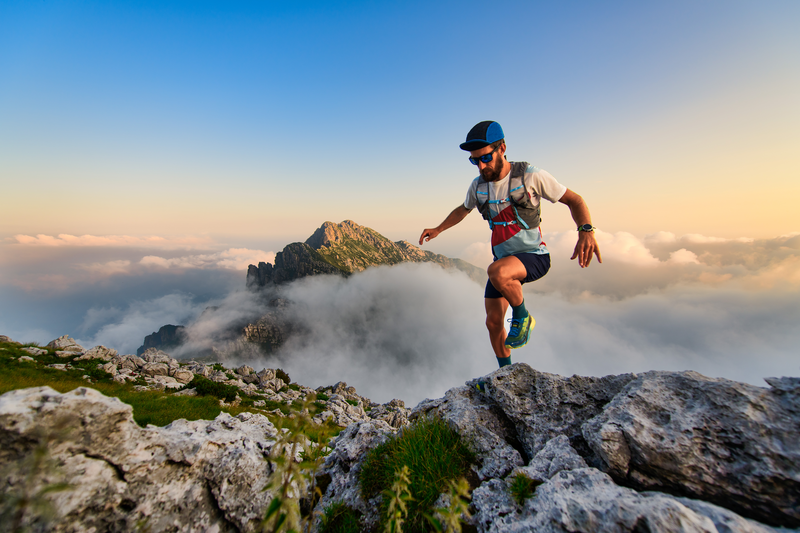
95% of researchers rate our articles as excellent or good
Learn more about the work of our research integrity team to safeguard the quality of each article we publish.
Find out more
MINI REVIEW article
Front. Cell Dev. Biol. , 29 July 2024
Sec. Epigenomics and Epigenetics
Volume 12 - 2024 | https://doi.org/10.3389/fcell.2024.1392391
This article is part of the Research Topic Epigenetic Silencing of Tumor Suppressor Genes and Their Functions in Cancer Development View all articles
The epigenetic layer of regulation has become increasingly relevant in the research focused on tumor suppressors. KLF4 is a well-described zinc-finger transcription factor, mainly known for its role in the acquisition of cell pluripotency. Here we report and describe the most relevant epigenetic regulation mechanisms that affect KLF4 expression in tumors. CpG island methylation emerges as the most common mechanism in several tumors including lung adenocarcinoma, hepatocellular carcinoma, non-Hodgkin lymphomas, among others. Further layers of regulation represented by histone methylation and acetylation and by non-coding RNAs are described. Overall, KLF4 emerges as a crucial target in the fight against cancer.
Krüppel-like factor 4 (KLF4) belongs to a family of transcription factors (TFs) playing a variety of functions and having a significant potential in cancer development and progression. The gene is located on human chromosome 9 and contains 5 exons (Figure 1A) (NCBI, 2023). KLF4 is a zinc finger-containing transcription factor and is among the four factors able to induce pluripotent stem cells (together with OCT3/4, SOX2 and c-MYC) (Dang et al., 2000; Kalra et al., 2011). The three zinc finger-motifs are adjacent and located at the C-terminus of the sequence. At the N-terminus there is a transactivation domain adjacent to a repression domain. These, together, define the specificity of the transcriptional regulation and the efficiency of DNA binding, in cooperation with other factors (Ghaleb and Yang, 2017). The gene contains also two nuclear localization signals, one close to the most amino-terminal zinc finger domain, while the second spanning the first and half of the second zinc finger domain (Shields and Yang, 1997). The simultaneous presence of activatory and repressory domains within the KLF4 gene allows this transcription factor to exert its specific activity on different targets, according to the specific tissue distribution (Li et al., 2015). The current knowledge points towards KLF4 as a tumor suppressor in both solid and hematological tumors, where the gene is silenced by means of a variety of mechanisms including DNA hypermethylation, interactions with miRNAs, histone modifications and others, described subsequently in the manuscript (Guan et al., 2010; Frazzi et al., 2017; Frazzi et al., 2022). A few evidences exist though supporting a role as an oncogene. For instance, the malignant progression of ductal carcinoma in situ (DCIS) is driven by the overexpression of KLF4 (Damaghi et al., 2021). This transcription factor, together with NF-kB activation, plays a role in the initial phase of the development of one of the most diagnosed human cancers: breast carcinoma. Thus, in DCIS, KLF4 plays an oncogenic role. The available literature reports a number of different epigenetic mechanisms that have been involved in the regulation of this zinc-finger transcription factor. In the present manuscript, the main mechanisms of epigenetic modulation of KLF4 expression in tumor cells are described and commented. Furthermore, some epigenetic modifications linked to the regulatory and topological functions of KLF4 TF are outlined. These histone modifications represent epigenetic marks that directly influence not only KLF4 expression but also its binding to targets. The literature up to 2023 spans a variety of tumors of different histological origins and unveils recurrent epigenetic layers of modulation.
Figure 1. The main layers of KLF4 epigenetic regulation discussed in the text are represented. (A) Structure of KLF4 gene (GRCh38.p14 assembly). (B) Methylation at specific CpG sites within KLF4 CpG islands. S-adenosyl-methionine is the universal methyl group donor in DNA- and histone-methylation reactions. (C) Methylation of specific histone residues represent repressory marks at specific DNA sequences. The interaction of DNMT1 with histone methyltransferases affects the chromatin architecture. (D) Histone methylation on specific histone residues within regulatory regions may represent activatory or repressory marks. The process is finely tuned through histone methyltransferases and demethylases. (E) KLF4 binding to enhancer regions, enriched in histone activation marks (H3K27ac, H3K4me1, H3K4me3), is a characteristic of the regulatory footprint of some tumors.
DNA methylation can mediate gene inactivation through different mechanisms (Figure 1B). It can attract CpG-methylated-DNA binding proteins or histone deacetylases (HDACs) and it may induce variations in histone methylation (Jin et al., 2011; Patra et al., 2011). The epigenetic layer of regulation has been demonstrated in lymphoma cells, were KLF4 is consistently hypermethylated in B lymphocytes sorted from diffuse large-B cell lymphomas (DLBCL), follicular lymphomas (FL) and non Hodgkin lymphoma (NHL) cell lines (Guan et al., 2010; Frazzi et al., 2017; Frazzi et al., 2022). The evidence that peripheral blood mononuclear cells from healthy donors display a consistently demethylated KLF4 promoter suggests that the hypermethylated phenotype is associated to B cell malignancy (Frazzi et al., 2022).
