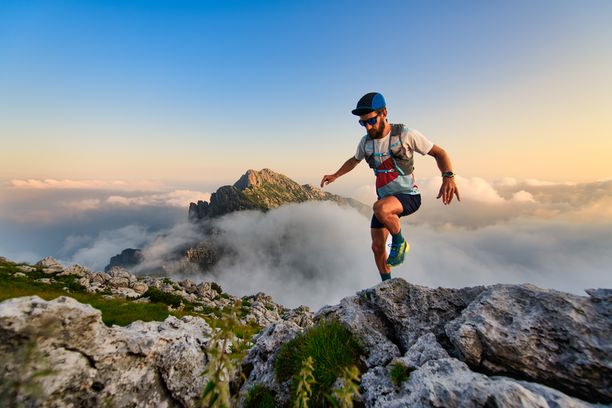
94% of researchers rate our articles as excellent or good
Learn more about the work of our research integrity team to safeguard the quality of each article we publish.
Find out more
REVIEW article
Front. Cell Dev. Biol., 08 February 2023
Sec. Molecular and Cellular Pathology
Volume 11 - 2023 | https://doi.org/10.3389/fcell.2023.1090386
Stress urinary incontinence (SUI) is very common in women. It affects patients’ mental and physical health, and imposed huge socioeconomic pressure. The therapeutic effect of conservative treatment is limited, and depends heavily on patient persistence and compliance. Surgical treatment often brings procedure-related adverse complications and higher costs for patients. Therefore, it is necessary to better understand the potential molecular mechanisms underlying stress urinary incontinence and develop new treatment methods. Although some progress has been made in the basic research in recent years, the specific molecular pathogenic mechanisms of SUI are still unclear. Here, we reviewed the published studies on the molecular mechanisms associated with nerves, urethral muscles, periurethral connective tissue and hormones in the pathogenesis of SUI. In addition, we provide an update on the recent progresses in research on the use of cell therapy for treating SUI, including research on stem cells therapy, exosome differentiation and gene regulation.
Stress urinary incontinence (SUI) is the most common type of urinary incontinence and is defined as the involuntary leakage of urine when bladder pressure exceeds urethral closure pressure due to physical exertion, exercise, coughing or sneezing (D'Ancona et al., 2019). The prevalence of SUI worldwide ranges widely from 10% to 70%, owing to different survey methodologies and study populations (Capobianco et al., 2018). In the United States, the prevalence of SUI in 2017–2020 was 46% among adult women and 31% among adult men with prior diagnoses of prostate and bladder cancer (Abufaraj et al., 2021; Cao et al., 2022). In China, the prevalence of SUI in women aged 20 years and above reached 18.9%, and SUI affected over one-third of the perimenopausal women (Li T. et al., 2019). The prevalence of SUI increases with age, and the peak incidence is in postmenopausal and pregnant women. Several other factors are related to SUI risk, including delivery, menopause, strenuous physical work, smoking, obesity and chronic cough (Tan et al., 2018).
Although SUI is not a life-threatening disease, it results in physical, social, and psychological adverse consequences, and leads to low self-esteem, impaired quality of life and social isolation for patients. Untreated SUI is also associated with falls and fractures, sleep disturbances, depression, and urinary tract infections (Lukacz et al., 2017). In addition, SUI contributes to a significant financial burden to the health system, with direct costs exceeding $12 billion in the United States alone (Chong et al., 2011). As the world’s population ages, the prevalence and management costs of SUI will increase in the next few decades.
There are two main mechanisms of SUI. The first is urethral hypermobility, in which the urethra and bladder neck fail to close adequately when abdominal pressure increases due to the loss of supportive structures of the pelvic floor, resulting in the leakage of urine. The second is intrinsic urethral sphincter deficiency, which results in poor urethral closure due to the loss of the urethral mucosa and muscle tone (Wu, 2021). However, the molecular pathogenic mechanisms in SUI are still unclear. In this review, we mainly summarized the recent progresses in research on the molecular mechanisms of SUI pathogenesis and the use of cell therapy in SUI treatment.
The lower urinary tract, composed of the bladder and urethra, is responsible for the excretion and storage of urine. The parasympathetic nerve stimulates the bladder detrusor muscle, mediated by muscarinic receptors (M2, M3) being activated by acetylcholine (ACh). The sympathetic nerve stimulates urethral smooth muscle and inhibits bladder detrusor, mediated by α1-adrenergic and β3-adrenergic receptors, respectively. The somatic pudendal nerve stimulates striated muscle of the external urethral sphincter (EUS), mediated by ACh activating N receptors (Yoshimura and Miyazato, 2012). During bladder filling, the parasympathetic innervation of the detrusor is inhibited, and the urethral smooth and striated sphincter are activated. When urinating, multiple parts of the brain are activated and generate a series of signals that ultimately lead to the activation of the pontine micturition center, which activates the sacral parasympathetic nerves, leading to bladder contraction, urethra relaxation and urine outflow (Fowler, 2006) (Figure 1). If a nerve in any one of these three systems is damaged by trauma, childbirth, or iatrogenic causes, it results in symptoms of SUI (Balog et al., 2019).
FIGURE 1. Neuroregulation of micturition and neurogenic factors affecting SUI. The parasympathetic nerve stimulates the bladder detrusor muscle, mediated by muscarinic receptors (M2, M3) being activated by ACh. The sympathetic nerve stimulates urethral smooth muscle and inhibits bladder detrusor, mediated by α1-adrenergic and β3-adrenergic receptors, respectively. The somatic pudendal nerve stimulates striated muscle of the external urethral sphincter, mediated by ACh activating N receptors. BDNF binds to Trk receptors and p75NTR receptors to facilitate regeneration of pudendal nerve, but impair NMJ formation and myogenic myoblast differentiation and maturation. ACh: acetylcholine; EUS: external urethral sphincter; IUS: internal urethral sphincter; SUI: stress urinary incontinence; PMC: pontine micturition center; BDNF: brain-derived neurotrophic factor; Trk: tropomyosin receptor kinase; p75NTR: pan neurotrophin receptors; NMJ: neuromuscular junctions.
SUI mainly occurs when the intravesical pressure exceeds the urethral pressure during stress moments. Passive as well as active urethral closure mechanisms are important for continence. Passive closure mechanisms can physiologically only occur on the proximal urethra, and the neurally mediated active closure mechanisms on the distal two-thirds of the urethra play an important role in the continence (de Vries et al., 2018). However, the active urethral closure mechanism is damaged in SUI patients as evidenced by a series of studies. These mechanisms include lower cough-induced leak point pressure (LPP), disappearance of the pressure increment in the urethra preceding cough, and a loss of voluntary increases in urethral pressure (Yoshimura et al., 2008). In addition, the urethral closure mechanism activated by a sudden increase in abdominal pressure is mediated by the somatic nerves and is under central nervous control via Onuf’s nucleus (Kamo et al., 2003). Normal people activate the pudendal nerve (PN) during sneezing, stimulate the contraction of urethral muscle and avoid of incontinence. While the active urethral continence reflex induced by sneezing may be impaired in women with SUI, due to the PN damage caused by childbirth (Kamo et al., 2006).
Neurotrophins (nerve growth factor (NGF), brain-derived neurotrophic factor (BDNF), neurotrophin-3 (NT-3), and neurotrophin-4 (NT-4)), mainly produced by Schwann cells, are widely recognized for their roles in promoting cell growth, survival and differentiation in several classes of neurons (Chang et al., 2019; Gordon, 2020). Neurotrophins bind to two main classes of receptors-tropomyosin receptor kinase (Trk) receptors and pan neurotrophin (p75NTR) receptors. The p75NTR receptors belongs to the tumor necrosis factor receptor family. They can bind to all neurotrophins and participate in apoptosis (Song et al., 2014). Individual neurotrophins bind specifically to Trk receptors, causing receptor dimerization and autophosphorylation of tyrosine residues. This lead to the activation of downstream signaling cascades including phosphatidylinositol 3-kinase (PI3K)/protein kinase B (Akt), Ras-mitogen-activated protein kinase (Ras-MAPK), and phospholipase CÎł (PLCÎł). These pathways have known roles in neuronal survival, axonal outgrowth, and synaptic plasticity (Song et al., 2014).
Clinical studies have shown that serum BDNF levels are significantly reduced in the 30th to 37th weeks of pregnancy and remain low for 2–3 months postpartum (Lommatzsch et al., 2006; Vega et al., 2011). The specific mechanism leading to this phenomenon is still unclear. After peripheral nerve injury, BDNF is upregulated in innervated target organs and axons distal to injury sites (Omura et al., 2005), and the use of BDNF has shown promising results in improving functional and anatomic recovery (Simon et al., 2003). Some studies have shown that BDNF expression increases in the EUS 1 day after pudendal nerve crush (PNC) in rats, promoting pudendal neuroregeneration via the retrograde transport of BDNF from the EUS to its innervating motor neuron cell bodies within Onuf’s nucleus (Pan et al., 2009). However, BDNF levels in the EUS are decreased in an animal model of SUI that simulates childbirth injury. Despite facilitating the regeneration of PNs, BDNF is detrimental to muscular recovery and development since it impairs neuromuscular junction (NMJ) formation as well as myogenic myoblast differentiation and maturation (Mousavi and Jasmin, 2006). The concurrent injury of the EUS muscle and the PN during childbirth results in the downregulation of BDNF in the EUS. This enables sphincter muscle and NMJ repair but likely impairs the PN neuroregenerative response. Direct application of BDNF to the pudendal nerve accelerates continence recovery through enhancing pudendal nerve recovery and facilitating the recovery of EUS anatomy and function in vaginal distension (VD) and PNC models (Gill et al., 2013). Electrical stimulation has been shown to upregulate the expression of BDNF in motor neurons and facilitate axon regeneration through an increase in βII-tubulin expression after injury (Jiang et al., 2013).
The excitatory pathway responsible for maintaining smooth muscle contraction in the urethra and bladder neck originates from sympathetic preganglionic neurons (Anderson, 1993). Pharmacological experiments have shown that the bladder sympathetic reflex pathway is regulated by the central noradrenergic system (Danuser et al., 1995). Norepinephrine (NE) stimulates urethral and bladder neck smooth muscle contraction via α1-adrenoceptors, and causes detrusor relaxation via β2-adrenoceptors or, most predominantly, via β3-adrenoceptors (Nomiya and Yamaguchi, 2003). Sympathetic activity can be inhibited by α 1-adrenergic receptor blockers or α 2-adrenergic receptor (AR) agonists. In addition, serotonin and norepinephrine can enhance pudendal nerve activity through glutamate release in Onuf’s nucleus, resulting in increased EUS tone. Serotonin also regulates collagen synthesis by activating transforming growth factor-β1 (TGF-β1). TGF-β1 is a key cytokine in extracellular matrix production, cell proliferation and fibrosis (Song et al., 2016). These neurotransmitters are being investigated as potential targets for the treatment of SUI.
