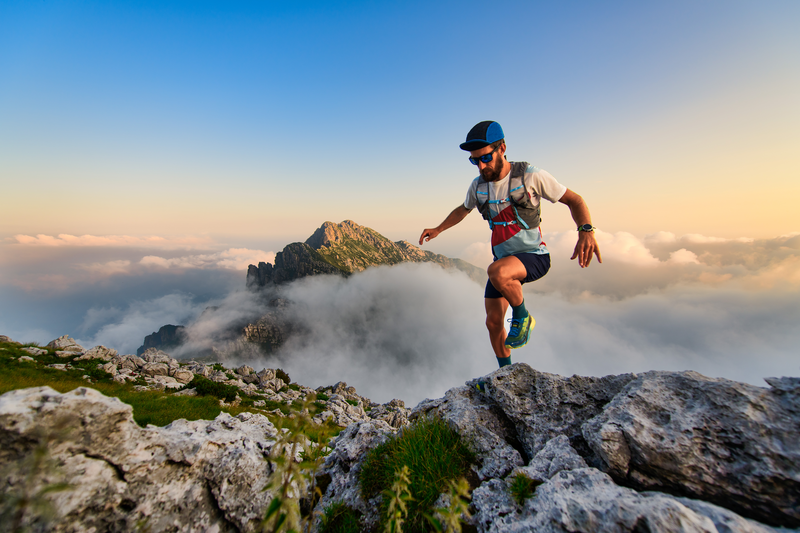
94% of researchers rate our articles as excellent or good
Learn more about the work of our research integrity team to safeguard the quality of each article we publish.
Find out more
REVIEW article
Front. Cardiovasc. Med. , 02 June 2022
Sec. General Cardiovascular Medicine
Volume 9 - 2022 | https://doi.org/10.3389/fcvm.2022.897106
As a leading cause of mortality and morbidity worldwide, cardiovascular disease and its diagnosis, quantification, and stratification remain significant health issues. Increasingly, patients present with cardiovascular disease in the absence of known risk factors, suggesting the presence of yet unrecognized pathological processes and disease predispositions. Fortunately, a host of emerging cardiovascular biomarkers characterizing and quantifying ischaemic heart disease have shown great promise in both laboratory settings and clinical trials. These have demonstrated improved predictive value additional to widely accepted biomarkers as well as providing insight into molecular phenotypes beneath the broad umbrella of cardiovascular disease that may allow for further personalized treatment regimens. However, the process of translation into clinical practice – particularly navigating the legal and commercial landscape – poses a number of challenges. Practical and legal barriers to the biomarker translational pipeline must be further considered to develop strategies to bring novel biomarkers into the clinical sphere and apply these advances at the patient bedside. Here we review the progress of emerging biomarkers in the cardiovascular space, with particular focus on those relevant to the unmet needs in ischaemic heart disease.
The burden of cardiovascular disease (CVD) has steadily increased over the past 30 years, contributing to significant morbidity and mortality (1). Often diagnosis occurs late in the disease process when considerable irreversible damage has already occurred during the subclinical phase (2). In the case of coronary artery disease (CAD), physicians and individual community members are limited to measuring risk factors that increase the probability of developing atherosclerotic disease and cardiovascular events such as cholesterol levels, blood pressure, and diabetes. However, up to 27% of first time heart attack patients have no standard modifiable risk factors (3). Furthermore, many individuals with intermediate to high risk have substantial variation in their susceptibility to disease (4–6). Thus, a biomarker of atherosclerosis itself, and particularly the more vulnerable plaque features would be the “holy grail” for tackling CAD. As our understanding of the complexity of heart disease increases, our ability to detect and quantify those complexities needs to increase accordingly. More and more we are recognizing CVD as multiple interrelated processes with a spectrum of phenotypes, rather than a simple linear progression of disease (7). Additionally, if it were to relate directly to the burden of disease in a given patient, the biomarker could guide individualized therapeutic interventions, furthering the transition toward personalized medicine. Many current biomarkers have been discovered due to their pathophysiological significance in CVD, however further discovery work has been augmented by the use of omics, unbiased analyses, and other high throughput technologies (8, 9). This manuscript gives an overview of emerging cardiac biomarkers and biomarker panels measurable in simple peripheral blood samples and at various stages of the translational pipeline, and considers the commercialization landscape that shapes this. Following this is a discussion of the feasibility, challenges, and opportunities present in the contemporary cardiovascular biomarker field, particularly in relation to subclinical disease detection and the commercial considerations surrounding regulation and patenting. The latter has particular importance to understanding pathways accelerating patient access to the potential benefits of novel biomarkers.