Burkitt lymphoma is another subtype where KLF4 oncosuppressive role has been recently demonstrated (Bialopiotrowicz et al., 2020). Histone H3K27 and DNA methylation decrease in BL cells led to the reactivation of the methylation-regulated tumor suppressor genes: Cyclin Dependent Kinase Inhibitor 2B (CDK2NB), KLF4, Inhibitor of DNA Binding 4 (ID4), and Thioredoxin Interacting Protein (TXNIP) (Bialopiotrowicz et al., 2020).
Furthermore, KLF4 is a known partner of YY1 in leukocytes and B-NHL. The evidences emerged especially in the last 7 years, demonstrate a constitutive interaction between KLF4 and YY1. In this context, KLF4 acts as an oncogene in B-NHL [(Khan et al., 2019; Morales-Martinez et al., 2019; Morales-Martinez et al., 2020; Montecillo-Aguado et al., 2021)].
KLF4 emerges also in a recent bioinformatics analysis of lung adenocarcinoma (including 497 tumors and 54 adjacent normal tissue samples). The low expression of KLF4 (together with other hub-genes) correlated significantly with the poor overall survival (OS) of lung cancer patients (Cai et al., 2022). KLF4 resulted downregulated due to increased miRNA and hypermethylation (Cai et al., 2022). These data confirm the previous ones published on NHL pointing towards the epigenetic regulation of KLF4 gene expression.
Approved for the therapy of renal cell, differentiated thyroid and hepatocellular carcinomas, sorafenib exerts an antiproliferative and antiangiogenic activity through the inhibition of serine/threonine and tyrosine kinases (Gong et al., 2017). Sorafenib acts by modulating epigenetic pathways involving DNA methyltransferase 1 (DNMT1) and KLF4 (Figure 1C). Upon DNMT1 inhibition, the expression of metallothionein 1G (MT1G) results upregulated (Wei et al., 2022). MT1G belongs to the family of metallothioneines and inhibits several types of human cancers as thyroid, colorectal, pancreatic and its upregulation is associated to sorafenib treatment (Houessinon et al., 2016; Zeng et al., 2018). The MT1G expression increases upon sorafenib and also parallels the dysregulation of two crucial genes: KLF4 and Carbonic Anhydrase 9 (CA9). KLF4 results upregulated and suppresses CA9, through the inhibition of Hypoxia Inducible Factor 1 alfa (HIF-1α). KLF4 also downregulates two more targets of the HIF-1α axis (Vascular Endothelial Growth Factor A and Solute Carrier Family 2 Member 1), possibly by reducing HIF-1α protein levels (Wei et al., 2022).
Epigenetic silencing has been also demonstrated in T-cell acute lymphoblastic leukemia (T-ALL), where KLF4 serves as tumor suppressor in experimental animal models and xenografts generated using lymphoblasts from pediatric T-ALL patients (Shen et al., 2017; Chen et al., 2021).
KLF4 emerged also in a panel of epigenetically regulated genes in colorectal cancer (CRC) (Li et al., 2021). The differentially methylated regions located within the promoters of TNNI2, PAX8, GUF1, KLF4, EVI2B, CEP112 and long non-coding RNA AC011298 genes are associated with the probability of liver metastasis in CRC patients. When analyzed through a model of predictive performance (leave-one-out cross validation; LOOCV), the area under the curve (AUC) was 0.701 while the ability to distinguish metastasis of different sites had an AUC = 0.933 (Li et al., 2021).
Loss of heterozigosity and promoter hypermethylation were demonstrated in pancreatic cancer as the mechanisms responsible for the downregulation of KLF4 (Xie et al., 2017). Thus, the activity of DNMT1 is specifically linked to KLF4 downregulation. The immunohistochemical (IHC) staining on a total of 84 human pancreatic samples demonstrates that DNMT1 expression inversely correlates with KLF4 (Xie et al., 2017).
More consistent data on the direct role of DNMT1 in controlling KLF4 expression were demonstrated in prostate cancer (PCa). The ability of prostate cell to undergo epithelial to mesenchimal transition (EMT) is key in driving malignant transformation (Lee et al., 2016). The induction of EMT initiates a self-renewal mechanism leading to the formation of the cancer stem cells (CSCs) (Mani et al., 2008; Jung et al., 2013). Interestingly, the downregulation of DNMT1 drives EMT and promotes CSCs formation in PCa. The histone demethylation following 5-azacytidine treatment (H3K9me3 and H3K27me3 specifically) of the KLF4 promoter is one of the downstream effects of DNMT1 reduction (Lee et al., 2016). The effects of DNMT1 downregulation are possibly due to the known function of this enzyme in maintaining the architecture of large heterochromatic regions. This is achieved also by modulating the histone H3 methylation and accompanied by an altered nuclear architecture (Espada et al., 2004). EZH2 (enhancer of zeste homolog 2), a member of the polycomb repressor complex-2 (PRC2) is a histone lysine methyltransferase known to interact directly with DNMT1 (Figure 1C). Moreover, HP1 (heterochromatin protein 1) represents a link between heterochromatin and the methylation machinery, interacting with DNMT1 directly and mediating the silencing of euchromatic genes (Jin et al., 2011). Preliminary evidences also show that KLF4, together with Zeb2 and N-cadherin increased expression are associated to advanced PCa. These last evidences support the tumor promoting role of KLF4 (Lee et al., 2016).
Preliminary data on 52 human samples show that KLF4 expression is low in high grade dysplasia and early stage esophageal squamous cell carcinoma (ESCC), while it becomes prevalent in high grade ESCC (Yang and Katz, 2016). However, KLF4 levels increased with tumor size and with the presence of nodal metastases compared to the ones without metastatic spread. One of the proposed mechanisms for low KLF4 expression, also in ESCC, is promoter methylation (Yang and Katz, 2016).
Oral squamous cell carcinoma (OSCC) is another cancer where the epigenetic regulation of KLF4 has been demonstrated. The classical function of tumor suppressor is studied through gene expression and promoter methylation approaches (Li et al., 2015). IHC demonstrates that KLF4 staining decreases going from well-differentiated to moderately differentiated and to poorly differentiated OSCC (n = 39). The quantitative methylation of the two CpG islands localized upstream of the transcription starting site consistently display a decreasing methylation percentage in the order: OSCC > dysplasia and healthy oral mucosa. The overexpression of KLF4 leads to the inhibition of tumor growth, cell proliferation and angiogenesis in vitro and in tumor xenografts, confirming its tumor suppressive role (Li et al., 2015).