Duloxetine, a dual 5-hydroxytryptamine (HT) and norepinephrine reuptake inhibitor, can stimulate pudendal motor neurons and increase EUS and pelvic floor muscle contractility (Dhaliwal et al., 2022). Duloxetine can reduce the frequency of incontinence episodes in SUI compared to placebo (Norton et al., 2002; Millard et al., 2004; van Kerrebroeck et al., 2004), and is the first drug in the world to be approved for the treatment of women with moderate-to-severe SUI. A study showed that the α2- AR blocker imidazoxan could enhance the effect of duloxetine on the sneeze-induced urethral continence reflex in rats, and this combination therapy may be an effective new treatment for SUI (Norton et al., 2002). Studies on 5-HT receptors showed that intrathecal administration of 8-hydroxy-2-(di-n-propylamino)-tetralin, a 5HT-1A agonist, reduced the urethral constriction response during sneezing by 48.9%, whereas when 5HT-2B/2C was administered, the agonist meta-chlorophenylpiperazine (mCPP) increased this response (Miyazato et al., 2009). These findings suggest that 5HT-2C activation of the receptor could actively enhance urethral closure during sneezing. In addition to duloxetine, imipramine, which is a norepinephrine and serotonin reuptake inhibitor, is thought to improve urethral smooth muscle contraction for the treatment of SUI or mixed urinary incontinence (Cipullo et al., 2014). Moreover, venlafaxine (0.1–10 mg/kg) and S-norfluoxetine (0.01–10 mg/kg) have also been shown to increase bladder volume, but they have not shown a clear therapeutic effect on SUI. Clenbuterol hydrochloride, a beta-AR agonist, increases periurethral striated muscle contraction by releasing acetylcholine at the NMJ and has been shown to be effective in the treatment of SUI (Alhasso et al., 2005). However, there have been few related studies, and this agonist has not been widely used. Drug development targeting 5-HT and adrenergic mechanisms requires further research.
The SUI level depends on the urethra’s ability of the urethra to maintain a strong urethral closure pressure during intra-abdominal pressure fluctuations. For females, the urethra is short and straight, and the urethral closure pressure could be impaired due to factors such as childbirth. However, the male urethra is divided into three parts, which are long and curved, and the size of the prostate gland increases with age, making males more prone to dysuria rather than incontinence. In male patients, SUI usually results from neurovascular bundle injury during radical prostatectomy or external urethral sphincter injury during transurethral resection or enucleation of the prostate (Koch and Kaufman, 2022). The concerted work of the urethral striated muscle, the urethral smooth muscles, and the vascular elements within the submucosa of the urethra ensure urethral sphincter closure and maintain continence. Some moleculars play a crucial role in maintaining urethral muscles growth, contraction and capacity (Figure 2). A damaged sphincteric unit or support system is all potential causes of an insufficient closure mechanism (Mistry et al., 2020).
FIGURE 2. Molecules affecting urethral muscles in SUI. Vitamin D plays an important role in regulating muscle strength and function, and low serum vitamin D levels are associated with an increased risk of SUI; ANO1 is essential for maintaining the spontaneous tone of urethral smooth muscle; MSTN negatively regulated human urethral RS satellite cells proliferation and differentiation and TNF-α decreased the number of human urethral RS cells. EUS: external urethral sphincter; IUS: internal urethral sphincter; MSTN: myostatin; TNF-α: tumor necrosis factor-α; ANO1:anoctamin-1; SUI:stress urinary incontinence; RS: rhabdosphincter.
Myostatin (MSTN), which is also known as growth differentiation factor 8 (GDF-8), is a member of the TGF-β superfamily. MSTN plays a role in the control and maintenance of skeletal muscle mass (McPherron et al., 1997). Certain studies have suggested that MSTN negatively regulates human urethral rhabdosphincter satellite cell proliferation and differentiation. Activation of its downstream targets, such as Smad2/3, inhibits myogenesis and promots fibrosis (Akita et al., 2013). Downregulation of MSTN by clustered regularly interspaced short palindromic repeats interference/deactivated Cas9 nuclease-null-kruppel associated box (CRISPRi/dCas9-KRAB)-mediated gene silencing in L6 rat myoblasts and in Zucker rats could significantly enhanced myogenesis in vitro, and significant increased the leak point pressure, the thickness of the urethral striated muscle layer, the ratio of urethral striated muscle to smooth muscle, and the number of NMJs in the SUI rat model (Yuan et al., 2020).
Tumor necrosis factor-α (TNF-α) induced apoptosis in murine skeletal muscle cells (Stewart et al., 2004), and systemic concentration of TNF-α increase with age (Krabbe et al., 2004). TNF-α might be involved in age-related decreases in the number of human RS cells and be a causative factor of SUI in the elderly population. Previous studies have reported that TNF-α inhibits the proliferation of striated muscle progenitor cells from the human urethral rhabdosphincter (RS), and a TNF-α antagonist inhibited these effects on these cells (Greiwe et al., 2001; Hanada et al., 2010). In addition, TNF-α was shown to inhibit the myogenic differentiation of human RS progenitor cells through the PI3K and p38-mitogen activated protein-kinase (MAPK) pathways in a dose-dependent manner. Based on this, the research group established an immortalized human RS cell line to identify a possible new therapy for SUI through autologous transplantation of muscle or adipose-derived stem cells (Shinohara et al., 2017).
Anoctamin-1 (ANO1), which is also known as transmembrane protein 16A (TMEM16A), has been shown to be a Ca2+-activated Cl-channel. ANO1 is essential for a variety of physiological functions, including smooth muscle contraction (Ji et al., 2019). Feng et al. showed that the ryanodine receptor (RyR)-ClCa-voltage-dependent calcium channel (VDCC) signaling system plays a crucial role in maintaining the spontaneous tone (STT) of the female urethral smooth muscles (USM) in mice and female urethra, and the STT of the female urethra is a key factor in maintaining the tone and contraction of the female urethra (Feng et al., 2019). Caffeine, which is a specific ryanodine receptor (RyR) agonist, can increase urethral contractility and intracellular Ca2+ concentrations in female mice compared with male mice. Furthermore, EACT, an ANO1 activator, can cause higher intracellular Ca2+ increases and stronger currents in female mice than in male mice (Chen et al., 2020). In addition, the urinary retention time was increased, and the urine output was decreased in smooth muscle cell-specific TMEM16A-knockout (TMEM16A smKO) mice (Feng et al., 2019).
Vitamin D plays an important role in regulating calcium and bone homeostasis; it can affect muscle strength and function, and low serum vitamin D levels are associated with decreased muscle mass, strength, and performance in older adults (Holick, 2003; Ceglia and Harris, 2013). Evidence suggests that vitamin D primarily affects the diameter and number of type II (fast-twitch) muscle fibers, and myopathies are mainly caused by type IIA muscle fiber atrophy. Type II fibers primarily generate energy under anaerobic conditions for rapid and forceful contractions; therefore, during activities with increased intra-abdominal pressure, atrophy of fast type II fibers may hinder effective urethral closure, leading to SUI (Marques et al., 2010). Studies have shown that in non-pregnant or pregnant women, low vitamin D levels are associated with an increased risk of SUI and there is a positive relationship between prenatal vitamin D levels and postpartum pelvic floor muscle strength and endurance (Badalian and Rosenbaum, 2010; Aydogmus et al., 2015; Navaneethan et al., 2015).
Currently, common theories regarding the pathogenesis of SUI include the integral theory and the Hammock hypothesis, which both stress that structural and functional defects in the supportive tissues of the urethra, including the anterior vaginal wall, contribute to the development of SUI (DeLancey, 1994; Farnsworth, 2002). Effective closure of the female urethra under stress is related to the combined action of various anatomical structures surrounding the urethra, including the connective tissue, pelvic fascia, ligaments and anterior vaginal wall (Delancey and Ashton-Miller, 2004). Connective tissue, which is an essential part, plays an important role in the continence mechanism (Ulmsten and Falconer, 1999). The mechanical stability of the genitourinary tract depends on intact, functional connective tissue to support the bladder neck, urethra and pelvic organs. When connective tissue function is defective, it may lead to the relaxation of the structures involved in the closure of the urethra, making it difficult to close the urethra, and leading to the symptoms of SUI (de Vries et al., 2018). The main constituent in the ligaments and suburethral wall is fibrous connective tissue contains collagen and elastic fibers, proteoglycans and glycoproteins forming a viscoelastic matrix, the extracellular matrix (ECM) (Piculo et al., 2014) (Figure 3). A study showed that there were differences in connective tissue in women with pelvic floor dysfunction, with changes in collagen content and enzymes involved in degradation in pelvic ligament tissue (Chen and Yeh, 2011).
FIGURE 3. Molecules associated with connective tissue defect in SUI. Connective tissue, mainly contains collagen and elastic fibers, plays an important role in the continence mechanism. In the connective tissue of patients with SUI, collagen and elastic fibers were significantly reduced and damaged. MMP-1, TIMP-1, calpain-2, AQP2, miR-34a and ERK1/2 signaling pathway regulated the metabolism of collagen, and neutrophil elastase, α-1 antitrypsin, LOXL1, fibrillin-1 and TNF-β1/Smad regulated the metabolism of elastic fibers. EUS: external urethral sphincter; IUS: internal urethral sphincter; SUI: stress urinary incontinence; MMPs: matrix metalloproteinases; TIMPs: tissue inhibitors of metalloproteinases; AQPs: aquaporins; LOXL1:lysyl oxidase like-1; TNF-β1:Tumor necrosis factor-β1.
Collagen forms the matrix of connective tissue and is synthesized by very complex posttranslational processing (Chen et al., 2002). Collagen types I, III and V are the major structural components of the vaginal epithelium and pelvic inner fascia. Type I collagen provides tissue strength, type III collagen helps maintain elasticity and the function of type V collagen is unknown. In the urethral support tissue of patients with SUI, the collagen content was significantly reduced. This finding has important implications in studying the underlying mechanisms of SUI (Song et al., 2007; Sangsawang, 2014). Continuous tissue remodeling makes the relationship between the production and degradation of collagen essential for maintaining tensile strength. Collagen degradation depends on the activity of matrix metalloproteinases (MMPs), a family of structurally related proteins that degrade extracellular matrix and basement membrane components. Collagens are cleaved by the MMP-1, MMP-8 and MMP-13. This process yields two fragments, and these fragments are highly susceptible to MMP-2 and MMP-9 degradation to amino acids. In turn, the activity of MMPs is regulated by the tissue inhibitors of metalloproteinases (TIMPs) (Chen and Yeh, 2011). The balance between MMPs and TIMPs determines collagen breakdown. Women with SUI had an increased ratio of MMP-1 to TIMP-1 mRNA expression and MMP-1 protein expression, but decreased TIMP-1 protein expression in vaginal wall tissue compared with controls (Chen et al., 2002).
