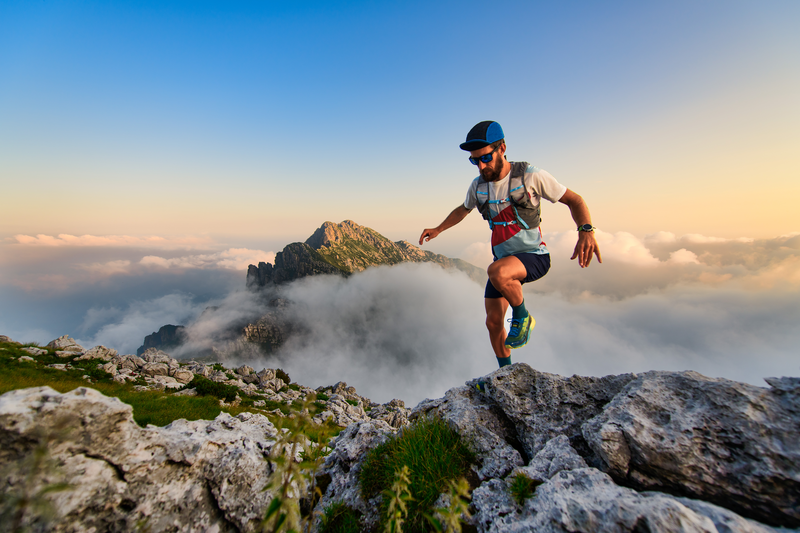
94% of researchers rate our articles as excellent or good
Learn more about the work of our research integrity team to safeguard the quality of each article we publish.
Find out more
REVIEW article
Front. Cell Dev. Biol. , 10 June 2024
Sec. Signaling
Volume 12 - 2024 | https://doi.org/10.3389/fcell.2024.1394339
This article is part of the Research Topic Revolutionizing Cancer Treatment: Navigating the Intricate Landscape of Cellular Signaling Networks View all 8 articles
Osteosarcoma, a malignant bone tumor predominantly affecting children and adolescents, presents significant therapeutic challenges, particularly in metastatic or recurrent cases. Conventional surgical and chemotherapeutic approaches have achieved partial therapeutic efficacy; however, the prognosis for long-term survival remains bleak. Recent studies have highlighted the imperative for a comprehensive exploration of the osteosarcoma immune microenvironment, focusing on the integration of diverse immunotherapeutic strategies—including immune checkpoint inhibitors, tumor microenvironment modulators, cytokine therapies, tumor antigen-specific interventions, cancer vaccines, cellular therapies, and antibody-based treatments—that are directly pertinent to modulating this intricate microenvironment. By targeting tumor cells, modulating the tumor microenvironment, and activating host immune responses, these innovative approaches have demonstrated substantial potential in enhancing the effectiveness of osteosarcoma treatments. Although most of these novel strategies are still in research or clinical trial phases, they have already demonstrated significant potential for individuals with osteosarcoma, suggesting the possibility of developing new, more personalized and effective treatment options. This review aims to provide a comprehensive overview of the current advancements in osteosarcoma immunotherapy, emphasizing the significance of integrating various immunotherapeutic methods to optimize therapeutic outcomes. Additionally, it underscores the imperative for subsequent research to further investigate the intricate interactions between the tumor microenvironment and the immune system, aiming to devise more effective treatment strategies. The present review comprehensively addresses the landscape of osteosarcoma immunotherapy, delineating crucial scientific concerns and clinical challenges, thereby outlining potential research directions.
Osteosarcoma, the most prevalent bone tumor among children and adolescents, is characterized by the formation of osteoid and immature bone at the metaphysis and diaphysis of long bones (Mirabello et al., 2009). Accounting for approximately 20% of all bone malignancies, this highly aggressive tumor is renowned for its aggressive proliferation and infiltration capabilities, frequently leading to rapid invasion of adjacent bone tissue and structures, subsequently resulting in significant bone destruction and functional impairment. Given its propensity for early pulmonary metastasis and aggressive characteristics, the treatment of osteosarcoma presents considerable challenges (Durfee et al., 2016; Harrison et al., 2018). Although surgical resection combined with neoadjuvant chemotherapy, involving agents such as doxorubicin, cisplatin, methotrexate, and ifosfamide, is proven to be effective in managing primary lesions, there is a notable absence of efficacious treatments for metastatic osteosarcoma (Ta et al., 2009). Remarkably, while the five-year survival rate for patients with localized osteosarcoma exceeds 78%, this rate falls to 25% for metastatic or recurrent osteosarcoma (Gaspar et al., 2018), highlighting the urgent necessity for innovative therapeutic approaches for osteosarcoma.
Recent research has increasingly focused on the tumor microenvironment (TME) of osteosarcoma, aiming to elucidate the tumor’s heterogeneity, progression, and metastasis, thereby contributing insights into this highly aggressive tumor. Osteosarcoma proliferates within a complex, dynamic bone microenvironment comprising osteoblasts, stromal cells, vascular cells, immune cells, and mineralized extracellular matrix (ECM) (Alfranca et al., 2015). Crucial to tumor progression are the intricate interactions among osteosarcoma cells and the adjacent microenvironment, influencing tumor progression, apoptosis, invasion, metastasis, angiogenesis, pre-metastatic niche formation, and therapeutic response (Tsagozis et al., 2020; Zhu et al., 2022). Characterized by considerable immune cell infiltration, the osteosarcoma-adjacent tissue manifests an intricate immune microenvironment, facilitating the intraosseous proliferation and persistence of osteosarcoma cells in an immunosuppressive background (Pierrevelcin et al., 2022; Somaiah et al., 2022). The components of this microenvironment are predominantly categorized into cellular elements, including tumor-associated macrophages (TAMs), tumor-associated neutrophils (TANs), myeloid-derived suppressor cells (MDSCs), mast cells (MCs), T cells, B cells, natural killer (NK) cells, dendritic cells (DCs), and non-cellular components such as mesenchymal stem cells (MSCs) and circulating tumor cells (CTCs), all of which contribute to interactions with the immune system, thereby promoting suppressive networks (Zheng Y. et al., 2018). Additionally, research involves the complement system and exosomes, recognized for their specific immune responses (Mathieu et al., 2019). The immunosuppressive microenvironment’s intensity correlates with increased activity of molecules including indoleamine 2,3-dioxygenase (IDO), programmed cell death protein 1 (PD-1), interleukin-10 (IL-10), transforming growth factor-beta (TGF-β), vascular endothelial growth factor (VEGF), and signal transducer and activator of transcription 3 (STAT3), primarily mediated by MDSCs, TAMs, and regulatory T lymphocytes (Tregs) with immunosuppressive characteristics (Xie et al., 2022; Kuo et al., 2023). Therefore, it is essential to elucidate the interactions between osteosarcoma cells and immune cells to develop the effectiveness of immunotherapies.
Recent trials have explored the efficacy of PD-1 and CTLA-4 targeted immunotherapies in osteosarcoma (Wang et al., 2016; Ratti et al., 2017; Hennessy et al., 2021). Subsequent clinical studies, however, have indicated a restrained response to anti-PD-1 immunotherapy among a limited patient cohort. Furthermore, the efficacy of anti-CTLA-4 immunotherapy in osteosarcoma clinical treatment remains ambiguous (Thanindratarn et al., 2019). The interactions among cancer, immune, and stromal cells in osteosarcoma lead to the development of an immunosuppressive TME, thereby facilitating cancer cells’ evasion of immune responses and contributing to the heterogeneity of the tumor, which is associated with treatment resistance and differences in patient responses (Suehara et al., 2019). Comprehending the heterogeneity of cancer cells and the dynamic tumor immune microenvironment might identify novel therapeutic targets for the treatment of osteosarcoma.
In conclusion, a profound exploration of the tumor’s biological characteristics and cellular interactions within its microenvironment is essential for the development of therapeutic strategies for osteosarcoma, particularly crucial for the management of metastatic osteosarcoma. This review comprehensively explores osteosarcoma from aspects including its pathophysiological characteristics, mechanisms of development, along with the contribution of immune cells in the tumor’s microenvironment. A novel approach is proposed to improve treatment efficacy by modulating the immune cells within the TME. The current review is innovative in its comprehensive investigation of the osteosarcoma immune microenvironment and its role in oncological progression, suggesting therapeutic strategies incorporating immune checkpoint inhibitors (ICIs), TME modulators, and cytokine therapy. These approaches focus on the intricate immune microenvironment of osteosarcoma, providing innovative treatment perspectives and methods, particularly for complicated cases of metastasis.
In osteosarcoma, TAMs are identified as predominant components, comprising approximately half of the tumor mass, an observation that highlights their substantial role within the tumor’s immune microenvironment (Huang et al., 2021). Demonstrating considerable flexibility and heterogeneity, these cells impact tumor characteristics by either promoting or suppressing tumor functions, dependent on tumor type and specific TME interactions (Mantovani et al., 2017; Kielbassa et al., 2019). TAMs manifesting M1-like features are generally associated with anti-tumorigenic effects, capable of inducing tumor cell apoptosis and enhancing immune responses, whereas most, exhibiting an M2-like phenotype with immunosuppressive properties, predominantly facilitate angiogenesis, promote extracellular matrix invasion, and enable immune evasion, thereby contributing to tumor advancement and metastasis (Noy and Pollard, 2014; Franklin and Li, 2016). Such dichotomy highlights the imperative to comprehend the regulatory mechanisms controlling macrophage polarization and the subsequent roles in osteosarcoma.
Within osteosarcoma, the role of TAMs is essential, notably in tumor progression and the advancement of metastasis. Han et al. indicated that in osteosarcoma, M2-type TAMs enhance the function of TIM-3(+)PD-1(+) T cells, thereby intensifying T cell immunosuppression within the TME (Han et al., 2016). Additionally, the eradication of these TAMs enhances T cell proliferation and pro-inflammatory cytokine secretion, according to findings from Uehara et al., metformin treatment stimulates a conversion in TAMs from M2 to M1 phenotype, as indicated by elevated IL-12 and TNF-α levels, MHC class II upregulation, and CD206 downregulation, indicating a potential transition of TAMs from tumor-enhancing to tumor-restraining roles following metformin administration (Uehara et al., 2019). Li et al. indicated that ZIM3 in osteosarcoma cells induces CCL25 expression, as a result, leading M2-type TAMs to pulmonary metastatic nodules, thus promoting metastatic proliferation and highlighting the adverse impact of TAM infiltration in osteosarcoma (Li et al., 2023). Lin et al. elucidated that in osteosarcoma, MerTK-mediated phagocytosis promotes macrophage M2 polarization and PD-L1 expression via the p38/STAT3 pathway, intensifying immune tolerance and tumor progression, emphasizing the adverse impact of M2 polarization in increasing tumor aggressiveness (Lin et al., 2022). Liu et al. indicated that miR-221-3p, derived from M2-type TAMs, enhanced osteosarcoma cell proliferation and invasion via the JAK2/STAT3 pathway, targeting SOCS3, thereby exacerbating tumor malignancy (Liu et al., 2021). Cheng et al. highlighted the impact of osteosarcoma-derived exosomes in macrophage M2-type polarization mediated by Tim-3, along with their release of cytokines, particularly IL-10, TGF-β, and VEGF, facilitating increased cell migration, invasion, EMT, and lung metastasis (Cheng et al., 2021). Collectively, TAMs’ functions and polarization in the osteosarcoma microenvironment critically influence tumor progression. Therapeutic approaches such as the utilization of the CSF1/CSF1R inhibitor Pexidartinib (PLX3397) have shown promise in counteracting M2-type TAM polarization, thereby mitigating its tumor-promoting effects and demonstrating the potential for tumor growth and metastasis suppression through TAM polarization modulation (Fujiwara et al., 2021). Ségaliny et al. demonstrated that IL-34, with its expression upregulated in osteosarcoma, markedly enhances angiogenesis and recruits M2-type macrophages, a circumstance associated with enhanced M2-TAM infiltration, thereby emphasizing the role of M2-TAMs in the advancement of osteosarcoma (Segaliny et al., 2015). This finding is consistent with Zhou et al.’s research, which employed single-cell RNA analysis across different phases of osteosarcoma progression, identifying critical gene expression patterns in tumor cells and the surrounding microenvironment, with particular emphasis on variations in immune cells, and detected substantial infiltration of pro-inflammatory FABP4+ macrophages in lung metastatic osteosarcoma (Zhou et al., 2020a). Additionally, Wolf-Dennen et al. revealed that exosomes released by metastatic osteosarcoma cells initiate a conversion in alveolar macrophages towards a tumor-promoting M2 phenotype by activating IL10, TGFB2, and CCL22 mRNA, a process correlated with diminished capability in eradicating apoptotic cells and tackling tumor cells, thereby contributing to increased immune suppression and tumor progression in osteosarcoma (Wolf-Dennen et al., 2020). Through scRNA-Seq analysis of osteosarcoma lung metastases, Zheng et al. established that in tumor metastasis, TAMs predominantly manifest an immunosuppressive M2 phenotype, with PD-1 expression impeding phagocytic function and advancing tumor metastasis and progression (Zheng C. et al., 2024). Chen et al. identified that TIPE1 mitigates tumor growth by reducing macrophage infiltration, suggesting a potential new target for osteosarcoma treatment (Chen et al., 2019). Han et al. observed that osteosarcoma-associated macrophages activate the COX-2/STAT3 axis to enhance tumor metastasis and invasion, with COX-2 inhibition reducing metastasis (Han et al., 2019). Studies also demonstrated that IL13 and IL4 induce M2-phenotype macrophage polarization in the osteosarcoma microenvironment, impacting tumor cell invasiveness and metastatic capability, with MMP12 and other factors secreted by M2-phenotype macrophages playing a crucial role in osteosarcoma dissemination (All-Trans Retinoic Acid Prevents, 2020). Similarly, osteosarcoma macrophages were observed to secrete exosomes with lncRNA LIFR-AS1, considerably enhancing tumor cell growth and invasiveness via the miR-29a/NFIA pathway, and simultaneously reducing the frequency of apoptosis (Zhang H. et al., 2021). Furthermore, the application of TLR9 agonists in osteosarcoma treatments has been effective in constraining tumor growth by reducing infiltration of M2-type macrophages (Cascini et al., 2023). In Ewing’s sarcoma treatment, liposomal chloroquine and trabectedin effectively reduced M2-phenotype macrophages, thereby enhancing the effectiveness of oncolytic virotherapy and further confirming the crucial role of M2-phenotype macrophages in tumor progression (Denton et al., 2018). Nirala et al. elucidated that upregulation of microRNA 17/20a by the MYC gene leads to decreased levels of macrophage colony-stimulating factor 1 (CSF1), culminating in a reduced macrophage population within the TME, potentially influencing osteosarcoma progression and metastasis (Nirala et al., 2023). Similarly, the study by Zhang et al. on microRNA let-7a demonstrated its suppressive effect on Ewing’s sarcoma’s malignant characteristics through the inhibition of macrophage infiltration, emphasizing the adverse influence of TAMs in tumor advancement (Zhang et al., 2016). Additionally, Chim et al.'s research in the osteosarcoma microenvironment determined that TAMs exacerbate inflammation and enhance resistance to chemotherapeutic drugs, indicating an adverse effect of these macrophages on tumor progression (Chim et al., 2023). Yan et al. observed that exosomes from TAMs, mediated through exosome-let-7a, advance osteosarcoma metastasis, emphasizing TAMs’ significant role in advancing osteosarcoma (Yan et al., 2023). In addition, studies indicated that curcumin enhanced cisplatin’s (CDDP) anti-tumor effect by inhibiting M2-type macrophage polarization, suggesting a correlation between M2-type macrophage infiltration and tumor chemotherapy resistance (Wang J. et al., 2022). Li et al.’s study on osteosarcoma-derived microparticles (T-MPs) indicated initiation of macrophage polarization towards the M2 phenotype through the TBK1-STAT6 pathway, and manipulation of osteosarcoma cell conduct via the CCL18/STAT3 signaling pathway, thereby enhancing migration ability and chemotherapeutic resistance (Li et al., 2024). Consequently, Liang et al.'s findings showed that macrophages decrease the sensitivity of osteosarcoma cells to chemotherapy agents by secreting IL-1β during neoadjuvant chemotherapy, implying a potential decrease in chemotherapy efficacy in the osteosarcoma microenvironment (Liang et al., 2020). However, macrophages have been observed to exhibit anti-tumor functions under certain conditions. Gomez-Brouchet et al. indicated that elevated levels of CD163-positive macrophages in osteosarcoma are associated with improved prognosis, implying a positive influence on immune regulation in the TME (Gomez-Brouchet et al., 2017). Studies demonstrated that SKP2 deletion results in a proliferation of macrophages, correlating with a favorable prognosis in osteosarcoma patients, indicating a suppressive effect on tumor development and dissemination in the Rb1/p53-deficient mouse osteosarcoma model (Ferrena et al., 2023).
Withers et al. identified a correlation between enhanced CD204+ macrophage infiltration in canine osteosarcoma tissues and prolonged disease-free survival, underscoring macrophages’ potential advantageous impact in managing osteosarcoma progression (Withers et al., 2019). Within the osteosarcoma microenvironment, macrophages exhibit two polarization states: the pro-inflammatory M1 and the tumor-promoting M2. Richert et al. revealed the therapeutic potential of M1 macrophages in inhibiting tumor growth by promoting the conversion from M2 to M1 phenotype, offering insights into leveraging the tumor-suppressive capabilities of M1 macrophages (Richert et al., 2023). Pahl et al. observed that M1 macrophages, activated by LPS and IFN-γ, effectively suppress osteosarcoma cell proliferation, demonstrating an advantageous role in osteosarcoma immunotherapy (Pahl et al., 2014). Furthermore, Ji et al. confirmed that osteosarcoma tumor thrombi contain a substantial proportion of M1-like macrophages (TAM-M1), exhibiting an immunostimulatory state, positively influencing the activation of anti-tumor immune responses (Ji et al., 2023). Simultaneously, according to Gong et al., MPIRx nanoparticles prompt a specific mechanism causing macrophage polarization towards the M1 phenotype, thereby augmenting macrophage phagocytic function against osteosarcoma cells and contributing to an anti-tumor effect (Gong et al., 2023). Dumars et al. observed a substantial presence of M1 macrophages in non-metastatic osteosarcoma (OS Meta-), suggesting a potential role in impeding tumor metastasis (Dumars et al., 2016). Growing comprehension of TAMs underscores the potential for developing strategies that enhance immunotherapy effectiveness in osteosarcoma, focusing on the distinct polarization states of TAMs to advantageously impact tumor progression.