The demonstration that KLF4 transcription is modulated through epigenetic mechanisms has been obtained also in hepatocellular carcinoma (HCC). A non-promoter CpG island located within the KLF4 sequence (proximal to the retained intron of KLF4-003, a non coding transcript of the KLF4 gene) has been pyrosequenced (Zhou et al., 2015). KLF4-003 was downregulated in HCC tumor samples compared to their normal counterparts (n = 54) and this was significantly associated with HCC recurrence but no other clinical parameters. The hypermethylation of the proximal non-promoter CpG island is likely the mechanism responsible for the KLF4-003 reduced expression (Zhou et al., 2015). Overall, the reprogramming towards pluripotency is considered a promoter for HCC progression and is mediated by promoter methylation regulation of key TFs (Wang et al., 2013).
KLF4 is downregulated also in lung cancer compared to nontumor tissues, presenting the characteristics of tumor suppressor. Furthermore, KLF4 deletion promotes lung tumor formation and progression in mouse models. The downregulation is achieved by means of epigenetic mechanisms and, to a little extent, genetic mutations (Yu et al., 2016). Histone deacetylase (HDAC) inhibition seems to be the most relevant mechanism controlling KLF4 expression in lung cancer cell lines A549 and H460. The rationale is given by the fact that HDAC1 and HDAC3 may interact with KLF4 modulating its transcription (Yu et al., 2016).
Head and neck squamous cell carcinoma (HNSCC) radioresistant tumors display a higher methylation compared to non resistant ones (Chen et al., 2015). This methylation phenotype affects the gene expression profile and is confirmed by human HNSCC datasets from the TCGA where an increase in DNA methylation is associated to radiation resistance (Chen et al., 2015).
Promoter hypermethylation is the mechanism responsible for KLF4 downregulation also in urothelial cancer, renal cell carcinoma, medulloblastoma, colorectal adenoma-carcinoma and gastric cancer (Choi et al., 2006; Cho et al., 2007; Xu et al., 2008; Nakahara et al., 2010; Li et al., 2013; Li et al., 2014), making the control of gene expression by means of promoter methylation a general strategy adopted by different tumors.
Interestingly, NOTCH1 can repress KLF4 expression through the formation of a repressory complex on the gene. This complex includes LSD1 histone demethylase and PRC2, a methyl transferase responsible for methylation of H3K27 histone. The deposition of the repressory mark H3K27me3 can explain the repressory function exerted by the NOTCH1-containing PRC2 complex on KLF4 gene (Han et al., 2017). The repression of KLF4 transcription may also be exerted through the epigenetic silencing due to H3K4 demethylation by KDM5B in acute myeloid leukemia (Faber et al., 2013). Collectively, these evidences demonstrate how the chromatin conformational changes mediated by histone methylation/demethylation represent a mechanism adopted by cancer cells to downregulate KLF4 (Figure 1D).
The gene regulation by miRNAs is a consolidated mechanism available for epigenetic modulation of gene expression in cancer cells (Ebrahimi et al., 2023; Szczepanek and Tretyn, 2023). Among the several examples of transcriptome programs affected by miRNAs, here are reported some examples that specifically involve KLF4. The miR-302/367 cluster is responsible for the reprogramming of glioblastoma U87 cells towards a more benign phenotype (Yang et al., 2015). The epigenetic reprogramming potential of miR-302/367 cluster can be applied to shift cancer cells abolishing their tumorigenic characteristics through a variety of targets. The pluripotency transcription factors OCT3/4, SOX2, KLF4, and c-MYC (OSKM) result downregulated upon miRNA cluster induction and, together with POU3F2, SALL2 and OLIG2, are required for the maintenance of glioblastoma stem-like tumor propagating cells (Yang et al., 2015) (Figure 2).
Figure 2. miRNAs regulate KLF4 gene expression at various levels. (A) Post-transcriptional hybridization to 3’-UTR has been demonstrated to be the main mechanism of miR-1233-3p and miR -92a in downregulating KLF4 expression (B). The interaction of miRNAs with histone modifying enzymes (writers and erasers) regulates chromosomes architecture and affects gene expression. It can be direct through the interaction with histone proteins or by affecting the expression of histone modifying enzymes (Szczepanek and Tretyn, 2023).
The same is true in CRC, where a reprogramming mediated by exogenous miR-302s and miR-369s leads to the expression of pluripotency factors. The re-expression of OSKM may lead to reprogramming of differentiated somatic cells back to pluripotent stem cells (Miyoshi et al., 2010; Ogawa et al., 2015). The suppression of the malignant phenotype that follows is achieved through promoter demethylation of oncosuppressor p16INK4A pathway and epigenetic alterations of the PRC2 affecting H3K27 methylation (Miyoshi et al., 2010) (Figure 2).
The epigenetic regulation mediated by miRNAs has been reported also in liver cancer, lung adenocarcinoma, breast cancer, CRC, gastric cancer, glioblastoma (Ogawa et al., 2015; Yang et al., 2015; Vidal et al., 2016; Chen et al., 2018; Lu et al., 2020; Cai et al., 2022; Jiang et al., 2023).
Circular RNA (circRNA) is a covalent head-to-tail looped RNA discovered and characterized mostly thanks to the next-generation sequencing techniques in the most recent years (Shen et al., 2015).
Tumor suppression is among the known roles played by circRNAs (Lu et al., 2020). Interestingly, KLF4 is upregulated by the circRNA circEHMT1 at mRNA and protein levels in breast cancer, consistently with its oncosuppressor role (Lu et al., 2020). Moreover, the circRNA CDR1as affects stemness and proliferation of hepatoblastoma cells in vitro. CDR1as interacts with miR-7-5p sponging this miRNA. KLF4 is a downstream target gene of miR-7-5p and mediates the inhibition of proliferation and stemness (Chen et al., 2020).
