing cryptococci (Sun and Shi, 2016). Neutrophils also form neutrophil extracellular traps (NETs) to trap cryptococci, and NETs limit the retaining and killing of cryptococci just within their own traps, so as to prevent excessive inflammatory factors associated with these traps from causing damage to the body (Springer et al., 2010; reviewed in Hernández-Chávez et al., 2017) (Figure 1).
However, it was found that although cryptococcal capsule GXM has chemotactic activity against neutrophils, the O-acetyl groups in it inhibit neutrophil migration and phagocytosis in addition to even restricting the formation of NETs, enhancing the ability of Cryptococcus to escape the immune effects of the host (Rocha et al., 2015; reviewed in Campuzano and Wormley, 2018). In addition, GXM induces the shedding of L-selectin and TNF-α receptors on the surface of neutrophils, thereby reducing neutrophil adhesion to the endothelial surface of blood vessels and preventing their migration to the site of infection, which explains the reduced infiltration of neutrophils into host tissues infected with disseminated cryptococci (Ellerbroek et al., 2004).
2.1.2 Immune escape of innate lymphoid cells
Innate lymphoid cells (ILCs), a vital part of the innate immune system, are stemmed from lymphoid lineages lacking PRRs (Spits et al., 2013). Although ILCs fail to recognize antigens specifically, they contribute immediately in tissue homeostasis, pathological inflammation, and immunity against infections through cytokine stimulation bringing about direct subsequent innate and adaptive immune responses (Elemam et al., 2021). ILCs are generally classified into three major subgroups of innate lymphoid cells: group 1 (ILC1s), including natural killer (NK) cells, group 2 (ILC2s), and group 3 (ILC3s) (Spits et al., 2013). ILC1s drive type 1 immune responses which respond to intracellular pathogenic bacteria and fungi with the secretion of IFN-γ and TNF (Elemam et al., 2021). NK cells play crucial roles in fungi clearance in various ways, including cytokine production (IFN-γ, GM-CSF, and TNF-α) and the secretion of cytotoxic molecules secretion (perforin, granzymes, and granulysin) (Elemam et al., 2021). Granulysin participates in interference with oxidative metabolism and the energy generation of fungi, and it can also directly kill extracellular pathogens by altering the membrane integrity, while further decreasing the viability of intracellular pathogens when combined with perforin (Elemam et al., 2021). ILC2s mediate type 2 immune responses producing Th2 cytokines, including IL-4, IL-5, and IL-13 (Elemam et al., 2021). ILC3s are found to act as equivalents of Th17 cells, and mainly maintain the microbiota-host homeostasis of intestinal immunity (Elemam et al., 2021).
Recent studies have illustrated that the activation of ILC1s and M1-type macrophages strongly restricts Cn infections during cryptococcosis, whereas type 2 immunity plays a reverse role in disease progression that exacerbates pulmonary infections with ILC2s as well as M2-type macrophages (Kindermann et al., 2020). Activated by the stimulation of alarmin IL-33, ILC2s were shown to exert high amounts of type 2 cytokines, which modulate mucus hyperproduction, eosinophilia, and the activation of alternatively activated macrophages (M2) in infected organs (Kabata et al., 2018; Kindermann et al., 2018). Furthermore, it was demonstrated that highly virulent Cryptococcus strains induced type 2 immunity significantly, including ILC2s induction, by triggering the alarmin IL-33 in alveolar type 2 epithelial cells (Flaczyk et al., 2013; Heyen et al., 2016; Knipfer et al., 2019; Kindermann et al., 2020). Remarkably, ILC2s suppress pulmonary type 1 immunity and classical macrophage (M1) activation, resulting in attenuated fungal control, suggesting a detrimental role of ILC2s during cryptococcosis (Kindermann et al., 2020) (Figure 1).
2.1.3 Immune escape of T lymphocyte
2.1.3.1 Immune escape of effector T lymphocytes
T-lymphocyte-mediated immune responses contributed greatly against Cryptococcus, as phagocytes process cryptococcal antigens and present them to T lymphocytes, inducing the initial T cells to proliferate and mature as well as differentiate into various subtypes (Elsegeiny et al., 2018). Studies have shown that both effector CD4+ T cells and CD8+ T cells are involved in the immune response to Cryptococcus, and both proliferate in large numbers as well as produce granulysin to kill cryptococci (Zheng et al., 2007). Pulmonary infiltrating effector T lymphocytes stimulated by cryptococcal antigens secrete both protective Th1-type (IFN-γ, IL-2) and Th17-type (IL-17) cytokines, as well as nonprotective Th2-type (IL-4, IL-5) cytokines (reviewed in Mukaremera and Nielsen, 2017; Mills, 2022). Both CD4+ T and CD8+ T cells produce anticryptococcal Th1-type and Th17-type cytokines, whereas Th2-type cytokines are only produced by CD4+ T cells (Mills, 2022). It was found that Cryptococcus was not sitting still and that capsule GXM prompted IL-4 and IL-10 secretion while inhibiting TNF-α and IFN-γ production, enhancing the ability of Cryptococcus to escape the killing effects of CD4+ T and CD8+ T cells (Scriven et al., 2016) (Figure 1). IL-4R was proven to be a major determinant affecting the host susceptibility to cryptococcosis, which is expressed on Th cells via Th2 responses (Müller et al., 2012). IL-4R complexes can bind to the IL-4 and IL-13 involved in Th2 responses, suppressing host resistance in pulmonary cryptococcosis with an enhancing type 2 immunity. Conversely, lacking IL-4R on Th cells shows a protective classically activated macrophages (M1 macrophages) response and controls the pulmonary cryptococcosis (Müller et al., 2012).
In addition, immunocompetent CD4+ T cells also cause the host to develop immune reconstitution inflammatory syndrome (IRIS) and postinfectious inflammatory response syndrome (PIIRS), which severely disrupt the central nervous system (CNS) (Shourian and Qureshi, 2019). Neal et al. (2017) found that although CD4+ T cells significantly reduced the fungal load of target organs, more than half of the mice died from neurological dysfunction in the late stages of infection. This explains in part the high morbidity and mortality associated with CNS cryptococcal infection in immunocompetent patients. Cryptococcus causes an immune overreaction in the host that disrupts the immune homeostasis and triggers a lethal inflammatory response.
2.1.3.2 Immune escape of other subtypes of T lymphocytes
Regulatory T cells (Tregs) are one of the CD4+ T cell subtypes that negatively regulate the host immune response during most fungal infections (Wiesner et al., 2016). When infected with Histoplasma and Candida albicans, fungal clearance is promoted with increasing proinflammatory cytokines and a reduction in Tregs. However, Tregs were discovered to suppress the nonprotective Th2 immune response during infections with Pneumocystis and Cryptococcus. With the assistance of the C-C chemokine receptor type 5 (CCR5) and IFN regulatory factor 4 (IRF4) in cryptococci-infected lungs, Tregs are able to localize the infected sites and subsequently suppress Th2 effector cells subsequently (Schulze et al., 2016; Wiesner et al., 2016). During pulmonary cryptococcal infection, elevating Tregs lead to limit Cryptococcus-infection-induced allergic airway inflammation by reducing IgE, mucus production, and Th2 cytokine production, while not altering the cryptococci burden (reviewed in Schulze et al., 2016; Mukaremera and Nielsen, 2017) (Figure 1).
Natural killer T (NKT) cells, especially Vα14+ NKT cells, activated by cryptococcal lipid antigens presentation via DCs and recruited to the lung by monocyte chemoattractant protein-1 (MCP-1) chemokines (Kawakami et al., 2001), play an important role in inducing a protective Th1 immune response. Further studies on Vα14+ NKT cells have revealed that, when activated by α-galactosylceramide, Vα14+ NKT cells induced Th1 immune responses with IFN-γ synthesis during Cryptococcus infection. At the same time, NKT cells also cause delayed-type hypersensitivity in the host and a local allergic inflammation in the lung, even necrosis (Sato et al., 2020).
γδ T cells are also activated by cryptococcal antigens presented by macrophages and DCs, while MCP-1 is not required during the accumulation. Notably, γδ T cells inhibit IFN-γ synthesis and downregulate protective Th1 immune responses, which impede host immunity towards cryptococci (Wiesner et al., 2016; reviewed in Mukaremera and Nielsen, 2017). The various subtypes of T lymphocytes in the host are functionally interlocked, allowing the opportunity for Cryptococcus to escape host immune clearance.