In the TME, MDSCs are essential in suppressing the immune response. Predominantly, this suppression functions via interactions with T lymphocytes, resulting in the generation of reactive oxygen species (ROS) and the depletion of L-arginine. Such processes obstruct T cell proliferation and facilitate apoptosis, ultimately diminishing immune functionality (Joshi and Sharabi, 2022). Distinct MDSCs subtypes suppress T cell activity through diverse mechanisms: PMN-MDSCs primarily generate ROS via activation of STAT3 and upregulation of NADPH oxidase, while M-MDSCs predominantly produce nitric oxide (NO) by activating STAT1 and upregulating NO synthase, thus inhibiting T cell function. MDSCs attenuate acquired anti-tumor immunity alongside diminishing innate anti-tumor responses. In addition to T cells, MDSCs also suppress the functions of NK cells and DCs (Yang et al., 2020). Additionally, comprehensive research demonstrates that MDSCs instigate T cell inactivation through increased PD-L1 expression, interacting with PD-1 on T cells (Noman et al., 2014). CD155 expression on MDSCs enhances MDSCs-mediated T cell suppression, with significant reduction of MDSCs immunosuppressive activity in vitro using anti-TIGIT antibodies against TIGIT/CD155 pathway (Wu et al., 2019). These results collectively underscore that immune checkpoint molecules on MDSCs adversely regulate T cell function.
Specifically, Fan et al. demonstrated an innovative Metal-Organic Framework (MOF) nanosystem in treating osteosarcoma, offering concurrent chemo-immunotherapy and targeting MDSCs alongside modifying IDO activity, thereby substantially reducing tumor progression through a focus on the critical function of MDSCs (Fan et al., 2022). Additionally, the SDF-1/CXCR4 axis in the osteosarcoma microenvironment was found to contribute to MDSCs accumulation, diminishing the effectiveness of PD-1 therapy, and resulting in MDSCs infiltration that impeded the proliferation of cytotoxic T cells, demonstrating MDSCs’ adverse role in osteosarcoma (Jiang et al., 2019). Guan et al. identified a positive correlation between IL-18 and MDSCs accumulation in osteosarcoma, potentially facilitating MDSCs migration and tumor infiltration. Combining anti-IL-18 with anti-PD1 therapy effectively reduced levels of G-MDSC and M-MDSC, enhancing T cell infiltration and immune function, providing a new strategy for osteosarcoma immunotherapy (Guan et al., 2017). Shi et al. found a significant increase in MDSCs in the peripheral blood of osteosarcoma patients, with these cells promoting tumor immune evasion by inhibiting T cell activation and infiltration, thereby obstructing effective immune responses (Shi et al., 2019). Long et al. observed that MDSCs in the osteosarcoma TME significantly impede the function of GD2-CAR T cells, thus impairing the efficacy of these cells in osteosarcoma treatment and highlighting a negative immunoregulatory role of MDSCs in osteosarcoma (Long et al., 2016). Uehara et al. revealed that metformin significantly reduces abundance of MDSCs in osteosarcoma, favorably contributing to tumor growth control, highlighting the negative influence of MDSCs in osteosarcoma (Uehara et al., 2019). Post-neoadjuvant chemotherapy, Deng et al. noted a decrease in the quantity of HLA-DR-CD33+ MDSCs. Given their role in suppressing immune responses within the TME, this reduction might enhance the immune response against the tumor (Deng et al., 2020). Lastly, Kansara et al. showed that infiltrating myeloid cells in osteosarcoma influence IL23 through GRM4, contributing to tumor progression and indicative of a poor prognosis (Kansara et al., 2019).
T lymphocytes, comprising the second most prevalent infiltrating cell type within the osteosarcoma TME, are functionally classified into cytotoxic T lymphocytes (CTLs), helper T lymphocytes (Th cells), and regulatory T cells (Tregs) (Itahashi et al., 2022). Overall, T lymphocytes exhibit a considerable heterogeneity and play a pivotal role in the immunotherapy of osteosarcoma. Notably, tumor-infiltrating lymphocytes (TILs) have been detected in 75% of osteosarcoma cases, with a higher incidence of 86% observed in metastatic osteosarcoma (Heymann et al., 2019).
Research has highlighted the essential role of CD8+ T cells and additional T cell subsets in osteosarcoma treatment. Investigations by Qi et al., through single-cell RNA sequencing, uncovered that low expression of GPR65 in osteosarcoma is prognostically unfavorable, and that its expression is positively connected with the enhanced activity and infiltration of immune cells, especially CD4+ and CD8+ T cells, that obstruct osteosarcoma progression (Qi et al., 2024). Subsequent investigations revealed that inhibition of c-Myc in osteosarcoma augmented the tumor immune microenvironment by elevating T cell chemokines and stimulating the CD40/CD40L pathway, facilitating the infiltration and activation of CTLs, highlighting the positive contribution of T cells in osteosarcoma treatment (Jiang et al., 2022). In addition, research conducted by Liu et al. elucidated modifications in the osteosarcoma immune microenvironment, specifically the perturbation of peripheral blood T lymphocyte subsets during osteosarcoma development, characterized by an increase in γδ T cells and Tregs, a decrease in CD8+ T cells, concomitant with peripheral immune suppression (Liu Q. et al., 2022). Ligon et al. underscored the distinctive response of T cells in the metastatic osteosarcoma TME, particularly at the tumor-lung interface, where T cells, resembling TILs, manifested significant immune suppression, with increased expression of immunosuppressive markers including PD-1 and LAG-3 (Ligon et al., 2021). Helm et al. observed a significant increase in CD8+ T cell infiltration in non-directly irradiated tumor areas following combined carbon ion radiotherapy and immune checkpoint inhibitor treatment, emphasizing the critical role of CD8+ T cells in limiting tumor growth and reducing lung metastases (Helm et al., 2021). Research by Yahiro et al. also indicated that activation of the TLR4 signaling pathway enhances the infiltration of CD8 positive cytotoxic lymphocytes in osteosarcoma lung metastases, effectively inhibiting osteosarcoma growth and metastasis, further confirming the importance of CD8+ cells in suppressing osteosarcoma progression (Yahiro et al., 2020). The infiltration of CD8 positive lymphocytes was significantly associated with improved survival in osteosarcoma patients treated with zoledronic acid, suggesting a positive immunoregulatory role of CD8 positive lymphocytes in inhibiting osteosarcoma growth and metastasis (Gomez-Brouchet et al., 2017). Research by Tang et al. revealed that upregulation of T-synthase in osteosarcoma enhances CD8+ T cell proliferation and function, thereby inhibiting tumor growth, while CD4+ T cells exert a suppressive effect on tumor cells through IFN-γ secretion (Tang et al., 2023). Moreover, the combined administration of IDO inhibitors and platinum(IV) prodrugs initiated the cGAS-STING pathway, enhancing CD8+ T cell function, which correlated with a more effective immune response and chemotherapy outcome, demonstrating potential in inhibiting tumor proliferation and dissemination (Xiang et al., 2023). Similarly, upregulation of miR-140 promoted increased infiltration of CD8+ T cells in the TME, directly related to the inhibition of osteosarcoma growth, suggesting a positive significance of increased CD8+ T cells in enhancing tumor immune response (Ji et al., 2018). However, the immunosuppressive environment introduces challenges to treatment. Research by Schell et al. in an SV40 large T antigen-induced osteosarcoma model found CTLs exhibited reduced responsiveness to particular epitopes, with a substantial reduction in specific CD8+ T cells, denoting tumor progression is associated with a decrease in immune cell efficacy, impacting tumor immune surveillance and response capabilities (Schell et al., 2000). Das et al. further emphasized the potential antitumor role of T cells in the osteosarcoma microenvironment, noting a positive correlation between T cell abundance and certain immune-related genes, such as PD-L1 and CD160 (Das et al., 2021). Additionally, Cillo et al. highlighted the suppressive effect of the TME on CD8+ T cell function, particularly through the expression of co-inhibitory receptors such as PD-1, revealing mechanisms of immune cell functional exhaustion in osteosarcoma recurrence (Cillo et al., 2022). Research by Liu et al. found that upregulation of microRNA-200a in osteosarcoma led to PD-L1-induced suppression of CD8+ T cell function, highlighting its potential as an immunotherapy target (Liu et al., 2020). Moreover, elevated levels of IL-35 in osteosarcoma patients inhibited the antitumor ability of CD8+ T cells, with Treg-secreted IL-35 related to the suppression of CD8+ T cell function (Liu MX. et al., 2019). Wang et al. discovered that Vγ9Vδ2 T cell activation enhanced the ability of CD8+ T cells to counter osteosarcoma, with CD8+ T cells managing osteosarcoma progression through the direct elimination of tumor cells (Wang S. et al., 2023). Wang et al. also observed that elevated PLOD2 expression in osteosarcoma was associated with enhanced CD8+ T cell infiltration, with such infiltration contributing positively to tumor suppression (Wang Z. et al., 2022). Concurrently, Cascio et al. noted that the expression levels of PD-L1 and HVEM in tumors were positively correlated with adjacent T cell infiltration, emphasizing the pivotal function of T cells in tumor immune surveillance (Cascio et al., 2021). Regardless of the challenges of immune escape and immunosuppression, emerging research has revealed strategies to tackle osteosarcoma by stimulating or enhancing T cell function. For instance, Shi et al. observed that the PI3Kδ/γ inhibitor SNA, by reducing MDSCs activity, improved the TME and augmented the effectiveness of anti-PD1 therapy, especially stressing the crucial role of CD8+ T cells’ activation in osteosarcoma (Shi et al., 2019). Furthermore, inhibition of the PD-1/PD-L1 pathway enabled CTLs to effectively attack osteosarcoma cells, contributing to tumor growth and metastasis suppression (Lussier et al., 2015). Similarly, the CD103+ cDC1s vaccine enhanced CD8+ T cell infiltration and activation in tumors, thereby inhibiting tumor growth and metastasis (Zhou et al., 2020b). Wang et al. demonstrated that in osteosarcoma treatment, sorafenib significantly inhibited the increase in PD-L1 expression induced by doxorubicin, thereby promoting the activity and proportion of CD8+ T cells in the TME, further confirming the significance of CD8+ T cells in resisting osteosarcoma (Wang et al., 2023b). Overall, studies consistently demonstrate the critical importance of T lymphocytes, particularly CD8+ T cells, in osteosarcoma treatment, highlighting their influence in impeding tumor development and metastatic development within a complex immunosuppressive environment.
In osteosarcoma’s tumor immune microenvironment, Tregs have been identified as crucial, especially in mechanisms of immune escape. While contributing to immune tolerance, Tregs may be utilized by tumor cells, impeding effective anti-tumor responses and thus emphasizing Tregs’ significance in both tumor biology and immunotherapeutic research (Cheng D. et al., 2023). Elevated levels of microRNA-200a in osteosarcoma, correlating with an increased presence of Tregs (Foxp3+), imply Tregs actively contributing to immune evasion and tumor growth (Liu et al., 2020). Additionally, Li et al. revealed Tregs enhance the immunosuppressive microenvironment in osteosarcoma through Gal9 secretion and activation of the Tim3/Gal9 signaling pathway, thereby reducing the efficacy of Th1-type immune responses and enhancing immune escape mechanisms (Li et al., 2017). Addressing Tregs’ immunosuppressive influence in osteosarcoma, Kohyama et al. utilized an anti-IL-2 monoclonal antibody, S4B6, to deplete Tregs, eliciting an autologous immune response against osteosarcoma cells, significantly reducing both tumor size and lung metastases, underlining the importance of Tregs reduction in amplifying osteosarcoma’s immune response and its antitumor impact (Kohyama et al., 2012). Similarly, Kawano et al. utilized a dendritic cell and anti-GITR antibody combination to reduce Tregs levels, consequently enhancing the immune response to osteosarcoma (Kawano et al., 2015). Subsequent research has substantiated Tregs’ role in facilitating tumor cell immune evasion. Notably, Yoshida et al. reported that anti-PD-1 antibodies reduced Tregs infiltration in osteosarcoma, correlating with tumor growth delay and prolonged survival (Yoshida et al., 2020). Biller et al.'s research on canine osteosarcoma demonstrated an increase in Tregs and a concurrent reduction in the CD8+ T cells to Tregs proportion, correlating with diminished survival times, further emphasizing the importance of Tregs in facilitating tumor immune escape (Biller et al., 2010). Cheng et al. established that in osteosarcoma, Tregs drive tumor progression by activating pathways such as oxidative phosphorylation, angiogenesis, and mTORC1, in addition to their interactions in the TME (Cheng D. et al., 2023). Conclusively, Tregs play a pivotal role in facilitating immune evasion and tumor progression in osteosarcoma, establishing a basis for therapeutic strategies targeting Tregs and introducing novel approaches for osteosarcoma treatment.
NK cells, innate immune cells possessing cytokine production and cytotoxic capabilities, have been identified within the TME of osteosarcoma alongside their role in cytotoxicity and supporting T-cell activation. Specifically, NK cell-mediated IFN-γ release plays a crucial role in the activation of CD4+ T cells and is vital for the proliferation of CD8+ T-cell precursors (Myers and Miller, 2021). Preclinical investigations have shown the capability of NK cells to efficiently eliminate osteosarcoma cells (Razmara et al., 2021).
Notably, the expression of USP6 in Ewing’s sarcoma has been shown to facilitate NK cell invasion and activation, thereby enhancing their tumor cell-killing potential and underscoring the positive impact of NK cell infiltration in impeding tumor growth (Jain et al., 2023). Furthermore, USP6 also stimulates the secretion of immune-related chemokines, augmenting the infiltration and activation of NK cells and additional immunocytes, significantly impeding Ewing’s sarcoma progression (Henrich et al., 2021). Regarding the treatment of osteosarcoma, NK cells have emerged as crucial in controlling tumor progression and metastasis through their ability to recognize and eliminate cancer cells, affirming their infiltration and activation as beneficial in osteosarcoma mitigation (Kisseberth and Lee, 2021). The administration of Valproic Acid (VPA) has been demonstrated to elevate MICA/B expression on osteosarcoma cell surfaces, thus strengthening the NK cells’ cytotoxic activity and further emphasizing their critical role in osteosarcoma cell eradication (Yamanegi et al., 2010). NKT cells, resembling NK cells, significantly contribute to osteosarcoma inhibition. Kansara et al. highlighted the restraining effect of NKT cell infiltration on osteosarcoma development, induced by an immune response during cell senescence regulated by the RB1 gene (Kansara et al., 2013). iNKT cells, recognizing CD1d molecules on tumor cell surfaces, trigger cytotoxic responses that eliminate tumor cells and concurrently enhance the effectiveness of chemotherapeutic agents (Fallarini et al., 2012). Further investigations have revealed that the activation of IL-15 significantly augments the NK cell-mediated eradication of chemotherapy-resistant osteosarcoma cells, an effect dependent on the DNAM-1 and NKG2D pathways, indicating the specificity of NK cells in recognizing and eliminating osteosarcoma cells (Buddingh et al., 2011). Similarly, blocking FSTL1 has been found to strengthen the immune response of NK cells, effectively suppressing osteosarcoma cells and highlighting the advantageous impact of NK cell activation in tumor therapy (Ogiwara et al., 2022). In summary, NK cells play a critical role in the immunosurveillance and suppression within the osteosarcoma TME. Elevating the activation and function of such cells could significantly improve therapeutic efficacy against osteosarcoma, providing pivotal insights for advancing cancer therapy approaches.
DCs are essential in the immune response, recognizing and presenting tumor antigens to helper and cytotoxic T cells and transitioning from immature to mature states, a key process for T cell activation; however, with osteosarcoma progression, tumor cells develop DC resistance, leading to diminished DC activation and consequent immune escape (Le et al., 2021). Specifically, CD103+ cDC1s have been identified as playing a crucial role in T cell activation and enhancing anti-tumor immune responses, particularly when combined with ICIs, exhibiting notable effectiveness against osteosarcoma (Zhou et al., 2020b). Additionally, tumor lysate-pulsed DC vaccines effectively activate the immune system, inhibiting osteosarcoma growth (Kawano et al., 2015), while DCs pulsed with EWS/FLI-l peptide epitopes exhibit significant capabilities in tackling Ewing’s sarcoma (Peng et al., 2014). Radiofrequency ablation (RFA) combined with OK-432 injection significantly increases intratumoral amounts of DCs, affirming the critical role of DCs in inducing distal immune effects and anti-tumor immune responses (Iwai et al., 2020). Moreover, the combined application of oncolytic adenovirus XVir-N-31, driven by YB-1, with CDK4/6 inhibitors, is observed to facilitate the maturation of monocyte-derived DCs, thus increasing their capacity in activating tumor antigen-specific T cells (Schober et al., 2023). However, as osteosarcoma advances, osteosarcoma cells develop resistance mutations against DCs and macrophages, resulting in decreased stimulation of DC activation and ultimately immune escape (Le et al., 2021). Research by Gao et al. revealed that in osteosarcoma patients, DC function is negatively regulated by miR-133a, an increase in which impairs DC maturation and activation, thereby promoting immune escape and tumor growth (Gao et al., 2016). In the immune microenvironment of osteosarcoma, regulatory DCs recruit Tregs, forming an immunosuppressive microenvironment that further facilitates tumor cells to evade immunosurveillance, assisting in tumor proliferation and dissemination (Liu et al., 2023). In summary, DCs exhibit a multifaceted yet crucial role in the immune response to osteosarcoma, capable of counteracting the tumor through immune activation but also potentially leading to immune escape due to resistance mechanisms in the TME. These findings provide critical evidence for potential therapeutic strategies targeting DCs, underscoring the significance of considering the modulation of DC functions in tumor therapy.
In conclusion, the immune microenvironment within osteosarcoma presents a complex landscape characterized by an intricate network of cell signaling mechanisms, regulatory pathways, and cellular interactions. The central functions of DCs, in conjunction with T cells, macrophages, MDSCs, Tregs, and NK cells, converge to influence tumor progression, immune surveillance, and the efficacy of therapeutic interventions. The interplay between immunosuppressive activity and antitumor immunity is determined by cellular dynamics, affecting the tumor’s ability for immune evasion and the effectiveness of immunotherapeutic interventions. The intricate interactions and interactive contributions of immune cells within the osteosarcoma TME, which underscore the investigated mechanisms, are concisely depicted, presenting a diagrammatic elucidation that enhances comprehension of such intricacies (Figure 1). To further illustrate the multifaceted roles and therapeutic targets of these immune cells, Table 1 presents a comprehensive overview of immune cell attributes in the osteosarcoma tumor microenvironment, detailing their diverse functions and roles as therapeutic targets, thereby enriching the understanding of tumor development and immune regulation.