Cell-fate decisions are concerted processes initiated by TFs that regulate gene expression together with epigenetic modifications (Sardina et al., 2018). Among the most relevant epigenetic modifications there is cytosine methylation in position 5 of the DNA (5mC), which is also relevant in directing the TF binding specificity (Figure 1A) (Sardina et al., 2018). Furthermore, the product of 5mC oxidation (5hmC) recruits chromatin remodelling complexes onto DNA. The B-cell reprogramming into iPSC is accompanied by an almost complete loss of 5mC, with a concomitant increase in 5hmC (Chen et al., 2020). The sustained 5hmC conversion corresponds to an enrichment of specific TFs onto gene regulatory elements (GRE) related to pluripotency and/or genome topology, including KLF4. Furthermore, the demethylation of the core pluripotency TFs (OSKM) leads to their activation.
During pluripotent cell reprogramming, there are some TFs triggering chromatin reconfiguration and TF occupancy. Namely, these are OSKM (Huyghe et al., 2022). Their activity leads to the increase in cellular plasticity and loss of cell specialization in mouse embryonic fibroblasts. This is followed by the transition to induced pluripotent stem cells (iPSC) due to the activation of the pluripotent network (Huyghe et al., 2022).
Transcriptional reprogramming and loss of identity share common characteristics with malignant transformation, both being constrained by oncogenic barriers. The increase of cell plasticity is driven by oncogenes like KRAS, whose oncogenic variant displays aspartic acid instead of glycine at position 12 (KRASG12D). Simultaneous p53 deficiency, c-Myc expression and KRASG12D mutation lead the cell towards plasticity, fostering the early malignant transformation in human cancers (Ischenko et al., 2013; Dost et al., 2020; Marjanovic et al., 2020).
Long non-coding RNAs (lncRNAs) also represent a relevant tool driving cell plasticity by modulating the epigenetic modifications that lead to pluripotency (Du et al., 2021). The lncRNA Platr10 interacts with Oct4 promoter and recruits TET1. This latter enzyme is responsible for the site-specific demethylation, a phenomenon known to be the initial step for the initiation of pluripotency. Among the multiple-pluripotency genes modulated through Platr10 there is also KLF4 (Du et al., 2021).
Also, pancreatic cancer stem cells (CSCs) result regulated by telomerase activity and telomere length. An interplay between stemness factors and telomere length has been recently reported (Walter et al., 2021). CSCs isolated from patient-derived xenografts (PDXs) present higher telomerase activity and significantly longer telomeres compared to bulk tumor cells. The telomere lengthening depends on the pluripotency/stemness factors OCT3/4, SOX2, NANOG and KLF4, which increase their expression specifically in CSCs. Of note, KLF4 is the one recruited by PARP1 and activating telomerase expression in embryonic stem cells (Hsieh et al., 2017). Interestingly, the upregulation of one of these factors is paralleled by the concomitant upregulation of the others, suggesting a coordinated mechanism of gene expression (Walter et al., 2021). The interaction between the activator of epithelial and pluripotency programmes JMJD3 and KLF4 is the basis for the recruitment of the former on enhancers and promoters of the specific genes (Huang et al., 2020).
The central role of KLF4 in the organization of three-dimensional (3D) enhancers network was elegantly demonstrated in the transition of mouse embryonic fibroblasts to pluripotent stem cells (PSCs) (Di Giammartino et al., 2019). Technologies aimed at defining local or large-scale 3D genomic architecture are represented by targeted or global chromatin conformation capture techniques. These demonstrate differences between somatic and iPSCs together with a strong association with the OSKM TFs, responsible for the somatic cell reprogramming. These reprogramming TFs thus feature an architectural role. KLF4 depletion, for instance, causes the abrogation of long-distance interactions at specific genomic loci (Wei et al., 2013).
The association of KLF4 with super-enhancers in head and neck squamous cell carcinomas HNSCC is a further evidence of the architectural role that this TF plays in cancer development (Tsompana et al., 2020). The dual oncogenic and tumor suppressor role of KLF4 emerges clearly in the association with chromatin and its structural properties. KLF4 is the only member of the KLF-family to be upregulated at mRNA and protein levels in human HNSCC epithelial oral cell line SCC25 compared to the primary human gingival epithelial cell line HGEP (Tsompana et al., 2020). ATAC-seq footprints confirmed these data suggesting that KLF4 is a key regulator in differential active chromatin modifications, activating genes that are relevant for HNSCC (Figure 1E). Further ChIP-seq experiments demonstrated an enrichment in the activatory H3K27ac, H3K4me1, and H3K4me3 histone modifications, bound by KLF4. Super-enhancers, defined by size, TF density, association with lineage-specific TFs and activatory histone modifications, were identified in HNSCC and were bound by KLF4 and deltaNp63 (Figure 1E) (Chapuy et al., 2013; Sethi et al., 2017; Tsompana et al., 2020).
Overall, these evidences confirm the context-dependent role of KLF4 that can act both as a tumor suppressor (as in the case of NHL and CRC) or as a tumor promoter (as in the case of HNSCC).
Another role for KLF4, emerged during the past few years, concerns the topological and tridimensional organization of the promoters-enhancers interactions. As known, KLF4 binds to sequences containing methylated CpG (mCpG) (Wan et al., 2017). The trans-activating capabilities of KLF4 are directed towards non-receptor tyrosine kinases like Src tyrosine-kinases which mediate cellular processes such as migration, adhesion, invasion, angiogenesis, proliferation, and differentiation (Oyinlade et al., 2018). In a model of glioblastoma, where KLF4 was inducible, the issue of the binding to highly-methylated enhancers was addressed. On a total of 3,802 binding fragments, 2,477 had at least one mCpG site (defined by a β value > 0.5) based on whole genome bisulfite sequencing (Oyinlade et al., 2018). The majority of these sites were associated to highly methylated KLF4 binding sites, H3K27ac modifications and located within gene bodies or intergenic enhancers. Another location was upstream of the gene and within the 5’UTR. The B-cell lymphocyte kinase, taken as a model, was upregulated by KLF4 binding to the mCpG at both the promoter region (5’UTR) and enhancer region, located at the 3’UTR (Oyinlade et al., 2018).
A notable example of parentally imprinted genes are the methylation-regulated IGF2 and H19 gene clusters (Schagdarsurengin et al., 2017). Within the P0 promoter of IGF2 there is a differentially methylated region containing a putative KLF4 binding site (DMR0). The hypomethylation of IGF2-DMR0 is associated to lower IGF2 expression in PCa tissues compared to benign prostate hyperplasia. KLF4 binds to the hypomethylated IGF2-DMR0, leading to reduced IGF2 mRNA expression (Schagdarsurengin et al., 2017).
