- 1Jiangsu Province Key Laboratory of Tonifying Kidney and Anti-senescence, Department of Nephrology, Jiangsu Province Hospital of Chinese Medicine, Affiliated Hospital of Nanjing University of Chinese Medicine, Nanjing, China
- 2The First Affiliated Hospital of Zhejiang Chinese Medical University (Zhejiang Provincial Hospital of Chinese Medicine), Hangzhou, China
As one of the key components of the immune system, mast cells are well known for their role in allergic reactions. However, they are also involved in inflammatory and fibrotic processes. Mast cells participate in all the stages of acute inflammatory responses, playing an immunomodulatory role in both innate and adaptive immunity. Mast cell-derived histamine, TNF-α, and IL-6 contribute to the inflammatory processes, while IL-10 mediates the suppression of inflammation. Crosstalk between mast cells and other immune cells is also involved in the development of inflammation. The cell–cell adhesion of mast cells and fibroblasts is crucial for fibrosis. Mast cell mediators, including cytokines and proteases, play contradictory roles in the fibrotic process. Here, we review the double-edged role of mast cells in inflammation and fibrosis.
1 Introduction
Mast cells (MCs) are important components of the innate and adaptive immune system (Tikoo et al., 2018). MCs are found in almost all kinds of tissues and organs, especially at sites close to the external environments, such as mucosal membranes, the skin, and perivascular areas, acting as sentinels (Piliponsky and Romani, 2018). The role of MCs has been widely investigated in allergic diseases such as urticaria, asthma, and rhinitis. It has been reported that the mediators released by activated MCs are crucial in allergic diseases (Anastasia et al., 2024). A protective role of mast cells in allergic responses has been reported recently (Thomas et al., 2023; Esther et al., 2023). These studies show the biphasic role of MCs in allergy. Because MCs are also demonstrated to be involved in inflammation and fibrosis (Ribatti et al., 2020; Guo et al., 2023), we will discuss the activating and inhibitory effects of MCs in these processes.
Using single-cell transcriptomics analysis, scientists proved that MCs derived from CD34+ pluripotent progenitor cells reside in the bone marrow (Wu et al., 2022). Mast cell progenitors leave the bone marrow and migrate into peripheral tissue, where they finish the process of maturation (Tahereh et al., 2022). Stem cell factor (SCF), which is the ligand of CD117, plays an important role in almost every stage of MC differentiation (Terhorst-Molawi et al., 2023). Mature MCs express a variety of stimulatory and inhibitory receptors on the cell surface (Daniel et al., 2019). Once activated through stimulatory receptors, MCs might release granules that contain a variety of mediators, including preformed mediators, newly synthesized lipid mediators, cytokines, and chemokines (Petri and Ilze, 2017). Due to the diversity of mediators in different MC subtypes, human mast cells are divided into two major subtypes: mast cells whose granules contain mostly tryptase (MC_T) and mast cells whose granules contain tryptase and chymase (MC_TC) (Baba et al., 2017). However, an intermediate phenotype linked to both MC_T and MC_TC was observed in human airway tissues (Dwyer et al., 2021). Another study identified six mast cell clusters in different human tissues (Tauber et al., 2023). These studies showed the natural diversity and heterogeneity of mast cells.
2 Mast cells in acute inflammatory responses
2.1 Mast cells in innate immunity
MCs are involved in innate inflammatory responses such as bacterial infection, venom damage, and tissue injury (Chompunud Na Ayudhya et al., 2020). MCs express a variety of stimulatory receptors that function in innate immunity (Daniel et al., 2019). Agonists of toll-like receptors and Mas-related G protein-coupled receptors (MRGPR), complement anaphylatoxins C3a and C5a, and endogenous peptides are involved in mast cell activation (Cho et al., 2021; Roy et al., 2021; Laumonnier et al., 2023). Unlike the adaptive signals, the innate stimuli lead to the release of small and spherical granules rapidly through the activation of MCs. Those small granules induce local and transient inflammation in innate inflammatory responses (Gaudenzio et al., 2016). Previous studies showed that the activation of MRGPRX2 enhanced the clearance of Staphylococcus aureus from infected mouse skin (Arifuzzaman et al., 2019). MCs were also demonstrated to protect against snake and honeybee toxins by releasing proteases (Yuki et al., 2023). In addition, substance P (SP) could activate Mrgprb2 and mediate thermal hyperalgesia and non-histaminergic itch (Green et al., 2019; Meixiong et al., 2019). These studies revealed the proinflammatory role of MCs in innate immunity.
A series of three successive phases had been described in the acute inflammatory responses (Aller et al., 2004). MCs were shown to be involved in all three stages (Figure 1) (Aller et al., 2019). The first phase of acute inflammation is the nervous phase, which manifests as vascular permeability changes through sensory and motor alterations. The activation of the hypothalamic–pituitary–adrenal axes and sympathetic nervous system was observed in acute tissue injury. In the immediate phase of inflammation, crosstalk of MCs and sensory nerves was crucial (Toyoshima and Okayama, 2022). Activation of the neuroendocrine system induces the release of activating signals, including adenosine, corticotrophin-releasing hormone, and substance P. Those stimuli could activate MCs. Activated MCs then release mediators such as histamine, vascular endothelial growth factor (VEGF), and prostacyclin, which increase vascular permeability and smooth muscle contraction. In addition, histamine, tryptase, and nerve growth factors released by MCs could stimulate nerve fibers and amplify the inflammatory courses.