Figure 1. Interactions within the Osteosarcoma Tumor Immune Microenvironment.This schematic illustrates the interplay among immune cells and their cytokines within the osteosarcoma microenvironment, featuring TAMs, DCs, T cells, MDSCs, Tregs, monocytes, and NK cells, with their cytokines—ILs and TNFs—central to their function in the tumor’s immunology. Arrows suggest interactive influences and potential immunotherapeutic targets, summarizing principal components that contribute to osteosarcoma’s immunological functioning and treatment response.
Regarding osteosarcoma treatment, current advancements have highlighted the capacity of varied targeted treatment strategies to counteract this malignancy effectively. Liao et al.’s investigation into sulfatinib, a novel tyrosine kinase inhibitor targeting FGFR1, CSF1R, and VEGFR1-3, revealed its efficacy in curtailing osteosarcoma cell proliferation and invasion. The inhibitory effects are attributed to a dual-action mechanism that encompasses direct tumor cell suppression and modulation of the TME. The study delineates the capacity of sulfatinib to diminish M2 macrophage polarization and reduce populations of M2-TAMs, Tregs, and MDSCs, concurrently amplifying cytotoxic T-cell presence, hence attenuating the immunosuppressive context of the TME (Liao et al., 2023). Subsequent research by Proença et al. indicated that cyanidin suppresses osteosarcoma cells by escalating Bax expression and caspase-3 activity, triggering the intrinsic apoptotic pathway, and concurrently decreasing the secretion of inflammatory cytokines like IL-6, IL-1β, and IL-12p70, illustrating its therapeutic potential in osteosarcoma (Proenca et al., 2023). Wang et al. synthesized a nanomedicine that targets osteosarcoma-specific molecular markers precisely, utilizing the self-assembly of ROS and esterase-activated linker-connected cabazitaxel (diCTX) with diDHA, which induces significant cytotoxicity in the TME upon activation (Wang et al., 2023c). Similarly, Dieudonné et al. enhanced the sensitivity to the chemotherapeutic drug doxorubicin by targeting the Wnt/TCF signaling pathway, emphasizing the importance of molecular targeted therapy in osteosarcoma treatment (Dieudonne et al., 2012). Research by Martins-Neves et al. demonstrated that IWR-1, by inhibiting the Wnt/β-catenin signaling pathway, diminishes the self-renewal capability of osteosarcoma cancer stem cells, consequently reducing tumor growth and chemoresistance, highlighting the potential of targeting the Wnt/β-catenin pathway in osteosarcoma treatment strategies (Martins-Neves et al., 2018). Zheng et al. identified that soy isoflavones suppress the AKT/mTOR pathway, initiating mitochondrial autophagy, which in turn impedes osteosarcoma progression at a molecular level (Zheng Z. et al., 2024). Yu et al.’s approach in treating pulmonary metastatic osteosarcoma involves engineered hepatic genetic circuits for efficient VEGFR2 siRNA delivery, directly targeting tumor cells, diminishing adverse drug reactions, and potentially improving immune cell functionality (Yu et al., 2023). Furthermore, research by Cheng et al. revealed that Psoralidin inhibits osteosarcoma growth and metastasis by targeting the FAK and PI3K/Akt signaling pathways and downregulating ITGB1 expression (Cheng S. et al., 2023). Regarding molecular mechanisms, Wu et al. indicated that FGD1 promotes tumor progression by inhibiting PTEN activity, with overexpression associated with poor prognosis in osteosarcoma patients, activating the PI3K/AKT signaling pathway, and affecting tumor cell behavior, suggesting FGD1 as a potential target for improving osteosarcoma treatment outcomes (Wu et al., 2020). Higuchi et al. demonstrated that the combination of pioglitazone and cisplatin is an effective strategy for molecular targeted therapy in osteosarcoma, significantly inhibiting tumor growth by targeting PPARγ (Higuchi et al., 2020). Clinical trials have also shown promising results. The study by Kopp et al. conducted a clinical trial of glembatumumab vedotin (GV) in patients with recurrent osteosarcoma, revealing that GV exhibits antitumor activity in the treatment of recurrent osteosarcoma (Kopp et al., 2019). Research by Gaspar et al. similarly indicated that the combined use of lenvatinib, etoposide, and ifosfamide demonstrated promising antitumor activity in patients with recurrent or refractory osteosarcoma, warranting further exploration in randomized phase II studies (Gaspar et al., 2021). The application of targeted therapies in osteosarcoma treatment, by inhibiting tumor growth, modulating the microenvironment, and enhancing drug response, introduces novel directions for treatment strategies and contributes to a more thorough perception of therapeutic mechanisms.
For osteosarcoma treatment, ICIs are considered promising due to the significantly higher quantity of TILs in osteosarcoma compared to other sarcomas (Wedekind et al., 2018). The increased TILs in osteosarcoma suggest potential for more effective utilization of ICIs (van Erp et al., 2017). Principal inhibitors include PD-1, PD-L1, and CTLA4, each applicable in osteosarcoma treatment (Yu et al., 2022). Liu et al. demonstrated that activation of the STAT3 pathway by Thrombospondin-1 (TSP1) results in the upregulation of PD-L1, subsequently inhibiting the immune response in osteosarcoma. Subsequent analyses showed that neutralizing antibodies against PD-L1 can mitigate TSP1’s suppressive impact on CD8+ T cells (Liu Z. et al., 2022). The findings of this study offer a foundation for comprehending TSP1’s contribution to immune suppression in osteosarcomas and developing PD-L1 centric therapeutic strategies. Additionally, Sorafenib was observed to inhibit the Doxorubicin-induced upregulation of PD-L1, thereby ameliorating the immunosuppressive microenvironment in osteosarcoma. The synergistic application of Sorafenib and Doxorubicin significantly suppressed tumor growth and increased the proportion of CD8+ CTLs secreting IFN-γ in the tumor tissue (Wang et al., 2023b). Such modulation of the immune microenvironment by these combined pharmaceuticals has been shown to augment the treatment efficacy for osteosarcoma. Furthermore, Sundara et al. established that in metastatic osteosarcoma lesions, there is an elevation in PD-L1 expression alongside augmented T cell infiltration, indicating that T cell-based immunotherapy might be beneficial for patients with metastatic osteosarcoma (Sundara et al., 2017). Subsequent investigations have further highlighted the role of ICIs in osteosarcoma therapy. Xiang et al. demonstrated that combining IDO inhibitors with a platinum (IV) prodrug activates the cGAS-STING pathway, which results in enhanced CD8+ T cell activity and an improved tumor immune microenvironment (Xiang et al., 2023). Correspondingly, Ji et al. conducted research on the function of miR-140 within the osteosarcoma immune microenvironment, revealing that its reduction of PD-L1 not only impedes tumor proliferation but also intensifies CD8+ T cell infiltration, thereby ameliorating the immune microenvironment (Ji et al., 2018). Research by Mochizuki et al. indicated that OBP-502, a telomerase-specific oncolytic virus, not only improves the efficacy of anti-PD-1 antibodies in osteosarcoma treatment but also induces immunogenic cell death in tumor cells through integrin binding, leading to an increase in calreticulin, ATP, and HMGB1, and enhancing CD8+ T cell infiltration in the tumor (Mochizuki et al., 2021). Similarly, the investigation conducted by Sung et al. revealed that ICSBP enhances PD-L1 expression in osteosarcoma cells, leading to increased cell proliferation, and showed that decreasing PD-L1 not only inhibits tumor cell expansion but also strengthens the impact of chemotherapeutic drugs, proposing new treatment modalities for osteosarcoma (Sung et al., 2022). Ahangar et al. further noted that silencing the immune checkpoint HHLA2 enhances the sensitivity of osteosarcoma cells to Paclitaxel, increasing its cytotoxic effects (Ahangar et al., 2023). Additionally, research has indicated that elevated PD-L1 mRNA expression in osteosarcoma tissues, along with inhibiting the PD-1/PD-L1 axis, improves Cisplatin’s therapeutic efficacy, and the concurrent administration of anti-PD-1 antibodies with Cisplatin markedly diminishes tumor cell growth and enhances apoptosis (Liu X. et al., 2019). However, in the treatment of osteosarcoma, overcoming immune evasion, particularly the overexpression of PD-L1 and diminished T cell function, remains a significant challenge. Research by Liu et al. indicated that microRNA-200a enhances PD-L1 expression through the upregulation of PTEN, subsequently suppressing the immune response and reducing the efficacy of CD8+ T cells, thereby promoting tumor growth and impacting the effectiveness of PD-L1 targeted immunotherapy (Liu et al., 2020). Studies suggest that specific inhibitors or combination therapies can effectively address these challenges. For instance, the specific inhibition of PI3Kδ/γ by SNA inhibitors was found to improve the TME and, in conjunction with anti-PD1 therapy, decelerates tumor progression while prolonging survival time (Shi et al., 2019). Additionally, Ge et al. discovered that CBZP nanocarriers, activating ICD and inhibiting PD-1/PD-L1 interactions, significantly enhanced the efficacy of PD-1/PD-L1 immune checkpoint blockade therapy. This nanotherapeutic strategy, incorporating CUR and BMS1166, modulates autophagy and improves the TME, increasing CD8+ T cell infiltration in osteosarcoma, thereby achieving notable anti-tumor effects (Ge et al., 2022). Subsequent studies have revealed that a combination approach using different ICIs, such as inhibitors targeting CTLA-4, PD-L1, and TIM3, is more efficacious in reducing osteosarcoma cell viability and inducing apoptosis relative to individual blockades (Sorkhabi et al., 2022). Moreover, the integration of high-energy carbon ion radiotherapy with ICIs (anti-PD-1 and anti-CTLA-4) has been shown to exert substantial therapeutic effects on osteosarcoma lung metastases, reducing the metastases while concurrently augmenting CD8+ immune cell infiltration in tumors not directly exposed to radiation (Helm et al., 2021). In osteosarcoma immunotherapy research, various strategies have been proposed to enhance treatment effects and overcome immune evasion mechanisms. Duan et al. revealed that sunitinib, by targeting STAT3 to suppress PD-L1 expression, remodels the immune landscape and impedes tumor cell migration and invasion. When utilized alongside ICIs, sunitinib substantially reduces lung metastasis and tumor proliferation, leading to improved survival rates (Duan et al., 2020). Furthermore, Kawano et al. proposed using anti-CTLA-4 antibodies and cryo-treated tumor lysate-loaded DCs in a mouse osteosarcoma model to enhance the anti-tumor immune response. This combination therapy effectively suppresses osteosarcoma lung metastasis and improves survival rates through the reduction of Tregs and an increase in CD8+ T cells (Kawano et al., 2013). Research by He et al. further emphasized the importance of combination therapy, finding that the combined treatment of L-arginine with α-PD-L1 antibodies enhances the immune system’s response to osteosarcoma. L-arginine promotes the proliferation, differentiation, and survival of CD8+ T cells, elevating serum levels of IFN-γ and CD8+ T cell infiltration, while α-PD-L1 antibodies protect these enhanced CD8+ T cells from exhaustion (He et al., 2017). Simultaneously, Yu et al. revealed that the combination of the autophagy inhibitor 3-MA with photodynamic therapy (PDT) exhibits potential, primarily through the suppression of PD-L1 protein expression, which in turn activates an immune response that is effective in suppressing the proliferation and dissemination of osteosarcoma cells (Yu et al., 2019). Zheng et al. examined the PD-1 pathway in musculoskeletal tumors, finding that while PD-L1 expression negatively correlated with osteosarcoma patient survival, PD-L2 and PD-1 expressions demonstrated positive and negative associations with survival, respectively; moreover, in humanized mouse models, nivolumab’s inhibition of osteosarcoma metastasis highlighted the PD-1 pathway as a promising target for immunotherapeutic intervention in metastatic osteosarcoma (Zheng B. et al., 2018). Further, Lussier et al. found that PD-L1 expression on metastatic osteosarcoma cells, through its interaction with PD-1 on CTLs, impaired CTL function; conversely, disruption of the PD-1/PD-L1 interaction substantially improved CTL functionality, reduced tumor burden, prolonged patient survival (Lussier et al., 2015). Additionally, Ratti et al. discovered that Trabectedin inhibits osteosarcoma through the promotion of osteosarcoma cell differentiation and an increase in tumor-infiltrating T cells. Owing to the elevated PD-1 expression on intratumoral CD8 T cells, the integration of PD-1 inhibitors with standard therapy significantly enhances therapeutic effectiveness (Ratti et al., 2017). The study by Gao et al. revealed that the PD-1/PD-L1 axis inhibits Tfh cell function and reduces IL-21 secretion in osteosarcoma patients, thus obstructing potent anti-tumor responses in osteosarcoma patients; conversely, the application of ICIs assists in restoring Tfh cell functionality, thereby enhancing CD8+ T cell efficacy (Gao et al., 2017). The application of ICIs in osteosarcoma treatment, particularly targeting key checkpoints such as PD-1, PD-L1, and CTLA-4, has been proven to effectively modulate the TME and stimulate the activity of TILs, thus eliciting a potent anti-tumor immune response, offering a novel perspective in the treatment of osteosarcoma.
Tumor microenvironment modulators in osteosarcoma immunotherapy have shown immense potential. Jiang et al. conducted a comprehensive analysis of single-cell samples from osteosarcoma, revealing the role of genes associated with aggregated autophagy, suggesting that interventions targeting aggregated autophagy may improve survival rates and quality of life for patients with osteosarcoma (Jiang et al., 2023). Huang et al. identified the significant immunomodulatory effects of Methionine Enkephalin (MENK) in osteosarcoma treatment. It was shown that MENK enhances the activity of M1 macrophages and reduces the proportion of M2 macrophages, thereby exerting an inhibitory effect on osteosarcoma. Furthermore, MENK also influences tumor-associated immune cells, such as the distribution of MDSCs, providing new research directions and potential therapeutic strategies for osteosarcoma immunotherapy (Huang et al., 2023). In the studies by Zhang et al., let-7a was confirmed to have a tumor-suppressive role in Ewing’s sarcoma, particularly through negatively regulating macrophage infiltration. By affecting the interactions between STAT3 and NF-κB, let-7a inhibits the recruitment of macrophages, thus reducing the malignancy of Ewing’s sarcoma (Zhang et al., 2016). Further research by Zhang et al. showed that EPA reduces DDP-induced high expression of PD-L1 and decreases immune evasion in osteosarcoma, a process that helps enhance the sensitivity of osteosarcoma to chemotherapy and may improve treatment outcomes (Zhang et al., 2023). Herrador-Cañete et al. demonstrated significant therapeutic effects in a pediatric osteosarcoma model by employing SFV-mediated Gal3 inhibitors, which not only weakened the binding of Gal3 to activated T cells but also inhibited tumor growth and pulmonary metastasis, thereby accentuating the significance of diminishing the tumor’s immunosuppressive environment by augmenting lymphocyte infiltration (Herrador-Canete et al., 2022). The study conducted by Denton et al. on Ewing’s sarcoma treatment highlighted the pivotal role of M2 macrophages in the TME in influencing tumor growth and response to therapy. Administering liposomal clodronate (Clodrosome) alongside trabectedin was proven to effectively diminishes M2 macrophage infiltration, thus amplifying the effect of HSV oncolytic virus therapy in Ewing’s sarcoma and emphasizing the critical necessity to regulate macrophage polarization states within the TME for improved therapeutic outcomes (Denton et al., 2018). Similarly, Wang et al. demonstrated the efficacy of combining curcumin with cisplatin in inhibiting tumor-associated macrophage polarization towards M2, enhancing cisplatin-induced apoptosis, effectively inhibiting osteosarcoma cell proliferation and migration (Wang J. et al., 2022). Zhou et al. revealed the potential of all-trans retinoic acid (ATRA) in reducing osteosarcoma metastasis by inhibiting M2 polarization of TAMs, further emphasizing the importance of regulating TAM polarization in osteosarcoma treatment (All-Trans Retinoic Acid Prevents, 2020). Belisario et al. elucidated the importance of ABCA1 and ABCB1 expression ratios in determining osteosarcoma cell sensitivity to chemotherapy and immunotherapy. Enhanced ABCB1 expression reduces intracellular doxorubicin accumulation, while increased ABCA1 expression bolsters Vγ9Vδ2 T cell activity. These findings imply that osteosarcoma cells with high ABCB1 and low ABCA1 expression are more resistant to chemotherapy and immunotherapy, with bisphosphonates potentially acting as sensitizing agents (Belisario et al., 2020). The research of Miallot et al. suggested that the vitamin B5 precursor pantothenamide enhances anti-tumor immunity in sarcomas, including promoting dendritic and myeloid cells toward an IFNγ-driven antigen presentation pathway, and fostering a high metabolic state in CD8+ T cells (Miallot et al., 2023). Wang et al. revealed that the combined application of valproic acid and zoledronic acid enhances γδ T cell-mediated cytotoxicity against osteosarcoma cells. The mechanism primarily involves the accumulation of methylhydroxybutyrate pathway intermediates and relies on T cell receptor-mediated granule exocytosis pathways (Wang S. et al., 2018). Gong et al. noted that the MPIRx nanomedicine, incorporating the sonosensitizer IR780 and CD47 inhibitor RRx-001 into PEG-PCL nanomicelles, effectively promotes macrophage polarization toward the M1 phenotype and enhances phagocytic activity against tumor cells, significantly inhibiting osteosarcoma and its pulmonary metastasis (Gong et al., 2023). Makielski et al. found that the oncolytic virus VSV-IFNβ-NIS is safe and effective in canine osteosarcoma, where VSV-treated tumors exhibited stronger inflammatory responses and increased expression of T cell immune gene clusters, enhancing anti-tumor immune responses and improving long-term survival rates; this study further emphasizes the potential of oncolytic viruses in activating immune responses within the TME (Makielski et al., 2023). Park et al.'s research on improving the TME demonstrated that VEGF inhibitors, such as bevacizumab and DC101, optimized tumor vasculature, enhancing EATs’ infiltration and cytotoxic impact in solid tumors. The study showed an increase in high endothelial venules and a significant promotion of T cell, especially CD8(+) TILs, infiltration into tumors, achieving notable anti-tumor effects in diverse xenograft tumor models without an increase in toxicity (Park et al., 2023). Wu et al. discovered that hydroxyapatite nanoparticles (HANPs) with varying aspect ratios enhanced immunogenic cell death in tumor cells, activated macrophages, and promoted the maturation of CD8+ T cells and DCs, with particles of higher aspect ratios exhibiting stronger anti-tumor activity (Wu et al., 2023). In a mouse model of Rb1/p53-deficient osteosarcoma, Ferrena et al. observed that SKP2 gene knockout resulted in increased immune cell infiltration and transcriptional changes associated with favorable prognosis. A notable increase in the expression of macrophage-specific genes was detected, positively influencing overall survival rates in osteosarcoma patients (Ferrena et al., 2023). Moreover, advancements in non-invasive modalities and targeted therapy strategies are under thorough investigation. Hay et al. demonstrated that the application of ultrasound technology was efficient in ablating tumors and inducing immune responses in the TME, as indicated by the proliferation of macrophages and DCs, and upregulation of genes associated with immune activation (Hay et al., 2023). In an osteosarcoma mouse model, Yahiro et al. demonstrated that activation of TLR4 markedly increased CD8-positive cell infiltration in lung metastases and inhibited osteosarcoma progression. Activation of TLR4 by LPS enhanced accumulation of CD8-positive cells in tumors, and its inhibition negated LPS’s tumor-suppressive effects, underscoring the pivotal role of CD8-positive cells in anti-tumor responses (Yahiro et al., 2020). Regarding targeted signaling pathways, Fujiwara et al. ascertained that Pexidartinib (PLX3397), a CSF1/CSF1R signaling inhibitor, reduced CSF1 secretion in osteosarcoma cells, leading to diminished polarization and chemotaxis of M2-type macrophages. Concurrently, it facilitated the infiltration of CD8-positive T cells at both primary and metastatic osteosarcoma sites, contributing to the inhibition of tumor progression and enhancement of patient prognosis (Fujiwara et al., 2021). Similarly, Li et al. indicated that osteosarcomas decrease CXCL12 expression via DNA methyltransferase 1 (DNMT1), impacting tumor metastasis and T cell infiltration. A positive correlation was observed between CXCL12 levels, intratumoral lymphocyte presence, and survival rates in patients. Inhibiting DNMT1 in a mouse model resulted in heightened CXCL12 expression, enhancing immune responses and impeding tumor metastasis and growth (Li et al., 2018). Specific immunotherapeutic strategies, such as those demonstrated by Richert et al., have shown significant antitumor effects in the treatment of osteosarcoma with the use of liposomal-encapsulated chemically detoxified lipopolysaccharides (Lipo-MP-LPS) acting as TLR4 agonists. The mechanism involves the conversion of M2-type macrophages to M1-type, altering the immune microenvironment of osteosarcoma to a pro-inflammatory state and enhancing the recruitment of T cells and antitumor immune responses (Richert et al., 2023). Further investigations have revealed that particular therapeutic approaches, including radiofrequency ablation, nanomedicine delivery, and oncolytic virotherapy, can also enhance the activity of specific immune cells within the TME. Studies by Iwai et al., which combined radiofrequency ablation with OK-432 injections, induced systemic antitumor effects primarily characterized by increased numbers of specific immune cells, such as DCs and CD8+ T cells, and the promotion of pro-inflammatory cytokines (IFN-γ and TNF-α) within the tumor, revealing the potential of combined physical and chemical interventions in modulating the TME (Iwai et al., 2020). Additionally, Zhang et al. employed self-stabilizing hyaluronic acid nanoparticles for targeted delivery of anticancer drugs, such as docetaxel and cisplatin, along with the immunostimulatory agent resiquimod, promoting immunogenic cell death and immune activation within the TME, which significantly inhibited tumor growth in osteosarcoma mouse models (Zhang Y. et al., 2021). Research by Li et al. found that osteosarcoma cell-secreted LGALS3BP binds to LGALS3 on the surface of M1-type macrophages, inducing secretion of HSPA1L through the Akt phosphorylation pathway, promoting the transformation of M1-type macrophages to an antitumor phenotype, and inducing tumor cell apoptosis through the IRAK1 and IRAK4 pathways (Li et al., 2022). Moreover, studies by Jiang et al. have shown that high expression of c-Myc in osteosarcoma is inversely correlated with T cell infiltration rates. The c-Myc inhibitor JQ-1 significantly reduced tumor burden and increased survival rates in mouse models of osteosarcoma by promoting T cell migration to the tumor and activating specific CTLs through the CD40/CD40L costimulatory pathway, thereby improving the tumor immune microenvironment (Jiang et al., 2022). Research by Uehara et al. revealed that metformin could inhibit osteosarcoma growth by affecting the metabolic and functional characteristics of CD11b+ cells, particularly by reducing the number of MDSCs and altering the phenotype of TAMs, suggesting that modulating the metabolic state of specific immune cells in the TME can achieve tumor growth inhibition (Uehara et al., 2019). Furthermore, studies by Jain et al. observed that USP6 inhibits tumor growth in Ewing’s sarcoma by activating NK cells and inducing the expression of chemokines. USP6 increases the surface levels of NK cell activation ligands, enhancing the interaction between NK cells and Ewing’s sarcoma cells, thus augmenting the tumor-killing capability of NK cells and highlighting the significance of USP6 in regulating the TME (Jain et al., 2023). Research by Pahl et al. emphasized that LPS and IFN-γ-activated M1-type macrophages could inhibit osteosarcoma growth, with a similar effect observed when M1-type macrophages were activated with L-MTP-PE and IFN-γ. Additionally, IL-10-induced polarized M2-type macrophages could inhibit osteosarcoma growth in the presence of anti-EGFR cetuximab through an antibody-dependent mechanism, demonstrating the potential of macrophages in osteosarcoma treatment (Pahl et al., 2014). Interventions targeting the microenvironment of osteosarcoma have demonstrated potential for therapy, exerting direct impacts on neoplastic cells and bolstering immune responses, thereby providing possibilities for improved treatment outcome.