The epigenetic regulation of the zinc-finger TF KLF4 plays a relevant role in several types of cancers, as demonstrated by the recent literature. DNA methylation at CpG sites represents a recurrent and widespread epigenetic mechanism for KLF4 gene silencing in both solid and hematological tumors (Table 1). Histone modifications (either activatory or inhibitory) may influence the chromatin spatial organization, bearing relevant implications for gene silencing.
Table 1. The main mechanisms of epigenetic regulation affecting KLF4 expression in tumors. The tumors where the mechanisms have been described are listed and the specific references reported.
The interactions of DNMTs with the enzymes responsible for histone modifications link several players of the epigenetic scenario, namely, CpG methylation, histone lysine/arginine methylation and, overall, influence the ability of KLF4 to bind and regulate target genes. miRNAs, lncRNAs and circRNAs as well can regulate KLF4 expression either directly or by modulating histone modifying enzymes.
The last two paragraphs of the present manuscript deal with the two functions played by the TF KLF4 connected to the chromatin conformation/topology. The former is the pivotal role of KLF4 in the reprogramming and generation of iPSC and the latter is represented by the chromatin conformational changes associated to KLF4 binding. In both cases, the CpG methylation emerges as a recurrent epigenetic tool driving further binding and topological events.
Overall, the epigenetic modulation emerges as a pivotal mechanism for regulating KLF4 functions and therefore represents a potential actionable target.
RF: Conceptualization, Visualization, Writing–original draft.
The author(s) declare that financial support was received for the research, authorship, and/or publication of this article. This study was partially supported by Italian Ministry of Health–Ricerca Corrente Annual Program 2025.
The author is grateful to Silvio Cavuto, Luisa Savoldi and Debora Formisano (AUSL-IRCCS di Reggio Emilia) for their precious support.
The author declares that the research was conducted in the absence of any commercial or financial relationships that could be construed as a potential conflict of interest.
The author(s) declared that they were an editorial board member of Frontiers, at the time of submission. This had no impact on the peer review process and the final decision.
All claims expressed in this article are solely those of the authors and do not necessarily represent those of their affiliated organizations, or those of the publisher, the editors and the reviewers. Any product that may be evaluated in this article, or claim that may be made by its manufacturer, is not guaranteed or endorsed by the publisher.
Bialopiotrowicz, E., Noyszewska-Kania, M., Kachamakova-Trojanowska, N., Loboda, A., Cybulska, M., Grochowska, A., et al. (2020). Serine biosynthesis pathway supports myc-mir-494-ezh2 feed-forward circuit necessary to maintain metabolic and epigenetic reprogramming of burkitt lymphoma cells. Cancers (Basel) 12, 580. doi:10.3390/cancers12030580
Cai, W., Jing, M., Wen, J., Guo, H., and Xue, Z. (2022). Epigenetic alterations of DNA methylation and mirna contribution to lung adenocarcinoma. Front. Genet. 13, 817552. doi:10.3389/fgene.2022.817552
Chapuy, B., McKeown, M. R., Lin, C. Y., Monti, S., Roemer, M. G., Qi, J., et al. (2013). Discovery and characterization of super-enhancer-associated dependencies in diffuse large b cell lymphoma. Cancer Cell 24, 777–790. doi:10.1016/j.ccr.2013.11.003
Chen, E., Li, Q., Wang, H., Yang, F., Min, L., and Yang, J. (2018). Mir-92a promotes tumorigenesis of colorectal cancer, a transcriptomic and functional based study. Biomed. Pharmacother. 106, 1370–1377. doi:10.1016/j.biopha.2018.07.098
Chen, L., Shi, J., Wu, Y., Qiu, R., Zeng, L., Lou, L., et al. (2020). CircRNA CDR1as promotes hepatoblastoma proliferation and stemness by acting as a miR-7-5p sponge to upregulate KLF4 expression. Aging (Albany NY) 12 (19), 19233–19253. doi:10.18632/aging.103748
Chen, T. J., Du, W., Junco, J. J., Bridges, C. S., Shen, Y., Puppi, M., et al. (2021). Inhibition of the map2k7-jnk pathway with 5z-7-oxozeaenol induces apoptosis in t-cell acute lymphoblastic leukemia. Oncotarget 12, 1787–1801. doi:10.18632/oncotarget.28040
Chen, X., Liu, L., Mims, J., Punska, E. C., Williams, K. E., Zhao, W., et al. (2015). Analysis of DNA methylation and gene expression in radiation-resistant head and neck tumors. Epigenetics 10, 545–561. doi:10.1080/15592294.2015.1048953
Cho, Y. G., Song, J. H., Kim, C. J., Nam, S. W., Yoo, N. J., Lee, J. Y., et al. (2007). Genetic and epigenetic analysis of the klf4 gene in gastric cancer. APMIS 115, 802–808. doi:10.1111/j.1600-0463.2007.apm_643.x
Choi, B. J., Cho, Y. G., Song, J. W., Kim, C. J., Kim, S. Y., Nam, S. W., et al. (2006). Altered expression of the klf4 in colorectal cancers. Pathol. Res. Pract. 202, 585–589. doi:10.1016/j.prp.2006.05.001
Damaghi, M., West, J., Robertson-Tessi, M., Xu, L., Ferrall-Fairbanks, M. C., Stewart, P. A., et al. (2021). The harsh microenvironment in early breast cancer selects for a warburg phenotype. Proc. Natl. Acad. Sci. U. S. A. 118, e2011342118. doi:10.1073/pnas.2011342118