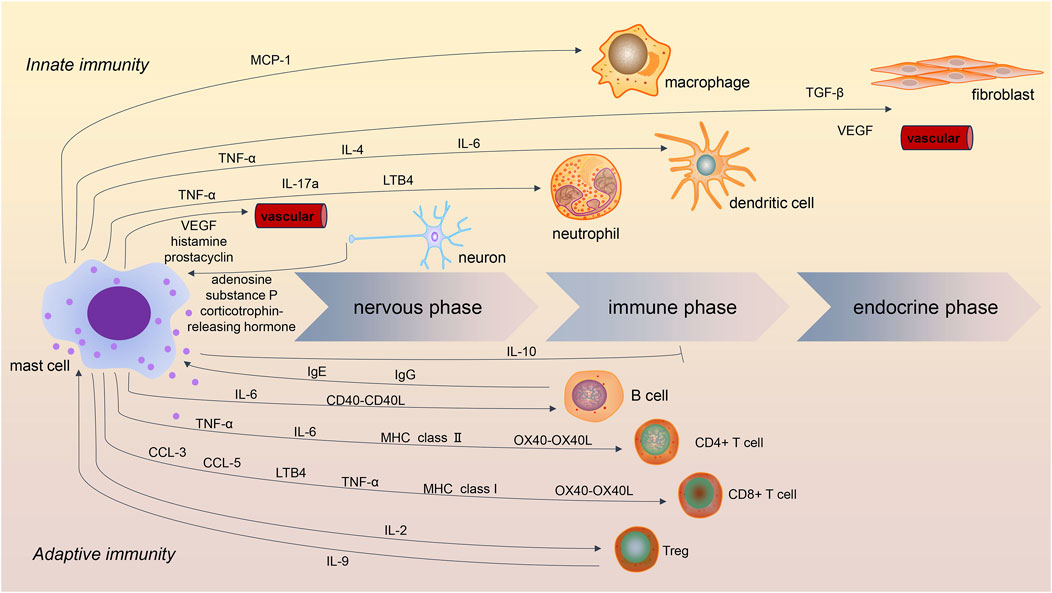
Figure 1. Role of mast cells in acute inflammatory processes. Mast cells are involved in innate and adaptive immunity in the three stages of acute inflammation. In addition to IL-10, most mast cell mediators are proinflammatory. Crosstalk between mast cells and macrophages, dendritic cells, and neutrophils contributes to innate immunity, while the interaction of mast cells with B cells and T cells contributes to adaptive immunity. Crosstalk between mast cells and Treg has been shown to be immunosuppressive in inflammatory processes.
In the later phase of inflammation, also called the immune phase, cytokines released by MCs contribute to the recruitment and maturation of other immune cells, such as macrophages, dendritic cells, and neutrophils. In this stage, MCs mainly play immunoregulatory roles in innate and adaptive immunity. Focusing on innate inflammation, MCs have been shown to contribute to macrophage recruitment and polarization by releasing cytokines such as monocyte chemotactic protein-1 (MCP-1) (Elieh Ali Komi et al., 2020; Zhang et al., 2020). MCs can also modulate neutrophils and dendritic cells. In the process of tissue injury, perivascular MCs were demonstrated to induce neutrophil recruitment and activation through the release of tumor necrosis factor (TNF)-α and interleukin (IL)-17a (Jan et al., 2021; Katia et al., 2013; Régis et al., 2022). Moreover, during bacterial infection, MC-derived leukotriene B4 (LTB4) contributed to host defense by mediating early neutrophil influx and bacterial clearance (Van Bruggen et al., 2023). Similarly, MC-derived TNF-α and IL-6 were shown to promote the migration and maturation of dendritic cells (Jan et al., 2015; Dudeck et al., 2019). Furthermore, MC-derived IL-4 mediated dendritic cell activation at the site of inflammation (Friedel et al., 2024). These results show that mast cells, macrophages, neutrophils, and dendritic cells comprise an important barrier of innate immunity.
Finally, in the last phase of acute inflammation, referred to as the endocrine phase, MCs could influence the microenvironment by inducing fibrogenesis and angiogenesis. MC-derived transforming growth factor (TGF)-β, VEGF, and fibroblast growth factor (FGF) are involved in this stage (Kyritsi et al., 2021). Those mediators are also essential in chronic inflammation and fibrosis.
2.2 Mast cells in adaptive immunity
MCs are believed to be a critical bridge in the transition from innate to adaptive immunity (Cardamone et al., 2016). MCs express high-affinity immunoglobulin E (IgE) receptors and several low-affinity IgG receptors on the cell surface (Daniel et al., 2019). These receptors allow antibodies to enroll MCs into immune responses in the presence of antigens. The role of MCs has been widely investigated in IgE-induced anaphylaxis (Dispenza et al., 2023). IgE and the high-affinity IgE receptors on MCs were also shown to be crucial for acquired resistance to honeybee venom (Marichal et al., 2013). In addition, the crosstalk between MCs and adaptive immune cells, including B cells and T cells, was essential for adaptive immunity.
MCs have been found to induce the activation and differentiation of B cells both in mouse models and humans (Breitling et al., 2017; Rurik et al., 2021). Direct cell–cell contact was reported between MCs and B cells. The CD40-CD40L interaction play- a key role in MC-mediated B-cell activation (Gwan Ui et al., 2015; Viviana et al., 2020). In addition, MC-derived IL-6 was involved in B cell differentiation and immunoglobulin A (IgA) secretion (Merluzzi et al., 2010; Valeri et al., 2021). Because B-cell-derived IgE could re-activate MCs and result in more mediator release, MC-B-cell interaction might exaggerate the adaptive inflammatory responses.
Human MCs were shown to release chemokines such as chemokine (C-C motif) ligand (CCL)3 and CCL5 to recruit cytotoxic cells, including CD8+T cells, during virus infection (Yana et al., 2021; Chen et al., 2023). MC-derived LTB4 and TNF-α were crucial for the amplifying of CD8+ T cells dominated adaptive immunity (Jan et al., 2015; Bodduluri et al., 2018). MCs were shown to be capable of antigen presentation via major histocompatibility complex (MHC) class I molecules to CD8+T cells (R et al., 1996). This crosstalk proved to be important in regulating CD8+T-cell proliferation, cytokine secretion, and cytotoxic activity. MCs were demonstrated to express costimulatory molecules OX40L for T-cell activation in human skin (Marta et al., 2014). Similarly, MCs are involved in the recruitment, activation, and differentiation of CD4+ T cells. Most of the modulating function of MCs on CD4+ T cells depends on the expression of MHC class Ⅱ molecules and OX40L (Sahar et al., 2017). TNF-α and IL-6 are also crucial in establishing synaptic contacts (Nicolas et al., 2013). Interestingly, evidence showed that MCs treated with nicotinamide adenine dinucleotide (NAD)+ could mediate CD4+ T-cell differentiation independently of MHC II and T-cell receptor signaling machinery (Hector et al., 2018).
In addition to the roles of initiator and effector in innate immunity, MCs are also important in the host’s transition from innate to adaptive immunity. The crosstalk between MCs and other immune cells indicated the immunomodulatory ability of MCs. The immunomodulatory ability might be more important than the effector-cell function under given circumstances. Further exploration is needed to understand how to regulate MCs in inflammatory processes.