Calpain-2 is a neutral protease commonly found in human tissues that is activated by elevated intracellular Ca2+ concentrations. It is associated with the degradation of various cytoskeletal proteins and plays an important role in the degradation of myofibers, elastic fibers, and collagen (Li et al., 2017). Compared with those in the controls, the mRNA and protein expression levels of calpain-2 were increased in the urethral tissue of SUI patients (Wu et al., 2010), suggesting that calpain-2 may play a role in the pathogenesis of SUI. Further studies showed that the expression of MMP-1 and collagen I could be regulated by calpain-2 and mediated by miR-93. When miR-93 was overexpressed in SUI, primary fibroblasts restored calpain-2 expression. This finding suggests that calpain-2 is negatively correlated with collagen expression and indicates that miR-93 may mediate the pathogenic mechanism of SUI through calpain-2 (Yang et al., 2018). In addition, a study on the mechanism of electrical stimulation in the treatment of SUI showed that the intracellular Ca2+ concentration was markedly enhanced by electrical stimulation. This leads to the activation of the calpain 2/talin 1/integrin β1/transforming growth factor (TGF)-β1 axis, which suppresses cell apoptosis and upregulate collagen (Li Y. et al., 2019). Zhang et al. found that aquaporins (AQPs) are involved in the pathogenesis of female SUI through collagen metabolism during ECM remodeling. It is family of transmembrane proteins, and mediators of transcellular water flow and play important roles in maintaining intra/extracellular fluid homeostasis. The expression of AQP2 in the anterior vaginal wall of women without SUI was significantly increased compared with that of women with SUI. Downregulating AQP2 significantly decreased the mRNA and protein expression of collagen I/III, and overexpressing AQP2 significantly increased the mRNA and protein expression of collagen I/III in fibroblasts (Zhang et al., 2017). A recent study illustrated poor miR-34a expression in SUI patients, miR-34a upregulation inhibited Nampt transcription to accelerate autophagy to suppress ECM degradation (increased collagen and TIMP-1; decreased MMP-2 and MMP-9), thus attenuating SUI (Zhou et al., 2022).
As one of the important MAPK pathways, the extracellular signal-related kinases 1 and 2 (ERK1/2) signaling pathway has been reported to regulate the growth of fibroblasts and collagen at multiple sites (Bhogal and Bona, 2008; Jeon et al., 2009; Choe et al., 2010). Studies have shown that compared with that in the control group, the level of p-ERK1/2 was decreased in female SUI; inhibiting the ERK1/2 signaling pathway can inhibit the synthesis of type I and type III collagen in vaginal wall fibroblasts (Huang et al., 2013). Recent studies also found that the inhibition of the TGF-β1/Smad3 and Nuclear factor-erythroid 2 p45-related factor 2(Nrf2)/antioxidant response element (ARE) signaling pathways also induced a metabolic disorder in the ECM, contributing to the pathogenesis of SUI (Li Y. et al., 2022; Liu et al., 2022).
Elastin is primarily formed during fetal development and is rarely synthesized in adult tissues. It provides supporting tissues with the ability to recoil after physical stress and distension, adding the component of resilience to pelvic tissues (Chen et al., 2005). If elastin is damaged or destroyed in adults, it can cause the tissue in which elastin is located to function abnormally. Transmission electron microscopy has been used to examine differences in elastin in the periurethral connective tissue of women with and without SUI, and elastin is irregularly fragmented in SUI patients. This findings indicate that elastin fiber dysfunction may be an important pathology of SUI (Goepel and Thomssen, 2006).
Elastin can be degraded by serine proteases, cysteine proteases, and MMPs. Of the serine proteases, Norepinephrine (NE) is the best known of the degradative enzyme and it is neutralized by α-1 antitrypsin (Wang et al., 2020). Chen et al. studied gene expression in the periurethral connective tissue of the vagina in human women with SUI and found that genes related to elastin metabolism were upregulated in these women compared with controls (Chen et al., 2006). Plasma elastase activity in women with SUI has been shown to be 3 times higher than activity in continent women. Elastolytic activity is increased in pelvic tissues from women with SUI, through an increase in Norepinephrine (NE) activity and a concurrent decrease in α-1 antitrypsin expression (Chen et al., 2004; Chen et al., 2007). Lee et al. found that lysyl oxidase like-1-knockout (LOXL1-KO) mice could not properly assemble elastic fibers and exhibited SUI symptoms (Lee et al., 2008), further indicating that the complete elastic fiber system plays an important role in SUI. Moreover, by constructing a delivery, vaginal distension and ovariectomy (DVDO) rat model of birth trauma, a study showed that birth trauma may activate the expression of urethral elastin through the TGF-β1-associated transcription factors Smad1 and Smad3/4, and estrogen interferes with this signal transduction, resulting in improper assembly of elastic fibers, and weakens the urethra’s ability to withstand the pressure, thus resulting in leakage urine (Lin et al., 2010; Li et al., 2012).
A study showed that the mRNA expression of fibrillin-1 was significantly lower in women with SUI than in women without SUI (Soderberg et al., 2010). Fibrillin is the main component of microfibrils and is important for elastic fiber assembly. Loss of tissue elasticity may lead to hypermobility of the urethra, which in turn results in SUI. In an SUI rat model of urethral injury, pure population of human smooth muscle progenitor cells derived from human pluripotent stem cells (iPSC-pSMCs) were injected around the urethra. The density of elastic fibers in the urethra of SUI rats was increased, and the shape was improved. iPSC-pSMCs can induce ECM remodeling around the urethra in SUI rats, which also suggests that the abnormal elastic fibers may have the same or related molecular mechanism as the ECM abnormalities in SUI. However, this study did not observe improvements in the behavioral marks of SUI rats (Li et al., 2016).
In addition, the macromolecular chondroitin sulfate proteoglycan Vcan and its binding partner hyaluronic acid (HA) accumulate in SUI tissues during inflammation and injury, interfering with elastic fiber assembly in various cases (Keire et al., 2016). A matrix rich in HA and Vcan can promote the expression of chemokines and enhance the binding and retention of inflammatory cells in injured tissues. This process is essential for myofibroblasts (Hattori et al., 2011) and is involved in the development of tissue fibrosis and the occurrence of SUI (Coleman, 2002).
The hormonal status in SUI patients might play an important and potentially modifiable role in preserving and restoring urinary continence (Kreydin et al., 2022). Pelvic floor tissue is regulated by the estrogen and androgen signaling pathways. Recently, the role of androgens in SUI has been explored and shown some preliminary promise. The levator ani muscle and pubocervical fascia are androgen sensitive and contain a large number of androgen receptors. Androgens can promote hypertrophy and hyperplasia in pelvic floor muscles and connective tissue. They cause an increase in fat-free muscle mass, muscle diameter and maximal muscle strength (Fitzgerald, 1994; Castelan et al., 2020). A previous study showed that testosterone levels were lower and SUI complaints were more prevalent in postmenopausal women than in perimenopausal women (Kwon et al., 2014). Kim et al. also reported that low serum testosterone is associated with an increased likelihood of SUI in women (Kim and Kreydin, 2018). Furthermore, a significant association was found between Δ4-androstenedione and the presence of SUI was found. More specifically, women with higher Δ4-androstenedione had lower odds for having SUI (Augoulea et al., 2017). As women age, androgen exposure decreases, which may be another pathophysiological factor leading to the development of SUI. Current evidence supports the ability of androgens to increase pelvic floor muscle mass and strength. Mammadov et al. utilized a rat model to demonstrated that testosterone has both preventive and curative effects on SUI (Mammadov et al., 2011). Some researchers have reported the effect of a selective androgen receptor modulators (SARMs) in the treatment of SUI by enhancing smooth- and striated muscle-mediated urethral function under stress conditions such as sneezing in a rat model (Kadekawa et al., 2020). However, in women, serum androgen levels are low. The effect size related to the serum testosterone difference between women with and without SUI is small, and the effect of Δ4-androstenedione on sex hormone-binding globulin (SHBG) is far less significant than that of endogenous estradiol. In addition, the long-term side effects of androgens and the role of SARMs remain unknown and deserve further study.
The interplay between estrogens and SUI has long been studied with equivocal results. Estrogen has an important impact on the function of the lower genitourinary system and continence mechanism. It raises the sensory threshold of the bladder, increases urethral closure pressure and improves pressure transmission to the proximal urethra (Ahn et al., 2011). The incidence of SUI increases with aging and appears to be associated with age-related declines in estrogen. Some studies have reported decreased E2 levels in postmenopausal and premenopausal women with SUI (Bodner-Adler et al., 2017). In addition, in premenopausal women, downregulation of estrogen receptor α (ERα) expression also plays an important role in SUI. An animal study using an Erα-deficient mouse model, showed a significantly decreased leak point pressure (LPP) in the urodynamics of knockout mice when compared to controls, suggesting an association between ERα and SUI (Ozbek et al., 2014).
However, a deleterious effect on SUI has been observed in some clinical studies. The large SUI subset of the Women’s Health Initiative (WHI) study found that SUI worsened with both estrogen alone and continuous combined estrogen/progestin treatment (Hendrix et al., 2005). This could be caused by several factors. First, oral estrogen therapy has a pronounced enterohepatic first pass effect. The estrogen load on the liver induces an increased production of SHBG. SHBG which binds testosterone and thereby reduces the amount of the free, biologically active hormone, thus worsening SUI through androgen deprivation (Siddle and Versi, 2022). Second, estrogen decreases the total collagen concentration and collagen cross-linking, and increases collagen turnover in peri-urethral tissues (Jackson et al., 2002), thereby affecting urethral motility and urethral closure mechanisms.
Stem cell therapy has emerged as a promising approach in the field of regenerative medicine in the past few years due to its ability to self-renew, form clonal populations and differentiate into different cell types (Gallo et al., 2019). These cells can restore and maintain normal function by differentiation to replace injured or diseased tissues such as smooth or striated muscle for urethral sphincter regeneration. In addition, stem cells can also exert therapeutic effects by secreting a variety of bioactively angiogenic and cytoprotective factors (Dissaranan et al., 2014). Current research indicates that SUI treatment mainly induces or promotes the formation of normal muscle fibers by various means, thereby replenishing damaged or aging muscle cells to improve muscle function.
Stem cells have shown great potential in SUI therapy and have been used in some clinical treatment studies (Table 1). Currently, the most widely used cells are muscle-derived stem cells (MDSCs) and non-muscle-derived stem cells, such as adipose-derived stem cells (ADSCs), bone marrow hematopoietic stem cells (BMDSCs), amniotic fluid stem cells (AFSCs) or cord blood stem cells (CBSCs) (Zhou et al., 2016). After verifying the plasticity of stem cells in proliferating and differentiating into functional cells in vitro, they were expanded to a certain number and injected directly, or differentiated into muscle precursor cells in vitro and injected, or mixed with some other cells or growth factors and injected into the urinary system or specific surrounding areas (Gallo et al., 2019) (Figure 4). Myogenic differentiation in the damaged EUS can improve or repair damaged striated muscle tone in patients with SUI, thereby improving the function of damaged tissues.