Dang, D. T., Pevsner, J., and Yang, V. W. (2000). The biology of the mammalian kruppel-like family of transcription factors. Int. J. Biochem. Cell Biol. 32, 1103–1121. doi:10.1016/s1357-2725(00)00059-5
Di Giammartino, D. C., Kloetgen, A., Polyzos, A., Liu, Y., Kim, D., Murphy, D., et al. (2019). Klf4 is involved in the organization and regulation of pluripotency-associated three-dimensional enhancer networks. Nat. Cell Biol. 21, 1179–1190. doi:10.1038/s41556-019-0390-6
Dost, A. F. M., Moye, A. L., Vedaie, M., Tran, L. M., Fung, E., Heinze, D., et al. (2020). Organoids model transcriptional hallmarks of oncogenic kras activation in lung epithelial progenitor cells. Cell Stem Cell 27, 663–678. doi:10.1016/j.stem.2020.07.022
Du, Z., Wen, X., Wang, Y., Jia, L., Zhang, S., Liu, Y., et al. (2021). Chromatin lncrna platr10 controls stem cell pluripotency by coordinating an intrachromosomal regulatory network. Genome Biol. 22, 233. doi:10.1186/s13059-021-02444-6
Ebrahimi, A., Bakhshaei Shahrebabaki, P., Fouladi, H., and Mansoori Derakhshan, S. (2023). The impact of micrornas on the resistance of breast cancer subtypes to chemotherapy. Pathol. Res. Pract. 249, 154702. doi:10.1016/j.prp.2023.154702
Espada, J., Ballestar, E., Fraga, M. F., Villar-Garea, A., Juarranz, A., Stockert, J. C., et al. (2004). Human DNA methyltransferase 1 is required for maintenance of the histone h3 modification pattern. J. Biol. Chem. 279, 37175–37184. doi:10.1074/jbc.M404842200
Faber, K., Bullinger, L., Ragu, C., Garding, A., Mertens, D., Miller, C., et al. (2013). CDX2-driven leukemogenesis involves KLF4 repression and deregulated PPARγ signaling. J. Clin. Invest 123, 299–314. doi:10.1172/JCI64745
Frazzi, R., Cusenza, V. Y., Pistoni, M., Canovi, L., Cascione, L., Bertoni, F., et al. (2022). Klf4, dapk1 and spg20 promoter methylation is not affected by dnmt1 silencing and hypomethylating drugs in lymphoma cells. Oncol. Rep. 47, 10. doi:10.3892/or.2021.8221
Frazzi, R., Zanetti, E., Pistoni, M., Tamagnini, I., Valli, R., Braglia, L., et al. (2017). Methylation changes of sirt1, klf4, dapk1 and spg20 in b-lymphocytes derived from follicular and diffuse large b-cell lymphoma. Leuk. Res. 57, 89–96. doi:10.1016/j.leukres.2017.02.012
Ghaleb, A. M., and Yang, V. W. (2017). Kruppel-like factor 4 (klf4): what we currently know. Gene 611, 27–37. doi:10.1016/j.gene.2017.02.025
Gong, L., Giacomini, M. M., Giacomini, C., Maitland, M. L., Altman, R. B., and Klein, T. E. (2017). Pharmgkb summary: sorafenib pathways. Pharmacogenet Genomics 27, 240–246. doi:10.1097/FPC.0000000000000279
Guan, H., Xie, L., Leithauser, F., Flossbach, L., Moller, P., Wirth, T., et al. (2010). Klf4 is a tumor suppressor in b-cell non-hodgkin lymphoma and in classic hodgkin lymphoma. Blood 116, 1469–1478. doi:10.1182/blood-2009-12-256446
Han, X., Ranganathan, P., Tzimas, C., Weaver, K. L., Jin, K., Astudillo, L., et al. (2017). Notch represses transcription by prc2 recruitment to the ternary complex. Mol. Cancer Res. 15, 1173–1183. doi:10.1158/1541-7786.MCR-17-0241
Houessinon, A., Francois, C., Sauzay, C., Louandre, C., Mongelard, G., Godin, C., et al. (2016). Metallothionein-1 as a biomarker of altered redox metabolism in hepatocellular carcinoma cells exposed to sorafenib. Mol. Cancer 15, 38. doi:10.1186/s12943-016-0526-2
Hsieh, M. H., Chen, Y. T., Chen, Y. T., Lee, Y. H., Lu, J., Chien, C. L., et al. (2017). Parp1 controls klf4-mediated telomerase expression in stem cells and cancer cells. Nucleic Acids Res. 45, 10492–10503. doi:10.1093/nar/gkx683
Huang, Y., Zhang, H., Wang, L., Tang, C., Qin, X., Wu, X., et al. (2020). Jmjd3 acts in tandem with klf4 to facilitate reprogramming to pluripotency. Nat. Commun. 11, 5061. doi:10.1038/s41467-020-18900-z
Huyghe, A., Furlan, G., Schroeder, J., Cascales, E., Trajkova, A., Ruel, M., et al. (2022). Comparative roadmaps of reprogramming and oncogenic transformation identify bcl11b and atoh8 as broad regulators of cellular plasticity. Nat. Cell Biol. 24, 1350–1363. doi:10.1038/s41556-022-00986-w
Ischenko, I., Zhi, J., Moll, U. M., Nemajerova, A., and Petrenko, O. (2013). Direct reprogramming by oncogenic ras and myc. Proc. Natl. Acad. Sci. U.S.A. 110, 3937–3942. doi:10.1073/pnas.1219592110
Jiang, X., Lu, Y., Xie, S., Chen, Y., Liu, X., Li, S., et al. (2023). Mir-624 accelerates the growth of liver cancer cells by inhibiting emc3. Noncoding RNA Res. 8, 641–644. doi:10.1016/j.ncrna.2023.09.005
Jin, B., Li, Y., and Robertson, K. D. (2011). DNA methylation: superior or subordinate in the epigenetic hierarchy?. Genes Cancer 2, 607–617. doi:10.1177/1947601910393957
Jung, Y., Kim, J. K., Shiozawa, Y., Wang, J., Mishra, A., Joseph, J., et al. (2013). Recruitment of mesenchymal stem cells into prostate tumours promotes metastasis. Nat. Commun. 4, 1795. doi:10.1038/ncomms2766
Kalra, I. S., Alam, M. M., Choudhary, P. K., and Pace, B. S. (2011). Kruppel-like factor 4 activates hbg gene expression in primary erythroid cells. Br. J. Haematol. 154, 248–259. doi:10.1111/j.1365-2141.2011.08710.x
Khan, H. N., Perlee, D., Schoenmaker, L., van der Meer, A. J., Franitza, M., Toliat, M. R., et al. (2019). Leukocyte transcriptional signatures dependent on lps dosage in human endotoxemia. J. Leukoc. Biol. 106, 1153–1160. doi:10.1002/JLB.4A0219-050R