2.3 Role of mast cells in the suppression of inflammatory responses
MCs were demonstrated to be suppressors of inflammatory responses. The anti-inflammatory role of MCs is partially dependent on the release of IL-10. Using three different types of MC-deficient mice and mice with ablated MC-derived IL-10, researchers showed that MC-derived IL-10 could limit inflammation and tissue pathology in contact hypersensitivity (Reber et al., 2017). In another study using IL-33 for the activation of synovial MCs, researchers found that the production of IL-10 and histamine was elevated, resulting in the suppression of monocyte-mediated disease activity in rheumatoid arthritis (Rivellese et al., 2015). MC-derived IL-10 also functions in graft-versus-host disease (GVHD). MC-derived IL-10 significantly reduced GVHD by decreasing conventional T-cell proliferation. This immunosuppressive ability was independent of Tregs (Leveson-Gower et al., 2013). In experimental murine myeloperoxidase (MPO)-ANCA-associated vasculitis (AAV), MC-derived IL-10 enhanced the immunosuppressive function of Treg and played a protective role in MPO-related inflammation (Gan et al., 2012; Gan et al., 2016).
In addition to IL-10, the immunosuppressive function of MCs also depends on the crosstalk of MCs and regulatory T cells. IL-9 produced by Treg is important in MC recruitment and activation, which then mediate regional immune suppression in tolerant allografts. MC-deficient mice are not capable of establishing long-term allograft tolerance (Li-Fan et al., 2006). In addition, MC-derived IL-2 is involved in suppressing chronic allergic dermatitis by promoting Treg expansion (Keith et al., 2023). MCs are also crucial for the resistance of streptozotocin-induced type 1 diabetes mellitus by promoting Treg-mediated immunological tolerance (Carlos et al., 2015).
Although many studies have discussed the proinflammatory and anti-inflammatory roles of MCs, it is still unclear which signals mediate the immunosuppressive function of MCs. One study showed that the MC stabilizer disodium cromoglycate inhibited MC degranulation without affecting IL-10 production and resulted in the protection of AAV disease activity (Gan et al., 2016). This study suggested that some medications might limit the deleterious function and retain the protective ability of MCs. Perhaps there will be more targeted treatments that regulate the function of MCs in inflammatory processes in the future.
3 Mast cells and fibrosis
3.1 Mast cells in the activation of fibroblasts
The transition of fibroblasts into myofibroblasts was shown to induce excessive deposition of extracellular matrix and then cause fibrosis in different tissues and organs (Younesi et al., 2024). Crosstalk between MCs and fibroblasts has been investigated in recent decades (Figure 2). One study showed that co-culture of dermal fibroblasts with human MC significantly enhanced contraction of the three-dimensional collagen lattices; the addition of antibodies against stem cell factor (SCF) and c-kit resulted in the inhibition of gel contraction. Nevertheless, adding the supernatants of MCs to cultured fibroblasts did not enhance the speed of gel contraction, which indicates the importance of cell–cell contact (Yamamoto et al., 2000). Several studies showed cell adhesion between MCs and fibroblasts (Yaksh et al., 2019; Pincha et al., 2018). Murine MCs adhere to fibroblasts through SCF-KIT interaction (Adachi et al., 1992), while the adhesion of human MCs and fibroblasts depends on the expression of the cell adhesion molecule (CADM)1-kit mechanism (Moiseeva et al., 2013). This cell–cell adhesion is the foundation of contact-dependent cell communication.
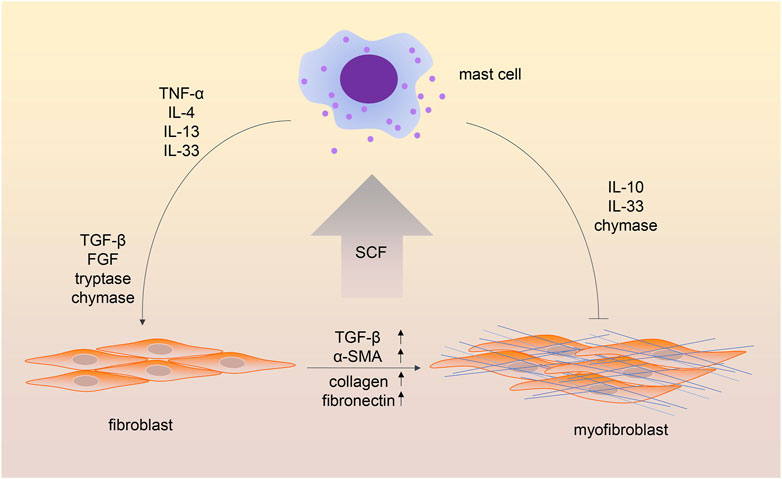
Figure 2. Crosstalk between mast cells and fibroblasts in fibrotic processes. The cell–cell adhesion between mast cells and fibroblasts depends on the stem cell factor-Kit (SCF-KIT) interaction. In addition, mast cell-derived cytokines and chemokines contribute to the transition of fibroblasts into myofibroblasts. Interestingly, some mast cell mediators have been shown to have both profibrotic and antifibrotic functions.
In addition to the contact-dependent cell communication, mediators released by both cells are crucial in the MC-fibroblast crosstalk. It is believed that fibroblasts triggered MC activation through the production of SCF (Luo et al., 2022). Meanwhile, several MC-derived mediators, such as TGF-β, are involved in activating fibroblasts. TNF-α was shown to be crucial for the MC-fibroblast interaction in myocardial fibrosis (Zhang et al., 2011). MC-derived IL-13/IL-4 is involved in disease progression in myelofibrosis (Melo-Cardenas et al., 2022). MCs could release IL-33, which stimulates fibroblasts and enhances collagen expression (Wulff et al., 2019). MC tryptase was demonstrated to induce lung fibroblast migration via protease-activated receptor-2 (PAR-2) activation (Bagher et al., 2018). In addition, MC tryptase was shown to promote inflammatory bowel disease-induced intestinal fibrosis through the PAR-2/Akt/mammalian target of the rapamycin (mTOR) pathway of fibroblasts (Liu et al., 2021). MC chymase was found to promote fibroblast proliferation and collagen synthesis through the activation of the TGF-β1/Smads signaling pathway (Chen et al., 2017). Chymase was also shown to be a major source of angiotensin II (Ang II) production (Ahmad and Ferrario, 2018), and Ang II was widely investigated for its pro-fibrosis role in different diseases (Lv et al., 2021).