FIGURE 4. Stem cells in the treatment of SUI. The most widely used stem cells for stress urinary incontinence (SUI) are muscle-derived stem cells (MDSCs), adipose-derived stem cells (ADSCs), bone marrow hematopoietic stem cells (BMDSCs), amniotic fluid stem cells (AFSCs) and cord blood stem cells (CBSCs). After verifying the plasticity of stem cells, they are expanded to a certain number and injected into the urinary system. EUS: external urethral sphincter; IUS: internal urethral sphincter; SUI:stress urinary incontinence.
MDSCs are currently the most widely used stem cells for the treatment of SUI. These cells are derived from satellite cells which between the basal lamina and sarcolemma of muscle fibers (Morgan and Partridge, 2003). MDSCs can be easily obtained from muscle tissue under local anesthesia. MDSCs are able to differentiate not only into muscle cells, but also to differentiate into neural and endothelial cell lines, making them an ideal material for the treatment of SUI.
The use of injectable cultured MDSCs for the treatment of SUI has been investigated in several animal studies, including those using rodent, porcine, canine and primate models, found good tissue regeneration, improved valsalva leak point pressure (VLPP) and recovered contractile urethral sphincter function (Hillary et al., 2020). The most commonly used animal model is rodents. It was initially reported that sphincter function was improved by injecting MDSCs into pudendal denervated rats (Lee et al., 2004). An early study demonstrated that muscle precursor cells harvested from limb skeletal muscles in mice, may accelerate sphincter muscle repair, as shown by a higher myofiber diameter and number at 7 days. One month after injection, muscle precursor cells were still detectable in the regenerating sphincters and participated in the formation of new myofibers (Yiou et al., 2002). Badra et al. reported that the injections of five million autologous green fluorescent protein labeled skeletal muscle precursor cells resulted in long-term structural and functional restoration of the injured sphincter complex in a monkey model (Badra et al., 2013).
Studies on using MDSCs to treat SUI have reached the clinical level. Several studies have investigated the safety and efficacy of autologous MDSCs therapy among SUI patients (Table 1). A study conducted a 1-year follow-up of eight female SUI patients (42–65 years old) who received MDSCs injection under local anesthesia. This study found that symptoms improved in five (62.5%) SUI patients, and one woman had complete remission (Carr et al., 2008). In the largest series of patients, 123 women with SUI were treated with transurethral ultrasonography-guided injections with autologous myoblasts and fibroblasts obtained from skeletal muscle biopsies. At 1 year after injecting these cells, 79% of women were subjectively completely continent, with significant improvements in the incontinence and quality of life instrument (I-QOL) scores, and the thickness, contractility and electromyographic activity of the rhabdosphincter (Mitterberger et al., 2007). Another large study investigating the use of MDSCs injection for the treatment of SUI, including 80 female patients, was published in 2014. The investigators pooled the data from two trials of autologous MDSCs injections at a dose range of 10 × 106–200 × 106. All treatment groups described subjective improvements sustained over the study period, and higher dose groups had greater percentages of patients with at least a 50% reduction in stress leaks and pad weight (Peters et al., 2014). Recently, a paper summarized 11 studies on the application of MDSCs in the clinical treatment of SUI and found that the median postoperative improvement rate of SUI patients after the injection of MDSCs was 77% (Barakat et al., 2020). Intraurethral injection of MDSCs is a simple surgical procedure that appears safe and effective in women with SUI. However, MDSCs have relatively poor proliferative potential and often require repeated injections to provide sufficient cells. Additionally, treatment efficacy declines significantly over time. Furthermore, the biopsy procedure to obtain a sufficient number of MDSCs is painful, and the cell harvesting procedure, if performed incorrectly, may increase the risk of infection in the patients.
ADSCs originate from the mesoderm and can be repeatedly and abundantly acquired from adipose tissues. ADSCs can proliferate rapidly and robustly in vitro, and differentiate into various mesodermal and ectodermal cells, including muscle cells, vascular smooth muscle cells, endothelial cells and neural cells. This is highly relevant for tissue regeneration and revascularization, in the context of a muscular and vascular structure that is denervated and weakened (Roche et al., 2010).
Some studies have used 5-aza-2′-deoxycytidine to induce the differentiation of ADSCs into myoblasts in vitro. Subsequently, the induced ADSCs were injected into the posterior muscularis urethra of an SUI rat model, and follow-up analysis demonstrated significant improvements in SUI symptoms (Fu et al., 2010). The implantation of autologous ADSCs into cryo-injured rabbit urethras could promote myogenic differentiation, nerve regeneration and neovascularization in the surrounding tissues and restore urethral function (Silwal Gautam et al., 2014). Furthermore, researchers in one study compared the effects of periurethral and intravenous injection of ADSCs on voiding function and tissue recovery in a SUI rat model. This study found that both two methods of ADSCs injection induced different degrees of recovery of the urethral sphincter, cytokine secretion levels and cell retention rates in the urethral tissues in SUI rats, however, there was no significant difference between the 2 methods (Li G. et al., 2022).
ADSCs have also been utilized in clinical treatment studies for SUI and show great therapeutic potential with a median postoperative improvement rate of 88% after injection (Barakat et al., 2020). One study investigated transurethral ADSC injections in women with SUI. Cells were suspended in a contigen matrix for injection. At 1 year, three of five patients had a negative cough test with the bladder filled to 500 mL. Validated questionnaires showed some subjective improvement in all five patients, but differences in postoperative urodynamic parameters were not significant (Kuismanen et al., 2014). Another study included 45 male SUI patients with mild-to-moderate urine leakage persisting more than 1 year after prostatectomy. Patients demonstrated significant improvements in leakage volume and King’s Health Questionnaire at 52 weeks after ADSCs injection (Gotoh et al., 2020). However, there are still few studies on the clinical application of ADSCs in the treatment of SUI, and there is insufficient evidence to support the long-term efficacy and possible adverse reactions of ADSCs in the treatment of SUI.
Bone marrow-derived stem cells (BMDSCs) have promising regenerative properties in preclinical studies. In one preclinical study, BMDSCs were injected into the urethral sphincter of New Zealand White rabbits that were cryo-injured by spraying liquid nitrogen. The proportions of myoglobin- and smooth muscle actin-expressing areas in cell-implanted regions were significantly higher than those of in the controls. By 14 days, these differentiated cells formed contacts with similar cells, creating layered muscle structures. At that time, the leak point pressure of the cell-implanted rabbits was significantly higher than that of the controls (Imamura et al., 2011). In another study, BMDSCs transplantation enhanced closing pressure and leak point pressure in a female urinary incontinence Sprague-Dawley rat model (Kim et al., 2011). However, bone marrow procurement requires general or spinal anesthesia, and yields a low number of stem cells upon processing. No trial in humans on the effect of BMDSCs injection for the treatment of SUI has been reported to date.
Although MDSCs and ADSCs can be obtained in large quantities under local anesthesia, collection remains an invasive procedure with the risk of morbidity. Stem cells can also be obtained from human cord blood or amniotic fluid. These cells have a high proliferation rate, induce immune tolerance, display embryonic stem cell properties and are able to differentiate into cells representing all three embryonic germ layers including cells of adipogenic, osteogenic, myogenic, endothelial, neural, and hepatic lineages (De Coppi et al., 2007). Peri-urethral injections of CBSCs for the treatment of SUI were investigated in one study, which included 39 patients with SUI. 29 patients (83%) were more than 50% satisfied according to the patient’s satisfaction results after 3 months, and 26 (72.2%) continuously showed more than 50% improvement after 12 months. Intrinsic sphincter deficiency and mixed stress incontinency improved in the ten patients evaluated by urodynamic study.
AFSCs displayed MSC characteristics and could differentiate into cells of the myogenic lineage. Kim et al. evaluated the therapeutic feasibility of periurethral injection of AFSCs in an SUI animal model. Four weeks after injection, the mean leak point pressure and closing pressure were significantly increased in the AFSC-injected group compared with the control group. Nerve regeneration and neuromuscular junction formation of injected AFSCs in vivo were confirmed by the expression of neuronal markers and acetylcholine receptors (Kim et al., 2012).
In addition, investigators continue to identify novel, less-invasive cell sources for SUI, including cells derived from hair follicles, menstrual fluid, and urine (Gallo et al., 2019). However, as there have been few studies as of July 2022, there are currently fewer than 20 clinical trials registered internationally (Table 2). Additional studies are essential to further investigate the efficacy and safety of stem cells in the treatment of SUI. Cell regeneration therapy in the treatment of SUI is currently progressing well. However, the survival time after stem cell transplantation, the side effects such as tissue swelling, and the optimal amounts of cells for injection still require more comprehensive and careful evaluation, and an optimization of technical processes is needed.
Using exosomes from stem cells to promote cell differentiation is a novel regenerative strategy. Exosomes are membrane-wrapped microcontainers that are separated from cell membranes by exocytosis; they have a diameter of approximately 30–150 nm. Exosomes are able to transport proteins, cytokines and mRNA to target cells and exert effects (Wu et al., 2019). Exosomes secreted by stem cells have been shown to have stem cell-like functions; they effectively prevent tissue damage and repair impaired tissues. Furthermore, they avoid the potential risks of cell transplantation, such as immunogenicity, tumorigenicity, or vascular obstruction. Exosomes containing soluble cytokines can be isolated by the ultrafiltration and centrifugation of cell culture supernatants and the selective separation of samples using ultrafiltration membranes with different relative molecular weight cutoffs (MWCOs) (Phetfong et al., 2022).
The treatment of SUI with exosomes is one of the emerging strategies in recent years. Initial studies showed that ADSC-derived exosomes could enhance the growth of skeletal muscle and Schwann cell lines in a dose-dependent manner. In vivo experiments showed that ADSC-derived exosomes could improve urethral function and histology after SUI, and the performance was slightly better than that of ADSCs (Ni et al., 2018). In addition to being able to differentiate into skeletal muscle cells, exosomes can also promote the synthesis of collagen fibers in vitro. Studies have reported that exosomes from mesenchymal stem cells could regulate the expression of TIMP-1 and MMP-1, promoting collagen synthesis and inhibiting collagen degradation. Thus, this may be a novel treatment strategy for SUI (Liu et al., 2018). In addition to exosomes secreted by common stem cells (Zhou et al., 2021), Schwann cell-derived exosomes (Hu et al., 2019; Wu et al., 2019; Huang et al., 2021) have also shown potential in the treatment of SUI in vivo. However, there have been few studies on the treatment of SUI with exosomes. The curative effect on clinical patients has not been examined, and further research is needed to gain a deeper understanding of exosomes.
With the increasing understanding of the molecular pathogenesis of SUI, some certain molecules have been confirmed to be involved in the pathophysiological process of the occurrence and development of SUI. Regulating the expression of pathogenic molecules in SUI to restore damaged tissue function is a feasible treatment strategy. In addition to the aforementioned direct silencing of MSTN expression using CRISPR/Cas9 technology to restore urination parameters in SUI rats (Yuan et al., 2020), other transgenic methods have also been applied in the treatment of SUI. The chemokine stromal derived factor-1(SDF-1) can enhance tissue regeneration through stem cells chemotaxis, neovascularization, and neuronal regeneration. The injection of the SDF-1 overexpression plasmid was shown to improve continence function in SUI female rat model. The increased amount of urethral sphincter muscle and higher vascular density provide a potential strategy for the treatment of SUI (Khalifa et al., 2020).