In the exploration of new strategies for osteosarcoma treatment, cytokine therapy has exhibited substantial potential. Specifically, IL-2 cytokine therapy in canine osteosarcoma is crucial for the immune response, as it stimulates T-cell activation and proliferation, thus substantially improving tumor cell identification and elimination (Flesner et al., 2020). The results of this study are in concordance with those presented by Hennessy et al., who demonstrated that BEMPEG (NKTR-214), as an IL-2 pathway agonist, delayed tumor progression and enhanced survival rates in murine models of osteosarcoma (Hennessy et al., 2021). Furthermore, Nastasi et al. established that the inhibition of IL-10 through combined administration of anti-IL-10 antibodies and Mifamurtide substantially increased tumor cell mortality and decreased metastasis in osteosarcoma treatment, emphasizing the significance of IL-10 regulation in immune cells and its potential to augment the effectiveness of Mifamurtide therapy (Nastasi et al., 2023). Concurrently, Rebhun et al. investigated the application of inhaled recombinant human interleukin-15 (rhIL-15) in the treatment of lung metastases in canine osteosarcoma and melanoma. Their findings suggested that diminished lymphocyte levels before treatment and heightened peak cytotoxicity were significantly associated with therapeutic effectiveness, demonstrating rhIL-15’s considerable clinical potential in inhibiting tumor metastasis (Rebhun et al., 2022). Morales-Molina et al. utilized a combined immunotherapeutic approach involving mesenchymal stem cells (MSCs) bearing tumor-specific oncolytic adenoviruses (OAds) and granulocyte colony-stimulating factor (G-CSF) in osteosarcoma models, substantially reducing tumor growth; it was observed that G-CSF improved infiltration and activity of CD8+ T cells and DCs in the tumor immune microenvironment, effectively enhancing tumor immunosurveillance and elimination while diminishing T cell exhaustion (Morales-Molina et al., 2021). In summary, cytokine therapy in osteosarcoma treatment has shown potential by enhancing immune cell function and reducing immune escape, offering new perspectives for therapy.
The specificity of therapies targeting tumor antigens has proven valuable in cancer treatment, particularly in osteosarcoma research, demonstrating the potential of enhancing treatment efficacy through targeting tumor antigens. Park et al. demonstrated that the use of dual-specificity antibodies targeting GD2 and HER2 (T-BsAb) significantly enhanced osteosarcoma treatment outcomes, with these antibodies substantially inhibiting tumor growth in both in vitro and in vivo models; the addition of anti-PD-L1 treatment further augmented their antitumor activity (Park and Cheung, 2020). Moreover, Wiebel et al. revealed an association between cell density and GD2 expression in osteosarcoma cells, finding that increased cell density enhanced the reactivity to GD2-specific CAR T cells, providing a basis for optimizing CAR T therapy (Wiebel et al., 2021). In the search for innovative immunological targets, Li et al. discerned two novel CTL epitopes derived from the osteosarcoma antigen papillomavirus binding factor (PBF) with immunostimulatory potential in the HLA-A11 context (Li et al., 2019). Watanabe et al. developed tumor-associated antigen-specific T cell receptor (TCR) multimers, particularly PBF TCR multimers, demonstrating high efficiency in recognizing naturally presented peptides and effectively identifying tumor-associated antigens on osteosarcoma cells, thus introducing novel strategies for immunotherapy (Watanabe et al., 2019). Research conducted by Tsukahara et al. further confirmed PBF as a significant tumor-associated antigen in osteosarcoma, providing novel peptide-based immunotherapeutic targets, with its extensive presence in osteosarcoma cells and tissues highlighting its potential for immunotherapeutic applications. Cytotoxic T lymphocyte clones’ recognition of specific PBF peptides emphasized their importance in therapy (Tsukahara et al., 2004). Additionally, the identification of novel epitopes has propelled advancements in oncological therapies. Osei-Hwedieh et al. demonstrated that T cell recognition of these epitopes is imperative for tumor immunosurveillance, with sarcomas, particularly osteosarcomas, exhibiting a range of antigenic profiles conducive to T cell-mediated immunotherapy enhancement (Osei-Hwedieh et al., 2023). Tsuda et al. identified the presence of SART3 antigen in osteosarcoma and showed that peptides derived from SART3 could activate specific cytotoxic T cells to target HLA-A24 positive osteosarcoma cells, presenting novel molecular targets for immunotherapy against osteosarcoma (Tsuda et al., 2001). In developing therapies targeting specific markers, Englisch et al. discovered that VEGFR2 is prevalently expressed in tumor-associated vascular endothelial cells of Ewing’s sarcoma and engineered CARs targeting VEGFR2 with variable hinge lengths, which, when expressed in human T cells, specifically lysed VEGFR2-expressing target cells through mechanisms including enhanced antigen-specific degranulation, effective tumor spheroid lysis, TNF-α secretion, sustained killing, and cellular proliferation, indicating VEGFR2 as a potent target for CAR T cell therapy in Ewing’s sarcoma (Englisch et al., 2020). Specificity in tumor antigen-targeted therapies has made significant strides in osteosarcoma research, effectively targeting tumor antigens with specific antibodies, CAR T cells, and T cell receptor multimers, revealing new immunological targets and therapeutic strategies, providing novel insights for treatment.
Significant progress has been made in the field of osteosarcoma treatment, particularly in vaccine development. Domingo-Musibay et al.'s findings indicated engineered measles virus (MV) as an effective agent against osteosarcoma cells, demonstrating substantial oncolytic impacts, including tumor growth reduction and increased survival durations in osteosarcoma xenograft mouse models (Domingo-Musibay et al., 2014). Additionally, Mason et al. investigated the recombinant Listeria ADXS31-164, expressing HER2/neu fusion protein, and their results validated its effectiveness in activating tumor-specific immune responses in canine osteosarcoma models, notably enhancing IFNγ responses, effectively reducing metastasis rates, and improving survival outcomes (Mason et al., 2016). Advancements in autologous cancer cell vaccine research were notable, as illustrated by Flesner et al., who employed these vaccines effectively in treating canine osteosarcoma, demonstrating their significant therapeutic impact by activating the canine immune system against the tumor, thereby underscoring the importance of personalized treatment and the potential of utilizing the autologous immune system in tumor management (Flesner et al., 2020). Furthermore, Peng et al. demonstrated that DCs, when pulsed with EWS/FLI-l peptides, effectively induced immune responses in Ewing’s sarcoma mouse models, not only triggering IFN-γ secretion from effector cells but also specifically targeting and eradicating specific tumor cells (Peng et al., 2014). Adenovirus and DNA vaccines have also shown therapeutic potential. Schober et al. revealed that the integration of YB-1 driven adenovirus XVir-N-31 with CDK4/6 inhibitors elevated the immunogenicity of Ewing’s sarcoma, enhancing both viral replication and oncolytic capacity, and, through the activation of HLA-I and IFNγ-inducible protein 10, it enhanced DCs maturation and specific T cell activation against tumor antigens (Schober et al., 2023). Tarone et al. demonstrated that the HuDo-CSPG4 DNA vaccine’s role in activating CD8+ T cells and anti-CSPG4 immune responses, leading to the induction of CD8+ T cells and serum that hinder osteosarcoma growth and metastasis, concurrently confirming its safety and survival-prolonging effects in canine osteosarcoma models (Tarone et al., 2023). Regarding dendritic cell vaccines, Zhou et al. demonstrated that CD103+ cDC1s vaccines, particularly when augmented with anti-CTLA-4 antibodies, significantly enhanced control over melanoma and osteosarcoma, completely eradicated osteosarcoma tumors, and effectively prevented lung metastasis (Zhou et al., 2020b). Miwa et al. established the safety of DC vaccine therapy in eliciting immune responses in patients with refractory sarcomas, acknowledging that, although clinical responses varied, DC vaccines demonstrated potential in enhancing anti-tumor immune responses (Miwa et al., 2017). Immunomodulators have shown promise in the treatment of osteosarcoma. Research by Cascini et al. revealed that local injections of TLR9 agonists in osteosarcoma mouse models significantly inhibited tumor growth and elicited notable effects in untreated contralateral tumors. These applications led to substantial changes in the immune microenvironment, including a reduction in M2-type macrophages, increased infiltration of DCs, and the activation of CD8 T cells, suggesting the potential of TLR9 agonists as in situ anti-tumor vaccines (Cascini et al., 2023). Wang et al. developed calcium phosphate nanoparticles for in situ vaccination against osteosarcoma, engineered to control the release of chemotherapeutic agents and immunomodulators, thus activating DCs and CD8+ T cells, and effectively inhibiting tumor progression and metastasis (Wang Y. et al., 2023). Additionally, Pritchard-Jones et al. discovered that the 105AD7 vaccine effectively elicited T cell responses and specific IFN-γ secretion in osteosarcoma patients post-intensive chemotherapy, underscoring its potential in immunoregulatory therapy (Pritchard-Jones et al., 2005). In osteosarcoma treatment, Chauvin et al. developed a novel vaccine based on rat-derived killer dendritic cells (KDCs), which, by activating KDCs to target and eliminate tumor cells and effectively present tumor antigens in vivo, induced a strong CD8 T cell-mediated immune response (Chauvin et al., 2008). Moreover, Jin et al. demonstrated that capsaicin induced calreticulin (CRT) exposure on human osteosarcoma cell surfaces, initiating immunogenic cell death (ICD) and augmenting the phagocytic capabilities of antigen-presenting cells along with lymphocyte IFN-γ secretion, contributing to the experimental foundation for tumor vaccine strategies in osteosarcoma (Jin et al., 2016). Cumulatively, these studies illustrate the potency of vaccines in precisely targeting and eliminating osteosarcoma through the stimulation of the immune system, advancing understanding of the tumor’s immune interactions and suggesting innovative directions for forthcoming treatments.
Cell-based therapies represent a significant advancement in osteosarcoma treatment, particularly in the domain of chimeric antigen receptor T-cell (CAR-T cell) therapy. Charan et al. revealed that combining HGF receptor-neutralizing antibodies (AMG102) with GD2-targeted CAR-T cell therapy inhibited tumor growth and metastasis in Ewing’s sarcoma, offering an effective treatment option for tumors with hyperactive HGF/c-MET pathways (Charan et al., 2020). Furthermore, Evans et al.’s exploration of EWS-FLI-1 in Ewing’s sarcoma family of tumors (ESFT) revealed that modified EWS-FLI-1 peptides notably activated CTLs against Ewing’s sarcoma, demonstrating significant cytotoxicity in vitro against ESFT and effectively suppressing tumor growth in mouse models (Evans et al., 2012). Another study, conducted by Hidalgo et al., presented evidence that FITC-labeled anti-B7-H3 antibodies used for controlled activation of CAR T cells targeting cancer cells enhanced specificity of the therapy, decreased side effects on normal tissues, and effectively treated osteosarcoma in animal models (Hidalgo et al., 2023). Research by Fallarini et al. found that invariant natural killer T cells (iNKT cells) in osteosarcoma therapy exerted cytotoxicity on osteosarcoma cells via a CD1d-dependent mechanism and augmented cell death caused by cisplatin, doxorubicin, and methotrexate (Fallarini et al., 2012). Additionally, according to Buddingh et al., IL-15 activated NK cells present a novel therapeutic approach against high-grade osteosarcoma, including cells resistant to chemotherapy (Buddingh et al., 2011). Kailayangiri et al. confirmed that Ewing’s sarcoma cells uniformly express G(D2) antigens, offering a theoretical rationale for applying CAR T cell immunotherapy, with GD2-targeted CAR T cells exhibiting high specificity and cytotoxicity against tumor cells, thus suggesting a novel approach for Ewing’s sarcoma treatment (Kailayangiri et al., 2012). The investigation by Mensali et al. revealed that ALPL-1 targeted CAR-T cells had significant impact in osteosarcoma treatment, selectively recognizing and destroying ALPL-positive osteosarcoma cells and exhibiting notable anti-tumor activity in in situ models, all while maintaining normal tissue safety (Mensali et al., 2023). Long et al., in their exploration of GD2-CAR T cells for osteosarcoma, revealed significant cytotoxic potential of these genetically engineered T cells against GD2ww-positive tumor cells, with the study highlighting the TME, notably the presence of MDSCs, as a potential barrier to efficacy, and recommending all-trans retinoic acid to reduce MDSCs and improve therapeutic outcomes (Long et al., 2016). In addition, a study highlighted the efficacy of CAR T cell therapy against CD166 in osteosarcoma, with CD166.BBζ CAR-T cells demonstrating significant cytotoxicity in vitro against osteosarcoma cells, and mouse models further confirming their effectiveness in tumor growth inhibition with minimal toxicity (Wang et al., 2019). Treatments based on γδ T cells and NK cells also showed therapeutic potential. Wang et al.’s findings indicated that human Vγ9Vδ2 T cells, as antigen-presenting cells, hold potential in osteosarcoma treatment; their activation increased CCL5 secretion through the upregulation of HSP90 production, MyD88, and JNK activation, effectively enhancing CD8+ T cell responses against osteosarcoma cells (Wang S. et al., 2023). A further study indicated that decitabine, a demethylating agent, amplified the expression of NKG2D ligands including MICB and ULBP1 on osteosarcoma cell surfaces, thus rendering them more susceptible to γδ T cell attack. Blocking the NKG2D receptor partly reversed the γδ T cell-induced cytotoxicity, suggesting a significant reliance on the NKG2D-NKG2DL axis, and highlighted the possibility of enhanced efficacy with combined decitabine and γδ T cell therapy (Wang Z. et al., 2018). Kisseberth et al. conducted research using autologous NK cell transplantation aimed at overcoming cancer-induced reductions in NK cell quantity and functionality, offering innovative treatment options for canine osteosarcoma (Kisseberth and Lee, 2021). Significant attention has been focused on the utilization of iPSC-derived CTLs and memory T cells in cell therapy applications. Ishii et al. demonstrated that iPSC-derived rejTs, focusing on neoantigens from the EWS/FLI1 fusion gene, induced notable immune effects against Ewing’s sarcoma. In co-culture with Ewing’s sarcoma cell lines, rejTs consistently exhibited suppressive effects on tumor cells, and the anti-tumor capacity of EWS/FLI1-rejT, along with enhanced survival rates, was further verified in mouse xenograft models of Ewing’s sarcoma (Ishii et al., 2021). Fernández et al. indicated that CD45RA-memory T cells, expressing the NKG2D-4-1BB-CD3z CAR, targeted osteosarcoma cells expressing NKG2D ligands effectively, with these cells showing greater cytotoxicity against osteosarcoma cells, which points to the essential role of immune cells, notably memory T cells, in focused osteosarcoma therapy (Fernandez et al., 2017). Additionally, some studies have focused on enhancing therapeutic efficacy by modifying PBMCs and incorporating immunomodulators. Yang et al. introduced a membrane-anchored and tumor-targeted IL12-PBMC therapy with profound effects on osteosarcoma. This strategy, through PBMC modification, eliminated the traditional CAR T therapy’s expansion phase and enhanced tumor-specific cytokine release, leading to effective inhibition of tumor growth (Yang et al., 2022). Hu et al. demonstrated that attIL12-T cells, equipped with membrane-anchored tumor-targeted IL-12, specifically engaged osteosarcoma cells through interaction with carapatin (CSV) on the tumor surface. This interaction led to increased IFNγ production, reduced TGF-β activity, apoptosis induction in CAFs, and disruption of the ECM, thereby facilitating T cell infiltration, enhancing immune cell function, and inhibiting tumor growth (Hu et al., 2024). Cell therapy, especially CAR-T technology, has introduced innovative approaches in osteosarcoma treatment, while advancements in iNKT, NK cells, and iPSC-derived cells have extended treatment methodologies, enhancing both specificity and effectiveness.