3.2 Mast cells in the inhibition of fibrosis
An antifibrotic role of MCs was also observed in several studies. One study observed the difference between the unilateral ureteral obstruction (UUO) model in W/Wv mice and the UUO model in the wild type. Higher levels of renal interstitium fibrosis, more infiltrating immune cells, and higher tissue levels of TGF-β1 were observed in MC-deficient mice, which indicated the protective role of MCs in renal fibrosis. A possible explanation of the protective role of MCs was that MC-derived heparin could inhibit TGF-β1 production (Kim et al., 2009; Beghdadi et al., 2013). As mentioned above, MCs could produce anti-inflammatory mediators, including IL-10 and IL-33 (Stephen et al., 2020). IL-10 has been shown to attenuate cardiac fibrosis by inhibiting TGF-β-induced myofibroblast differentiation (Verma et al., 2017). Another study observed that IL-33 impaired fibroblast migration and gel contraction in age-related macular degeneration (Theodoropoulou et al., 2015). Because IL-33 has been reported for its profibrotic role in diseased states, the function of IL-33 in fibrosis might be contradictory. Another MC mediator was proved to be both profibrotic and antifibrotic. MC chymase was shown to protect against renal fibrosis by activating matrix metalloproteinases and degrading interstitial deposits of fibronectin (Beghdadi et al., 2013; MacColl and Khalil, 2015).
3.3 Confounding factors of mast cells in fibrosis
Unlike the role of MCs in the inflammatory process, the role of MCs in fibrosis is more complicated. The anti-inflammatory function of MCs is mostly mediated by IL-10 and crosstalk between MCs and regular T cells. However, in fibrotic processes, both a profibrotic and antifibrotic role can be observed in a specific MC-derived mediator. In addition, results from cell culture and animal models are often contradictory. One explanation is that some of the MC-deficient animal models are not appropriate for the study under the given circumstances. Some reactions have been demonstrated to be induced by the other consequences of the genetic mutation instead of the absence of MCs (Chmelař et al., 2016). The different types of stimuli may also be responsible for the paradoxical results. The reactions of MCs are not consistent for acute tissue injury and chronic tissue damage. One study investigated the role of MCs in both the early and late phases in murine models of acute and chronic renal ischemia-reperfusion injury (IRI). MCs were found to promote fibrosis in the acute phase of IRI but not in the chronic phase of the disease (Danelli et al., 2017). Moreover, the most widely used MC stabilizers, disodium cromoglycate and ketotifen, can inhibit IgE-mediated MC degranulation but have no impact on mediators independently of degranulation. A high concentration of disodium cromoglycate has an effect beyond its inhibition on MCs (Oka et al., 2012). This makes targeting the antifibrotic effects of MCs more challenging.
4 Conclusion
The heterogeneity and diversity of mediators make the role of MCs contradictory in inflammation and fibrosis. For further research, animal models should be carefully selected to eliminate the effect of MCs. Using two or more MC-deficient animal models, as well as MC-engrafted methods, will be more precise than using one single animal model. In addition, most research animal models are designed for acute injury; perhaps an animal model of chronic damage may reflect the functions of MCs in chronic inflammation and fibrosis more exactly. Moreover, the specificity of MC stabilizers is not satisfactory; targeting a single mediator of MCs will be more important in research and clinical applications.
Author contributions
XW: conceptualization, writing–original draft, writing–review and editing. PZ: data curation, writing–review and editing. YT: writing–review and editing. YC: writing–review and editing. EZ: supervision, validation, writing–review and editing. KG: conceptualization, supervision, validation, writing–original draft.
Funding
The author(s) declare that financial support was received for the research, authorship, and/or publication of this article. This work was supported by the National Natural Science Foundation of China (82104750 AND 82274432).
Conflict of interest
The authors declare that the research was conducted in the absence of any commercial or financial relationships that could be construed as a potential conflict of interest.
Publisher’s note
All claims expressed in this article are solely those of the authors and do not necessarily represent those of their affiliated organizations, or those of the publisher, the editors and the reviewers. Any product that may be evaluated in this article, or claim that may be made by its manufacturer, is not guaranteed or endorsed by the publisher.
References
Adachi, S., Ebi, Y., Nishikawa, S., Hayashi, S., Yamazaki, M., Kasugai, T., et al. (1992). Necessity of extracellular domain of W (C-kit) receptors for attachment of murine cultured mast cells to fibroblasts. Blood 79 (3), 650–656. doi:10.1182/blood.v79.3.650.650
Ahmad, S., and Ferrario, C. M. (2018). Chymase inhibitors for the treatment of cardiac diseases: a patent review (2010-2018). Expert Opin. Ther. Pat. 28 (11), 755–764. Epub 20181010. doi:10.1080/13543776.2018.1531848
Aller, M. A., Arias, A., Arias, J. I., and Arias, J. (2019). Carcinogenesis: the cancer cell-mast cell connection. Inflamm. Res. 68 (2), 103–116. Epub 20181120. doi:10.1007/s00011-018-1201-4