2.2 Immune escape of host humoral immunity
The humoral immune responses against cryptococci are mainly mediated by B lymphocytes and antibodies. B-1 cells, a subtype of mature B cells, play a crucial role in enhancing resistance to and preventing the dissemination of cryptococci. Antibodies produced against cryptococcal proteins and capsule GXM are detected in the sera of the vast majority of children, suggesting that humans can develop humoral immune responses to environmental exposure to cryptococci at an early age (Goldman et al., 2001). B-1 cells from hosts infected with Cryptococcus secrete cryptococci-binding IgM, the depletion of which would result in reduced alveolar macrophages phagocytosis and increased fungal dissemination to the brain (Rohatgi and Pirofski, 2012). IgM suppresses pulmonary cryptococcal load and reduces host susceptibility to cryptococci, as well as limiting cryptococcal Titan cell formation (García-Barbazán et al., 2016; Trevijano-Contador et al., 2020), while IgG antibodies enhance the clearance of cryptococci by splenic NK cells (Nabavi and Murphy, 1986; reviewed in Mukaremera and Nielsen, 2017). The levels of serum GXM-binding IgM and IgG are far lower in HIV-infected than HIV-uninfected individuals, as well as in HIV-uninfected organ transplant recipients infected with Cryptococcus than those who are not (Jalali et al., 2006). It has also been shown that B lymphocytes and antibodies prevent systemic infection with Cryptococcus when T lymphocyte immune function is impaired (Aguirre and Johnson, 1997; reviewed in Mukaremera and Nielsen, 2017).
Moreover, the antibodies also act as opsonins to enhance the phagocytic uptake of cryptococci (Aslanyan et al., 2017). Anti-β-glucan monoclonal antibodies and anti-GXM monoclonal antibodies, which bind to the cryptococcal cell wall and capsule separately, enhance the macrophage killing of cryptococci in vitro (Torosantucci et al., 2009; Probert et al., 2019). The prophylactic infusion of anti-Cryptococcus antibodies can also control the fungal load of target organs and reduce the damage caused by cryptococcal infection (Torosantucci et al., 2009).
In a previous review, antibodies such as IgM, IgG, and IgA were attributed to protect hosts against Cryptococcus (reviewed in Mukaremera and Nielsen, 2017). However, diverse studies have revealed that the protective efficacy of antibodies against cryptococci is dependent on not only antibodies’ classification, but also their specificity. Two clonally related immunoglobulin M (IgM) monoclonal antibodies (MAbs) (12A1 and 13F1) differ in specificity and protective efficacy, presumably due to variable (V)-region sequence differences. MAb 12A1 is protective in Cryptococcus infection, while MAb 13F1 is nonprotective (Nakouzi et al., 2001). As for IgGs sharing identical V regions, IgG1, IgG2a, and IgG2b MAbs to the capsular glucuronoxylomannan of Cryptococcus prolong the host survival, while IgG3 displays a nonprotective role in enhancing infection (Beenhouwer et al., 2001). IgA mediates the complement-independent phagocytosis of Cryptococcus by macrophages with the assistance of complement receptor 3 (CR3) expression, promoting protective efficacy in hosts (Taborda and Casadevall, 2002). Furthermore, increased IgE is associated with a nonprotective type 2 immune response that exacerbates the Cryptococcus infection (Zaragoza et al., 2007; Qiu et al., 2013) (Figure 1). These results suggest that antibody-mediated immunity against cryptococcal infection is a complex process and that cryptococci can survive within the host humoral immune environment through an immune escape effect.
2.3 Immune escape of host cytokine-mediated immune responses
Cytokines are small molecular proteins that mediate the interactions and signaling functions between different immune cells. During cryptococcal infection, protective and nonprotective cytokines are produced by the host.The main protective cytokines include IFN-γ, IL-12, and IL-2, all of which enhance the macrophage expression of ROS in addition to RNS phenotypes and reduce the target organ fungal load, while decreasing host susceptibility (Wang et al., 2015; Firacative et al., 2018). In addition, a class of supporting cytokines are involved in the induction of Th1-type immune responses, including TNF-α, IL-6, IL-8, IL-18, IL-23, and IP10. Of these, TNF-α, IL-6, IL-8 and IP10 are associated with prognoses in patients with HIV/AIDS cryptococcal meningitis (Jarvis et al., 2015; Mora et al., 2015) and IL-6 is also involved in anticryptococcal drug resistance (Blasi et al., 1995).
Nonprotective cytokines are usually produced by Th2-type immune responses, mainly IL-4, IL-5, and IL-13, all of which induce increased lung fungal load and lung eosinophil production, as well as enhanced host susceptibility (Wiesner et al., 2015; Liu et al., 2020). In addition, high levels of IL-13 in cerebrospinal fluid are positively associated with high morbidity and mortality in patients infected with HIV/AIDS cryptococcal meningitis (Scriven et al., 2015). Th1-type and Th2-type immune responses are supposed to be some of the mechanisms by which the body maintains immune homeostasis, and cryptococci break this homeostasis by suppressing Th1-type and enhancing Th2-type immune responses, escaping host immune surveillance and killing.
In addition, there is a specific nonprotective cytokine-related immune response known as immune reconstitution inflammatory syndrome (IRIS), a pathological inflammation that results from an over-recovery of the body’s immune response following antiretroviral therapy in patients with HIV/AIDS cryptococcal meningitis (Eschke et al., 2015). Studies have confirmed that multiple cytokines are associated with cryptococcal IRIS, including serum proinflammatory cytokines such as TNF-α, granulocyte colony-stimulating factor (G-CSF), granulocyte-macrophage colony-stimulating factor (GM-CSF) and vascular endothelial growth factor (VEGF) (Brienze et al., 2021) (Table 1).
3 Fungal structure and corresponding immune escape mechanisms associated with Cryptococcus escaping host immunity
3.1 Cryptococcal fungal structure and immune escape
3.1.1 Immune escape effect of the capsule
Polysaccharide capsules (PC) are the outermost protective structure of Cryptococcus, activating and participating throughout the host immune response, including phagocytic uptake, antigen presentation, antibody production, and mediating the production of functionally diverse cytokines (O'Meara and Alspaugh, 2012; reviewed in Rohatgi and Pirofski, 2015). PC is composed of 90%-95% GXM, 5% galactoxylomannan (GalXM), and less than 1% mannoprotein (Reuwsaat et al., 2018). As the most important virulence factor of Cryptococcus, the capsule is also the most important immune-escape-related structure of Cryptococcus. In the host, the capsule has multiple self-protective functions, including inhibiting macrophage phagocytosis, reducing the antigen presentation capacity of APCs, downregulating inflammatory cytokine levels, and depleting complement components, thereby attenuating the host immune response (O'Meara and Alspaugh, 2012; Denham et al., 2018; Colombo et al., 2019; Freitas and Santos, 2021). Additionally, once in macrophages, the capsule can also resist killing by host ROS and RNS (Naslund et al., 1995; Casadevall et al., 2019). The capsule can actively sense the immune environment, induce Titan cell formation, and even drive the fungi into dormancy, and its major component, GXM, can induce the apoptosis of macrophages mediated by the Fas/FasL pathway, inhibiting subsequent activation of immune function in addition to maximizing the protection of Cryptococcus from attack and clearance by the host immune system (Santiago-Tirado et al., 2017). Moreover, GalXM contributes to immune escaping by inducing apoptosis of T cells, which attributes to the caspase 8 activation and the consequent DNA fragmentation (Pericolini et al., 2006). Denham (Denham et al., 2018) compared the virulence and immune escape of the cryptococcal capsule to “a sword and a shield”, illustrating its crucial function (Figure 2).