Oxidative stress and inflammatory pathway activation are important mechanisms for the occurrence of SUI, and increased apoptosis and oxidative damage can be found in fibroblasts in a mouse model of SUI induced by mechanical injury (Liu et al., 2022). Nuclear factor erythroid 2-related factor 2 (Nrf2) is a widely used inducer of antioxidant genes that has been shown to reduce oxidative damage and apoptosis. The Nrf2 gene was overexpressed by lentivirus transfection in a mouse model of vaginal dilatation (VD)-induced SUI, and Nrf2 overexpression significantly reduced VD-induced vaginal anterior wall abnormalities. Thus, this may be a promising treatment strategy for mechanical trauma-related SUI (Tang et al., 2019).
Gene regulation is not always used as a monotherapy for SUI, and can also be used in combination with other regimens, such as cell therapy. Lentiviral vector-mediated Smad3 shRNA blockade in SUI rats has been shown to inhibit the differentiation of MDSCs into fibroblasts (but without affecting their myogenic differentiation), which can improve MDSC-mediated repair of urinary sphincter function (Wang et al., 2011). Similarly, Haralampieva D et al. overexpressed human peroxisome proliferator-activated receptor gamma coactivator 1-α (hPGC-1α) in human muscle precursor cells (hMPCs) with an adenovirus. hPGC-1α-overexpressing hMPCs were injected into injured skeletal muscle. hMPCs could significantly promoted muscle regeneration after injury, reduced the expression of proinflammatory cytokines (TNF-α) and enhanced the healing process. (Haralampieva et al., 2018).
The main pathological mechanisms of SUI are the dysfunction of the nervous system regulating the continence structure, the functional defects in the urethral support structure, and the decreased function of the urethral sphincter, resulting in the inability of the urethra to maintain closure when the abdominal pressure increases. The occurrence of SUI may be affected by a variety of molecules, including neurogenic, muscle-derived and connective tissue-derived molecules. Although the current treatments include conservative and surgical treatment, only 25% of women with SUI currently receive treatment due to the low efficacy of conservative treatment and complications associated with surgery (Lukacz et al., 2017). An increasing number of researchers are beginning to pay attention to new treatment methods. With the continuous advancement of technology, the development of various technologies, including cell therapy, exosome differentiation, gene regulation and other technologies, will provide broad prospects for the treatment of SUI. Most of the research is limited to the cell or animal research stage. Although some studies also involve clinical research, the observation time is short. Additionally, the long-term efficacy and safety of new treatment methods still need further careful and long-term evaluation.
The X-xW investigated and wrote the original draft of the manuscript. LZ reviewed and edited the manuscript. YL proposed the theme of the review and helped in writing the manuscript. All authors read and approved the final manuscript.
The authors declare that the research was conducted in the absence of any commercial or financial relationships that could be construed as a potential conflict of interest.
All claims expressed in this article are solely those of the authors and do not necessarily represent those of their affiliated organizations, or those of the publisher, the editors and the reviewers. Any product that may be evaluated in this article, or claim that may be made by its manufacturer, is not guaranteed or endorsed by the publisher.
Abufaraj, M., Xu, T., Cao, C., Siyam, A., Isleem, U., Massad, A., et al. (2021). Prevalence and trends in urinary incontinence among women in the United States, 2005-2018. Am. J. Obstet. Gynecol. 225, 166.e1–166166.e12. doi:10.1016/j.ajog.2021.03.016
Ahn, K. H., Kim, T., Hur, J. Y., Kim, S. H., Lee, K. W., and Kim, Y. T. (2011). Relationship between serum estradiol and follicle-stimulating hormone levels and urodynamic results in women with stress urinary incontinence. Int. Urogynecol J. 22, 731–737. doi:10.1007/s00192-011-1359-6
Akita, Y., Sumino, Y., Mori, K., Nomura, T., Sato, F., and Mimata, H. (2013). Myostatin inhibits proliferation of human urethral rhabdosphincter satellite cells. Int. J. Urol. 20, 522–529. doi:10.1111/j.1442-2042.2012.03186.x
Alhasso, A., Glazener, C. M., Pickard, R., and N'dow, J. (2005). Adrenergic drugs for urinary incontinence in adults. London, UK: Cochrane Database Syst Rev, CD001842.
Anderson, K. E. (1993). Pharmacology of lower urinary tract smooth muscles and penile erectile tissues. Pharmacol. Rev. 45, 253–308.
Augoulea, A., Sioutis, D., Rizos, D., Panoulis, C., Triantafyllou, N., Armeni, E., et al. (2017). Stress urinary incontinence and endogenous sex steroids in postmenopausal women. Neurourol. Urodyn. 36, 121–125. doi:10.1002/nau.22885
Aydogmus, S., Kelekci, S., Aydogmus, H., Demir, M., Yilmaz, B., and Sutcu, R. (2015). Association of antepartum vitamin D levels with postpartum pelvic floor muscle strength and symptoms. Int. Urogynecol J. 26, 1179–1184. doi:10.1007/s00192-015-2671-3
Badalian, S. S., and Rosenbaum, P. F. (2010). Vitamin D and pelvic floor disorders in women: Results from the national health and nutrition examination survey. Obstet. Gynecol. 115, 795–803. doi:10.1097/AOG.0b013e3181d34806
Badra, S., Andersson, K. E., Dean, A., Mourad, S., and Williams, J. K. (2013). Long-term structural and functional effects of autologous muscle precursor cell therapy in a nonhuman primate model of urinary sphincter deficiency. J. Urol. 190, 1938–1945. doi:10.1016/j.juro.2013.04.052
Balog, B. M., Deng, K., Labhasetwar, V., Jones, K. J., and Damaser, M. S. (2019). Electrical stimulation for neuroregeneration in urology: A new therapeutic paradigm. Curr. Opin. Urol. 29, 458–465. doi:10.1097/MOU.0000000000000632
Barakat, B., Franke, K., Schakaki, S., Hijazi, S., Hasselhof, V., and Vogeli, T. A. (2020). Stem cell applications in regenerative medicine for stress urinary incontinence: A review of effectiveness based on clinical trials. Arab. J. Urol. 18, 194–205. doi:10.1080/2090598X.2020.1750864
Bhogal, R. K., and Bona, C. A. (2008). Regulatory effect of extracellular signal-regulated kinases (ERK) on type I collagen synthesis in human dermal fibroblasts stimulated by IL-4 and IL-13. Int. Rev. Immunol. 27, 472–496. doi:10.1080/08830180802430974
Bodner-Adler, B., Bodner, K., Kimberger, O., Halpern, K., Rieken, M., Koelbl, H., et al. (2017). Role of serum steroid hormones in women with stress urinary incontinence: A case-control study. BJU Int. 120, 416–421. doi:10.1111/bju.13902
Cao, C., Zhang, C., Sriskandarajah, C., Xu, T., Gotto, G., Sutcliffe, S., et al. (2022). Trends and racial disparities in the prevalence of urinary incontinence among men in the USA, 2001-2020. Eur. Urol. Focus 8, 1758–1767. doi:10.1016/j.euf.2022.04.015
Capobianco, G., Madonia, M., Morelli, S., Dessole, F., De Vita, D., Cherchi, P. L., et al. (2018). Management of female stress urinary incontinence: A care pathway and update. Maturitas 109, 32–38. doi:10.1016/j.maturitas.2017.12.008
Carr, L. K., Steele, D., Steele, S., Wagner, D., Pruchnic, R., Jankowski, R., et al. (2008). 1-year follow-up of autologous muscle-derived stem cell injection pilot study to treat stress urinary incontinence. Int. Urogynecol J. Pelvic Floor Dysfunct. 19, 881–883. doi:10.1007/s00192-007-0553-z
Castelan, F., Cuevas-Romero, E., and Martinez-Gomez, M. (2020). The expression of hormone receptors as a gateway toward understanding endocrine actions in female pelvic floor muscles. Endocr. Metab. Immune Disord. Drug Targets 20, 305–320. doi:10.2174/1871530319666191009154751
Ceglia, L., and Harris, S. S. (2013). Vitamin D and its role in skeletal muscle. Calcif. Tissue Int. 92, 151–162. doi:10.1007/s00223-012-9645-y
Chang, H. M., Wu, H. C., Sun, Z. G., Lian, F., and Leung, P. C. K. (2019). Neurotrophins and glial cell line-derived neurotrophic factor in the ovary: Physiological and pathophysiological implications. Hum. Reprod. Update 25, 224–242. doi:10.1093/humupd/dmy047
Chen, B. H., Wen, Y., Li, H., and Polan, M. L. (2002). Collagen metabolism and turnover in women with stress urinary incontinence and pelvic prolapse. Int. Urogynecol J. Pelvic Floor Dysfunct. 13, 80–87. ; discussion 87. doi:10.1007/s001920200020
Chen, B., Wen, Y., and Polan, M. L. (2004). Elastolytic activity in women with stress urinary incontinence and pelvic organ prolapse. Neurourol. Urodyn. 23, 119–126. doi:10.1002/nau.20012
Chen, B., Wen, Y., Yu, X., and Polan, M. L. (2005). Elastin metabolism in pelvic tissues: Is it modulated by reproductive hormones? Am. J. Obstet. Gynecol. 192, 1605–1613. doi:10.1016/j.ajog.2004.11.027
Chen, B., Wen, Y., Yu, X., and Polan, M. L. (2007). The role of neutrophil elastase in elastin metabolism of pelvic tissues from women with stress urinary incontinence. Neurourol. Urodyn. 26, 274–279. doi:10.1002/nau.20347
Chen, B., Wen, Y., Zhang, Z., Guo, Y., Warrington, J. A., and Polan, M. L. (2006). Microarray analysis of differentially expressed genes in vaginal tissues from women with stress urinary incontinence compared with asymptomatic women. Hum. Reprod. 21, 22–29. doi:10.1093/humrep/dei276
Chen, B., and Yeh, J. (2011). Alterations in connective tissue metabolism in stress incontinence and prolapse. J. Urol. 186, 1768–1772. doi:10.1016/j.juro.2011.06.054
Chen, D., Meng, W., Shu, L., Liu, S., Gu, Y., Wang, X., et al. (2020). ANO1 in urethral SMCs contributes to sex differences in urethral spontaneous tone. Am. J. Physiol. Ren. Physiol. 319, F394-F402–F402. doi:10.1152/ajprenal.00174.2020
Choe, J. Y., Jung, H. J., Park, K. Y., Kum, Y. S., Song, G. G., Hyun, D. S., et al. (2010). Anti-fibrotic effect of thalidomide through inhibiting TGF-beta-induced ERK1/2 pathways in bleomycin-induced lung fibrosis in mice. Inflamm. Res. 59, 177–188. doi:10.1007/s00011-009-0084-9
Chong, E. C., Khan, A. A., and Anger, J. T. (2011). The financial burden of stress urinary incontinence among women in the United States. Curr. Urol. Rep. 12, 358–362. doi:10.1007/s11934-011-0209-x
Cipullo, L. M., Zullo, F., Cosimato, C., Di Spiezio Sardo, A., Troisi, J., and Guida, M. (2014). Pharmacological treatment of urinary incontinence. Female Pelvic Med. Reconstr. Surg. 20, 185–202. doi:10.1097/SPV.0000000000000076
Coleman, P. J. (2002). Evidence for a role of hyaluronan in the spacing of fibrils within collagen bundles in rabbit synovium. Biochim. Biophys. Acta 1571, 173–182. doi:10.1016/s0304-4165(02)00213-1
D'ancona, C., Haylen, B., Oelke, M., Abranches-Monteiro, L., Arnold, E., Goldman, H., et al. (2019). The International Continence Society (ICS) report on the terminology for adult male lower urinary tract and pelvic floor symptoms and dysfunction. Neurourol. Urodyn. 38, 433–477. doi:10.1002/nau.23897
Danuser, H., Bemis, K., and Thor, K. B. (1995). Pharmacological analysis of the noradrenergic control of central sympathetic and somatic reflexes controlling the lower urinary tract in the anesthetized cat. J. Pharmacol. Exp. Ther. 274, 820–825.