Aller, M. A., Arias, J. L., Nava, M. P., and Arias, J. (2004). Posttraumatic inflammation is a complex response based on the pathological expression of the nervous, immune, and endocrine functional systems. Exp. Biol. Med. (Maywood) 229 (2), 170–181. doi:10.1177/153537020422900206
Anastasia, N. P., Maxim, S. S., Boris, V. C., and Maria, A. C. (2024). The role played by autophagy in fcεri-dependent activation of mast cells. Cells 13 (8), 690. doi:10.3390/cells13080690
Arifuzzaman, M., Mobley, Y. R., Choi, H. W., Bist, P., Salinas, C. A., Brown, Z. D., et al. (2019). Mrgpr-mediated activation of local mast cells clears cutaneous bacterial infection and protects against reinfection. Sci. Adv. 5 (1), eaav0216. Epub 20190102. doi:10.1126/sciadv.aav0216
Baba, S., Kondo, K., Suzukawa, M., Ohta, K., and Yamasoba, T. (2017). Distribution, subtype population, and ige positivity of mast cells in chronic rhinosinusitis with nasal polyps. Ann. Allergy Asthma Immunol. 119 (2), 120–128. Epub 20170619. doi:10.1016/j.anai.2017.05.019
Bagher, M., Larsson-Callerfelt, A. K., Rosmark, O., Hallgren, O., Bjermer, L., and Westergren-Thorsson, G. (2018). Mast cells and mast cell tryptase enhance migration of human lung fibroblasts through protease-activated receptor 2. Cell Commun. Signal 16 (1), 59. Epub 20180915. doi:10.1186/s12964-018-0269-3
Beghdadi, W., Madjene, L. C., Claver, J., Pejler, G., Beaudoin, L., Lehuen, A., et al. (2013). Mast cell chymase protects against renal fibrosis in murine unilateral ureteral obstruction. Kidney Int. 84 (2), 317–326. Epub 20130320. doi:10.1038/ki.2013.98
Bodduluri, S. R., Mathis, S., Maturu, P., Krishnan, E., Satpathy, S. R., Chilton, P. M., et al. (2018). Mast cell-dependent Cd8(+) T-cell recruitment mediates immune surveillance of intestinal tumors in apc(min/+) mice. Cancer Immunol. Res. 6 (3), 332–347. Epub 20180130. doi:10.1158/2326-6066.Cir-17-0424
Breitling, S., Hui, Z., Zabini, D., Hu, Y., Hoffmann, J., Goldenberg, N. M., et al. (2017). The mast cell-B cell Axis in lung vascular remodeling and pulmonary hypertension. Am. J. Physiol. Lung Cell Mol. Physiol. 312 (5), L710-L721–l21. Epub 20170224. doi:10.1152/ajplung.00311.2016
Cardamone, C., Parente, R., Feo, G. D., and Triggiani, M. (2016). Mast cells as effector cells of innate immunity and regulators of adaptive immunity. Immunol. Lett. 178, 10–14. Epub 20160705. doi:10.1016/j.imlet.2016.07.003
Carlos, D., Yaochite, J. N., Rocha, F. A., Toso, V. D., Malmegrim, K. C., Ramos, S. G., et al. (2015). Mast cells control insulitis and increase Treg cells to confer protection against stz-induced type 1 diabetes in mice. Eur. J. Immunol. 45 (10), 2873–2885. Epub 20150814. doi:10.1002/eji.201545498
Chen, H., Xu, Y., Yang, G., Zhang, Q., Huang, X., Yu, L., et al. (2017). Mast cell chymase promotes hypertrophic scar fibroblast proliferation and collagen synthesis by activating TGF-β1/Smads signaling pathway. Exp. Ther. Med. 14 (5), 4438–4442. Epub 20170831. doi:10.3892/etm.2017.5082
Chen, Y., Griffiths, C. E. M., and Bulfone-Paus, S. (2023). Exploring mast cell-Cd8 T cell interactions in inflammatory skin diseases. Int. J. Mol. Sci. 24 (2), 1564. Epub 20230113. doi:10.3390/ijms24021564
Chmelař, J., Chatzigeorgiou, A., Chung, K. J., Prucnal, M., Voehringer, D., Roers, A., et al. (2016). No role for mast cells in obesity-related metabolic dysregulation. Front. Immunol. 7, 524. Epub 20161124. doi:10.3389/fimmu.2016.00524
Cho, K. A., Choi, D. W., Park, M., Kim, Y. H., and Woo, S. Y. (2021). Toll-like receptor 7 (Tlr7) mediated transcriptomic changes on human mast cells. Ann. Dermatol 33 (5), 402–408. Epub 20210908. doi:10.5021/ad.2021.33.5.402
Chompunud Na Ayudhya, C., Roy, S., Thapaliya, M., and Ali, H. (2020). Roles of a mast cell-specific receptor Mrgprx2 in host defense and inflammation. J. Dent. Res. 99 (8), 882–890. Epub 20200511. doi:10.1177/0022034520919107
Danelli, L., Madjene, L. C., Madera-Salcedo, I., Gautier, G., Pacreau, E., Ben, M. S., et al. (2017). Early phase mast cell activation determines the chronic outcome of renal ischemia-reperfusion injury. J. Immunol. 198 (6), 2374–2382. Epub 20170206. doi:10.4049/jimmunol.1601282
Daniel, E. A. K., Stefan, W., and Leonard, B. (2019). Mast cell biology at molecular level: a comprehensive review. Clin. Rev. Allergy Immunol. 58 (3), 342–365. doi:10.1007/s12016-019-08769-2
Dispenza, M. C., Metcalfe, D. D., and Olivera, A. (2023). Research advances in mast cell biology and their translation into novel therapies for anaphylaxis. J. Allergy Clin. Immunol. Pract. 11 (7), 2032–2042. Epub 20230321. doi:10.1016/j.jaip.2023.03.015
Dudeck, J., Froebel, J., Kotrba, J., Lehmann, C. H. K., Dudziak, D., Speier, S., et al. (2019). Engulfment of mast cell secretory granules on skin inflammation boosts dendritic cell migration and priming efficiency. J. Allergy Clin. Immunol. 143 (5), 1849–1864. Epub 20181017. doi:10.1016/j.jaci.2018.08.052
Dwyer, D. F., Ordovas-Montanes, J., Allon, S. J., Buchheit, K. M., Vukovic, M., Derakhshan, T., et al. (2021). Human airway mast cells proliferate and acquire distinct inflammation-driven phenotypes during type 2 inflammation. Sci. Immunol. 6 (56), eabb7221. doi:10.1126/sciimmunol.abb7221
Elieh Ali Komi, D., Shafaghat, F., and Christian, M. (2020). Crosstalk between mast cells and adipocytes in physiologic and pathologic conditions. Clin. Rev. Allergy Immunol. 58 (3), 388–400. doi:10.1007/s12016-020-08785-7