In osteosarcoma treatment research, the application of antibodies has shown significant potential for immunomodulation. Initially, Kohyama et al. revealed that employing the monoclonal antibody S4B6, which targets IL-2, resulted in significant inhibition of tumor growth in osteosarcoma models in mice. S4B6 operates by depleting Tregs and activating autoimmune responses, with its administration at multiple time points around LM8 cell transplantation consistently diminishing tumor size and lung metastases (Kohyama et al., 2012). Subsequently, Kawano et al. established that the combined deployment of agonistic anti-GITR antibodies with DCs enhanced immune responsiveness in osteosarcoma therapy, reducing the presence of Tregs and effectively suppressing tumor proliferation. This dual therapy approach not only increased CD8+ T cell levels and IFN-γ but also reduced immunosuppressive factors within the tumor, introducing an innovative method for osteosarcoma immunotherapy (Kawano et al., 2015). Additionally, the combined administration of anti-TGF-β antibodies and DCs in a mouse model for osteosarcoma significantly enhanced systemic immune responses, thereby restricting metastatic tumor growth by boosting CD8+ T cell levels and reducing the prevalence of Tregs (Kawano et al., 2012). The combined therapy of CD47 mAb and doxorubicin, as shown by Mohanty et al., effectively increased macrophage and other immune cell infiltration in osteosarcoma, resulting in substantial tumor growth suppression (Mohanty et al., 2019). These research findings demonstrate that antibody application in osteosarcoma treatment not only directly suppresses tumor growth but also adjusts crucial cell types and immune responses, offering innovative strategies and approaches for osteosarcoma therapy.
Investigating immunotherapeutic strategies in osteosarcoma reveals crucial interventions modifying tumor-immune interactions. Table 2 presents targeted immune cells, corresponding treatments, their mechanisms, and immune consequences, emphasizing the principal aspects of therapy and their impacts on immune regulation.
This review comprehensively outlines significant advancements in the field of osteosarcoma treatment, with particular emphasis on the potential of novel therapies such as ICIs, tumor microenvironment modulators, cytokine therapy, and targeted treatments against specific antigens. Developments in CAR-T cell therapy alongside the employment of iPSC-derived CTLs and memory T cells represent a paradigm shift in cancer treatment strategies towards personalized and targeted approaches. The integration of antibodies further enhances these therapeutic strategies, improving specificity and effectiveness.
Despite these advancements, several limitations remain. A primary impediment is the intricacy of the TME and its role in immune evasion. The heterogeneity of osteosarcoma cells and disparate treatment outcomes among distinct patient groups also pose substantial hurdles. Moreover, numerous novel therapies are still in developmental or clinical trial phases, with their long-term efficacy and safety yet to be fully established. More rigorous clinical trials are required to validate the effectiveness of these therapies across diverse patient demographics.
In the future, sustained investigation is imperative to surmount these obstacles and to enrich comprehension of the complex interplays between osteosarcoma cells and the immune mechanism. Subsequent studies should focus on developing more effective combination therapies that can synergistically enhance treatment outcomes. Personalized medicine approaches, utilizing genomic and proteomic profiling, could offer more tailored and effective treatment strategies. The exploration of novel immunotherapeutic targets and the optimization of current treatment modalities are crucial for improving patient outcomes. Finally, fostering multidisciplinary collaborations will be essential in accelerating the translation of these scientific insights into clinical practice, providing new hope for patients with osteosarcoma.
HL: Formal Analysis, Methodology, Writing–original draft, Writing–review and editing. MC: Data curation, Validation, Writing–review and editing. JT: Investigation, Resources, Supervision, Writing–review and editing. XC: Conceptualization, Funding acquisition, Supervision, Writing–review and editing.
The author(s) declare that financial support was received for the research, authorship, and/or publication of this article. This research received financial support from the Natural Science Foundation of Hubei Province of China, No. 2022CFB686; the Science Foundation of Union Hospital, No. 2021xhyn102.
The authors declare that the research was conducted in the absence of any commercial or financial relationships that could be construed as a potential conflict of interest.
All claims expressed in this article are solely those of the authors and do not necessarily represent those of their affiliated organizations, or those of the publisher, the editors and the reviewers. Any product that may be evaluated in this article, or claim that may be made by its manufacturer, is not guaranteed or endorsed by the publisher.
TME, Tumor Microenvironment; TAMs, Tumor-Associated Macrophages; MDSCs, Myeloid-Derived Suppressor Cells; NK cells, Natural Killer Cells; DCs, Dendritic Cells; IDO, Indoleamine 2,3-Dioxygenase; PD-1, Programmed Cell Death Protein 1; CTLs, Cytotoxic T Lymphocytes; Th cells, Helper T Lymphocytes; Tregs, Regulatory T Cells; TILs, Tumor-Infiltrating Lymphocytes; ICIs, Immune Checkpoint Inhibitors; CAR-T, Chimeric Antigen Receptor T-Cell; PBMCs, Peripheral Blood Mononuclear Cells; G-CSF, Granulocyte Colony-Stimulating Factor; TCR, T Cell Receptor; iNKT cells, Invariant Natural Killer T Cells.
Ahangar, N. K., Khalaj-Kondori, M., Alizadeh, N., Mokhtarzadeh, A., Baghbanzadeh, A., Shadbad, M. A., et al. (2023). Silencing tumor-intrinsic HHLA2 potentiates the anti-tumoral effect of paclitaxel on MG63 cells: another side of immune checkpoint. Gene 855, 147086. [published Online First: 20221216]. doi:10.1016/j.gene.2022.147086
Alfranca, A., Martinez-Cruzado, L., Tornin, J., Abarrategi, A., Amaral, T., de Alava, E., et al. (2015). Bone microenvironment signals in osteosarcoma development. Cell Mol. Life Sci. 72 (16), 3097–3113. [published Online First: 20150503]. doi:10.1007/s00018-015-1918-y
All-Trans Retinoic Acid Prevents (2020). Correction: all-trans retinoic acid Prevents osteosarcoma metastasis by inhibiting M2 polarization of tumor-associated macrophages. Cancer Immunol. Res. 8 (2), 280. doi:10.1158/2326-6066.CIR-19-0971
Belisario, D. C., Akman, M., Godel, M., Campani, V., Patrizio, M. P., Scotti, L., et al. (2020). ABCA1/ABCB1 ratio determines chemo- and immune-sensitivity in human osteosarcoma. Cells 9 (3), 647. [published Online First: 20200306]. doi:10.3390/cells9030647
Biller, B. J., Guth, A., Burton, J. H., and Dow, S. W. (2010). Decreased ratio of CD8+ T cells to regulatory T cells associated with decreased survival in dogs with osteosarcoma. J. Vet. Intern Med. 24 (5), 1118–1123. [published Online First: 20100728]. doi:10.1111/j.1939-1676.2010.0557.x
Buddingh, E. P., Schilham, M. W., Ruslan, S. E., Berghuis, D., Szuhai, K., Suurmond, J., et al. (2011). Chemotherapy-resistant osteosarcoma is highly susceptible to IL-15-activated allogeneic and autologous NK cells. Cancer Immunol. Immunother. 60 (4), 575–586. [published Online First: 20110115]. doi:10.1007/s00262-010-0965-3
Cascini, C., Ratti, C., Botti, L., Parma, B., Cancila, V., Salvaggio, A., et al. (2023). Rewiring innate and adaptive immunity with TLR9 agonist to treat osteosarcoma. J. Exp. Clin. Cancer Res. 42 (1), 154. [published Online First: 20230626]. doi:10.1186/s13046-023-02731-z
Cascio, M. J., Whitley, E. M., Sahay, B., Cortes-Hinojosa, G., Chang, L. J., Cowart, J., et al. (2021). Canine osteosarcoma checkpoint expression correlates with metastasis and T-cell infiltrate. Vet. Immunol. Immunopathol. 232, 110169. [published Online First: 20201215]. doi:10.1016/j.vetimm.2020.110169
Charan, M., Dravid, P., Cam, M., Audino, A., Gross, A. C., Arnold, M. A., et al. (2020). GD2-directed CAR-T cells in combination with HGF-targeted neutralizing antibody (AMG102) prevent primary tumor growth and metastasis in Ewing sarcoma. Int. J. Cancer 146 (11), 3184–3195. [published Online First: 20191106]. doi:10.1002/ijc.32743
Chauvin, C., Philippeau, J. M., Hemont, C., Hubert, F. X., Wittrant, Y., Lamoureux, F., et al. (2008). Killer dendritic cells link innate and adaptive immunity against established osteosarcoma in rats. Cancer Res. 68 (22), 9433–9440. doi:10.1158/0008-5472.CAN-08-0104
Chen, P., Zhou, J., Li, J., Zhang, Q., and Zuo, Q. (2019). TIPE1 suppresses osteosarcoma tumor growth by regulating macrophage infiltration. Clin. Transl. Oncol. 21 (3), 334–341. [published Online First: 20180730]. doi:10.1007/s12094-018-1927-z
Cheng, D., Zhang, Z., Mi, Z., Tao, W., Liu, D., Fu, J., et al. (2023a). Deciphering the heterogeneity and immunosuppressive function of regulatory T cells in osteosarcoma using single-cell RNA transcriptome. Comput. Biol. Med. 165, 107417. [published Online First: 20230901]. doi:10.1016/j.compbiomed.2023.107417
Cheng, S., Liu, S., Chen, B., Du, C., Xiao, P., Luo, X., et al. (2023b). Psoralidin inhibits osteosarcoma growth and metastasis by downregulating ITGB1 expression via the FAK and PI3K/Akt signaling pathways. Chin. Med. 18 (1), 34. [published Online First: 20230331]. doi:10.1186/s13020-023-00740-w
Cheng, Z., Wang, L., Wu, C., Huang, L., Ruan, Y., and Xue, W. (2021). Tumor-derived exosomes induced M2 macrophage polarization and promoted the metastasis of osteosarcoma cells through tim-3. Arch. Med. Res. 52 (2), 200–210. [published Online First: 20201105]. doi:10.1016/j.arcmed.2020.10.018
Chim, L. K., Williams, I. L., Bashor, C. J., and Mikos, A. G. (2023). Tumor-associated macrophages induce inflammation and drug resistance in a mechanically tunable engineered model of osteosarcoma. Biomaterials 296, 122076. [published Online First: 20230307]. doi:10.1016/j.biomaterials.2023.122076
Cillo, A. R., Mukherjee, E., Bailey, N. G., Onkar, S., Daley, J., Salgado, C., et al. (2022). Ewing sarcoma and osteosarcoma have distinct immune signatures and intercellular communication networks. Clin. Cancer Res. 28 (22), 4968–4982. doi:10.1158/1078-0432.CCR-22-1471
Das, S., Idate, R., Regan, D. P., Fowles, J. S., Lana, S. E., Thamm, D. H., et al. (2021). Immune pathways and TP53 missense mutations are associated with longer survival in canine osteosarcoma. Commun. Biol. 4 (1), 1178. [published Online First: 20211011]. doi:10.1038/s42003-021-02683-0
Deng, C., Xu, Y., Fu, J., Zhu, X., Chen, H., Xu, H., et al. (2020). Reprograming the tumor immunologic microenvironment using neoadjuvant chemotherapy in osteosarcoma. Cancer Sci. 111 (6), 1899–1909. [published Online First: 20200423]. doi:10.1111/cas.14398
Denton, N. L., Chen, C. Y., Hutzen, B., Currier, M. A., Scott, T., Nartker, B., et al. (2018). Myelolytic treatments enhance oncolytic herpes virotherapy in models of ewing sarcoma by modulating the immune microenvironment. Mol. Ther. Oncolytics 11, 62–74. [published Online First: 20181018]. doi:10.1016/j.omto.2018.10.001
Dieudonne, F. X., Marion, A., Marie, P. J., and Modrowski, D. (2012). Targeted inhibition of T-cell factor activity promotes syndecan-2 expression and sensitization to doxorubicin in osteosarcoma cells and bone tumors in mice. J. Bone Min. Res. 27 (10), 2118–2129. doi:10.1002/jbmr.1650
Domingo-Musibay, E., Allen, C., Kurokawa, C., Hardcastle, J. J., Aderca, I., Msaouel, P., et al. (2014). Measles Edmonston vaccine strain derivatives have potent oncolytic activity against osteosarcoma. Cancer Gene Ther. 21 (11), 483–490. [published Online First: 20141114]. doi:10.1038/cgt.2014.54
Duan, X. L., Guo, J. P., Li, F., Xiu, C., and Wang, H. (2020). Sunitinib inhibits PD-L1 expression in osteosarcoma by targeting STAT3 and remodels the immune system in tumor-bearing mice. Future Oncol. 16 (24), 1815–1824. [published Online First: 20200608]. doi:10.2217/fon-2019-0725
Dumars, C., Ngyuen, J. M., Gaultier, A., Lanel, R., Corradini, N., Gouin, F., et al. (2016). Dysregulation of macrophage polarization is associated with the metastatic process in osteosarcoma. Oncotarget 7 (48), 78343–78354. doi:10.18632/oncotarget.13055
Durfee, R. A., Mohammed, M., and Luu, H. H. (2016). Review of osteosarcoma and current management. Rheumatol. Ther. 3 (2), 221–243. [published Online First: 20161019]. doi:10.1007/s40744-016-0046-y
Englisch, A., Altvater, B., Kailayangiri, S., Hartmann, W., and Rossig, C. (2020). VEGFR2 as a target for CAR T cell therapy of Ewing sarcoma. Pediatr. Blood Cancer 67 (10), e28313. [published Online First: 20200730]. doi:10.1002/pbc.28313
Evans, C. H., Liu, F., Porter, R. M., O'Sullivan, R. P., Merghoub, T., Lunsford, E. P., et al. (2012). EWS-FLI-1-targeted cytotoxic T-cell killing of multiple tumor types belonging to the Ewing sarcoma family of tumors. Clin. Cancer Res. 18 (19), 5341–5351. [published Online First: 20120809]. doi:10.1158/1078-0432.CCR-12-1985
Fallarini, S., Paoletti, T., Orsi, B. N., and Lombardi, G. (2012). Invariant NKT cells increase drug-induced osteosarcoma cell death. Br. J. Pharmacol. 167 (7), 1533–1549. doi:10.1111/j.1476-5381.2012.02108.x
Fan, Q., Zuo, J., Tian, H., Huang, C., Nice, E. C., Shi, Z., et al. (2022). Nanoengineering a metal-organic framework for osteosarcoma chemo-immunotherapy by modulating indoleamine-2,3-dioxygenase and myeloid-derived suppressor cells. J. Exp. Clin. Cancer Res. 41 (1), 162. [published Online First: 20220503]. doi:10.1186/s13046-022-02372-8
Fernandez, L., Metais, J. Y., Escudero, A., Vela, M., Valentín, J., Vallcorba, I., et al. (2017). Memory T cells expressing an nkg2d-CAR efficiently target osteosarcoma cells. Clin. Cancer Res. 23 (19), 5824–5835. [published Online First: 20170628]. doi:10.1158/1078-0432.CCR-17-0075