De Coppi, P., Bartsch, G., Siddiqui, M. M., Xu, T., Santos, C. C., Perin, L., et al. (2007). Isolation of amniotic stem cell lines with potential for therapy. Nat. Biotechnol. 25, 100–106. doi:10.1038/nbt1274
De Vries, A. M., Venema, P. L., and Heesakkers, J. (2018). Midurethral support is also necessary for reflex closure of the urethra. Neurourol. Urodyn. 37, 2965–2972. doi:10.1002/nau.23807
Delancey, J. O., and Ashton-Miller, J. A. (2004). Pathophysiology of adult urinary incontinence. Gastroenterology 126, S23–S32. doi:10.1053/j.gastro.2003.10.080
Delancey, J. O. (1994). Structural support of the urethra as it relates to stress urinary incontinence: The hammock hypothesis. Am. J. Obstet. Gynecol. 170, 17131720–17201713. doi:10.1016/s0002-9378(94)70346-9
Dhaliwal, J. S., Spurling, B. C., and Molla, M. (2022). “Duloxetine,” in StatPearls (Treasure Island (FL).
Dissaranan, C., Cruz, M. A., Kiedrowski, M. J., Balog, B. M., Gill, B. C., Penn, M. S., et al. (2014). Rat mesenchymal stem cell secretome promotes elastogenesis and facilitates recovery from simulated childbirth injury. Cell. Transpl. 23, 1395–1406. doi:10.3727/096368913X670921
Farnsworth, B. (2002). The integral theory of female urinary incontinence. Aust. N. Z. J. Obstet. Gynaecol. 42, 99; author reply 100. author reply 100. doi:10.1111/j.0004-8666.2002.111_1.x
Feng, M., Wang, Z., Liu, Z., Liu, D., Zheng, K., Lu, P., et al. (2019). The RyR-ClCa -VDCC axis contributes to spontaneous tone in urethral smooth muscle. J. Cell. Physiol. 234, 23256–23267. doi:10.1002/jcp.28892
Fowler, C. J. (2006). Integrated control of lower urinary tract--clinical perspective. Br. J. Pharmacol. 147 (2), S14–S24. doi:10.1038/sj.bjp.0706629
Fu, Q., Song, X. F., Liao, G. L., Deng, C. L., and Cui, L. (2010). Myoblasts differentiated from adipose-derived stem cells to treat stress urinary incontinence. Urology 75, 718–723. doi:10.1016/j.urology.2009.10.003
Gallo, F., Ninotta, G., Schenone, M., Cortese, P., and Giberti, C. (2019). Advances in stem cell therapy for male stress urinary incontinence. Expert Opin. Biol. Ther. 19, 293–300. doi:10.1080/14712598.2019.1578343
Gill, B. C., Balog, B. M., Dissaranan, C., Jiang, H. H., Steward, J. B., Lin, D. L., et al. (2013). Neurotrophin therapy improves recovery of the neuromuscular continence mechanism following simulated birth injury in rats. Neurourol. Urodyn. 32, 82–87. doi:10.1002/nau.22264
Goepel, C., and Thomssen, C. (2006). Changes in the extracellular matrix in periurethral tissue of women with stress urinary incontinence. Acta histochem. 108, 441–445. doi:10.1016/j.acthis.2006.07.001
Gordon, T. (2020). Peripheral nerve regeneration and muscle reinnervation. Int. J. Mol. Sci. 21, 8652. doi:10.3390/ijms21228652
Gotoh, M., Shimizu, S., Yamamoto, T., Ishizuka, O., Yamanishi, T., Mizokami, A., et al. (2020). Regenerative treatment for male stress urinary incontinence by periurethral injection of adipose-derived regenerative cells: Outcome of the ADRESU study. Int. J. Urol. 27, 859–865. doi:10.1111/iju.14311
Greiwe, J. S., Cheng, B., Rubin, D. C., Yarasheski, K. E., and Semenkovich, C. F. (2001). Resistance exercise decreases skeletal muscle tumor necrosis factor alpha in frail elderly humans. Faseb J. 15, 475–482. doi:10.1096/fj.00-0274com
Hanada, M., Sumino, Y., Hirata, Y., Sato, F., and Mimata, H. (2010). Growth inhibition and apoptosis induction by tumor necrosis factor-α in human urethral rhabdosphincter satellite cells. J. Urol. 183, 2445–2450. doi:10.1016/j.juro.2010.01.063
Haralampieva, D., Salemi, S., Betzel, T., Dinulovic, I., Kramer, S. D., Schibli, R., et al. (2018). Injected human muscle precursor cells overexpressing PGC-1α enhance functional muscle regeneration after trauma. Stem Cells Int. 2018, 4658503. doi:10.1155/2018/4658503
Hattori, N., Carrino, D. A., Lauer, M. E., Vasanji, A., Wylie, J. D., Nelson, C. M., et al. (2011). Pericellular versican regulates the fibroblast-myofibroblast transition: A role for ADAMTS5 protease-mediated proteolysis. J. Biol. Chem. 286, 34298–34310. doi:10.1074/jbc.M111.254938
Hendrix, S. L., Cochrane, B. B., Nygaard, I. E., Handa, V. L., Barnabei, V. M., Iglesia, C., et al. (2005). Effects of estrogen with and without progestin on urinary incontinence. Jama 293, 935–948. doi:10.1001/jama.293.8.935
Hillary, C. J., Roman, S., Macneil, S., Aicher, W. K., Stenzl, A., and Chapple, C. R. (2020). Regenerative medicine and injection therapies in stress urinary incontinence. Nat. Rev. Urol. 17, 151–161. doi:10.1038/s41585-019-0273-4
Holick, M. F. (2003). Vitamin D: A millenium perspective. J. Cell. Biochem. 88, 296–307. doi:10.1002/jcb.10338
Hu, M., Hong, L., Liu, C., Hong, S., He, S., Zhou, M., et al. (2019). Electrical stimulation enhances neuronal cell activity mediated by Schwann cell derived exosomes. Sci. Rep. 9, 4206. doi:10.1038/s41598-019-41007-5
Huang, G., Hu, M., Lu, D., and Hong, L. (2021). Protective effect and potential mechanism of Schwann cell-derived exosomes on mechanical damage of rat dorsal root ganglion cells. J. Obstet. Gynaecol. Res. 47, 3691–3701. doi:10.1111/jog.14968
Huang, Q., Jin, H., Xie, Z., Wang, M., Chen, J., and Zhou, Y. (2013). The role of the ERK1/2 signalling pathway in the pathogenesis of female stress urinary incontinence. J. Int. Med. Res. 41, 1242–1251. doi:10.1177/0300060513493995
Imamura, T., Ishizuka, O., Kinebuchi, Y., Kurizaki, Y., Nakayama, T., Ishikawa, M., et al. (2011). Implantation of autologous bone-marrow-derived cells reconstructs functional urethral sphincters in rabbits. Tissue Eng. Part A 17, 1069–1081. doi:10.1089/ten.TEA.2010.0478
Jackson, S., James, M., and Abrams, P. (2002). The effect of oestradiol on vaginal collagen metabolism in postmenopausal women with genuine stress incontinence. Bjog 109, 339–344. doi:10.1111/j.1471-0528.2002.01052.x
Jeon, Y. M., Kook, S. H., Son, Y. O., Kim, E. M., Park, S. S., Kim, J. G., et al. (2009). Role of MAPK in mechanical force-induced up-regulation of type I collagen and osteopontin in human gingival fibroblasts. Mol. Cell. Biochem. 320, 45–52. doi:10.1007/s11010-008-9897-z
Ji, Q., Guo, S., Wang, X., Pang, C., Zhan, Y., Chen, Y., et al. (2019). Recent advances in TMEM16A: Structure, function, and disease. J. Cell. Physiol. 234, 7856–7873. doi:10.1002/jcp.27865
Jiang, H. H., Gill, B. C., Dissaranan, C., Zutshi, M., Balog, B. M., Lin, D., et al. (2013). Effects of acute selective pudendal nerve electrical stimulation after simulated childbirth injury. Am. J. Physiol. Ren. Physiol. 304, F239–F247. doi:10.1152/ajprenal.00235.2012
Kadekawa, K., Kawamorita, N., Shimizu, T., Kurobe, M., Turnbull, P. S., Chandra, S., et al. (2020). Effects of a selective androgen receptor modulator (SARM), GSK2849466A, on stress urinary incontinence and bladder activity in rats with ovariectomy-induced oestrogen deficiency. BJU Int. 125, 911–919. doi:10.1111/bju.15022
Kamo, I., Kaiho, Y., Canon, T. W., Chancellor, M. B., De Groat, W. C., Prantil, R. L., et al. (2006). Functional analysis of active urethral closure mechanisms under sneeze induced stress condition in a rat model of birth trauma. J. Urol. 176, 2711–2715. doi:10.1016/j.juro.2006.07.139
Kamo, I., Torimoto, K., Chancellor, M. B., De Groat, W. C., and Yoshimura, N. (2003). Urethral closure mechanisms under sneeze-induced stress condition in rats: A new animal model for evaluation of stress urinary incontinence. Am. J. Physiol. Regul. Integr. Comp. Physiol. 285, R356–R365. doi:10.1152/ajpregu.00010.2003
Keire, P. A., Bressler, S. L., Mulvihill, E. R., Starcher, B. C., Kang, I., and Wight, T. N. (2016). Inhibition of versican expression by siRNA facilitates tropoelastin synthesis and elastic fiber formation by human SK-LMS-1 leiomyosarcoma smooth muscle cells in vitro and in vivo. Matrix Biol. 50, 67–81. doi:10.1016/j.matbio.2015.12.010
Khalifa, A. O., Kavran, M., Mahran, A., Isali, I., Woda, J., Flask, C. A., et al. (2020). Stromal derived factor-1 plasmid as a novel injection for treatment of stress urinary incontinence in a rat model. Int. Urogynecol J. 31, 107–115. doi:10.1007/s00192-019-03867-3
Kim, B. S., Chun, S. Y., Lee, J. K., Lim, H. J., Bae, J. S., Chung, H. Y., et al. (2012). Human amniotic fluid stem cell injection therapy for urethral sphincter regeneration in an animal model. BMC Med. 10, 94. doi:10.1186/1741-7015-10-94
Kim, M. M., and Kreydin, E. I. (2018). The association of serum testosterone levels and urinary incontinence in women. J. Urol. 199, 522–527. doi:10.1016/j.juro.2017.08.093
Kim, S. O., Na, H. S., Kwon, D., Joo, S. Y., Kim, H. S., and Ahn, Y. (2011). Bone-marrow-derived mesenchymal stem cell transplantation enhances closing pressure and leak point pressure in a female urinary incontinence rat model. Urol. Int. 86, 110–116. doi:10.1159/000317322
Koch, G. E., and Kaufman, M. R. (2022). Male stress urinary incontinence. Urol. Clin. North Am. 49, 403–418. doi:10.1016/j.ucl.2022.04.005
Krabbe, K. S., Pedersen, M., and Bruunsgaard, H. (2004). Inflammatory mediators in the elderly. Exp. Gerontol. 39, 687–699. doi:10.1016/j.exger.2004.01.009
Kreydin, E., Siddle, N., and Versi, E. (2022). Androgen deficiency and stress urinary incontinence. Int. Urogynecol J. 33, 1353. doi:10.1007/s00192-022-05116-6
Kuismanen, K., Sartoneva, R., Haimi, S., Mannerström, B., Tomás, E., Miettinen, S., et al. (2014). Autologous adipose stem cells in treatment of female stress urinary incontinence: Results of a pilot study. Stem Cells Transl. Med. 3, 936–941. doi:10.5966/sctm.2013-0197
Kwon, J. K., Kim, J. H., Choi, H., Chang, I. H., Park, B. R., Kwon, S. S., et al. (2014). Voiding characteristics and related hormonal changes in peri-menopausal and post-menopausal women: A preliminary study. Maturitas 79, 311–315. doi:10.1016/j.maturitas.2014.07.011
Lee, J. Y., Paik, S. Y., Yuk, S. H., Lee, J. H., Ghil, S. H., and Lee, S. S. (2004). Long term effects of muscle-derived stem cells on leak point pressure and closing pressure in rats with transected pudendal nerves. Mol. Cells 18, 309–313.