Lee, E., Wang, J., Yumoto, K., Jung, Y., Cackowski, F. C., Decker, A. M., et al. (2016). Dnmt1 regulates epithelial-mesenchymal transition and cancer stem cells, which promotes prostate cancer metastasis. Neoplasia 18, 553–566. doi:10.1016/j.neo.2016.07.007
Li, H., Wang, J., Xiao, W., Xia, D., Lang, B., Wang, T., et al. (2014). Epigenetic inactivation of klf4 is associated with urothelial cancer progression and early recurrence. J. Urol. 191, 493–501. doi:10.1016/j.juro.2013.08.087
Li, H., Wang, J., Xiao, W., Xia, D., Lang, B., Yu, G., et al. (2013). Epigenetic alterations of kruppel-like factor 4 and its tumor suppressor function in renal cell carcinoma. Carcinogenesis 34, 2262–2270. doi:10.1093/carcin/bgt189
Li, W., Guo, L., Tang, W., Ma, Y., Wang, X., Shao, Y., et al. (2021). Identification of DNA methylation biomarkers for risk of liver metastasis in early-stage colorectal cancer. Clin. Epigenetics 13, 126. doi:10.1186/s13148-021-01108-3
Li, W., Liu, M., Su, Y., Zhou, X., Liu, Y., and Zhang, X. (2015). The janus-faced roles of kruppel-like factor 4 in oral squamous cell carcinoma cells. Oncotarget 6, 44480–44494. doi:10.18632/oncotarget.6256
Lu, M., Wu, Y., Zeng, B., Sun, J., Li, Y., Luo, J., et al. (2020). Circehmt1 inhibits metastatic potential of breast cancer cells by modulating mir-1233-3p/klf4/mmp2 axis. Biochem. Biophys. Res. Commun. 526, 306–313. doi:10.1016/j.bbrc.2020.03.084
Mani, S. A., Guo, W., Liao, M. J., Eaton, E. N., Ayyanan, A., Zhou, A. Y., et al. (2008). The epithelial-mesenchymal transition generates cells with properties of stem cells. Cell 133, 704–715. doi:10.1016/j.cell.2008.03.027
Marjanovic, N. D., Hofree, M., Chan, J. E., Canner, D., Wu, K., Trakala, M., et al. (2020). Emergence of a high-plasticity cell state during lung cancer evolution. Cancer Cell 38, 229–246. doi:10.1016/j.ccell.2020.06.012
Miyoshi, N., Ishii, H., Nagai, K., Hoshino, H., Mimori, K., Tanaka, F., et al. (2010). Defined factors induce reprogramming of gastrointestinal cancer cells. Proc. Natl. Acad. Sci. U. S. A. 107, 40–45. doi:10.1073/pnas.0912407107
Montecillo-Aguado, M., Morales-Martinez, M., Huerta-Yepez, S., and Vega, M. I. (2021). Klf4 inhibition by kenpaullone induces cytotoxicity and chemo sensitization in b-nhl cell lines via yy1 independent. Leuk. Lymphoma 62, 1422–1431. doi:10.1080/10428194.2020.1869960
Morales-Martinez, M., Valencia-Hipolito, A., Vega, G. G., Neri, N., Nambo, M. J., Alvarado, I., et al. (2019). Regulation of kruppel-like factor 4 (klf4) expression through the transcription factor yin-yang 1 (yy1) in non-hodgkin b-cell lymphoma. Oncotarget 10, 2173–2188. doi:10.18632/oncotarget.26745
Morales-Martinez, M., Vega, G. G., Neri, N., Nambo, M. J., Alvarado, I., Cuadra, I., et al. (2020). Microrna-7 regulates migration and chemoresistance in non-hodgkin lymphoma cells through regulation of klf4 and yy1. Front. Oncol. 10, 588893. doi:10.3389/fonc.2020.588893
Nakahara, Y., Northcott, P. A., Li, M., Kongkham, P. N., Smith, C., Yan, H., et al. (2010). Genetic and epigenetic inactivation of kruppel-like factor 4 in medulloblastoma. Neoplasia 12, 20–27. doi:10.1593/neo.91122
NCBI (2023). Genome data viewer. Available at: https://www.ncbi.nlm.nih.gov/genome/gdv/browser/genome/?id=GCF_000001405.40 (Accessed January 22, 2024).
Ogawa, H., Wu, X., Kawamoto, K., Nishida, N., Konno, M., Koseki, J., et al. (2015). Micrornas induce epigenetic reprogramming and suppress malignant phenotypes of human colon cancer cells. PLoS One 10, e0127119. doi:10.1371/journal.pone.0127119
Oyinlade, O., Wei, S., Kammers, K., Liu, S., Wang, S., Ma, D., et al. (2018). Analysis of klf4 regulated genes in cancer cells reveals a role of DNA methylation in promoter-enhancer interactions. Epigenetics 13, 751–768. doi:10.1080/15592294.2018.1504592
Patra, S. K., Deb, M., and Patra, A. (2011). Molecular marks for epigenetic identification of developmental and cancer stem cells. Clin. Epigenetics 2, 27–53. doi:10.1007/s13148-010-0016-0
Sardina, J. L., Collombet, S., Tian, T. V., Gomez, A., Di Stefano, B., Berenguer, C., et al. (2018). Transcription factors drive tet2-mediated enhancer demethylation to reprogram cell fate. Cell Stem Cell 23, 905–906. doi:10.1016/j.stem.2018.11.001
Schagdarsurengin, U., Lammert, A., Schunk, N., Sheridan, D., Gattenloehner, S., Steger, K., et al. (2017). Impairment of igf2 gene expression in prostate cancer is triggered by epigenetic dysregulation of igf2-dmr0 and its interaction with klf4. Cell Commun. Signal 15, 40. doi:10.1186/s12964-017-0197-7
Sethi, I., Gluck, C., Zhou, H., Buck, M. J., and Sinha, S. (2017). Evolutionary re-wiring of p63 and the epigenomic regulatory landscape in keratinocytes and its potential implications on species-specific gene expression and phenotypes. Nucleic Acids Res. 45, 8208–8224. doi:10.1093/nar/gkx416
Shen, T., Han, M., Wei, G., and Ni, T. (2015). An intriguing rna species--perspectives of circularized rna. Protein Cell 6, 871–880. doi:10.1007/s13238-015-0202-0
Shen, Y., Park, C. S., Suppipat, K., Mistretta, T. A., Puppi, M., Horton, T. M., et al. (2017). Inactivation of klf4 promotes t-cell acute lymphoblastic leukemia and activates the map2k7 pathway. Leukemia 31, 1314–1324. doi:10.1038/leu.2016.339