Ferrena, A., Wang, J., Zhang, R., Karadal-Ferrena, B., Al-Hardan, W., Singh, S., et al. (2023). SKP2 knockout in Rb1/p53 deficient mouse models of osteosarcoma induces immune infiltration and drives a transcriptional program with a favorable prognosis. Mol. Cancer Ther. 23, 223–234. [published Online First: 20231024]. doi:10.1158/1535-7163.MCT-23-0173
Flesner, B. K., Wood, G. W., Gayheart-Walsten, P., Sonderegger, F. L., Henry, C. J., Tate, D. J., et al. (2020). Autologous cancer cell vaccination, adoptive T-cell transfer, and interleukin-2 administration results in long-term survival for companion dogs with osteosarcoma. J. Vet. Intern Med. 34 (5), 2056–2067. [published Online First: 20200710]. doi:10.1111/jvim.15852
Franklin, R. A., and Li, M. O. (2016). Ontogeny of tumor-associated macrophages and its implication in cancer regulation. Trends Cancer 2 (1), 20–34. doi:10.1016/j.trecan.2015.11.004
Fujiwara, T., Yakoub, M. A., Chandler, A., Christ, A. B., Yang, G., Ouerfelli, O., et al. (2021). CSF1/CSF1R signaling inhibitor Pexidartinib (PLX3397) reprograms tumor-associated macrophages and stimulates T-cell infiltration in the sarcoma microenvironment. Mol. Cancer Ther. 20 (8), 1388–1399. [published Online First: 20210604]. doi:10.1158/1535-7163.MCT-20-0591
Gao, W., Zhou, J., and Ji, B. (2017). Evidence of interleukin 21 reduction in osteosarcoma patients due to PD-1/PD-L1-mediated suppression of follicular helper T cell functionality. DNA Cell Biol. 36 (9), 794–800. [published Online First: 20170626]. doi:10.1089/dna.2017.3669
Gao, X., Han, D., and Fan, W. (2016). Down-regulation of RBP-J mediated by microRNA-133a suppresses dendritic cells and functions as a potential tumor suppressor in osteosarcoma. Exp. Cell Res. 349 (2), 264–272. [published Online First: 20161026]. doi:10.1016/j.yexcr.2016.10.019
Gaspar, N., Occean, B. V., Pacquement, H., Bompas, E., Bouvier, C., Brisse, H. J., et al. (2018). Results of methotrexate-etoposide-ifosfamide based regimen (M-EI) in osteosarcoma patients included in the French OS2006/sarcome-09 study. Eur. J. Cancer 88, 57–66. [published Online First: 20171128]. doi:10.1016/j.ejca.2017.09.036
Gaspar, N., Venkatramani, R., Hecker-Nolting, S., Melcon, S. G., Locatelli, F., Bautista, F., et al. (2021). Lenvatinib with etoposide plus ifosfamide in patients with refractory or relapsed osteosarcoma (ITCC-050): a multicentre, open-label, multicohort, phase 1/2 study. Lancet Oncol. 22 (9), 1312–1321. [published Online First: 20210817]. doi:10.1016/S1470-2045(21)00387-9
Ge, Y. X., Zhang, T. W., Zhou, L., Ding, W., Liang, H. F., Hu, Z. C., et al. (2022). Enhancement of anti-PD-1/PD-L1 immunotherapy for osteosarcoma using an intelligent autophagy-controlling metal organic framework. Biomaterials 282, 121407. [published Online First: 20220217]. doi:10.1016/j.biomaterials.2022.121407
Gomez-Brouchet, A., Illac, C., Gilhodes, J., Bouvier, C., Aubert, S., Guinebretiere, J. M., et al. (2017). CD163-positive tumor-associated macrophages and CD8-positive cytotoxic lymphocytes are powerful diagnostic markers for the therapeutic stratification of osteosarcoma patients: an immunohistochemical analysis of the biopsies fromthe French OS2006 phase 3 trial. Oncoimmunology 6 (9), e1331193. [published Online First: 20170824]. doi:10.1080/2162402X.2017.1331193
Gong, M., Huang, Y., Feng, H., Lin, J., Huang, A., Hu, J., et al. (2023). A nanodrug combining CD47 and sonodynamic therapy efficiently inhibits osteosarcoma deterioration. J. Control Release 355, 68–84. [published Online First: 20230202]. doi:10.1016/j.jconrel.2023.01.038
Guan, Y., Zhang, R., Peng, Z., Dong, D., Wei, G., and Wang, Y. (2017). Inhibition of IL-18-mediated myeloid derived suppressor cell accumulation enhances anti-PD1 efficacy against osteosarcoma cancer. J. Bone Oncol. 9, 59–64. [published Online First: 20171101]. doi:10.1016/j.jbo.2017.10.002
Han, Q., Shi, H., and Liu, F. (2016). CD163(+) M2-type tumor-associated macrophage support the suppression of tumor-infiltrating T cells in osteosarcoma. Int. Immunopharmacol. 34, 101–106. [published Online First: 20160301]. doi:10.1016/j.intimp.2016.01.023
Han, Y., Guo, W., Ren, T., Huang, Y., Wang, S., Liu, K., et al. (2019). Tumor-associated macrophages promote lung metastasis and induce epithelial-mesenchymal transition in osteosarcoma by activating the COX-2/STAT3 axis. Cancer Lett. 440-441, 116–125. [published Online First: 20181019]. doi:10.1016/j.canlet.2018.10.011
Harrison, D. J., Geller, D. S., Gill, J. D., Lewis, V. O., and Gorlick, R. (2018). Current and future therapeutic approaches for osteosarcoma. Expert Rev. Anticancer Ther. 18 (1), 39–50. [published Online First: 20171214]. doi:10.1080/14737140.2018.1413939
Hay, A. N., Imran, K. M., Hendricks-Wenger, A., Gannon, J. M., Sereno, J., Simon, A., et al. (2023). Ablative and immunostimulatory effects of histotripsy ablation in a murine osteosarcoma model. Biomedicines 11 (10), 2737. [published Online First: 20231009]. doi:10.3390/biomedicines11102737
He, X., Lin, H., Yuan, L., and Li, B. (2017). Combination therapy with L-arginine and α-PD-L1 antibody boosts immune response against osteosarcoma in immunocompetent mice. Cancer Biol. Ther. 18 (2), 94–100. [published Online First: 20170103]. doi:10.1080/15384047.2016.1276136
Helm, A., Tinganelli, W., Simoniello, P., Kurosawa, F., Fournier, C., Shimokawa, T., et al. (2021). Reduction of lung metastases in a mouse osteosarcoma model treated with carbon ions and immune checkpoint inhibitors. Int. J. Radiat. Oncol. Biol. Phys. 109 (2), 594–602. [published Online First: 20200924]. doi:10.1016/j.ijrobp.2020.09.041
Hennessy, M., Wahba, A., Felix, K., Cabrera, M., Segura, M. G., Kundra, V., et al. (2021). Bempegaldesleukin (BEMPEG; NKTR-214) efficacy as a single agent and in combination with checkpoint-inhibitor therapy in mouse models of osteosarcoma. Int. J. Cancer 148 (8), 1928–1937. [published Online First: 20201125]. doi:10.1002/ijc.33382
Henrich, I. C., Jain, K., Young, R., Quick, L., Lindsay, J. M., Park, D. H., et al. (2021). Ubiquitin-specific protease 6 functions as a tumor suppressor in ewing sarcoma through immune activation. Cancer Res. 81 (8), 2171–2183. [published Online First: 20210208]. doi:10.1158/0008-5472.CAN-20-1458
Herrador-Canete, G., Zalacain, M., Labiano, S., Laspidea, V., Puigdelloses, M., Marrodan, L., et al. (2022). Galectin-3 inhibition boosts the therapeutic efficacy of Semliki Forest virus in pediatric osteosarcoma. Mol. Ther. Oncolytics 26, 246–264. [published Online First: 20220709]. doi:10.1016/j.omto.2022.07.004
Heymann, M. F., Lezot, F., and Heymann, D. (2019). The contribution of immune infiltrates and the local microenvironment in the pathogenesis of osteosarcoma. Cell Immunol. 343, 103711. [published Online First: 20171102]. doi:10.1016/j.cellimm.2017.10.011
Hidalgo, L., Somovilla-Crespo, B., Garcia-Rodriguez, P., Morales-Molina, A., Rodriguez-Milla, M. A., and Garcia-Castro, J. (2023). Switchable CAR T cell strategy against osteosarcoma. Cancer Immunol. Immunother. 72 (8), 2623–2633. [published Online First: 20230416]. doi:10.1007/s00262-023-03437-z
Higuchi, T., Yamamoto, J., Sugisawa, N., Tashiro, Y., Nishino, H., Yamamoto, N., et al. (2020). PPARγ agonist pioglitazone in combination with cisplatinum arrests a chemotherapy-resistant osteosarcoma PDOX model. Cancer Genomics Proteomics 17 (1), 35–40. doi:10.21873/cgp.20165
Hu, J., Lazar, A. J., Ingram, D., Wang, W. L., Zhang, W., Jia, Z., et al. (2024). Cell membrane-anchored and tumor-targeted IL-12 T-cell therapy destroys cancer-associated fibroblasts and disrupts extracellular matrix in heterogenous osteosarcoma xenograft models. J. Immunother. Cancer 12 (1), e006991. [published Online First: 20240109]. doi:10.1136/jitc-2023-006991
Huang, H., Wang, X., Zhang, S., Bai, X., Griffin, N., Shan, Y., et al. (2023). In vitro and in vivo killing effects of methionine enkephalin on osteosarcoma. Int. Immunopharmacol. 125 (Pt B), 111226. [published Online First: 20231115]. doi:10.1016/j.intimp.2023.111226
Huang, Q., Liang, X., Ren, T., Huang, Y., Zhang, H., Yu, Y., et al. (2021). The role of tumor-associated macrophages in osteosarcoma progression - therapeutic implications. Cell Oncol. (Dordr) 44 (3), 525–539. [published Online First: 20210331]. doi:10.1007/s13402-021-00598-w
Ishii, M., Ando, J., Yamazaki, S., Toyota, T., Ohara, K., Furukawa, Y., et al. (2021). iPSC-derived neoantigen-specific CTL therapy for ewing sarcoma. Cancer Immunol. Res. 9 (10), 1175–1186. [published Online First: 20210812]. doi:10.1158/2326-6066.CIR-21-0193
Itahashi, K., Irie, T., and Nishikawa, H. (2022). Regulatory T-cell development in the tumor microenvironment. Eur. J. Immunol. 52 (8), 1216–1227. [published Online First: 20220725]. doi:10.1002/eji.202149358
Iwai, T., Oebisu, N., Hoshi, M., Orita, K., Yamamoto, A., Hamamoto, S., et al. (2020). Promising abscopal effect of combination therapy with thermal tumour ablation and intratumoural OK-432 injection in the rat osteosarcoma model. Sci. Rep. 10 (1), 9679. [published Online First: 20200615]. doi:10.1038/s41598-020-66934-6
Jain, K., Henrich, I. C., Quick, L., Young, R., Mondal, S., Oliveira, A. M., et al. (2023). Natural killer cell activation by ubiquitin-specific protease 6 mediates tumor suppression in ewing sarcoma. Cancer Res. Commun. 3 (8), 1615–1627. [published Online First: 20230822]. doi:10.1158/2767-9764.CRC-22-0505
Ji, T., Shi, Q., Mei, S., Xu, J., Liang, H., Xie, L., et al. (2023). Integrated analysis of single-cell and bulk RNA sequencing data reveals an immunostimulatory microenvironment in tumor thrombus of osteosarcoma. Oncogenesis 12 (1), 31. [published Online First: 20230527]. doi:10.1038/s41389-023-00474-2
Ji, X., Wang, E., and Tian, F. (2018). MicroRNA-140 suppresses osteosarcoma tumor growth by enhancing anti-tumor immune response and blocking mTOR signaling. Biochem. Biophys. Res. Commun. 495 (1), 1342–1348. [published Online First: 20171121]. doi:10.1016/j.bbrc.2017.11.120
Jiang, K., Li, J., Zhang, J., Wang, L., Zhang, Q., Ge, J., et al. (2019). SDF-1/CXCR4 axis facilitates myeloid-derived suppressor cells accumulation in osteosarcoma microenvironment and blunts the response to anti-PD-1 therapy. Int. Immunopharmacol. 75, 105818. [published Online First: 20190819]. doi:10.1016/j.intimp.2019.105818
Jiang, K., Zhang, Q., Fan, Y., Li, J., Zhang, J., Wang, W., et al. (2022). MYC inhibition reprograms tumor immune microenvironment by recruiting T lymphocytes and activating the CD40/CD40L system in osteosarcoma. Cell Death Discov. 8 (1), 117. [published Online First: 20220315]. doi:10.1038/s41420-022-00923-8
Jiang, Y., Ning, Y., Cheng, S., Huang, Y., Deng, M., and Chen, C. (2023). Single-cell aggrephagy-related patterns facilitate tumor microenvironment intercellular communication, influencing osteosarcoma progression and prognosis. Apoptosis 29, 521–535. [published Online First: 20231208]. doi:10.1007/s10495-023-01922-5
Jin, T., Wu, H., Wang, Y., and Peng, H. (2016). Capsaicin induces immunogenic cell death in human osteosarcoma cells. Exp. Ther. Med. 12 (2), 765–770. [published Online First: 20160519]. doi:10.3892/etm.2016.3368
Joshi, S., and Sharabi, A. (2022). Targeting myeloid-derived suppressor cells to enhance natural killer cell-based immunotherapy. Pharmacol. Ther. 235, 108114. [published Online First: 20220202]. doi:10.1016/j.pharmthera.2022.108114
Kailayangiri, S., Altvater, B., Meltzer, J., Pscherer, S., Luecke, A., Dierkes, C., et al. (2012). The ganglioside antigen G(D2) is surface-expressed in Ewing sarcoma and allows for MHC-independent immune targeting. Br. J. Cancer 106 (6), 1123–1133. [published Online First: 20120228]. doi:10.1038/bjc.2012.57
Kansara, M., Leong, H. S., Lin, D. M., Popkiss, S., Pang, P., Garsed, D. W., et al. (2013). Immune response to RB1-regulated senescence limits radiation-induced osteosarcoma formation. J. Clin. Invest. 123 (12), 5351–5360. [published Online First: 20131115]. doi:10.1172/JCI70559
Kansara, M., Thomson, K., Pang, P., Dutour, A., Mirabello, L., Acher, F., et al. (2019). Infiltrating myeloid cells drive osteosarcoma progression via GRM4 regulation of IL23. Cancer Discov. 9 (11), 1511–1519. [published Online First: 20190916]. doi:10.1158/2159-8290.CD-19-0154
Kawano, M., Itonaga, I., Iwasaki, T., Tsuchiya, H., and Tsumura, H. (2012). Anti-TGF-β antibody combined with dendritic cells produce antitumor effects in osteosarcoma. Clin. Orthop. Relat. Res. 470 (8), 2288–2294. [published Online First: 20120314]. doi:10.1007/s11999-012-2299-2
Kawano, M., Itonaga, I., Iwasaki, T., and Tsumura, H. (2013). Enhancement of antitumor immunity by combining anti-cytotoxic T lymphocyte antigen-4 antibodies and cryotreated tumor lysate-pulsed dendritic cells in murine osteosarcoma. Oncol. Rep. 29 (3), 1001–1006. [published Online First: 20130104]. doi:10.3892/or.2013.2224
Kawano, M., Tanaka, K., Itonaga, I., Iwasaki, T., Miyazaki, M., Ikeda, S., et al. (2015). Dendritic cells combined with anti-GITR antibody produce antitumor effects in osteosarcoma. Oncol. Rep. 34 (4), 1995–2001. [published Online First: 20150729]. doi:10.3892/or.2015.4161
Kielbassa, K., Vegna, S., Ramirez, C., and Akkari, L. (2019). Understanding the origin and diversity of macrophages to tailor their targeting in solid cancers. Front. Immunol. 10, 2215. [published Online First: 20190925]. doi:10.3389/fimmu.2019.02215
Kisseberth, W. C., and Lee, D. A. (2021). Adoptive natural killer cell immunotherapy for canine osteosarcoma. Front. Vet. Sci. 8, 672361. [published Online First: 20210607]. doi:10.3389/fvets.2021.672361
Kohyama, K., Sugiura, H., Kozawa, E., Wasa, J., Yamada, K., Nishioka, A., et al. (2012). Antitumor activity of an interleukin-2 monoclonal antibody in a murine osteosarcoma transplantation model. Anticancer Res. 32 (3), 779–782.