Lee, U. J., Gustilo-Ashby, A. M., Daneshgari, F., Kuang, M., Vurbic, D., Lin, D. L., et al. (2008). Lower urogenital tract anatomical and functional phenotype in lysyl oxidase like-1 knockout mice resembles female pelvic floor dysfunction in humans. Am. J. Physiol. Ren. Physiol. 295, F545–F555. doi:10.1152/ajprenal.00063.2008
Li, G. Y., Cui, W. S., Zhou, F., Gao, Z. Z., Xin, H., Liu, T., et al. (2012). Pathology of urethral fibromuscular system related to parturition-induced stress urinary incontinence and TGF-β1/Smad pathway. Mol. Cell. Biochem. 364, 329–335. doi:10.1007/s11010-012-1234-x
Li, G., Yu, C., Yu, P., Peng, Q., Wang, Q., Ren, S., et al. (2022a). Periurethral and intravenous injections of adipose-derived stem cells to promote local tissue recovery in a rat model of stress urinary incontinence. Urology 167, 82–89. doi:10.1016/j.urology.2022.05.018
Li, T., Zhang, Y. J., Zhang, H. L., Ding, X. H., Yu, Z. J., and Lu, S. (2019a). Prevalence and risk factors of stress urinary incontinence among perimenopausal women and its influence on daily life in women with sexual desire problem. Curr. Med. Sci. 39, 615–621. doi:10.1007/s11596-019-2082-7
Li, Y., Liu, C., Li, B., Hong, S., Min, J., Hu, M., et al. (2019b). Electrical stimulation activates calpain 2 and subsequently upregulates collagens via the integrin β1/TGF-β1 signaling pathway. Cell. Signal 59, 141–151. doi:10.1016/j.cellsig.2019.03.023
Li, Y., Liu, C., Yang, L., Li, L., and Hong, L. (2022b). Puerarin protects fibroblasts against mechanical stretching injury through Nrf2/TGF-β1 signaling pathway. Int. Urogynecol J. 33, 2565–2576. doi:10.1007/s00192-022-05325-z
Li, Y., Wen, Y., Wang, Z., Wei, Y., Wani, P., Green, M., et al. (2016). Smooth muscle progenitor cells derived from human pluripotent stem cells induce histologic changes in injured urethral sphincter. Stem Cells Transl. Med. 5, 1719–1729. doi:10.5966/sctm.2016-0035
Li, Z., Li, X., Gao, X., Shen, Q. W., Du, M., and Zhang, D. (2017). Phosphorylation prevents in vitro myofibrillar proteins degradation by mu-calpain. Food Chem. 218, 455–462. doi:10.1016/j.foodchem.2016.09.048
Lin, G., Ning, H., Wang, G., Banie, L., Lue, T. F., and Lin, C. S. (2010). Effects of birth trauma and estrogen on urethral elastic fibers and elastin expression. Urology 76, 1018.e8–13. doi:10.1016/j.urology.2010.02.055
Liu, C., Wang, Y., Li, Y., Tang, J., Hong, S., and Hong, L. (2022). Dimethyl fumarate ameliorates stress urinary incontinence by reversing ECM remodeling via the Nrf2-TGF-β1/Smad3 pathway in mice. Int. Urogynecol J. 33, 1231–1242. doi:10.1007/s00192-021-05061-w
Liu, X., Wang, S., Wu, S., Hao, Q., Li, Y., Guo, Z., et al. (2018). Exosomes secreted by adipose-derived mesenchymal stem cells regulate type I collagen metabolism in fibroblasts from women with stress urinary incontinence. Stem Cell. Res. Ther. 9, 159. doi:10.1186/s13287-018-0899-9
Lommatzsch, M., Hornych, K., Zingler, C., Schuff-Werner, P., Hoppner, J., and Virchow, J. C. (2006). Maternal serum concentrations of BDNF and depression in the perinatal period. Psychoneuroendocrinology 31, 388–394. doi:10.1016/j.psyneuen.2005.09.003
Lukacz, E. S., Santiago-Lastra, Y., Albo, M. E., and Brubaker, L. (2017). Urinary incontinence in women: A review. Jama 318, 1592–1604. doi:10.1001/jama.2017.12137
Mammadov, R., Simsir, A., Tuglu, I., Evren, V., Gurer, E., and Özyurt, C. (2011). The effect of testosterone treatment on urodynamic findings and histopathomorphology of pelvic floor muscles in female rats with experimentally induced stress urinary incontinence. Int. Urol. Nephrol. 43, 1003–1008. doi:10.1007/s11255-011-9938-5
Marques, A., Stothers, L., and Macnab, A. (2010). The status of pelvic floor muscle training for women. Can. Urol. Assoc. J. 4, 419–424. doi:10.5489/cuaj.10026
Mcpherron, A. C., Lawler, A. M., and Lee, S. J. (1997). Regulation of skeletal muscle mass in mice by a new TGF-beta superfamily member. Nature 387, 83–90. doi:10.1038/387083a0
Millard, R. J., Moore, K., Rencken, R., Yalcin, I., Bump, R. C., and Duloxetine, U. I. S. G. (2004). Duloxetine vs placebo in the treatment of stress urinary incontinence: A four-continent randomized clinical trial. BJU Int. 93, 311–318. doi:10.1111/j.1464-410x.2004.04607.x
Mistry, M. A., Klarskov, N., Delancey, J. O., and Lose, G. (2020). A structured review on the female urethral anatomy and innervation with an emphasis on the role of the urethral longitudinal smooth muscle. Int. Urogynecol J. 31, 63–71. doi:10.1007/s00192-019-04104-7
Mitterberger, M., Marksteiner, R., Margreiter, E., Pinggera, G. M., Colleselli, D., Frauscher, F., et al. (2007). Autologous myoblasts and fibroblasts for female stress incontinence: A 1-year follow-up in 123 patients. BJU Int. 100, 1081–1085. doi:10.1111/j.1464-410X.2007.07119.x
Miyazato, M., Kaiho, Y., Kamo, I., Kitta, T., Chancellor, M. B., Sugaya, K., et al. (2009). Role of spinal serotonergic pathways in sneeze-induced urethral continence reflex in rats. Am. J. Physiol. Ren. Physiol. 297, F1024–F1031. doi:10.1152/ajprenal.00297.2009
Morgan, J. E., and Partridge, T. A. (2003). Muscle satellite cells. Int. J. Biochem. Cell. Biol. 35, 1151–1156. doi:10.1016/s1357-2725(03)00042-6
Mousavi, K., and Jasmin, B. J. (2006). BDNF is expressed in skeletal muscle satellite cells and inhibits myogenic differentiation. J. Neurosci. 26, 5739–5749. doi:10.1523/JNEUROSCI.5398-05.2006
Navaneethan, P. R., Kekre, A., Jacob, K. S., and Varghese, L. (2015). Vitamin D deficiency in postmenopausal women with pelvic floor disorders. J. Midlife Health 6, 66–69. doi:10.4103/0976-7800.158948
Ni, J., Li, H., Zhou, Y., Gu, B., Xu, Y., Fu, Q., et al. (2018). Therapeutic potential of human adipose-derived stem cell exosomes in stress urinary incontinence - an in vitro and in vivo study. Cell. Physiol. Biochem. 48, 1710–1722. doi:10.1159/000492298
Nomiya, M., and Yamaguchi, O. (2003). A quantitative analysis of mRNA expression of alpha 1 and beta-adrenoceptor subtypes and their functional roles in human normal and obstructed bladders. J. Urol. 170, 649–653. doi:10.1097/01.ju.0000067621.62736.7c
Norton, P. A., Zinner, N. R., Yalcin, I., Bump, R. C., and Duloxetine Urinary Incontinence Study, G. (2002). Duloxetine versus placebo in the treatment of stress urinary incontinence. Am. J. Obstet. Gynecol. 187, 40–48. doi:10.1067/mob.2002.124840
Omura, T., Sano, M., Omura, K., Hasegawa, T., Doi, M., Sawada, T., et al. (2005). Different expressions of BDNF, NT3, and NT4 in muscle and nerve after various types of peripheral nerve injuries. J. Peripher Nerv. Syst. 10, 293–300. doi:10.1111/j.1085-9489.2005.10307.x
Ozbek, E., Dursun, M., Otunctemur, A., Sami Cakir, S., and Can Polat, E. (2014). Stress urinary incontinence in premenopausal and postmenopausal women: Evaluation of serum estradiol levels and bone mineral density. Minerva Ginecol. 66, 293–298.