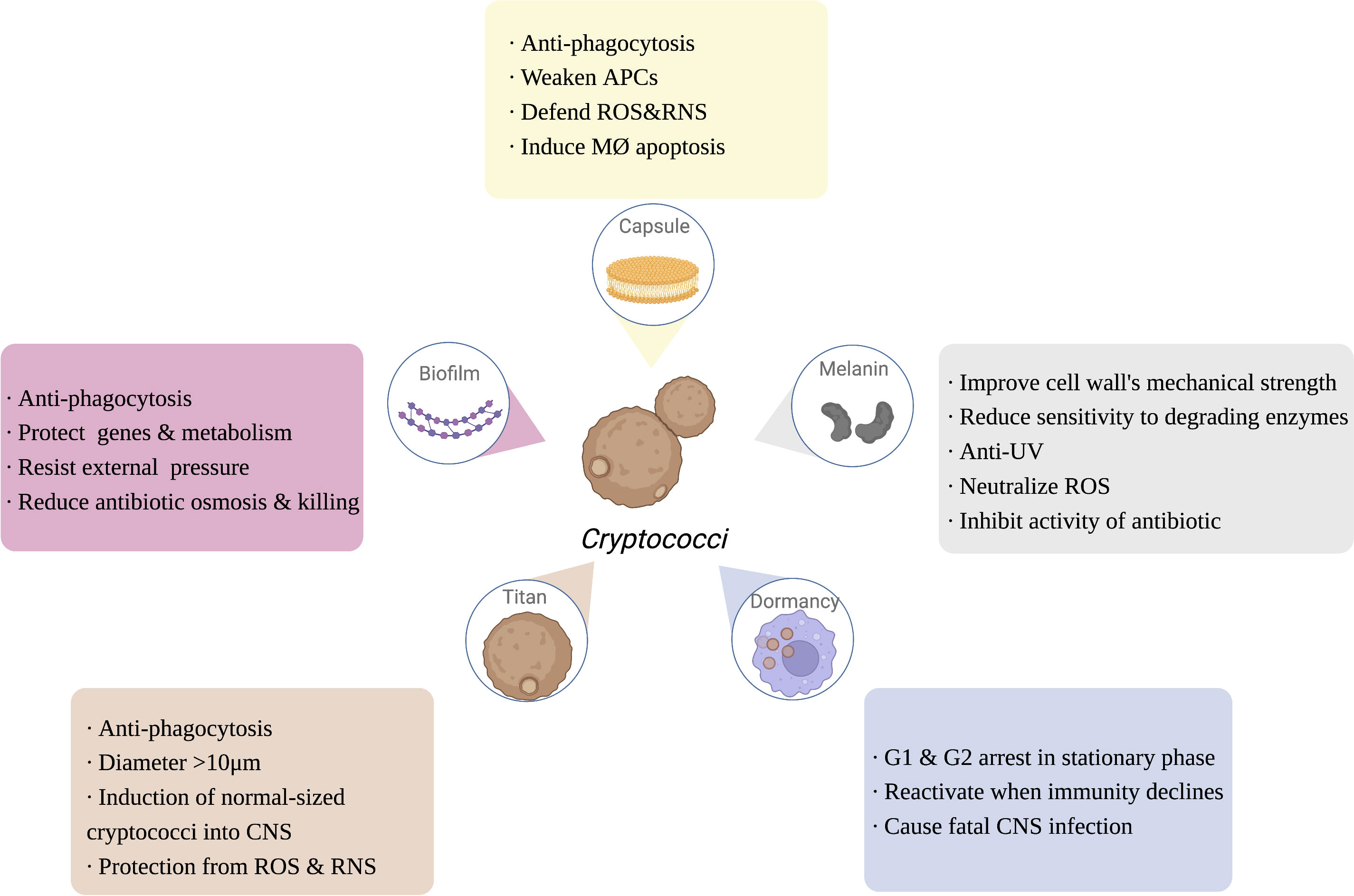
Figure 2 The function of Cryptococcus immune escaping. Capsule, melanin, dormancy, titan cells and biofilm of Cryptococcus are also involved in the process of escaping the host’s immunity, enhancing the ability of Cryptococcus to infect the host. APCs, antigen presenting cells; ROS, reactive oxygen species; RNS, reactive nitrogen species; MØ, APCs, antigen presenting cells; ROS, reactive oxygen species; RNS, reactive nitrogen species; MØ, macrophage; UV, ultra violet; CNS, central nervous system. Created in BioRender.com.
The analysis of inositol polyphosphate kinases (IPKs) in Cn by Li et al. (2017) revealed that, among IPKs, cell-wall-related Arg1 regulates capsule production to defend phagocytosis by the innate immune system. Greater exposure of surface β-glucan and mannoprotein by reduced capsule size in the arg1Δ cryptococci mutant to the binding of opsonizing antibodies is suggested to increase phagocyte recognition and phagocytosis. Furthermore, the polysaccharide capsule of Cg was demonstrated to block the functional maturation of human-monocyte-derived DCs, thereby reducing subsequent Cryptococcus phagocytosis (Huston et al., 2016). It was also found that GXM not only adheres to the surface of the cryptococcal cell wall to form capsules, but also enters the blood or cerebrospinal fluid in the free form of exo-GXM. Exo-GXM inhibits the migration of immune cells to the brain and also suppresses the host systemic inflammatory response, thereby exacerbating the host systemic infection, especially in the central nervous system (Denham et al., 2018).
3.1.2 Immune escape effect of melanin
Melanin is a negatively charged, high-molecular-weight hydrophobic substance within the cell wall of Cryptococcus, which helps to improve the mechanical strength of the cell wall, enhance resistance to environmental UV light, and reduce the sensitivity of the fungus to degradative enzymes, while melanin reduces cytokine reactivity, attenuates phagocytosis, neutralizes the oxidative substances (e.g., ROS) released by inflammatory cells, and inhibits antifungal drugs activity, serving to protect the fungus and escape from host immune function (reviewed in Nosanchuk et al., 2015; Eisenman et al., 2020). Melanin is regulated by a synthetic network of core transcription factors, including Bzp4, Usv101, Hob1, and Mbs1, as well as the core kinases Gsk3 and Kic1, which all provide possible targets for the clinical development of drugs that inhibit melanin synthesis (Lee et al., 2019a) (Figure 2).
3.2 Three specific mechanisms of cryptococcal escape immunity
3.2.1 The role of dormancy in immune escape
The close interaction of Cryptococcus and phagocytes in the host dictates why it is defined as a facultative intracellular pathogen (Wang et al., 2022). Phagocytes provide a protective niche for Cryptococcus to replicate, proliferate, and even be dormant when the host immunity is overexpressed. The ability of Cryptococcus to escape the immune effects of the host is enhanced by its greater adaptability to the immune environment (Sorrell et al., 2016). Previous research has observed differences in the cell wall immune recognition epitopes between Cg and Cn, and phagocytic cells were less likely to recognize Cg (Huston et al., 2016), so this section only focuses on the dormant characteristic of Cn to escape host immunity.
Cn infection consists of three stages: early exposure to the immune environment activates the host immune system; subsequently, cryptococci is dormant and latent; and, finally, cryptococci will be reactivated when the immune system declines. Cn is largely nonpathogenic in immunocompetent hosts, and patients are often diagnosed by a pathological biopsy of unexplained pulmonary nodules (Walsh et al., 2019; Alanio, 2020). Studies have shown that the G1 and G2 phases of the interphase of dormant Cn are at standstill, and that highly energetic behaviors such as division and proliferation are terminated, leaving only basic metabolic functions, such as membrane potential and cell morphology, to be maintained (Takeo et al., 1995). However, when the host is exposed to severe immunosuppressive events, such as HIV/AIDS infection, organ transplantation, and severe burns, the dormant cryptococci rapidly activate and cause fatal central nervous system infections, becoming the “last straw” to crush the immunosuppressed patient (Gerstein et al., 2019). Thus, dormancy is essential for the immune escape of Cn, making it the first fungal pathogen to be studied concerning host immunity (Alanio, 2020) (Figure 2).
3.2.2 The role of Titan cells in immune escape
Titan cells are macrophage-induced cryptococci larger than 10 μm in diameter, up to 100 μm, which cannot be cleared by alveolar macrophages and are an important immune escape mechanism for Cryptococcus (Crabtree et al., 2012; Dambuza et al., 2018). Morphological transition of cryptococci from normal size to titanization was generally stimulated by exposure to external signals, such as CO2, hypoxia, and serum stimulation (Dambuza et al., 2018; Trevijano-Contador et al., 2018). Titanization was proved to be related with cAMP-mediated signaling, which depends on Gpr4/Gpr5 receptors, adenylyl cyclase Cac1, Pka1 kinase, and the transcription factor Rim101 (Okagaki et al., 2011; Choi et al., 2012). Electron microscopy revealed that Cryptococcal Titan cells were enlarged (> 10 μm) and contained features such as mononuclear polyploids, large cytoplasmic vesicles, dense capsules, and a broad cell wall (Hommel et al., 2018). Although Titan cells are unable to cause CNS infection via the Trojan horse model, it has been found that they can induce a normal size of surrounding cryptococci into the CNS (Crabtree et al., 2012). Recent studies have shown that the transcription factor Pdr802 is involved in regulating the formation of Cryptococcal Titan cells and mediates the entry of Cryptococcus into the CNS to cause infection (Reuwsaat et al., 2021). Progeny proliferated by Cryptococcal Titan cells are resistant to unfavorable immune environments such as oxidation and nitrosylation, and have a greater capacity to proliferate within phagocytes (García-Rodas et al., 2019). Titan cells are an important form of Cryptococcus resistance to host immune phagocytosis, escape from host immune responses, and exacerbate the infection of the host CNS (Figure 2).