Shields, J. M., and Yang, V. W. (1997). Two potent nuclear localization signals in the gut-enriched kruppel-like factor define a subfamily of closely related kruppel proteins. J. Biol. Chem. 272, 18504–18507. doi:10.1074/jbc.272.29.18504
Szczepanek, J., and Tretyn, A. (2023). Microrna-mediated regulation of histone-modifying enzymes in cancer: mechanisms and therapeutic implications. Biomolecules 13, 1590. doi:10.3390/biom13111590
Tsompana, M., Gluck, C., Sethi, I., Joshi, I., Bard, J., Nowak, N. J., et al. (2020). Reactivation of super-enhancers by klf4 in human head and neck squamous cell carcinoma. Oncogene 39, 262–277. doi:10.1038/s41388-019-0990-4
Vidal, A. F., Cruz, A. M., Magalhaes, L., Pereira, A. L., Anaissi, A. K., Alves, N. C., et al. (2016). Hsa-mir-29c and hsa-mir-135b differential expression as potential biomarker of gastric carcinogenesis. World J. Gastroenterol. 22, 2060–2070. doi:10.3748/wjg.v22.i6.2060
Walter, K., Rodriguez-Aznar, E., Ferreira, M. S. V., Frappart, P. O., Dittrich, T., Tiwary, K., et al. (2021). Telomerase and pluripotency factors jointly regulate stemness in pancreatic cancer stem cells. Cancers (Basel) 13, 3145. doi:10.3390/cancers13133145
Wan, J., Su, Y., Song, Q., Tung, B., Oyinlade, O., Liu, S., et al. (2017). Methylated cis-regulatory elements mediate klf4-dependent gene transactivation and cell migration. Elife 6, e20068. doi:10.7554/eLife.20068
Wang, X. Q., Ng, R. K., Ming, X., Zhang, W., Chen, L., Chu, A. C., et al. (2013). Epigenetic regulation of pluripotent genes mediates stem cell features in human hepatocellular carcinoma and cancer cell lines. PLoS One 8, e72435. doi:10.1371/journal.pone.0072435
Wei, T., Lin, R., Fu, X., Lu, Y., Zhang, W., Li, Z., et al. (2022). Epigenetic regulation of the dnmt1/mt1g/klf4/ca9 axis synergises the anticancer effects of sorafenib in hepatocellular carcinoma. Pharmacol. Res. 180, 106244. doi:10.1016/j.phrs.2022.106244
Wei, Z., Gao, F., Kim, S., Yang, H., Lyu, J., An, W., et al. (2013). Klf4 organizes long-range chromosomal interactions with the oct4 locus in reprogramming and pluripotency. Cell Stem Cell 13, 36–47. doi:10.1016/j.stem.2013.05.010
Xie, V. K., Li, Z., Yan, Y., Jia, Z., Zuo, X., Ju, Z., et al. (2017). DNA-methyltransferase 1 induces dedifferentiation of pancreatic cancer cells through silencing of kruppel-like factor 4 expression. Clin. Cancer Res. 23, 5585–5597. doi:10.1158/1078-0432.CCR-17-0387
Xu, J., Lu, B., Xu, F., Gu, H., Fang, Y., Huang, Q., et al. (2008). Dynamic down-regulation of kruppel-like factor 4 in colorectal adenoma-carcinoma sequence. J. Cancer Res. Clin. Oncol. 134, 891–898. doi:10.1007/s00432-008-0353-y
Yang, C. M., Chiba, T., Brill, B., Delis, N., von Manstein, V., Vafaizadeh, V., et al. (2015). Expression of the mir-302/367 cluster in glioblastoma cells suppresses tumorigenic gene expression patterns and abolishes transformation related phenotypes. Int. J. Cancer 137, 2296–2309. doi:10.1002/ijc.29606
Yang, Y., and Katz, J. P. (2016). Klf4 is downregulated but not mutated during human esophageal squamous cell carcinogenesis and has tumor stage-specific functions. Cancer Biol. Ther. 17, 422–429. doi:10.1080/15384047.2016.1156260
Yu, T., Chen, X., Zhang, W., Liu, J., Avdiushko, R., Napier, D. L., et al. (2016). Klf4 regulates adult lung tumor-initiating cells and represses k-ras-mediated lung cancer. Cell Death Differ. 23, 207–215. doi:10.1038/cdd.2015.85
Zeng, J. D., Zhang, N., Zhao, G. J., Xu, L. X., Yang, Y., Xu, X. Y., et al. (2018). Mt1g is silenced by DNA methylation and contributes to the pathogenesis of hepatocellular carcinoma. J. Cancer 9, 2807–2816. doi:10.7150/jca.25680
Keywords: KLF4, epigenetic regulation, DNA methylation, histone marks, tumor suppressor
Citation: Frazzi R (2024) KLF4 is an epigenetically modulated, context-dependent tumor suppressor. Front. Cell Dev. Biol. 12:1392391. doi: 10.3389/fcell.2024.1392391
Received: 27 February 2024; Accepted: 01 July 2024;
Published: 29 July 2024.
Edited by:
Yong Tao, Yunnan University, ChinaReviewed by:
François Hermetet, INSERM U1231 Lipides, Nutrition, Cancer (LNC), FranceCopyright © 2024 Frazzi. This is an open-access article distributed under the terms of the Creative Commons Attribution License (CC BY). The use, distribution or reproduction in other forums is permitted, provided the original author(s) and the copyright owner(s) are credited and that the original publication in this journal is cited, in accordance with accepted academic practice. No use, distribution or reproduction is permitted which does not comply with these terms.
*Correspondence: Raffaele Frazzi, cmFmZmFlbGUuZnJhenppQGF1c2wucmUuaXQ=
Disclaimer: All claims expressed in this article are solely those of the authors and do not necessarily represent those of their affiliated organizations, or those of the publisher, the editors and the reviewers. Any product that may be evaluated in this article or claim that may be made by its manufacturer is not guaranteed or endorsed by the publisher.
Research integrity at Frontiers
Learn more about the work of our research integrity team to safeguard the quality of each article we publish.