Esther, B. F., Nathaniel, D. B., Jaime, L. C., Bruna, G. C. L., Mahdieh, G., Fernando, C., et al. (2023). Immune sensing of food allergens promotes avoidance behaviour. Nature 620 (7974), 643–650. doi:10.1038/s41586-023-06362-4
Friedel, J., Pierre, S., Kolbinger, A., Schäufele, T. J., Aliraj, B., Weigert, A., et al. (2024). Mast cell-derived interleukin-4 mediates activation of dendritic cell during toll-like receptor 2-mediated inflammation. Front. Immunol. 15, 1353922. Epub 20240430. doi:10.3389/fimmu.2024.1353922
Gan, P. Y., O'Sullivan, K. M., Ooi, J. D., Alikhan, M. A., Odobasic, D., Summers, S. A., et al. (2016). Mast cell stabilization ameliorates autoimmune anti-myeloperoxidase glomerulonephritis. J. Am. Soc. Nephrol. 27 (5), 1321–1333. Epub 20150915. doi:10.1681/asn.2014090906
Gan, P. Y., Summers, S. A., Ooi, J. D., O'Sullivan, K. M., Tan, D. S., Muljadi, R. C., et al. (2012). Mast cells contribute to peripheral tolerance and attenuate autoimmune vasculitis. J. Am. Soc. Nephrol. 23 (12), 1955–1966. Epub 20121108. doi:10.1681/asn.2012060572
Gaudenzio, N., Sibilano, R., Marichal, T., Starkl, P., Reber, L. L., Cenac, N., et al. (2016). Different activation signals induce distinct mast cell degranulation strategies. J. Clin. Invest 126 (10), 3981–3998. Epub 20160919. doi:10.1172/jci85538
Green, D. P., Limjunyawong, N., Gour, N., Pundir, P., and Dong, X. (2019). A mast-cell-specific receptor mediates neurogenic inflammation and pain. Neuron 101 (3), 412–420. Epub 20190124. doi:10.1016/j.neuron.2019.01.012
Guo, X., Sun, M., Yang, P., Meng, X., and Liu, R. (2023). Role of mast cells activation in the tumor immune microenvironment and immunotherapy of cancers. Eur. J. Pharmacol. 960, 176103. Epub 20231016. doi:10.1016/j.ejphar.2023.176103
Gwan Ui, H., Ji Yeun, L., Nam Goo, K., Joo-Ho, S., and Jai Youl, R. (2015). Ige and iga produced by ox40-ox40l or Cd40-Cd40l interaction in B cells-mast cells Re-activate fcεri or fcαri on mast cells in mouse allergic asthma. Eur. J. Pharmacol. 754 (0), 199–210. doi:10.1016/j.ejphar.2015.02.023
Hector, R. C. B., Timm, H., Hirofumi, U., Virginia, C., Koichiro, M., Yeqi, N., et al. (2018). Mast cells regulate Cd4(+) T-cell differentiation in the absence of antigen presentation. J. Allergy Clin. Immunol. 142 (6), 1894–1908.e7. doi:10.1016/j.jaci.2018.01.038
Jan, D., Johanna, K., Roland, I., Aaron, H., Martin, V., Vasileia Ismini, A., et al. (2021). Directional mast cell degranulation of tumor necrosis factor into blood vessels primes neutrophil extravasation. Immunity 54 (3), 468–483.e5. doi:10.1016/j.immuni.2020.12.017
Jan, D., Shanawaz, M. G., Christian, H. K. L., Anja, H., Nadja, S., Sergei, A. N., et al. (2015). Mast-cell-derived tnf amplifies Cd8(+) dendritic cell functionality and Cd8(+) T cell priming. Cell Rep. 13 (2), 399–411. doi:10.1016/j.celrep.2015.08.078
Katia, D. F., Anne, D., Mike, H., Emma, N., Nico, vR., Karin, H., et al. (2013). Mast cell and macrophage chemokines cxcl1/cxcl2 control the early stage of neutrophil recruitment during tissue inflammation. Blood 121 (24), 4930–4937. doi:10.1182/blood-2013-02-486217
Keith, Y. H., Egawa, G., Honda, T., and Kabashima, K. (2023). Mast cells in type 2 skin inflammation: maintenance and function. Eur. J. Immunol. 53 (8), e2250359. Epub 20230407. doi:10.1002/eji.202250359
Kim, D., Moon, S., Jung, Y., Lee, A., Kang, K., Lee, T., et al. (2009). Mast cells decrease renal fibrosis in unilateral ureteral obstruction. Kidney Int. 75 (10), 1031–1038. doi:10.1038/ki.2009.1
Kyritsi, K., Kennedy, L., Meadows, V., Hargrove, L., Demieville, J., Pham, L., et al. (2021). Mast cells induce ductular reaction mimicking liver injury in mice through mast cell-derived transforming growth factor beta 1 signaling. Hepatology 73 (6), 2397–2410. Epub 20210419. doi:10.1002/hep.31497
Laumonnier, Y., Korkmaz, R., Nowacka, A. A., and Köhl, J. (2023). Complement-mediated immune mechanisms in allergy. Eur. J. Immunol. 53 (10), e2249979. Epub 20230709. doi:10.1002/eji.202249979
Leveson-Gower, D. B., Sega, E. I., Kalesnikoff, J., Florek, M., Pan, Y., Pierini, A., et al. (2013). Mast cells suppress murine gvhd in a mechanism independent of Cd4+Cd25+ regulatory T cells. Blood 122 (22), 3659–3665. Epub 20130912. doi:10.1182/blood-2013-08-519157
Li-Fan, L., Evan, F. L., David, C. G., Kathy, A. B., Michael, W. G., Karina, P.-L., et al. (2006). Mast cells are essential intermediaries in regulatory T-cell tolerance. Nature 442 (7106), 997–1002. doi:10.1038/nature05010
Liu, B., Yang, M. Q., Yu, T. Y., Yin, Y. Y., Liu, Y., Wang, X. D., et al. (2021). Mast cell tryptase promotes inflammatory bowel disease-induced intestinal fibrosis. Inflamm. Bowel Dis. 27 (2), 242–255. doi:10.1093/ibd/izaa125
Luo, Y., Zhang, H., Yu, J., Wei, L., Li, M., and Xu, W. (2022). Stem cell factor/mast cell/ccl2/monocyte/macrophage Axis promotes coxsackievirus B3 myocarditis and cardiac fibrosis by increasing ly6c(high) monocyte influx and fibrogenic mediators production. Immunology 167 (4), 590–605. Epub 20220823. doi:10.1111/imm.13556
Lv, S. L., Zeng, Z. F., Gan, W. Q., Wang, W. Q., Li, T. G., Hou, Y. F., et al. (2021). Lp-Pla2 inhibition prevents Ang ii-induced cardiac inflammation and fibrosis by blocking macrophage Nlrp3 inflammasome activation. Acta Pharmacol. Sin. 42 (12), 2016–2032. Epub 20210705. doi:10.1038/s41401-021-00703-7
MacColl, E., and Khalil, R. A. (2015). Matrix metalloproteinases as regulators of vein structure and function: implications in chronic venous disease. J. Pharmacol. Exp. Ther. 355 (3), 410–428. Epub 20150828. doi:10.1124/jpet.115.227330