3.2.3 The role of biofilms in immune escape
Biofilms are functional three-dimensional structures commonly found on the surface of pathogens that enhance the ability of the organism to survive in a hostile immune environment by protecting important intracellular genetic material and energy metabolic activities against external physical and chemical stresses (Butassi et al., 2021). Biofilm structures can be observed around cryptococci in the host and help Cryptococcus to resist the immune effects of the host (Schlafer et al., 2018). Biofilm formation is also observed around cryptococci during nonlytic exocytosis from macrophages, suggesting that biofilms are involved in the transmission of Cryptococcus (Lee et al., 2019b). The protective effect of biofilms greatly limits the penetration of antifungal drugs, and therefore cryptococci are often clinically resistant to amphotericin B, fluconazole and voriconazole (Tits et al., 2020) (Figure 2). As previous studies have reviewed (reviewed in Moranova et al., 2009; Hernández-Chávez et al., 2017; Alanio, 2020; Rizzo et al., 2021), the strategy of a low proliferation rate and a specific transcriptome of Cryptococcus under hypoxia may induce cryptococcal dormancy as well as biofilm formation for immune escaping and survival in host tissue, possibly resulting in a life-threatening secondary acute infection.
4 Conclusion
Cryptococcus is a serious threat to human life and health; although host immunity plays a major role in defending against Cryptococcus, this fatal fungal pathogen has evolved complicated immune escaping strategies to evade surveillance and clearance by the host immune system, enhancing the ability of Cryptococcus to infect its hosts. Understanding its immune escaping strategies is crucial for infection control and the development of immunotherapies. This review summarized a series of immune escaping mechanisms of Cryptococcus, focusing on anticellular immunity, antihumoral immunity, as well as fungal structures and special mechanisms that contribute to immune escaping. Among these, we have particularly highlighted throughout the detrimental role of the cryptococcal capsule against host immunity. Recent studies (Freitas and Santos, 2021; Rizzo et al., 2021) have revealed that capsular polysaccharide (CP) GXM as well as its extracellular vesicles were deemed as vital targets in the field of developing vaccines against cryptococcosis. Further research on the role of capsule immune escape indeed helps increase the understanding of cryptococcal infection and immune escape, and also sheds new light on enhancing the clearance of cryptococci and solving difficult clinical problems, such as cryptococcal drug resistance.
In addition, normal immunity is an important safeguard against cryptococcal infection but an overactive immune response not only disrupts the body’s immune homeostasis, but also causes an excessive inflammatory response. Therefore, clinical treatment of cryptococcal infections should not only activate the host’s immune function or reduce the immune escape of cryptococci, but also focus on maintaining the body’s immune homeostasis to maximize the patient’s prognosis.
Author contributions
Writing—original draft preparation, CY; writing—review and editing, YH, YZ, X.Z, HD, YL, DS, XX; figure handling, CY; supervision, DS, XX; funding acquisition, XX. All authors have read and agreed to the published version of the manuscript.
Funding
This research was funded by the grants from the National Key Research and Development Program (No. 2021YFC2302100), Capital Health Development Scientific Research Unit Matching Fund (No. 2020-2Z-2086), Beijing Hospitals Authority Clinical medicine Development of special funding support (No. XMLX202115), National Capacity Building Project for Multidisciplinary Diagnosis and Treatment of Major Diseases (No. 2019YLFW), Beijing Key clinical specialty project (No. 2020ZDZK1), and National Key Research and Development Program (No. 2020YFC2005404).
Conflict of interest
The authors declare that the research was conducted in the absence of any commercial or financial relationships that could be construed as a potential conflict of interest.
Publisher’s note
All claims expressed in this article are solely those of the authors and do not necessarily represent those of their affiliated organizations, or those of the publisher, the editors and the reviewers. Any product that may be evaluated in this article, or claim that may be made by its manufacturer, is not guaranteed or endorsed by the publisher.
References
Aguirre, K. M., Johnson, L. L. (1997). A role for b cells in resistance to cryptococcus neoformans in mice. Infect. Immun. 65 (2), 525–530. doi: 10.1128/iai.65.2.525-530.1997
Alanio, A. (2020). Dormancy in cryptococcus neoformans: 60 years of accumulating evidence. J. Clin. Invest. 130 (7), 3353–3360. doi: 10.1172/JCI136223
Aslanyan, L., Ekhar, V. V., DeLeon-Rodriguez, C. M., Martinez, L. R. (2017). Capsular specific IgM enhances complement-mediated phagocytosis and killing of cryptococcus neoformans by methamphetamine-treated J774.16 macrophage-like cells. Int. Immunopharmacol. 49, 77–84. doi: 10.1016/j.intimp.2017.05.024
Barreto-Bergter, E., Figueiredo, R. T. (2014). Fungal glycans and the innate immune recognition. Front. Cell Infect. Microbiol. 4. doi: 10.3389/fcimb.2014.00145
Beenhouwer, D. O., Shapiro, S., Feldmesser, M., Casadevall, A., Scharff, M. D. (2001). Both Th1 and Th2 cytokines affect the ability of monoclonal antibodies to protect mice against cryptococcus neoformans. Infect. Immun. 69 (10), 6445–6455. doi: 10.1128/IAI.69.10.6445-6455.2001
Blasi, E., Barluzzi, R., Mazzolla, R., Pitzurra, L., Puliti, M., Saleppico, S., et al. (1995). Biomolecular events involved in anticryptococcal resistance in the brain. Infect. Immun. 63 (4), 1218–1222. doi: 10.1128/iai.63.4.1218-1222.1995
Brienze, V. M. S., André, J. C., Liso, E., Louis, I. V. (2021). Cryptococcal immune reconstitution inflammatory syndrome: From blood and cerebrospinal fluid biomarkers to treatment approaches. Life (Basel) 11 (2), 95. doi: 10.3390/life11020095
Butassi, E., Svetaz, L., Carpinella, M. C., Efferth, T., Zacchino, S. (2021). Fungal biofilms as a valuable target for the discovery of natural products that cope with the resistance of medically important fungi-latest findings. Antibiotics (Basel) 10 (9), 1053. doi: 10.3390/antibiotics10091053
Campuzano, A., Wormley, F. L. (2018). Innate immunity against cryptococcus, from recognition to elimination. J. Fungi (Basel) 4 (1), 33. doi: 10.3390/jof4010033
Casadevall, A., Coelho, C., Cordero, R. J. B., Dragotakes, Q., Jung, E., Vij, R., et al. (2019). The capsule of cryptococcus neoformans. Virulence 10 (1), 822–831. doi: 10.1080/21505594.2018.1431087