Pan, H. Q., Kerns, J. M., Lin, D. L., Sypert, D., Steward, J., Hoover, C. R., et al. (2009). Dual simulated childbirth injury delays anatomic recovery. Am. J. Physiol. Ren. Physiol. 296, F277–F283. doi:10.1152/ajprenal.90602.2008
Peters, K. M., Dmochowski, R. R., Carr, L. K., Robert, M., Kaufman, M. R., Sirls, L. T., et al. (2014). Autologous muscle derived cells for treatment of stress urinary incontinence in women. J. Urol. 192, 469–476. doi:10.1016/j.juro.2014.02.047
Phetfong, J., Tawonsawatruk, T., Kamprom, W., Ontong, P., Tanyong, D., Borwornpinyo, S., et al. (2022). Bone marrow-mesenchymal stem cell-derived extracellular vesicles affect proliferation and apoptosis of leukemia cells in vitro. FEBS Open Bio 12, 470–479. doi:10.1002/2211-5463.13352
Piculo, F., Marini, G., Barbosa, A. M., Damasceno, D. C., Matheus, S. M., Felisbino, S. L., et al. (2014). Urethral striated muscle and extracellular matrix morphological characteristics among mildly diabetic pregnant rats: Translational approach. Int. Urogynecol J. 25, 403–415. doi:10.1007/s00192-013-2218-4
Roche, R., Festy, F., and Fritel, X. (2010). Stem cells for stress urinary incontinence: The adipose promise. J. Cell. Mol. Med. 14, 135–142. doi:10.1111/j.1582-4934.2009.00915.x
Sangsawang, B. (2014). Risk factors for the development of stress urinary incontinence during pregnancy in primigravidae: A review of the literature. Eur. J. Obstet. Gynecol. Reprod. Biol. 178, 27–34. doi:10.1016/j.ejogrb.2014.04.010
Shinohara, M., Sumino, Y., Sato, F., Kiyono, T., Hashimoto, N., and Mimata, H. (2017). Tumor necrosis factor-alpha inhibits differentiation of myogenic cells in human urethral rhabdosphincter. Int. J. Urol. 24, 461–467. doi:10.1111/iju.13330
Siddle, N., and Versi, E. (2022). Stress urinary incontinence and the forgotten female hormones. Int. Urogynecol J. 33, 1711–1716. doi:10.1007/s00192-022-05178-6
Silwal Gautam, S., Imamura, T., Ishizuka, O., Lei, Z., Yamagishi, T., Yokoyama, H., et al. (2014). Implantation of autologous adipose-derived cells reconstructs functional urethral sphincters in rabbit cryoinjured urethra. Tissue Eng. Part A 20, 1971–1979. doi:10.1089/ten.TEA.2013.0491
Simon, M., Porter, R., Brown, R., Coulton, G. R., and Terenghi, G. (2003). Effect of NT-4 and BDNF delivery to damaged sciatic nerves on phenotypic recovery of fast and slow muscles fibres. Eur. J. Neurosci. 18, 2460–2466. doi:10.1046/j.1460-9568.2003.02978.x
Soderberg, M. W., Bystrom, B., Hammarstrom, M., Malmstrom, A., and Ekman-Ordeberg, G. (2010). Decreased gene expression of fibrillin-1 in stress urinary incontinence. Neurourol. Urodyn. 29, 476–481. doi:10.1002/nau.20735
Song, Q. X., Balog, B. M., Lin, D. L., Jiang, H. H., Gurel, V., Spaulding, J., et al. (2016). Combination histamine and serotonin treatment after simulated childbirth injury improves stress urinary incontinence. Neurourol. Urodyn. 35, 703–710. doi:10.1002/nau.22793
Song, Q. X., Chermansky, C. J., Birder, L. A., Li, L., and Damaser, M. S. (2014). Brain-derived neurotrophic factor in urinary continence and incontinence. Nat. Rev. Urol. 11, 579–588. doi:10.1038/nrurol.2014.244
Song, Y., Hong, X., Yu, Y., and Lin, Y. (2007). Changes of collagen type III and decorin in paraurethral connective tissue from women with stress urinary incontinence and prolapse. Int. Urogynecol J. Pelvic Floor Dysfunct. 18, 1459–1463. doi:10.1007/s00192-007-0356-2
Stewart, C. E., Newcomb, P. V., and Holly, J. M. (2004). Multifaceted roles of TNF-alpha in myoblast destruction: A multitude of signal transduction pathways. J. Cell. Physiol. 198, 237–247. doi:10.1002/jcp.10387
Tan, R., Pu, D., Cao, J., Ge, H., Chang, X., Ye, G., et al. (2018). Prevalence of stress urinary incontinence in women with premature ovarian insufficiency. J. Womens Health (Larchmt) 27, 1508–1512. doi:10.1089/jwh.2018.6935
Tang, J., Liu, C., Li, B., Hong, S., Li, Q., Wang, L., et al. (2019). Protective role of nuclear factor erythroid-2-related factor 2 against mechanical trauma-induced apoptosis in a vaginal distension-induced stress urinary incontinence mouse model. Oxid. Med. Cell. Longev. 2019, 2039856. doi:10.1155/2019/2039856
Ulmsten, U., and Falconer, C. (1999). Connective tissue in female urinary incontinence. Curr. Opin. Obstet. Gynecol. 11, 509–515. doi:10.1097/00001703-199910000-00017
Van Kerrebroeck, P., Abrams, P., Lange, R., Slack, M., Wyndaele, J. J., Yalcin, I., et al. (2004). Duloxetine versus placebo in the treatment of European and Canadian women with stress urinary incontinence. BJOG 111, 249–257. doi:10.1111/j.1471-0528.2004.00067.x
Vega, S. R., Kleinert, J., Sulprizio, M., Hollmann, W., Bloch, W., and Struder, H. K. (2011). Responses of serum neurotrophic factors to exercise in pregnant and postpartum women. Psychoneuroendocrinology 36, 220–227. doi:10.1016/j.psyneuen.2010.07.012
Wang, Y., Song, E. C., and Resnick, M. B. (2020). Elastin in the tumor microenvironment. Adv. Exp. Med. Biol. 1272, 1–16. doi:10.1007/978-3-030-48457-6_1
Wang, Y., Xu, H., Liu, X., Liu, L., and Liang, Z. (2011). Inhibition of fibroblast differentiation of muscle-derived stem cells in cell implantation treatment of stress urinary incontinence. Cell. Reprogr. 13, 459–464. doi:10.1089/cell.2010.0105
Wu, J. M. (2021). Stress incontinence in women. N. Engl. J. Med. 384, 2428–2436. doi:10.1056/NEJMcp1914037
Wu, R., Huang, C., Wu, Q., Jia, X., Liu, M., Xue, Z., et al. (2019). Exosomes secreted by urine-derived stem cells improve stress urinary incontinence by promoting repair of pubococcygeus muscle injury in rats. Stem Cell. Res. Ther. 10, 80. doi:10.1186/s13287-019-1182-4
Wu, Y., Zhang, L., Jin, H., Zhou, J., and Xie, Z. (2010). The role of calpain-calpastatin system in the development of stress urinary incontinence. Int. Urogynecol J. 21, 63–68. doi:10.1007/s00192-009-0988-5
Yang, S. J., Wang, J., Xu, J., Bai, Y., and Guo, Z. J. (2018). miR-93mediated collagen expression in stress urinary incontinence via calpain-2. Mol. Med. Rep. 17, 624–629. doi:10.3892/mmr.2017.7910
Yiou, R., Dreyfus, P., Chopin, D. K., Abbou, C. C., and Lefaucheur, J. P. (2002). Muscle precursor cell autografting in a murine model of urethral sphincter injury. BJU Int. 89, 298–302. doi:10.1046/j.1464-4096.2001.01618.x
Yoshimura, N., Kaiho, Y., Miyazato, M., Yunoki, T., Tai, C., Chancellor, M. B., et al. (2008). Therapeutic receptor targets for lower urinary tract dysfunction. Naunyn Schmiedeb. Arch. Pharmacol. 377, 437–448. doi:10.1007/s00210-007-0209-z
Yoshimura, N., and Miyazato, M. (2012). Neurophysiology and therapeutic receptor targets for stress urinary incontinence. Int. J. Urol. 19, 524–537. doi:10.1111/j.1442-2042.2012.02976.x
Yuan, H., Ruan, Y., Tan, Y., Reed-Maldonado, A. B., Chen, Y., Zhao, D., et al. (2020). Regenerating urethral striated muscle by CRISPRi/dCas9-KRAB-mediated myostatin silencing for obesity-associated stress urinary incontinence. Crispr J. 3, 562–572. doi:10.1089/crispr.2020.0077
Zhang, Z., Xu, P., Xie, Z., Shen, F., Chen, N., Yu, L., et al. (2017). Downregulation of AQP2 in the anterior vaginal wall is associated with the pathogenesis of female stress urinary incontinence. Mol. Med. Rep. 16, 3503–3509. doi:10.3892/mmr.2017.7014
Zhou, M., Li, B., Liu, C., Hu, M., Tang, J., Min, J., et al. (2021). M2 Macrophage-derived exosomal miR-501 contributes to pubococcygeal muscle regeneration. Int. Immunopharmacol. 101, 108223. doi:10.1016/j.intimp.2021.108223
Zhou, S., Zhang, K., Atala, A., Khoury, O., Murphy, S. V., Zhao, W., et al. (2016). Stem cell therapy for treatment of stress urinary incontinence: The current status and challenges. Stem Cells Int. 2016, 7060975. doi:10.1155/2016/7060975
Keywords: stress urinary incontinence, molecular pathogenesis, stem cell therapy, gene regulation, exosome differentiation
Citation: Wang X-x, Zhang L and Lu Y (2023) Advances in the molecular pathogenesis and cell therapy of stress urinary incontinence. Front. Cell Dev. Biol. 11:1090386. doi: 10.3389/fcell.2023.1090386
Received: 08 November 2022; Accepted: 30 January 2023;
Published: 08 February 2023.
Edited by:
Arjun Singh, Memorial Sloan Kettering Cancer Center, United StatesReviewed by:
Alvaro Munoz, University of Guadalajara, MexicoCopyright © 2023 Wang, Zhang and Lu. This is an open-access article distributed under the terms of the Creative Commons Attribution License (CC BY). The use, distribution or reproduction in other forums is permitted, provided the original author(s) and the copyright owner(s) are credited and that the original publication in this journal is cited, in accordance with accepted academic practice. No use, distribution or reproduction is permitted which does not comply with these terms.
*Correspondence: Ye Lu, songqinlu@sina.com
Disclaimer: All claims expressed in this article are solely those of the authors and do not necessarily represent those of their affiliated organizations, or those of the publisher, the editors and the reviewers. Any product that may be evaluated in this article or claim that may be made by its manufacturer is not guaranteed or endorsed by the publisher.
Research integrity at Frontiers
Learn more about the work of our research integrity team to safeguard the quality of each article we publish.