Marichal, T., Starkl, P., Reber, L. L., Kalesnikoff, J., Oettgen, H. C., Tsai, M., et al. (2013). A beneficial role for immunoglobulin E in host defense against honeybee venom. Immunity 39 (5), 963–975. Epub 20131024. doi:10.1016/j.immuni.2013.10.005
Marta, B., Federica, Z., Alfredo, R., Patrick, K., Vladimir, E. E., Amos, G., et al. (2014). Abnormal interactions between perifollicular mast cells and Cd8+ T-cells may contribute to the pathogenesis of alopecia areata. PLoS One 9 (5), e94260. doi:10.1371/journal.pone.0094260
Meixiong, J., Anderson, M., Limjunyawong, N., Sabbagh, M. F., Hu, E., Mack, M. R., et al. (2019). Activation of mast-cell-expressed mas-related G-protein-coupled receptors drives non-histaminergic itch. Immunity 50 (5), 1163–1171. Epub 20190423. doi:10.1016/j.immuni.2019.03.013
Melo-Cardenas, J., Bezavada, L., Crawford, J. C., Gurbuxani, S., Cotton, A., Kang, G., et al. (2022). Il-13/Il-4 signaling contributes to fibrotic progression of the myeloproliferative neoplasms. Blood 140 (26), 2805–2817. doi:10.1182/blood.2022017326
Merluzzi, S., Frossi, B., Gri, G., Parusso, S., Tripodo, C., and Pucillo, C. (2010). Mast cells enhance proliferation of B lymphocytes and drive their differentiation toward iga-secreting plasma cells. Blood 115 (14), 2810–2817. Epub 20100125. doi:10.1182/blood-2009-10-250126
Moiseeva, E. P., Roach, K. M., Leyland, M. L., and Bradding, P. (2013). Cadm1 is a key receptor mediating human mast cell adhesion to human lung fibroblasts and airway smooth muscle cells. PLoS One 8 (4), e61579. Epub 20130419. doi:10.1371/journal.pone.0061579
Nicolas, G., Camille, L., Salvatore, V., and Eric, E. (2013). Human mast cells drive memory Cd4+ T cells toward an inflammatory il-22+ phenotype. J. Allergy Clin. Immunol. 131 (5), 1400–1407. doi:10.1016/j.jaci.2013.01.029
Oka, T., Kalesnikoff, J., Starkl, P., Tsai, M., and Galli, S. J. (2012). Evidence questioning cromolyn's effectiveness and selectivity as a 'mast cell stabilizer' in mice. Lab. Invest 92 (10), 1472–1482. Epub 20120820. doi:10.1038/labinvest.2012.116
Petri, T. K., and Ilze, B. (2017). Mast cells in atherosclerotic cardiovascular disease - activators and actions. Eur. J. Pharmacol. 816 (0), 37–46. doi:10.1016/j.ejphar.2017.10.013
Piliponsky, A., and Romani, L. (2018). The contribution of mast cells to bacterial and fungal infection immunity. Immunol. Rev. 282 (1), 188–197. doi:10.1111/imr.12623
Pincha, N., Hajam, E. Y., Badarinath, K., Batta, S. P. R., Masudi, T., Dey, R., et al. (2018). Pai1 mediates fibroblast-mast cell interactions in skin fibrosis. J. Clin. Invest 128 (5), 1807–1819. Epub 20180326. doi:10.1172/jci99088
Reber, L. L., Sibilano, R., Starkl, P., Roers, A., Grimbaldeston, M. A., Tsai, M., et al. (2017). Imaging protective mast cells in living mice during severe contact hypersensitivity. JCI Insight 2 (9), e92900. Epub 20170504. doi:10.1172/jci.insight.92900
Régis, J., Idaira María, G.-F., Tamara, G., Jonathon, A. C., Monja, S., Laura, V.-M., et al. (2022). Neutrophil breaching of the blood vessel pericyte layer during diapedesis Requires mast cell-derived il-17a. Nat. Commun. 13 (1), 7029. doi:10.1038/s41467-022-34695-7
Ribatti, D., Tamma, R., and Annese, T. (2020). Mast cells and angiogenesis in multiple sclerosis. Inflamm. Res. 69 (11), 1103–1110. Epub 20200817. doi:10.1007/s00011-020-01394-2
Rivellese, F., Suurmond, J., Habets, K., Dorjée, A. L., Ramamoorthi, N., Townsend, M. J., et al. (2015). Ability of interleukin-33- and immune complex-triggered activation of human mast cells to down-regulate monocyte-mediated immune responses. Arthritis Rheumatol. 67 (9), 2343–2353. doi:10.1002/art.39192
R, M., N, J. T., E, A. R., S, N. A., and J, D. P. (1996). Mast cells process bacterial ags through a phagocytic route for class I mhc presentation to T cells. J. Immunol. 156 (4), 1490–1496. doi:10.4049/jimmunol.156.4.1490
Roy, S., Chompunud Na Ayudhya, C., Thapaliya, M., Deepak, V., and Ali, H. (2021). Multifaceted Mrgprx2: new insight into the role of mast cells in health and disease. J. Allergy Clin. Immunol. 148 (2), 293–308. Epub 20210504. doi:10.1016/j.jaci.2021.03.049
Rurik, J. G., Aghajanian, H., and Epstein, J. A. (2021). Immune cells and immunotherapy for cardiac injury and repair. Circ. Res. 128 (11), 1766–1779. Epub 20210527. doi:10.1161/circresaha.121.318005
Sahar, L.-E., Brant, R. W., Quang, T. L., Andrea, L. P., Masoud, H. M., Judith, A. W., et al. (2017). Human mast cells present antigen to autologous Cd4(+) T cells. J. Allergy Clin. Immunol. 141 (1). doi:10.1016/j.jaci.2017.02.048
Stephen, J. G., Nicolas, G., and Mindy, T. (2020). Mast cells in inflammation and disease: recent progress and ongoing concerns. Annu. Rev. Immunol. 38 (0), 49–77. doi:10.1146/annurev-immunol-071719-094903
Tahereh, D., Joshua, A. B., and Daniel, F. D. (2022). Defining mast cell differentiation and heterogeneity through single-cell transcriptomics analysis. J. Allergy Clin. Immunol. 150 (4), 739–747. doi:10.1016/j.jaci.2022.08.011
Tauber, M., Basso, L., Martin, J., Bostan, L., Pinto, M. M., Thierry, G. R., et al. (2023). Landscape of mast cell populations across organs in mice and humans. J. Exp. Med. 220 (10), e20230570. Epub 20230718. doi:10.1084/jem.20230570
Terhorst-Molawi, D., Hawro, T., Grekowitz, E., Kiefer, L., Merchant, K., Alvarado, D., et al. (2023). Anti-kit antibody, barzolvolimab, reduces skin mast cells and disease activity in chronic inducible urticaria. Allergy 78 (5), 1269–1279. Epub 20221203. doi:10.1111/all.15585