Charlier, C., Nielsen, K., Daou, S., Brigitte, M., Chretien, F., Dromer, F. (2009). Evidence of a role for monocytes in dissemination and brain invasion by cryptococcus neoformans. Infect. Immun. 77 (1), 120–127. doi: 10.1128/IAI.01065-08
Chiller, T., Farrokhshad, K., Brummer, E., Stevens, D. A. (2002). Effect of granulocyte colony-stimulating factor and granulocyte-macrophage colony-stimulating factor on polymorphonuclear neutrophils, monocytes or monocyte-derived macrophages combined with voriconazole against cryptococcus neoformans. Med. Mycol. 40 (1), 21–26. doi: 10.1080/mmy.40.1.21.26
Choi, J., Vogl, A. W., Kronstad, J. W. (2012). Regulated expression of cyclic AMP-dependent protein kinase a reveals an influence on cell size and the secretion of virulence factors in cryptococcus neoformans. Mol. Microbiol. 85 (4), 700–715. doi: 10.1111/j.1365-2958.2012.08134.x
Colombo, A. C., Rella, A., Normile, T., Joffe, L. S., Tavares, P. M., de S Araújo, G. R., et al. (2019). Cryptococcus neoformans glucuronoxylomannan and sterylglucoside are required for host protection in an animal vaccination model. mBio 10 (2), e02909–e02918. doi: 10.1128/mBio.02909-18
Condon, T. V., Sawyer, R. T., Fenton, M. J., Riches, D. W. (2011). Lung dendritic cells at the innate-adaptive immune interface. J. Leukoc. Biol. 90 (5), 883–895. doi: 10.1189/jlb.0311134
Crabtree, J. N., Okagaki, L. H., Wiesner, D. L., Strain, A. K., Nielsen, J. N., Nielsen, K. (2012). Titan cell production enhances the virulence of cryptococcus neoformans. Infect. Immun. 80 (11), 3776–3785. doi: 10.1128/IAI.00507-12
Dambuza, I. M., Drake, T., Chapuis, A., Zhou, X., Correia, J., Taylor-Smith, L., et al. (2018). The cryptococcus neoformans titan cell is an inducible and regulated morphotype underlying pathogenesis. PloS Pathog. 14 (5), e1006978. doi: 10.1371/journal.ppat.1006978
Davis, M. J., Tsang, T. M., Qiu, Y., Dayrit, J. K., Freij, J. B., Huffnagle, G. B., et al. (2013). Macrophage M1/M2 polarization dynamically adapts to changes in cytokine microenvironments in cryptococcus neoformans infection. mBio 4 (3), e00264–e00213. doi: 10.1128/mBio.00264-13
Denham, S. T., Verma, S., Reynolds, R. C., Worne, C. L., Daugherty, J. M., Lane, T. E., et al. (2018). Regulated release of cryptococcal polysaccharide drives virulence and suppresses immune cell infiltration into the central nervous system. Infect. Immun. 86 (3), e00662–e00617. doi: 10.1128/IAI.00662-17
Eastman, A. J., He, X., Qiu, Y., Davis, M. J., Vedula, P., Lyons, D. M., et al. (2015a). Cryptococcal heat shock protein 70 homolog Ssa1 contributes to pulmonary expansion of cryptococcus neoformans during the afferent phase of the immune response by promoting macrophage M2 polarization. J. Immunol. 194 (12), 5999–6010. doi: 10.4049/jimmunol.1402719
Eastman, A. J., Osterholzer, J. J., Olszewski, M. A. (2015b). Role of dendritic cell-pathogen interactions in the immune response to pulmonary cryptococcal infection. Future Microbiol. 10 (11), 1837–1857. doi: 10.2217/fmb.15.92
Eisenman, H. C., Greer, E. M., McGrail, C. W. (2020). The role of melanins in melanotic fungi for pathogenesis and environmental survival. Appl. Microbiol. Biotechnol. 104 (10), 4247–4257. doi: 10.1007/s00253-020-10532-z
Elemam, N. M., Ramakrishnan, R. K., Hundt, J. E., Halwani, R., Maghazachi, A. A., Hamid, Q. (2021). Innate lymphoid cells and natural killer cells in bacterial infections: Function, dysregulation, and therapeutic targets. Front. Cell Infect. Microbiol. 11. doi: 10.3389/fcimb.2021.733564
Ellerbroek, P. M., Lefeber, D. J., van Veghel, R., Scharringa, J., Brouwer, E., Gerwig, G. J., et al. (2004). O-Acetylation of cryptococcal capsular glucuronoxylomannan is essential for interference with neutrophil migration. J. Immunol. 173 (12), 7513–7520. doi: 10.4049/jimmunol.173.12.7513
Elsegeiny, W., Marr, K. A., Williamson, P. R. (2018). Immunology of cryptococcal infections: Developing a rational approach to patient therapy. Front. Immunol. 9. doi: 10.3389/fimmu.2018.00651
Eschke, M., Piehler, D., Schulze, B., Richter, T., Grahnert, A., Protschka, M., et al. (2015). A novel experimental model of cryptococcus neoformans-related immune reconstitution inflammatory syndrome (IRIS) provides insights into pathogenesis. Eur. J. Immunol. 45 (12), 3339–3350. doi: 10.1002/eji.201545689
Firacative, C., Gressler, A. E., Schubert, K., Schulze, B., Müller, U., Brombacher, F., et al. (2018). Identification of T helper (Th)1- and Th2-associated antigens of cryptococcus neoformans in a murine model of pulmonary infection. Sci. Rep. 8 (1), 2681. doi: 10.1038/s41598-018-21039-z
Flaczyk, A., Duerr, C. U., Shourian, M., Lafferty, E. I., Fritz, J. H., Qureshi, S. T. (2013). IL-33 signaling regulates innate and adaptive immunity to cryptococcus neoformans. J. Immunol. 191 (5), 2503–2513. doi: 10.4049/jimmunol.1300426
Franco-Paredes, C., Womack, T., Bohlmeyer, T., Sellers, B., Hays, A., Patel, K., et al. (2015). Management of cryptococcus gattii meningoencephalitis. Lancet Infect. Dis. 15 (3), 348–355. doi: 10.1016/S1473-3099(14)70945-4
Freitas, G. J. C., Santos, D. A. (2021). Cryptococcus gattii polysaccharide capsule: An insight on fungal-host interactions and vaccine studies. Eur. J. Immunol. 51 (9), 2206–2209. doi: 10.1002/eji.202149349
García-Barbazán, I., Trevijano-Contador, N., Rueda, C., de Andrés, B., Pérez-Tavárez, R., Herrero-Fernández, I., et al. (2016). The formation of titan cells in cryptococcus neoformans depends on the mouse strain and correlates with induction of Th2-type responses. Cell Microbiol. 18 (1), 111–124. doi: 10.1111/cmi.12488
García-Rodas, R., de Oliveira, H. C., Trevijano-Contador, N., Zaragoza, O. (2019). Cryptococcal titan cells: When yeast cells are all grown up. Curr. Top. Microbiol. Immunol. 422, 101–120. doi: 10.1007/82_2018_145
Gerstein, A. C., Jackson, K. M., McDonald, T. R., Wang, Y., Lueck, B. D., Bohjanen, S., et al. (2019). Identification of pathogen genomic differences that impact human immune response and disease during cryptococcus neoformans infection. mBio 10 (4), e01440–19. doi: 10.1128/mBio.01440-19
Goldman, D. L., Khine, H., Abadi, J., Lindenberg, D. J., Pirofski, L., Niang, R., et al. (2001). Serologic evidence for cryptococcus neoformans infection in early childhood. Pediatrics 107 (5), E66. doi: 10.1542/peds.107.5.e66
Hatinguais, R., Willment, J. A., Brown, G. D. (2020). PAMPs of the fungal cell wall and mammalian PRRs. Curr. Top. Microbiol. Immunol. 425, 187–223. doi: 10.1007/82PMID:32180018
Heitman, J., Kozel, T. R., Chung, K. (2010). Cryptococcus: from human pathogen to model yeast (Washington, DC, USA: ASM Press), 646.