Kopp, L. M., Malempati, S., Krailo, M., Gao, Y., Buxton, A., Weigel, B. J., et al. (2019). Phase II trial of the glycoprotein non-metastatic B-targeted antibody-drug conjugate, glembatumumab vedotin (CDX-011), in recurrent osteosarcoma AOST1521: a report from the Children's Oncology Group. Eur. J. Cancer 121, 177–183. [published Online First: 20191003]. doi:10.1016/j.ejca.2019.08.015
Kuo, C. L., Chou, H. Y., Lien, H. W., Yeh, C. A., Wang, J. R., Chen, C. H., et al. (2023). A Fc-VEGF chimeric fusion enhances PD-L1 immunotherapy via inducing immune reprogramming and infiltration in the immunosuppressive tumor microenvironment. Cancer Immunol. Immunother. 72 (2), 351–369. [published Online First: 20220727]. doi:10.1007/s00262-022-03255-9
Le, T., Su, S., Kirshtein, A., and Shahriyari, L. (2021). Data-driven mathematical model of osteosarcoma. Cancers (Basel) 13 (10), 2367. [published Online First: 20210514]. doi:10.3390/cancers13102367
Li, B., Wang, Z., Wu, H., Xue, M., Lin, P., Wang, S., et al. (2018). Epigenetic regulation of CXCL12 plays a critical role in mediating tumor progression and the immune response in osteosarcoma. Cancer Res. 78 (14), 3938–3953. [published Online First: 20180507]. doi:10.1158/0008-5472.CAN-17-3801
Li, C., Xiang, F., Gong, Y., Fu, Y., Chen, G., Wang, Z., et al. (2024). Tumor-derived microparticles promoted M2-like macrophages polarization to stimulate osteosarcoma progression. Int. J. Biochem. Cell Biol. 166, 106494. [published Online First: 20231111]. doi:10.1016/j.biocel.2023.106494
Li, D., Toji, S., Watanabe, K., Torigoe, T., and Tsukahara, T. (2019). Identification of novel human leukocyte antigen-A*11:01-restricted cytotoxic T-lymphocyte epitopes derived from osteosarcoma antigen papillomavirus binding factor. Cancer Sci. 110 (4), 1156–1168. [published Online First: 20190312]. doi:10.1111/cas.13973
Li, J., Zhao, C., Li, Y., Wen, J., Wang, S., Wang, D., et al. (2022). Osteosarcoma exocytosis of soluble LGALS3BP mediates macrophages toward a tumoricidal phenotype. Cancer Lett. 528, 1–15. [published Online First: 20211221]. doi:10.1016/j.canlet.2021.12.023
Li, J., Zhao, C., Wang, D., Wang, S., Dong, H., Yang, Y., et al. (2023). ZIM3 activation of CCL25 expression in pulmonary metastatic nodules of osteosarcoma recruits M2 macrophages to promote metastatic growth. Cancer Immunol. Immunother. 72 (4), 903–916. [published Online First: 20220926]. doi:10.1007/s00262-022-03300-7
Li, X., Chen, Y., Liu, X., Zhang, J., He, X., Teng, G., et al. (2017). Tim3/Gal9 interactions between T cells and monocytes result in an immunosuppressive feedback loop that inhibits Th1 responses in osteosarcoma patients. Int. Immunopharmacol. 44, 153–159. [published Online First: 20170116]. doi:10.1016/j.intimp.2017.01.006
Liang, X., Guo, W., Ren, T., Huang, Y., Sun, K., Zhang, H., et al. (2020). Macrophages reduce the sensitivity of osteosarcoma to neoadjuvant chemotherapy drugs by secreting Interleukin-1 beta. Cancer Lett. 480, 4–14. [published Online First: 20200324]. doi:10.1016/j.canlet.2020.03.019
Liao, S., Li, J., Gao, S., Han, Y., Han, X., Wu, Y., et al. (2023). Sulfatinib, a novel multi-targeted tyrosine kinase inhibitor of FGFR1, CSF1R, and VEGFR1-3, suppresses osteosarcoma proliferation and invasion via dual role in tumor cells and tumor microenvironment. Front. Oncol. 13, 1158857. [published Online First: 20230608]. doi:10.3389/fonc.2023.1158857
Ligon, J. A., Choi, W., Cojocaru, G., Fu, W., Hsiue, E. H. C., Oke, T. F., et al. (2021). Pathways of immune exclusion in metastatic osteosarcoma are associated with inferior patient outcomes. J. Immunother. Cancer 9 (5), e001772. doi:10.1136/jitc-2020-001772
Lin, J., Xu, A., Jin, J., Zhang, M., Lou, J., Qian, C., et al. (2022). MerTK-mediated efferocytosis promotes immune tolerance and tumor progression in osteosarcoma through enhancing M2 polarization and PD-L1 expression. Oncoimmunology 11 (1), 2024941. [published Online First: 20220112]. doi:10.1080/2162402X.2021.2024941
Liu, M. X., Liu, Q. Y., Liu, Y., Cheng, Z. M., Liu, L., Zhang, L., et al. (2019a). Interleukin-35 suppresses antitumor activity of circulating CD8(+) T cells in osteosarcoma patients. Connect. Tissue Res. 60 (4), 367–375. [published Online First: 20190107]. doi:10.1080/03008207.2018.1552267
Liu, Q., Xu, R., Xu, X., Huang, Y., and Ma, Z. (2022a). Characteristics and significance of T lymphocyte subsets in peripheral blood of osteosarcoma mice. Transl. Cancer Res. 11 (6), 1503–1509. doi:10.21037/tcr-22-264
Liu, W., Hu, H., Shao, Z., Lv, X., Zhang, Z., Deng, X., et al. (2023). Characterizing the tumor microenvironment at the single-cell level reveals a novel immune evasion mechanism in osteosarcoma. Bone Res. 11 (1), 4. [published Online First: 20230103]. doi:10.1038/s41413-022-00237-6
Liu, W., Long, Q., Zhang, W., Zeng, D., Hu, B., Liu, S., et al. (2021). miRNA-221-3p derived from M2-polarized tumor-associated macrophage exosomes aggravates the growth and metastasis of osteosarcoma through SOCS3/JAK2/STAT3 axis. Aging (Albany NY) 13 (15), 19760–19775. [published Online First: 20210813]. doi:10.18632/aging.203388
Liu, X., He, S., Wu, H., Xie, H., Zhang, T., and Deng, Z. (2019b). Blocking the PD-1/PD-L1 axis enhanced cisplatin chemotherapy in osteosarcoma in vitro and in vivo. Environ. Health Prev. Med. 24 (1), 79. [published Online First: 20191221]. doi:10.1186/s12199-019-0835-3
Liu, Z., Wen, J., Hu, F., Wang, J., Hu, C., and Zhang, W. (2022b). Thrombospondin-1 induced programmed death-ligand 1-mediated immunosuppression by activating the STAT3 pathway in osteosarcoma. Cancer Sci. 113 (2), 432–445. [published Online First: 20211223]. doi:10.1111/cas.15237
Liu, Z., Wen, J., Wu, C., Hu, C., Wang, J., Bao, Q., et al. (2020). MicroRNA-200a induces immunosuppression by promoting PTEN-mediated PD-L1 upregulation in osteosarcoma. Aging (Albany NY) 12 (2), 1213–1236. [published Online First: 20200124]. doi:10.18632/aging.102679
Long, A. H., Highfill, S. L., Cui, Y., Smith, J. P., Walker, A. J., Ramakrishna, S., et al. (2016). Reduction of MDSCs with all-trans retinoic acid improves CAR therapy efficacy for sarcomas. Cancer Immunol. Res. 4 (10), 869–880. [published Online First: 20160822]. doi:10.1158/2326-6066.CIR-15-0230
Lussier, D. M., O'Neill, L., Nieves, L. M., McAfee, M. S., Holechek, S. A., Collins, A. W., et al. (2015). Enhanced T-cell immunity to osteosarcoma through antibody blockade of PD-1/PD-L1 interactions. J. Immunother. 38 (3), 96–106. doi:10.1097/CJI.0000000000000065
Makielski, K. M., Sarver, A. L., Henson, M. S., Stuebner, K. M., Borgatti, A., Suksanpaisan, L., et al. (2023). Neoadjuvant systemic oncolytic vesicular stomatitis virus is safe and may enhance long-term survivorship in dogs with naturally occurring osteosarcoma. Mol. Ther. Oncolytics 31, 100736. [published Online First: 20231014]. doi:10.1016/j.omto.2023.100736
Mantovani, A., Marchesi, F., Malesci, A., Laghi, L., and Allavena, P. (2017). Tumour-associated macrophages as treatment targets in oncology. Nat. Rev. Clin. Oncol. 14 (7), 399–416. [published Online First: 20170124]. doi:10.1038/nrclinonc.2016.217
Martins-Neves, S. R., Paiva-Oliveira, D. I., Fontes-Ribeiro, C., Bovée, J. V. M. G., Cleton-Jansen, A. M., and Gomes, C. M. F. (2018). IWR-1, a tankyrase inhibitor, attenuates Wnt/β-catenin signaling in cancer stem-like cells and inhibits in vivo the growth of a subcutaneous human osteosarcoma xenograft. Cancer Lett. 414, 1–15. [published Online First: 20171108]. doi:10.1016/j.canlet.2017.11.004
Mason, N. J., Gnanandarajah, J. S., Engiles, J. B., Gray, F., Laughlin, D., Gaurnier-Hausser, A., et al. (2016). Immunotherapy with a HER2-targeting Listeria induces HER2-specific immunity and demonstrates potential therapeutic effects in a phase I trial in canine osteosarcoma. Clin. Cancer Res. 22 (17), 4380–4390. [published Online First: 20160318]. doi:10.1158/1078-0432.CCR-16-0088
Mathieu, M., Martin-Jaular, L., Lavieu, G., and Théry, C. (2019). Specificities of secretion and uptake of exosomes and other extracellular vesicles for cell-to-cell communication. Nat. Cell Biol. 21 (1), 9–17. [published Online First: 20190102]. doi:10.1038/s41556-018-0250-9
Mensali, N., Koksal, H., Joaquina, S., Wernhoff, P., Casey, N. P., Romecin, P., et al. (2023). ALPL-1 is a target for chimeric antigen receptor therapy in osteosarcoma. Nat. Commun. 14 (1), 3375. [published Online First: 20230608]. doi:10.1038/s41467-023-39097-x
Miallot, R., Millet, V., Roger, A., Fenouil, R., Tardivel, C., Martin, J. C., et al. (2023). The coenzyme A precursor pantethine enhances antitumor immunity in sarcoma. Life Sci. Alliance 6 (12), e202302200. [published Online First: 20231013]. doi:10.26508/lsa.202302200
Mirabello, L., Troisi, R. J., and Savage, S. A. (2009). Osteosarcoma incidence and survival rates from 1973 to 2004: data from the surveillance, epidemiology, and end results program. Cancer 115 (7), 1531–1543. doi:10.1002/cncr.24121
Miwa, S., Nishida, H., Tanzawa, Y., Takeuchi, A., Hayashi, K., Yamamoto, N., et al. (2017). Phase 1/2 study of immunotherapy with dendritic cells pulsed with autologous tumor lysate in patients with refractory bone and soft tissue sarcoma. Cancer 123 (9), 1576–1584. [published Online First: 20170227]. doi:10.1002/cncr.30606
Mochizuki, Y., Tazawa, H., Demiya, K., Kure, M., Kondo, H., Komatsubara, T., et al. (2021). Telomerase-specific oncolytic immunotherapy for promoting efficacy of PD-1 blockade in osteosarcoma. Cancer Immunol. Immunother. 70 (5), 1405–1417. [published Online First: 20201105]. doi:10.1007/s00262-020-02774-7
Mohanty, S., Aghighi, M., Yerneni, K., Theruvath, J. L., and Daldrup-Link, H. E. (2019). Improving the efficacy of osteosarcoma therapy: combining drugs that turn cancer cell 'don't eat me' signals off and 'eat me' signals on. Mol. Oncol. 13 (10), 2049–2061. [published Online First: 20190813]. doi:10.1002/1878-0261.12556
Morales-Molina, A., Gambera, S., Leo, A., and García-Castro, J. (2021). Combination immunotherapy using G-CSF and oncolytic virotherapy reduces tumor growth in osteosarcoma. J. Immunother. Cancer 9 (3), e001703. doi:10.1136/jitc-2020-001703
Myers, J. A., and Miller, J. S. (2021). Exploring the NK cell platform for cancer immunotherapy. Nat. Rev. Clin. Oncol. 18 (2), 85–100. [published Online First: 20200915]. doi:10.1038/s41571-020-0426-7
Nastasi, N., Pasha, A., Bruno, G., Subbiani, A., Pietrovito, L., Leo, A., et al. (2023). Blockade of IL-10 signaling ensures Mifamurtide efficacy in metastatic osteosarcoma. Cancers (Basel) 15 (19), 4744. [published Online First: 20230927]. doi:10.3390/cancers15194744
Nirala, B. K., Patel, T. D., Kurenbekova, L., Shuck, R., Dasgupta, A., Rainusso, N., et al. (2023). MYC regulates CSF1 expression via microRNA 17/20a to modulate tumor-associated macrophages in osteosarcoma. JCI Insight 8 (13), e164947. [published Online First: 20230710]. doi:10.1172/jci.insight.164947
Noman, M. Z., Desantis, G., Janji, B., Hasmim, M., Karray, S., Dessen, P., et al. (2014). PD-L1 is a novel direct target of HIF-1α, and its blockade under hypoxia enhanced MDSC-mediated T cell activation. J. Exp. Med. 211 (5), 781–790. [published Online First: 20140428]. doi:10.1084/jem.20131916
Noy, R., and Pollard, J. W. (2014). Tumor-associated macrophages: from mechanisms to therapy. Immunity 41 (1), 49–61. doi:10.1016/j.immuni.2014.06.010
Ogiwara, Y., Nakagawa, M., Nakatani, F., Uemura, Y., Zhang, R., and Kudo-Saito, C. (2022). Blocking FSTL1 boosts NK immunity in treatment of osteosarcoma. Cancer Lett. 537, 215690. [published Online First: 20220416]. doi:10.1016/j.canlet.2022.215690
Osei-Hwedieh, D. O., Sedlacek, A. L., Hernandez, L. M., Yamoah, A. A., Iyer, S. G., Weiss, K. R., et al. (2023). Immunosurveillance shapes the emergence of neo-epitope landscapes of sarcomas, revealing prime targets for immunotherapy. JCI Insight 8 (13), e170324. [published Online First: 20230710]. doi:10.1172/jci.insight.170324
Pahl, J. H., Kwappenberg, K. M., Varypataki, E. M., Santos, S. J., Kuijjer, M. L., Mohamed, S., et al. (2014). Macrophages inhibit human osteosarcoma cell growth after activation with the bacterial cell wall derivative liposomal muramyl tripeptide in combination with interferon-γ. J. Exp. Clin. Cancer Res. 33 (1), 27. [published Online First: 20140310]. doi:10.1186/1756-9966-33-27
Park, J. A., and Cheung, N. V. (2020). GD2 or HER2 targeting T cell engaging bispecific antibodies to treat osteosarcoma. J. Hematol. Oncol. 13 (1), 172. [published Online First: 20201210]. doi:10.1186/s13045-020-01012-y
Park, J. A., Espinosa-Cotton, M., Guo, H. F., Monette, S., and Cheung, N. K. V. (2023). Targeting tumor vasculature to improve antitumor activity of T cells armed ex vivo with T cell engaging bispecific antibody. J. Immunother. Cancer 11 (3), e006680. doi:10.1136/jitc-2023-006680
Peng, W., Huang, X., and Yang, D. (2014). EWS/FLI-l peptide-pulsed dendritic cells induces the antitumor immunity in a murine Ewing's sarcoma cell model. Int. Immunopharmacol. 21 (2), 336–341. [published Online First: 20140524]. doi:10.1016/j.intimp.2014.05.013
Pierrevelcin, M., Flacher, V., Mueller, C. G., Vauchelles, R., Guerin, E., Lhermitte, B., et al. (2022). Engineering novel 3D models to recreate high-grade osteosarcoma and its immune and extracellular matrix microenvironment. Adv. Healthc. Mater 11 (19), e2200195. [published Online First: 20220904]. doi:10.1002/adhm.202200195
Pritchard-Jones, K., Spendlove, I., Wilton, C., Whelan, J., Weeden, S., Lewis, I., et al. (2005). Immune responses to the 105AD7 human anti-idiotypic vaccine after intensive chemotherapy, for osteosarcoma. Br. J. Cancer 92 (8), 1358–1365. doi:10.1038/sj.bjc.6602500
Proenca, C., Rufino, A. T., Santos, I., Albuquerque, H. M. T., Silva, A. M. S., Fernandes, E., et al. (2023). Gossypetin is a novel modulator of inflammatory cytokine production and a suppressor of osteosarcoma cell growth. Antioxidants (Basel) 12 (9), 1744. [published Online First: 20230910]. doi:10.3390/antiox12091744
Qi, J., Liu, S., and Zhang, Z. (2024). What role does GPR65 play in the progression of osteosarcoma? Its mechanism and clinical significance. Cancer Cell Int. 24 (1), 31. [published Online First: 20240113]. doi:10.1186/s12935-024-03216-5
Ratti, C., Botti, L., Cancila, V., Galvan, S., Torselli, I., Garofalo, C., et al. (2017). Trabectedin overrides osteosarcoma differentiative block and reprograms the tumor immune environment enabling effective combination with immune checkpoint inhibitors. Clin. Cancer Res. 23 (17), 5149–5161. [published Online First: 20170609]. doi:10.1158/1078-0432.CCR-16-3186
Razmara, A. M., Judge, S. J., Gingrich, A. A., Cruz, S. M., Culp, W. T. N., Kent, M. S., et al. (2021). Natural killer and T cell infiltration in canine osteosarcoma: clinical implications and translational relevance. Front. Vet. Sci. 8, 771737. [published Online First: 20211116]. doi:10.3389/fvets.2021.771737
Rebhun, R. B., York, D., Cruz, S. M., Judge, S. J., Razmara, A. M., Farley, L. E., et al. (2022). Inhaled recombinant human IL-15 in dogs with naturally occurring pulmonary metastases from osteosarcoma or melanoma: a phase 1 study of clinical activity and correlates of response. J. Immunother. Cancer 10 (6), e004493. doi:10.1136/jitc-2022-004493
Richert, I., Berchard, P., Abbes, L., Novikov, A., Chettab, K., Vandermoeten, A., et al. (2023). A TLR4 agonist induces osteosarcoma regression by inducing an antitumor immune response and reprogramming M2 macrophages to M1 macrophages. Cancers (Basel) 15 (18), 4635. [published Online First: 20230919]. doi:10.3390/cancers15184635
Schell, T. D., Knowles, B. B., and Tevethia, S. S. (2000). Sequential loss of cytotoxic T lymphocyte responses to simian virus 40 large T antigen epitopes in T antigen transgenic mice developing osteosarcomas. Cancer Res. 60 (11), 3002–3012.