Theodoropoulou, S., Copland, D. A., Liu, J., and Dick, A. D. (2015). Role of interleukin 33/st2 Axis in the immune-mediated pathogenesis of age-related macular degeneration. Lancet 385 (Suppl. 1), S97. doi:10.1016/s0140-6736(15)60412-3
Thomas, P., Rebecca, B., Robin, T., Fuwei, S., Daniel, P., Candice, F., et al. (2023). Mast cells link immune sensing to antigen-Avoidance behaviour. Nature 620 (7974), 634–642. doi:10.1038/s41586-023-06188-0
Tikoo, S., Barki, N., Jain, R., Zulkhernain, N. S., Buhner, S., Schemann, M., et al. (2018). Imaging of mast cells. Immunol. Rev. 282 (1), 58–72. Epub 2018/02/13. doi:10.1111/imr.12631
Toyoshima, S., and Okayama, Y. (2022). Neuro-allergology: mast cell-nerve cross-talk. Allergol. Int. 71 (3), 288–293. Epub 20220607. doi:10.1016/j.alit.2022.04.002
Valeri, V., Tonon, S., Vibhushan, S., Gulino, A., Belmonte, B., Adori, M., et al. (2021). Mast cells crosstalk with B cells in the gut and sustain iga response in the inflamed intestine. Eur. J. Immunol. 51 (2), 445–458. Epub 20201014. doi:10.1002/eji.202048668
Van Bruggen, S., Jarrot, P. A., Thomas, E., Sheehy, C. E., Silva, C. M. S., Hsu, A. Y., et al. (2023). Nlrp3 is essential for neutrophil polarization and chemotaxis in response to leukotriene B4 gradient. Proc. Natl. Acad. Sci. U. S. A. 120 (35), e2303814120. Epub 20230821. doi:10.1073/pnas.2303814120
Verma, S. K., Garikipati, V. N. S., Krishnamurthy, P., Schumacher, S. M., Grisanti, L. A., Cimini, M., et al. (2017). Interleukin-10 inhibits bone marrow fibroblast progenitor cell-mediated cardiac fibrosis in pressure-overloaded myocardium. Circulation 136 (10), 940–953. Epub 20170630. doi:10.1161/circulationaha.117.027889
Viviana, V., Silvia, T., Shamila, V., Alessandro, G., Beatrice, B., Monika, A., et al. (2020). Mast cells crosstalk with B cells in the gut and sustain iga response in the inflamed intestine. Eur. J. Immunol. 51 (2), 445–458. doi:10.1002/eji.202048668
Wu, C., Boey, D., Bril, O., Grootens, J., Vijayabaskar, M. S., Sorini, C., et al. (2022). Single-cell transcriptomics reveals the identity and regulators of human mast cell progenitors. Blood Adv. 6 (15), 4439–4449. doi:10.1182/bloodadvances.2022006969
Wulff, B. C., Pappa, N. K., and Wilgus, T. A. (2019). Interleukin-33 encourages scar formation in murine fetal skin wounds. Wound Repair Regen. 27 (1), 19–28. Epub 20181123. doi:10.1111/wrr.12687
Yaksh, T. L., Eddinger, K. A., Kokubu, S., Wang, Z., DiNardo, A., Ramachandran, R., et al. (2019). Mast cell degranulation and fibroblast activation in the morphine-induced spinal mass: role of mas-related G protein-coupled receptor signaling. Anesthesiology 131 (1), 132–147. doi:10.1097/aln.0000000000002730
Yamamoto, T., Hartmann, K., Eckes, B., and Krieg, T. (2000). Mast cells enhance contraction of three-dimensional collagen lattices by fibroblasts by cell-cell interaction: role of stem cell factor/C-kit. Immunology 99 (3), 435–439. doi:10.1046/j.1365-2567.2000.00973.x
Yana, H., Frank, S., Max, L., Marcus, M., and Melba, M. (2021). Mast cells modulate antigen-specific Cd8(+) T cell activation during lcmv infection. Front. Immunol. 12 (0), 688347. doi:10.3389/fimmu.2021.688347
Younesi, F. S., Miller, A. E., Barker, T. H., Rossi, F. M. V., and Hinz, B. (2024). Fibroblast and myofibroblast activation in normal tissue repair and fibrosis. Nat. Rev. Mol. Cell Biol. 25 (8), 617–638. Epub 20240408. doi:10.1038/s41580-024-00716-0
Yuki, F., Tatsuro, N., Toko, M., Akane, H., Kosuke, A., and Takahisa, M. (2023). Mast cell-derived prostaglandin D(2) limits the subcutaneous absorption of honey bee venom in mice. Proc. Natl. Acad. Sci. U. S. A. 120 (22), e2300284120. doi:10.1073/pnas.2300284120
Zhang, W., Chancey, A. L., Tzeng, H. P., Zhou, Z., Lavine, K. J., Gao, F., et al. (2011). The development of myocardial fibrosis in transgenic mice with targeted overexpression of tumor necrosis factor requires mast cell-fibroblast interactions. Circulation 124 (19), 2106–2116. Epub 20111024. doi:10.1161/circulationaha.111.052399
Zhang, X., Li, J., Luo, S., Wang, M., Huang, Q., Deng, Z., et al. (2020). Ige contributes to atherosclerosis and obesity by affecting macrophage polarization, macrophage protein network, and foam cell formation. Arterioscler. Thromb. Vasc. Biol. 40 (3), 597–610. Epub 20200130. doi:10.1161/atvbaha.119.313744
Keywords: mast cell, inflammation, fibrosis, fibroblast, innate immunity, adaptive immunity
Citation: Wang X, Zhang P, Tang Y, Chen Y, Zhou E and Gao K (2024) Mast cells: a double-edged sword in inflammation and fibrosis. Front. Cell Dev. Biol. 12:1466491. doi: 10.3389/fcell.2024.1466491
Received: 19 July 2024; Accepted: 26 August 2024;
Published: 17 September 2024.
Edited by:
Katarzyna Nazimek, Jagiellonian University Medical College, PolandReviewed by:
Gregorio Gomez, University of Houston, United StatesCopyright © 2024 Wang, Zhang, Tang, Chen, Zhou and Gao. This is an open-access article distributed under the terms of the Creative Commons Attribution License (CC BY). The use, distribution or reproduction in other forums is permitted, provided the original author(s) and the copyright owner(s) are credited and that the original publication in this journal is cited, in accordance with accepted academic practice. No use, distribution or reproduction is permitted which does not comply with these terms.
*Correspondence: Kun Gao, ZHIuZ2Fva3VuQG91dGxvb2suY29t