Hernández-Chávez, M. J., Pérez-García, L. A., Niño-Vega, G. A., Mora-Montes, H. M. (2017). Fungal strategies to evade the host immune recognition. J. Fungi (Basel) 3 (4), 51. doi: 10.3390/jof3040051
Heung, L. J., Hohl, T. M. (2019). Inflammatory monocytes are detrimental to the host immune response during acute infection with cryptococcus neoformans. PloS Pathog. 15 (3), e1007627. doi: 10.1371/journal.ppat.1007627
Heyen, L., Müller, U., Siegemund, S., Schulze, B., Protschka, M., Alber, G., et al. (2016). Lung epithelium is the major source of IL-33 and is regulated by IL-33-dependent and IL-33-independent mechanisms in pulmonary cryptococcosis. Pathog. Dis. 74 (7), ftw086. doi: 10.1093/femspd/ftw086
Hole, C. R., Leopold Wager, C. M., Mendiola, A. S., Wozniak, K. L., Campuzano, A., Lin, X., et al. (2016). Antifungal activity of plasmacytoid dendritic cells against cryptococcus neoformans In vitro requires expression of dectin-3 (CLEC4D) and reactive oxygen species. Infect. Immun. 84 (9), 2493–2504. doi: 10.1128/IAI.00103-16
Hommel, B., Mukaremera, L., Cordero, R. J. B., Coelho, C., Desjardins, C. A., Sturny-Leclère, A., et al. (2018). Titan cells formation in cryptococcus neoformans is finely tuned by environmental conditions and modulated by positive and negative genetic regulators. PloS Pathog. 14 (5), e1006982. doi: 10.1371/journal.ppat.1006982
Huston, S. M., Ngamskulrungroj, P., Xiang, R. F., Ogbomo, H., Stack, D., Li, S. S., et al. (2016). Cryptococcus gattii capsule blocks surface recognition required for dendritic cell maturation independent of internalization and antigen processing. J. Immunol. 196 (3), 1259–1271. doi: 10.4049/jimmunol.1501089
Jalali, Z., Ng, L., Singh, N., Pirofski, L. A. (2006). Antibody response to cryptococcus neoformans capsular polysaccharide glucuronoxylomannan in patients after solid-organ transplantation. Clin. Vaccine Immunol. 13 (7), 740–746. doi: 10.1128/CVI.00139-06
Jamil, K., Polyak, M. J., Feehan, D. D., Surmanowicz, P., Stack, D., Li, S. S., et al. (2020). Phagosomal f-actin retention by cryptococcus gattii induces dendritic cell immunoparalysis. mBio 11 (6), e01821–e01820. doi: 10.1128/mBio.01821-20
Jarvis, J. N., Meintjes, G., Bicanic, T., Buffa, V., Hogan, L., Mo, S., et al. (2015). Cerebrospinal fluid cytokine profiles predict risk of early mortality and immune reconstitution inflammatory syndrome in HIV-associated cryptococcal meningitis. PloS Pathog. 11 (4), e1004754. doi: 10.1371/journal.ppat.1004754
Kabata, H., Moro, K., Koyasu, S. (2018). The group 2 innate lymphoid cell (ILC2) regulatory network and its underlying mechanisms. Immunol. Rev. 286 (1), 37–52. doi: 10.1111/imr.12706
Kawakami, K., Kinjo, Y., Uezu, K., Yara, S., Miyagi, K., Koguchi, Y., et al. (2001). Monocyte chemoattractant protein-1-dependent increase of V alpha 14 NKT cells in lungs and their roles in Th1 response and host defense in cryptococcal infection. J. Immunol. 167 (11), 6525–6532. doi: 10.4049/jimmunol.167.11.6525
Kindermann, M., Knipfer, L., Atreya, I., Wirtz, S. (2018). ILC2s in infectious diseases and organ-specific fibrosis. Semin. Immunopathol. 40 (4), 379–392. doi: 10.1007/s00281-018-0677-x
Kindermann, M., Knipfer, L., Obermeyer, S., Müller, U., Alber, G., Bogdan, C., et al. (2020). Group 2 innate lymphoid cells (ILC2) suppress beneficial type 1 immune responses during pulmonary cryptococcosis. Front. Immunol. 11. doi: 10.3389/fimmu.2020.00209
Knipfer, L., Schulz-Kuhnt, A., Kindermann, M., Greif, V., Symowski, C., Voehringer, D., et al. (2019). CCL1/CCR8-dependent feed-forward mechanism drives ILC2 functions in type 2-mediated inflammation. J. Exp. Med. 216 (12), 2763–2777. doi: 10.1084/jem.20182111
Lee, H. H., Del Pozzo, J., Salamanca, S. A., Hernandez, H., Martinez, L. R. (2019b). Reduced phagocytosis and killing of cryptococcus neoformans biofilm-derived cells by J774.16 macrophages is associated with fungal capsular production and surface modification. Fungal Genet. Biol. 132, 103258. doi: 10.1016/j.fgb.2019.103258
Lee, D., Jang, E. H., Lee, M., Kim, S. W., Lee, Y., Lee, K. T., et al. (2019a). Unraveling melanin biosynthesis and signaling networks in cryptococcus neoformans. mBio 10 (5), e02267–e02219. doi: 10.1128/mBio.02267-19
Leopold Wager, C. M., Wormley, F. L., Jr. (2014). Classical versus alternative macrophage activation: the ying and the yang in host defense against pulmonary fungal infections. Mucosal Immunol. 7 (5), 1023–1035. doi: 10.1038/mi.2014.65
Li, C., Lev, S., Desmarini, D., Kaufman-Francis, K., Saiardi, A., Silva, A. P. G., et al. (2017). IP3-4 kinase Arg1 regulates cell wall homeostasis and surface architecture to promote cryptococcus neoformans infection in a mouse model. Virulence 8 (8), 1833–1848. doi: 10.1080/21505594.2017.1385692
Li, Z., Lu, G., Meng, G. (2019). Pathogenic fungal infection in the lung. Front. Immunol. 10. doi: 10.3389/fimmu.2019.01524
Lim, J., Coates, C. J., Seoane, P. I., Garelnabi, M., Taylor-Smith, L. M., Monteith, P., et al. (2018). Characterizing the mechanisms of nonopsonic uptake of cryptococci by macrophages. J. Immunol. 200 (10), 3539–3546. doi: 10.4049/jimmunol.1700790
Liu, Q., Gao, Y., Zhang, B., Sun, F., Yang, Q., Liu, Y., et al. (2020). Cytokine profiles in cerebrospinal fluid of patients with meningitis at a tertiary general hospital in China. J. Microbiol. Immunol. Infect. 53 (2), 216–224. doi: 10.1016/j.jmii.2018.08.019
Mills, K. H. G. (2022). IL-17 and IL-17-producing cells in protection versus pathology. Nat. Rev. Immunol. 5, 1–17. doi: 10.1038/s41577-022-00746-9
Mora, D. J., Fortunato, L. R., Andrade-Silva, L. E., Ferreira-Paim, K., Rocha, I. H., Vasconcelos, R. R., et al. (2015). Cytokine profiles at admission can be related to outcome in AIDS patients with cryptococcal meningitis. PloS One 10 (3), e0120297. doi: 10.1371/journal.pone.0120297
Moranova, Z., Kawamoto, S., Raclavsky, V. (2009). Hypoxia sensing in cryptococcus neoformans: biofilm-like adaptation for dormancy? BioMed. Pap. Med. Fac. Univ. Palacky Olomouc. Czech. Repub. 153 (3), 189–193. doi: 10.5507/bp.2009.031
Mukaremera, L., Nielsen, K. (2017). Adaptive immunity to cryptococcus neoformans infections. J. Fungi (Basel) 3 (4), 64. doi: 10.3390/jof3040064
Müller, U., Piehler, D., Stenzel, W., Köhler, G., Frey, O., Held, J., et al. (2012). Lack of IL-4 receptor expression on T helper cells reduces T helper 2 cell polyfunctionality and confers resistance in allergic bronchopulmonary mycosis. Mucosal Immunol. 5 (3), 299–310. doi: 10.1038/mi.2012.9
Nabavi, N., Murphy, J. W. (1986). Antibody-dependent natural killer cell-mediated growth inhibition of cryptococcus neoformans. Infect. Immun. 51 (2), 556–562. doi: 10.1128/iai.51.2.556-562.1986
Nakouzi, A., Valadon, P., Nosanchuk, J., Green, N., Casadevall, A. (2001). Molecular basis for immunoglobulin m specificity to epitopes in cryptococcus neoformans polysaccharide that elicit protective and nonprotective antibodies. Infect. Immun. 69 (5), 3398–3409. doi: 10.1128/IAI.69.5.3398-3409.2001
Naslund, P. K., Miller, W. C., Granger, D. L. (1995). Cryptococcus neoformans fails to induce nitric oxide synthase in primed murine macrophage-like cells. Infect. Immun. 63 (4), 1298–1304. doi: 10.1128/iai.63.4.1298-1304.1995
Neal, L. M., Xing, E., Xu, J., Kolbe, J. L., Osterholzer, J. J., Segal, B. M., et al. (2017). CD4+ T cells orchestrate lethal immune pathology despite fungal clearance during cryptococcus neoformans meningoencephalitis. mBio 8 (6), e01415–e01417. doi: 10.1128/mBio.01415-17
Nelson, B. N., Beakley, S. G., Posey, S., Conn, B., Maritz, E., Seshu, J., et al. (2021). Antifungal activity of dendritic cell lysosomal proteins against cryptococcus neoformans. Sci. Rep. 11 (1), 13619. doi: 10.1038/s41598-021-92991-6
Nelson, B. N., Hawkins, A. N., Wozniak, K. L. (2020). Pulmonary macrophage and dendritic cell responses to cryptococcus neoformans. Front. Cell Infect. Microbiol. 10. doi: 10.3389/fcimb.2020.00037
Nosanchuk, J. D., Stark, R. E., Casadevall, A. (2015). Fungal melanin: What do we know about structure? Front. Microbiol. 6. doi: 10.3389/fmicb.2015.01463
NYu, C. H., Sephton-Clark, P., Tenor, J. L., Toffaletti, D. L., Giamberardino, C., Haverkamp, M., et al. (2021). Gene expression of diverse cryptococcus isolates during infection of the human central nervous system. mBio 12 (6), e0231321. doi: 10.1128/mBio.02313-21
O'Meara, T. R., Alspaugh, J. A. (2012). The cryptococcus neoformans capsule: a sword and a shield. Clin. Microbiol. Rev. 25 (3), 387–408. doi: 10.1128/CMR.00001-12
Ohshima, Y., Yang, L. P., Uchiyama, T., Tanaka, Y., Baum, P., Sergerie, M., et al. (1998). OX40 costimulation enhances interleukin-4 (IL-4) expression at priming and promotes the differentiation of naive human CD4(+) T cells into high IL-4-producing effectors. Blood 92 (9), 3338–3345.