Schober, S. J., Schoening, C., Eck, J., Middendorf, C., Lutsch, J., Knoch, P., et al. (2023). The oncolytic adenovirus XVir-N-31 joins forces with CDK4/6 inhibition augmenting innate and adaptive antitumor immunity in ewing sarcoma. Clin. Cancer Res. 29 (10), 1996–2011. doi:10.1158/1078-0432.CCR-22-1961
Segaliny, A. I., Mohamadi, A., Dizier, B., Lokajczyk, A., Brion, R., Lanel, R., et al. (2015). Interleukin-34 promotes tumor progression and metastatic process in osteosarcoma through induction of angiogenesis and macrophage recruitment. Int. J. Cancer 137 (1), 73–85. [published Online First: 20141213]. doi:10.1002/ijc.29376
Shi, X., Li, X., Wang, H., Yu, Z., Zhu, Y., and Gao, Y. (2019). Specific inhibition of PI3Kδ/γ enhances the efficacy of anti-PD1 against osteosarcoma cancer. J. Bone Oncol. 16, 100206. [published Online First: 20181107]. doi:10.1016/j.jbo.2018.11.001
Somaiah, N., Conley, A. P., Parra, E. R., Lin, H., Amini, B., Solis Soto, L., et al. (2022). Durvalumab plus tremelimumab in advanced or metastatic soft tissue and bone sarcomas: a single-centre phase 2 trial. Lancet Oncol. 23 (9), 1156–1166. [published Online First: 20220804]. doi:10.1016/S1470-2045(22)00392-8
Sorkhabi, A. D., Sarkesh, A., Fotouhi, A., Saeedi, H., and Aghebati-Maleki, L. (2022). Cancer combination therapies by silencing of CTLA-4, PD-L1, and TIM3 in osteosarcoma. IUBMB Life 74 (9), 908–917. [published Online First: 20220617]. doi:10.1002/iub.2655
Suehara, Y., Alex, D., Bowman, A., Middha, S., Zehir, A., Chakravarty, D., et al. (2019). Clinical genomic sequencing of pediatric and adult osteosarcoma reveals distinct molecular subsets with potentially targetable alterations. Clin. Cancer Res. 25 (21), 6346–6356. [published Online First: 20190607]. doi:10.1158/1078-0432.CCR-18-4032
Sundara, Y. T., Kostine, M., Cleven, A. H., Bovée, J. V. M. G., Schilham, M. W., and Cleton-Jansen, A. M. (2017). Increased PD-L1 and T-cell infiltration in the presence of HLA class I expression in metastatic high-grade osteosarcoma: a rationale for T-cell-based immunotherapy. Cancer Immunol. Immunother. 66 (1), 119–128. [published Online First: 20161116]. doi:10.1007/s00262-016-1925-3
Sung, J. Y., Kim, J. H., Kang, H. G., Park, J. W., Park, S. Y., Park, B. K., et al. (2022). ICSBP-induced PD-L1 enhances osteosarcoma cell growth. Front. Oncol. 12, 918216. [published Online First: 20220923]. doi:10.3389/fonc.2022.918216
Ta, H. T., Dass, C. R., Choong, P. F., and Dunstan, D. E. (2009). Osteosarcoma treatment: state of the art. Cancer Metastasis Rev. 28 (1-2), 247–263. doi:10.1007/s10555-009-9186-7
Tang, L., Cegang, F., Zhao, H., Wang, B., Jia, S., Chen, H., et al. (2023). Up-regulation of core 1 beta 1, 3-galactosyltransferase suppresses osteosarcoma growth with induction of IFN-gamma secretion and proliferation of CD8(+) T cells. Curr. Cancer Drug Targets 23 (4), 265–277. doi:10.2174/1568009622666221010105701
Tarone, L., Giacobino, D., Camerino, M., Maniscalco, L., Iussich, S., Parisi, L., et al. (2023). A chimeric human/dog-DNA vaccine against CSPG4 induces immunity with therapeutic potential in comparative preclinical models of osteosarcoma. Mol. Ther. 31 (8), 2342–2359. [published Online First: 20230612]. doi:10.1016/j.ymthe.2023.06.004
Thanindratarn, P., Dean, D. C., Nelson, S. D., Hornicek, F. J., and Duan, Z. (2019). Advances in immune checkpoint inhibitors for bone sarcoma therapy. J. Bone Oncol. 15, 100221. [published Online First: 20190129]. doi:10.1016/j.jbo.2019.100221
Tsagozis, P., Gonzalez-Molina, J., Georgoudaki, A. M., Lehti, K., Carlson, J., Lundqvist, A., et al. (2020). Sarcoma tumor microenvironment. Adv. Exp. Med. Biol. 1296, 319–348. doi:10.1007/978-3-030-59038-3_20
Tsuda, N., Murayama, K., Ishida, H., Matsunaga, K., Komiya, S., Itoh, K., et al. (2001). Expression of a newly defined tumor-rejection antigen SART3 in musculoskeletal tumors and induction of HLA class I-restricted cytotoxic T lymphocytes by SART3-derived peptides. J. Orthop. Res. 19 (3), 346–351. doi:10.1016/S0736-0266(00)90031-7
Tsukahara, T., Nabeta, Y., Kawaguchi, S., Ikeda, H., Sato, Y., Shimozawa, K., et al. (2004). Identification of human autologous cytotoxic T-lymphocyte-defined osteosarcoma gene that encodes a transcriptional regulator, papillomavirus binding factor. Cancer Res. 64 (15), 5442–5448. doi:10.1158/0008-5472.CAN-04-0522
Uehara, T., Eikawa, S., Nishida, M., Kunisada, Y., Yoshida, A., Fujiwara, T., et al. (2019). Metformin induces CD11b+-cell-mediated growth inhibition of an osteosarcoma: implications for metabolic reprogramming of myeloid cells and anti-tumor effects. Int. Immunol. 31 (4), 187–198. doi:10.1093/intimm/dxy079
van Erp, A. E. M., Versleijen-Jonkers, Y. M. H., Hillebrandt-Roeffen, M. H. S., van Houdt, L., Gorris, M. A. J., van Dam, L. S., et al. (2017). Expression and clinical association of programmed cell death-1, programmed death-ligand-1 and CD8(+) lymphocytes in primary sarcomas is subtype dependent. Oncotarget 8 (41), 71371–71384. [published Online First: 20170707]. doi:10.18632/oncotarget.19071
Wang, J., Hu, F., Yu, P., Liu, Z., Bao, Q., Zhang, W., et al. (2023b). Sorafenib inhibits doxorubicin-induced PD-L1 upregulation to improve immunosuppressive microenvironment in Osteosarcoma. J. Cancer Res. Clin. Oncol. 149 (8), 5127–5138. [published Online First: 20221108]. doi:10.1007/s00432-022-04458-4
Wang, J., Jin, J., Chen, T., and Zhou, Q. (2022a). Curcumol synergizes with cisplatin in osteosarcoma by inhibiting M2-like polarization of tumor-associated macrophages. Molecules 27 (14), 4345. [published Online First: 20220706]. doi:10.3390/molecules27144345
Wang, J., Xu, P., Zhang, Y., Han, S., Wang, G., Wang, H., et al. (2023c). Dynamic nanoassemblies derived from small-molecule homodimeric prodrugs for in situ drug activation and safe osteosarcoma treatment. iScience 26 (8), 107409. [published Online First: 20230717]. doi:10.1016/j.isci.2023.107409
Wang, S., Li, H., Chen, T., Zhou, H., Zhang, W., Lin, N., et al. (2023a). Human γδ T cells induce CD8+ T cell antitumor responses via antigen-presenting effect through HSP90-MyD88-mediated activation of JNK. Cancer Immunol. Immunother. 72 (6), 1803–1821. [published Online First: 20230121]. doi:10.1007/s00262-023-03375-w
Wang, S., Li, H., Ye, C., Lin, P., Zhang, W., Sun, L., et al. (2018a). Valproic acid combined with zoledronate enhance γδ T cell-mediated cytotoxicity against osteosarcoma cells via the accumulation of mevalonate pathway intermediates. Front. Immunol. 9, 377. [published Online First: 20180227]. doi:10.3389/fimmu.2018.00377
Wang, S. D., Li, H. Y., Li, B. H., Xie, T., Zhu, T., Sun, L. L., et al. (2016). The role of CTLA-4 and PD-1 in anti-tumor immune response and their potential efficacy against osteosarcoma. Int. Immunopharmacol. 38, 81–89. [published Online First: 20160531]. doi:10.1016/j.intimp.2016.05.016
Wang, Y., Wu, Y., Li, L., Zhang, S., Lin, S., Zhang, L. W., et al. (2023d). Chemotherapy-sensitized in situ vaccination for malignant osteosarcoma enabled by bioinspired calcium phosphonate nanoagents. ACS Nano 17 (7), 6247–6260. [published Online First: 20230324]. doi:10.1021/acsnano.2c09685
Wang, Y., Yu, W., Zhu, J., Wang, J., Xia, K., Liang, C., et al. (2019). Anti-CD166/4-1BB chimeric antigen receptor T cell therapy for the treatment of osteosarcoma. J. Exp. Clin. Cancer Res. 38 (1), 168. [published Online First: 20190417]. doi:10.1186/s13046-019-1147-6
Wang, Z., Fan, G., Zhu, H., Yu, L., Wei, Y., Huang, J., et al. (2022b). PLOD2 high expression associates with immune infiltration and facilitates cancer progression in osteosarcoma. Front. Oncol. 12, 980390. [published Online First: 20221005]. doi:10.3389/fonc.2022.980390
Wang, Z., Wang, Z., Li, S., Sun, L., Li, H., Lin, P., et al. (2018b). Decitabine enhances Vγ9Vδ2 T cell-mediated cytotoxic effects on osteosarcoma cells via the nkg2dl-nkg2d Axis. Front. Immunol. 9, 1239. [published Online First: 20180601]. doi:10.3389/fimmu.2018.01239
Watanabe, K., Tsukahara, T., Toji, S., Saitoh, S., Hirohashi, Y., Nakatsugawa, M., et al. (2019). Development of a T-cell receptor multimer with high avidity for detecting a naturally presented tumor-associated antigen on osteosarcoma cells. Cancer Sci. 110 (1), 40–51. [published Online First: 20181201]. doi:10.1111/cas.13854
Wedekind, M. F., Wagner, L. M., and Cripe, T. P. (2018). Immunotherapy for osteosarcoma: where do we go from here? Pediatr. Blood Cancer 65 (9), e27227. [published Online First: 20180619]. doi:10.1002/pbc.27227
Wiebel, M., Kailayangiri, S., Altvater, B., Meltzer, J., Grobe, K., Kupich, S., et al. (2021). Surface expression of the immunotherapeutic target G(D2) in osteosarcoma depends on cell confluency. Cancer Rep. Hob. 4 (5), e1394. [published Online First: 20210402]. doi:10.1002/cnr2.1394
Withers, S. S., Skorupski, K. A., York, D., Choi, J. W., Woolard, K. D., Laufer-Amorim, R., et al. (2019). Association of macrophage and lymphocyte infiltration with outcome in canine osteosarcoma. Vet. Comp. Oncol. 17 (1), 49–60. [published Online First: 20180919]. doi:10.1111/vco.12444
Wolf-Dennen, K., Gordon, N., and Kleinerman, E. S. (2020). Exosomal communication by metastatic osteosarcoma cells modulates alveolar macrophages to an M2 tumor-promoting phenotype and inhibits tumoricidal functions. Oncoimmunology 9 (1), 1747677. [published Online First: 20200412]. doi:10.1080/2162402X.2020.1747677
Wu, H., Wang, R., Li, S., Chen, S., Liu, S., Li, X., et al. (2023). Aspect ratio-dependent dual-regulation of the tumor immune microenvironment against osteosarcoma by hydroxyapatite nanoparticles. Acta Biomater. 170, 427–441. [published Online First: 20230825]. doi:10.1016/j.actbio.2023.08.046
Wu, L., Mao, L., Liu, J. F., Chen, L., Yu, G. T., Yang, L. L., et al. (2019). Blockade of TIGIT/CD155 signaling reverses T-cell exhaustion and enhances antitumor capability in head and neck squamous cell carcinoma. Cancer Immunol. Res. 7 (10), 1700–1713. [published Online First: 20190806]. doi:10.1158/2326-6066.CIR-18-0725
Wu, W., Jing, D., Meng, Z., Hu, B., Zhong, B., Deng, X., et al. (2020). FGD1 promotes tumor progression and regulates tumor immune response in osteosarcoma via inhibiting PTEN activity. Theranostics 10 (6), 2859–2871. [published Online First: 20200203]. doi:10.7150/thno.41279
Xiang, D., Han, X., Li, J., Zhang, J., Xiao, H., Li, T., et al. (2023). Combination of Ido inhibitors and platinum(IV) prodrugs reverses low immune responses to enhance cancer chemotherapy and immunotherapy for osteosarcoma. Mater Today Bio 20, 100675. [published Online First: 20230524]. doi:10.1016/j.mtbio.2023.100675
Xie, X., Feng, Y., Zhang, H., Su, Q., Song, T., Yang, G., et al. (2022). Remodeling tumor immunosuppressive microenvironment via a novel bioactive nanovaccines potentiates the efficacy of cancer immunotherapy. Bioact. Mater 16, 107–119. [published Online First: 20220311]. doi:10.1016/j.bioactmat.2022.03.008
Yahiro, K., Matsumoto, Y., Yamada, H., Endo, M., Setsu, N., Fujiwara, T., et al. (2020). Activation of TLR4 signaling inhibits progression of osteosarcoma by stimulating CD8-positive cytotoxic lymphocytes. Cancer Immunol. Immunother. 69 (5), 745–758. [published Online First: 20200211]. doi:10.1007/s00262-020-02508-9
Yamanegi, K., Yamane, J., Kobayashi, K., Kato-Kogoe, N., Ohyama, H., Nakasho, K., et al. (2010). Sodium valproate, a histone deacetylase inhibitor, augments the expression of cell-surface NKG2D ligands, MICA/B, without increasing their soluble forms to enhance susceptibility of human osteosarcoma cells to NK cell-mediated cytotoxicity. Oncol. Rep. 24 (6), 1621–1627. doi:10.3892/or_00001026
Yan, C. F., Xia, J., Qun, W. S., Bing, W. Y., Guo, W. J., Yong, H. G., et al. (2023). Tumor-associated macrophages-derived exo-let-7a promotes osteosarcoma metastasis via targeting C15orf41 in osteosarcoma. Environ. Toxicol. 38 (6), 1318–1331. [published Online First: 20230315]. doi:10.1002/tox.23766
Yang, Q., Hu, J., Jia, Z., Wang, Q., Wang, J., Dao, L. H., et al. (2022). Membrane-anchored and tumor-targeted IL12 (attIL12)-PBMC therapy for osteosarcoma. Clin. Cancer Res. 28 (17), 3862–3873. doi:10.1158/1078-0432.CCR-22-0721
Yang, Y., Li, C., Liu, T., Dai, X., and Bazhin, A. V. (2020). Myeloid-derived suppressor cells in tumors: from mechanisms to antigen specificity and microenvironmental regulation. Front. Immunol. 11, 1371. [published Online First: 20200722]. doi:10.3389/fimmu.2020.01371
Yoshida, K., Okamoto, M., Sasaki, J., Kuroda, C., Ishida, H., Ueda, K., et al. (2020). Anti-PD-1 antibody decreases tumour-infiltrating regulatory T cells. BMC Cancer 20 (1), 25. [published Online First: 20200108]. doi:10.1186/s12885-019-6499-y
Yu, L., Fan, G., Wang, Q., Zhu, Y., Zhu, H., Chang, J., et al. (2023). In vivo self-assembly and delivery of VEGFR2 siRNA-encapsulated small extracellular vesicles for lung metastatic osteosarcoma therapy. Cell Death Dis. 14 (9), 626. [published Online First: 20230922]. doi:10.1038/s41419-023-06159-3
Yu, L., Zhang, J., and Li, Y. (2022). Effects of microenvironment in osteosarcoma on chemoresistance and the promise of immunotherapy as an osteosarcoma therapeutic modality. Front. Immunol. 13, 871076. [published Online First: 20221013]. doi:10.3389/fimmu.2022.871076
Yu, W., Wang, Y., Zhu, J., Jin, L., Liu, B., Xia, K., et al. (2019). Autophagy inhibitor enhance ZnPc/BSA nanoparticle induced photodynamic therapy by suppressing PD-L1 expression in osteosarcoma immunotherapy. Biomaterials 192, 128–139. [published Online First: 20181113]. doi:10.1016/j.biomaterials.2018.11.019
Zhang, H., Yu, Y., Wang, J., Han, Y., Ren, T., Huang, Y., et al. (2021a). Macrophages-derived exosomal lncRNA LIFR-AS1 promotes osteosarcoma cell progression via miR-29a/NFIA axis. Cancer Cell Int. 21 (1), 192. [published Online First: 20210401]. doi:10.1186/s12935-021-01893-0
Zhang, Y., Shen, G., Meng, T., Lv, Z., Li, X., Li, J., et al. (2023). Eicosapentaenoic acid enhances the sensitivity of osteosarcoma to cisplatin by inducing ferroptosis through the DNA-PKcs/AKT/NRF2 pathway and reducing PD-L1 expression to attenuate immune evasion. Int. Immunopharmacol. 125 (Pt B), 111181. [published Online First: 20231109]. doi:10.1016/j.intimp.2023.111181
Zhang, Y., Yuan, T., Li, Z., Luo, C., Wu, Y., Zhang, J., et al. (2021b). Hyaluronate-based self-stabilized nanoparticles for immunosuppression reversion and immunochemotherapy in osteosarcoma treatment. ACS Biomater. Sci. Eng. 7 (4), 1515–1525. [published Online First: 20210401]. doi:10.1021/acsbiomaterials.1c00081
Zhang, Z., Li, Y., Huang, L., Xiao, Q., Chen, X., Zhong, J., et al. (2016). Let-7a suppresses macrophage infiltrations and malignant phenotype of Ewing sarcoma via STAT3/NF-κB positive regulatory circuit. Cancer Lett. 374 (2), 192–201. [published Online First: 20160220]. doi:10.1016/j.canlet.2016.02.027
Zheng, B., Ren, T., Huang, Y., Sun, K., Wang, S., Bao, X., et al. (2018b). PD-1 axis expression in musculoskeletal tumors and antitumor effect of nivolumab in osteosarcoma model of humanized mouse. J. Hematol. Oncol. 11 (1), 16. [published Online First: 20180206]. doi:10.1186/s13045-018-0560-1
Zheng, C., Li, H., Zhao, X., Yang, S., Zhan, J., Liu, H., et al. (2024a). Expression of PD-1 mitigates phagocytic activities TAM in osteosarcoma. Heliyon 10 (1), e23498. [published Online First: 20231213]. doi:10.1016/j.heliyon.2023.e23498
Zheng, Y., Wang, G., Chen, R., Hua, Y., and Cai, Z. (2018a). Mesenchymal stem cells in the osteosarcoma microenvironment: their biological properties, influence on tumor growth, and therapeutic implications. Stem Cell Res. Ther. 9 (1), 22. [published Online First: 20180131]. doi:10.1186/s13287-018-0780-x
Zheng, Z., Zhao, X., Yuan, B., Jiang, S., Yan, R., Dong, X., et al. (2024b). Soy isoflavones induces mitophagy to inhibit the progression of osteosarcoma by blocking the AKT/mTOR signaling pathway. Mol. Med. 30 (1), 5. [published Online First: 20240108]. doi:10.1186/s10020-024-00778-y
Zhou, Y., Slone, N., Chrisikos, T. T., Kyrysyuk, O., Babcock, R. L., Medik, Y. B., et al. (2020b). Vaccine efficacy against primary and metastatic cancer with in vitro-generated CD103(+) conventional dendritic cells. J. Immunother. Cancer 8 (1), e000474. doi:10.1136/jitc-2019-000474
Zhou, Y., Yang, D., Yang, Q., Lv, X., Huang, W., Zhou, Z., et al. (2020a). Single-cell RNA landscape of intratumoral heterogeneity and immunosuppressive microenvironment in advanced osteosarcoma. Nat. Commun. 11 (1), 6322. [published Online First: 20201210]. doi:10.1038/s41467-020-20059-6
Keywords: osteosarcoma, tumor immune microenvironment, immunotherapy, tumor microenvironment modulators, cell-based therapies, immune checkpoint inhibitors
Citation: Liang H, Cui M, Tu J and Chen X (2024) Advancements in osteosarcoma management: integrating immune microenvironment insights with immunotherapeutic strategies. Front. Cell Dev. Biol. 12:1394339. doi: 10.3389/fcell.2024.1394339
Received: 01 March 2024; Accepted: 29 May 2024;
Published: 10 June 2024.
Edited by:
Ming Yi, Zhejiang University, ChinaReviewed by:
Songfeng Chen, First Affiliated Hospital of Zhengzhou University, ChinaCopyright © 2024 Liang, Cui, Tu and Chen. This is an open-access article distributed under the terms of the Creative Commons Attribution License (CC BY). The use, distribution or reproduction in other forums is permitted, provided the original author(s) and the copyright owner(s) are credited and that the original publication in this journal is cited, in accordance with accepted academic practice. No use, distribution or reproduction is permitted which does not comply with these terms.
*Correspondence: Xinyi Chen, cxyjozee@foxmail.com; Jingyao Tu, tujingyao0702@163.com
Disclaimer: All claims expressed in this article are solely those of the authors and do not necessarily represent those of their affiliated organizations, or those of the publisher, the editors and the reviewers. Any product that may be evaluated in this article or claim that may be made by its manufacturer is not guaranteed or endorsed by the publisher.
Research integrity at Frontiers
Learn more about the work of our research integrity team to safeguard the quality of each article we publish.