Okagaki, L. H., Wang, Y., Ballou, E. R., O'Meara, T. R., Bahn, Y. S., Alspaugh, J. A., et al. (2011). Cryptococcal titan cell formation is regulated by G-protein signaling in response to multiple stimuli. Eukaryot. Cell. 10 (10), 1306–1316. doi: 10.1128/EC.05179-11
Osterholzer, J. J., Chen, G. H., Olszewski, M. A., Curtis, J. L., Huffnagle, G. B., Toews, G. B. (2009a). Accumulation of CD11b+ lung dendritic cells in response to fungal infection results from the CCR2-mediated recruitment and differentiation of ly-6Chigh monocytes. J. Immunol. 183 (12), 8044–8053. doi: 10.4049/jimmunol.0902823
Osterholzer, J. J., Surana, R., Milam, J. E., Montano, G. T., Chen, G. H., Sonstein, J., et al. (2009b). Cryptococcal urease promotes the accumulation of immature dendritic cells and a non-protective T2 immune response within the lung. the accumulation of immature dendritic cells and a non-protective T2 immune response within the lung. Am. J. Pathol. 174 (3), 932–943. doi: 10.2353/ajpath.2009.080673
Pericolini, E., Cenci, E., Monari, C., De Jesus, M., Bistoni, F., Casadevall, A., et al. (2006). Cryptococcus neoformans capsular polysaccharide component galactoxylomannan induces apoptosis of human T-cells through activation of caspase-8. Cell Microbiol. 8 (2), 267–275. doi: 10.1111/j.1462-5822.2005.00619.x
Probert, M., Zhou, X., Goodall, M., Johnston, S. A., Bielska, E., Ballou, E. R., et al. (2019). Glucuronoxylomannan epitope exhibits serotype-specific accessibility and redistributes towards the capsule surface during titanization of the fungal pathogen cryptococcus neoformans. Infect. Immun. 87 (4), e00731–e00718. doi: 10.1128/IAI.00731-18
Qiu, Y., Dayrit, J. K., Davis, M. J., Carolan, J. F., Osterholzer, J. J., Curtis, J. L., et al. (2013). Scavenger receptor a modulates the immune response to pulmonary cryptococcus neoformans infection. J. Immunol. 191 (1), 238–248. doi: 10.4049/jimmunol.1203435
Rajasingham, R., Smith, R. M., Park, B. J., Jarvis, J. N., Govender, N. P., Chiller, T. M., et al. (2017). Global burden of disease of HIV-associated cryptococcal meningitis: an updated analysis. Lancet Infect. Dis. 17 (8), 873–881. doi: 10.1016/S1473-3099(17)30243-8
Reuwsaat, J. C. V., Agustinho, D. P., Motta, H., Chang, A. L., Brown, H., Brent, M. R., et al. (2021). The transcription factor Pdr802 regulates titan cell formation and pathogenicity of cryptococcus neoformans. mBio 12 (2), e03457–e03420. doi: 10.1128/mBio.03457-20
Reuwsaat, J. C. V., Motta, H., Garcia, A. W. A., Vasconcelos, C. B., Marques, B. M., Oliveira, N. K., et al. (2018). A predicted mannoprotein participates in cryptococcus gattii capsular structure. mSphere 3 (2), e00023–e00018. doi: 10.1128/mSphere.00023-18
Rizzo, J., Wong, S. S. W., Gazi, A. D., Moyrand, F., Chaze, T., Commere, P. H., et al. (2021). Cryptococcus extracellular vesicles properties and their use as vaccine platforms. J. Extracell. Vesicles 10 (10), e12129. doi: 10.1002/jev2.12129
Rocha, J. D., Nascimento, M. T., Decote-Ricardo, D., Côrte-Real, S., Morrot, A., Heise, N., et al. (2015). Capsular polysaccharides from cryptococcus neoformans modulate production of neutrophil extracellular traps (NETs) by human neutrophils. Sci. Rep. 5, 8008. doi: 10.1038/srep08008
Rohatgi, S., Pirofski, L. A. (2012). Molecular characterization of the early b cell response to pulmonary cryptococcus neoformans infection. J. Immunol. 189 (12), 5820–5830. doi: 10.4049/jimmunol.1201514
Rohatgi, S., Pirofski, L. A. (2015). Host immunity to cryptococcus neoformans. Future Microbiol. 10 (4), 565–581. doi: 10.2217/fmb.14.132
Sabiiti, W., Robertson, E., Beale, M. A., Johnston, S. A., Brouwer, A. E., Loyse, A., et al. (2014). Efficient phagocytosis and laccase activity affect the outcome of HIV-associated cryptococcosis. J. Clin. Invest. 124 (5), 2000–2008. doi: 10.1172/JCI72950
Santiago-Tirado, F. H., Onken, M. D., Cooper, J. A., Klein, R. S., Doering, T. L. (2017). Trojan Horse transit contributes to blood-brain barrier crossing of a eukaryotic pathogen. mBio 8 (1), e02183–e02116. doi: 10.1128/mBio.02183-16
Sato, K., Yamamoto, H., Nomura, T., Kasamatsu, J., Miyasaka, T., Tanno, D., et al. (2020). Production of IL-17A at innate immune phase leads to decreased Th1 immune response and attenuated host defense against infection with cryptococcus deneoformans. J. Immunol. 205 (3), 686–698. doi: 10.4049/jimmunol.1901238jimmunol.1901238
Schlafer, S., Kamp, A., Garcia, J. E. (2018). A confocal microscopy based method to monitor extracellular pH in fungal biofilms. FEMS Yeast Res. 18 (5):foy049. doi: 10.1093/femsyr/foy049
Schulze, B., Piehler, D., Eschke, M., Heyen, L., Protschka, M., Köhler, G., et al. (2016). Therapeutic expansion of CD4+FoxP3+ regulatory T cells limits allergic airway inflammation during pulmonary fungal infection. Pathog. Dis. 74 (4), ftw020. doi: 10.1093/femspd/ftw020
Scriven, J. E., Graham, L. M., Schutz, C., Scriba, T. J., Wilkinson, K. A., Wilkinson, R. J., et al. (2016). Glucuronoxylomannan- associated immune signature, characterized by monocyte deactivation and an increased interleukin 10 level, is a predictor of death in cryptococcal meningitis. J. Infect. Dis. 213 (11), 1725–1734. doi: 10.1093/infdis/jiw007
Scriven, J. E., Rhein, J., Hullsiek, K. H., von Hohenberg, M., Linder, G., Rolfes, M. A., et al. (2015). Early ART after cryptococcal meningitis is associated with cerebrospinal fluid pleocytosis and macrophage activation in a multisite randomized trial. J. Infect. Dis. 212 (5), 769–778. doi: 10.1093/infdis/jiv067
Shourian, M., Qureshi, S. T. (2019). Resistance and tolerance to cryptococcal infection: an intricate balance that controls the development of disease. Front. Immunol. 10. doi: 10.3389/fimmu.2019.00066
Sorrell, T. C., Juillard, P. G., Djordjevic, J. T., Kaufman-Francis, K., Dietmann, A., Milonig, A., et al. (2016). Cryptococcal transmigration across a model brain blood-barrier: evidence of the Trojan horse mechanism and differences between cryptococcus neoformans var. grubii strain H99 and cryptococcus gattii strain R265. Microbes Infect. 18 (1), 57–67. doi: 10.1016/j.micinf.2015.08.017
Spits, H., Artis, D., Colonna, M., Diefenbach, A., Di Santo, J. P., Eberl, G., et al. (2013). Innate lymphoid cells–a proposal for uniform nomenclature. Nat. Rev. Immunol. 13 (2), 145–149. doi: 10.1038/nri3365
Springer, D. J., Ren, P., Raina, R., Dong, Y., Behr, M. J., McEwen, B. F., et al. (2010). Extracellular fibrils of pathogenic yeast cryptococcus gattii are important for ecological niche, murine virulence and human neutrophil interactions. PloS One 5 (6), e10978. doi: 10.1371/journal.pone.0010978
Subramani, A., Griggs, P., Frantzen, N., Mendez, J., Tucker, J., Murriel, J., et al. (2020). Intracellular cryptococcus neoformans disrupts the transcriptome profile of M1- and M2-polarized host macrophages. PloS One 15 (8), e0233818. doi: 10.1371/journal.pone.0233818
Sun, D., Shi, M. (2016). Neutrophil swarming toward cryptococcus neoformans is mediated by complement and leukotriene B4. Biochem. Biophys. Res. Commun. 477 (4), 945–951. doi: 10.1016/j.bbrc.2016.07.005
Sun, D., Sun, P., Li, H., Zhang, M., Liu, G., Strickland, A. B., et al. (2019). Fungal dissemination is limited by liver macrophage filtration of the blood. Nat. Commun. 10 (1), 4566. doi: 10.1038/s41467-019-12381-5
Sun, D., Zhang, M., Liu, G., Wu, H., Zhu, X., Zhou, H., et al. (2015). Real-time imaging of interactions of neutrophils with cryptococcus neoformans demonstrates a crucial role of complement C5a-C5aR signaling. Infect. Immun. 84 (1), 216–229. doi: 10.1128/IAI.01197-15
Taborda, C. P., Casadevall, A. (2002). CR3 (CD11b/CD18) and CR4 (CD11c/CD18) are involved in complement-independent antibody-mediated phagocytosis of cryptococcus neoformans. Immunity 16 (6), 791–802. doi: 10.1016/s1074-7613(02)00328-x
Takeo, K., Tanaka, R., Miyaji, M., Nishimura, K. (1995). Unbudded G2 as well as G1 arrest in the stationary phase of the basidiomycetous yeast cryptococcus neoformans. FEMS Microbiol. Lett. 129 (2-3), 231–235. doi: 10.1111/j.1574-6968.1995.tb07585.x
Tits, J., Cammue, B. P. A., Thevissen, K. (2020). Combination therapy to treat fungal biofilm-based infections. Int. J. Mol. Sci. 21 (22), 8873. doi: 10.3390/ijms21228873
Torosantucci, A., Chiani, P., Bromuro, C., De Bernardis, F., Palma, A. S., Liu, Y., et al. (2009). Protection by anti-beta-glucan antibodies is associated with restricted beta-1,3 glucan binding specificity and inhibition of fungal growth and adherence. PloS One 4 (4), e5392. doi: 10.1371/journal.pone.0005392
Trevijano-Contador, N., de Oliveira, H. C., García-Rodas, R., Rossi, S. A., Llorente, I., Zaballos, Á., et al. (2018). Cryptococcus neoformans can form titan-like cells in vitro in response to multiple signals. PloS Pathog. 14 (5), e1007007. doi: 10.1371/journal.ppat.1007007
Trevijano-Contador, N., Pianalto, K. M., Nichols, C. B., Zaragoza, O., Alspaugh, J. A., Pirofski, et al. (2020). Human IgM inhibits the formation of titan-like cells in cryptococcus neoformans. Infect. Immun. 88 (4), e00046–e00020. doi: 10.1128/IAI.00046-20
Trevijano-Contador, N., Rueda, C., Zaragoza, O. (2016). Fungal morphogenetic changes inside the mammalian host. Semin. Cell Dev. Biol. 57, 100–109. doi: 10.1016/j.semcdb.2016.04.008
Trombetta, E. S., Mellman, I. (2005). Cell biology of antigen processing in vitro and in vivo. Annu. Rev. Immunol. 23, 975–1028. doi: 10.1146/annurev.immunol.22.012703.104538
Urai, M., Kaneko, Y., Ueno, K., Okubo, Y., Aizawa, T., Fukazawa, H., et al. (2016). Evasion of innate immune responses by the highly virulent cryptococcus gattii by altering capsule glucuronoxylomannan structure. Front. Cell Infect. Microbiol. 5. doi: 10.3389/fcimb.2015.00101
Vieira, P. L., de Jong, E. C., Wierenga, E. A., Kapsenberg, M. L., Kaliński, P. (2000). Development of Th1-inducing capacity in myeloid dendritic cells requires environmental instruction. J. Immunol. 164 (9), 4507–4512. doi: 10.4049/jimmunol.164.9.4507
Villena, S. N., Pinheiro, R. O., Pinheiro, C. S., Nunes, M. P., Takiya, C. M., DosReis, G. A., et al. (2008). Capsular polysaccharides galactoxylomannan and glucuronoxylomannan from cryptococcus neoformans induce macrophage apoptosis mediated by fas ligand. Cell Microbiol. 10 (6), 1274–1285. doi: 10.1111/j.1462-5822.2008.01125.x
Walsh, N. M., Botts, M. R., McDermott, A. J., Ortiz, S. C., Wüthrich, M., Klein, B., et al. (2019). Infectious particle identity determines dissemination and disease outcome for the inhaled human fungal pathogen cryptococcus. PloS Pathog. 15 (6), e1007777. doi: 10.1371/journal.ppat.1007777
Wang, Y., Pawar, S., Dutta, O., Wang, K., Rivera, A., Xue, C. (2022). Macrophage mediated immunomodulation during cryptococcus pulmonary infection. Front. Cell Infect. Microbiol. 12. doi: 10.3389/fcimb.2022.859049
Wang, J., Zeng, Y., Luo, W., Xie, X., Li, S. (2015). The role of cryptococcus in the immune system of pulmonary cryptococcosis patients. PloS One 10 (12), e0144427. doi: 10.1371/journal.pone.0144427
Wiesner, D. L., Smith, K. D., Kotov, D. I., Nielsen, J. N., Bohjanen, P. R., Nielsen, K. (2016). Regulatory T cell induction and retention in the lungs drives suppression of detrimental type 2 Th cells during pulmonary cryptococcal infection. J. Immunol. 196 (1), 365–374. doi: 10.4049/jimmunol.1501871
Wiesner, D. L., Specht, C. A., Lee, C. K., Smith, K. D., Mukaremera, L., Lee, S. T., et al. (2015). Chitin recognition via chitotriosidase promotes pathologic type-2 helper T cell responses to cryptococcal infection. PloS Pathog. 11 (3), e1004701. doi: 10.1371/journal.ppat.1004701
Wozniak, K. L., Vyas, J. M., Levitz, S. M. (2006). In vivo role of dendritic cells in a murine model of pulmonary cryptococcosis. Infect. Immun. 74 (7), 3817–3824. doi: 10.1128/IAI.00317-06
Zaragoza, O., Alvarez, M., Telzak, A., Rivera, J., Casadevall, A. (2007). The relative susceptibility of mouse strains to pulmonary cryptococcus neoformans infection is associated with pleiotropic differences in the immune response. Infect. Immun. 75 (6), 2729–2739. doi: 10.1128/IAI.00094-07
Keywords: Cryptococcus, Cryptococcus neoformans, Cryptococcus gattii, immune escape, cellular immunity, humoral immunity, cytokines
Citation: Yang C, Huang Y, Zhou Y, Zang X, Deng H, Liu Y, Shen D and Xue X (2022) Cryptococcus escapes host immunity: What do we know? Front. Cell. Infect. Microbiol. 12:1041036. doi: 10.3389/fcimb.2022.1041036
Received: 10 September 2022; Accepted: 30 September 2022;
Published: 13 October 2022.
Edited by:
Yong-Sun Bahn, Yonsei University, South KoreaReviewed by:
Tong-Bao Liu, Southwest University, ChinaJintao Xu, University of Michigan, United States
Copyright © 2022 Yang, Huang, Zhou, Zang, Deng, Liu, Shen and Xue. This is an open-access article distributed under the terms of the Creative Commons Attribution License (CC BY). The use, distribution or reproduction in other forums is permitted, provided the original author(s) and the copyright owner(s) are credited and that the original publication in this journal is cited, in accordance with accepted academic practice. No use, distribution or reproduction is permitted which does not comply with these terms.
*Correspondence: Dingxia Shen, shendingxia301@sina.com; Xinying Xue, xuexinying2988@bjsjth.cn