In this section we aim to summarize emerging cardiovascular biomarkers, their discovery, validation and commercialization journey. These have been grouped by their associated pathophysiological processes – atherosclerosis and platelet dysfunction, myocardial ischaemia, fibrosis and remodeling, deranged hemodynamics and contractility, and cardiometabolic disease – visualized in Figure 1. The interplay between these categories is substantial, and the exact pathophysiological processes of many biomarkers are still being elucidated. Recent patents held in these areas are summarized in Table 1. In some advanced areas, panels of multiple biomarkers have been used to compute scores focused on guiding specific treatment decisions, as further detailed in Section Biomarker Panels for Guiding Therapy.
Figure 1. Mechanisms of cardiovascular disease – components modified from (10) with permission.
Approximately three quarters of acute coronary events are linked to atherosclerotic plaque rupture (35). Despite this, assessing individualized risk of rupture remains a challenge. Myriad radiographic and percutaneous methods exist to quantify plaque burden, each with its own limitations (36–38). Blood biomarkers quantifying degree of atherosclerosis, plaque stability, and deranged platelet function have been proposed that may give a more detailed and personalized assessment of occlusive risk without requiring invasive procedures, or better identify those who would benefit from these procedures.
The extracellular matrix proteinase, matrix metalloproteinase-9 (MMP-9), is overexpressed in stressed endothelium and is a known contributor to plaque architecture and composition (39). MMP-9 is also correlated with vascular endothelial growth factor (VEGF), a neovascularisation stimulus that further destabilizes plaque. Virtual histology-intravascular ultrasound is an invasive technique using radiofrequency backscatter patterns on an intravascular probe to measure coronary plaque burden, composition, and vulnerability (40). Elevated levels of MMP-9 are independent predictors of unstable coronary plaque; in fact, virtual histology-intravascular ultrasound during coronary angiography in 32 patients with stable coronary disease found unstable coronary plaque properties, including increased necrotic core volume and fibro-fatty content, in patients with more elevated levels of MMP-9 (11). Circulating levels of MMP-9 have also been correlated with femoral intima-media thickness, though there were mixed results regarding carotid plaques (35, 41).
In a cohort of 343 ACS patients requiring ICU admission, MMP-9 levels predicted short-term mortality while both its levels and rate of change during recovery predicted likelihood of a second major cardiac event over a 6 year follow up period (42). Likewise, in a cohort of 866 middle-aged participants apparently free of coronary disease, baseline plasma MMP-9 was associated with first-time coronary disease over an 8 year follow up, independent of known risk factors, high sensitivity CRP (hsCRP), and IL-6 (43).
Adiponectin is a peptide hormone derived from adipose tissue that has protective cardiometabolic effects (44). This is achieved through various mechanisms including promoting insulin sensitivity and modulating lipid and glucose metabolism (45). A cross-sectional study of 284 patients found lower serum adiponectin levels were associated with metabolic syndrome status, even when confounding factors (e.g., serum lipid and insulin levels) were controlled (12). This relationship is significant given the predictive value of metabolic syndrome in the development of arteriovascular events (46).
Serum adiponectin levels are thought to also have a direct limiting effect on atherosclerosis by inhibiting key processes in atheroma formation. This includes improving endothelial cell function and suppressing smooth muscle proliferation and foam cell formation (45). A case-control study of 306 patients (101 cases, 205 controls) supports these properties as lower serum adiponectin levels were found to be associated with the progression of coronary artery calcification (13).
P-selectin is a cell-surface adhesion molecule expressed on endothelium, platelets, and leukocytes, and has been implicated in plaque development (47). In particular, platelet release of P-selectin complexes with P-selectin glycoprotein ligand-1 (PSGL-1) on leukocytes (48) activates pro-thrombotic pathways, including upregulation of tissue factor and stabilization of platelet-platelet interactions required for aggregation. In a cohort of 345 apparently healthy women, participants with elevated soluble P-selectin were at a 2.2-fold higher risk of having a CVD event than age- and sex-matched counterparts at 3.5 year follow up, independent of other risk factors (14). Similarly, in a cohort of 142 patients under 55 years old with known CAD, platelet P-selectin levels were significantly higher in ACS than stable angina, which in turn were higher than healthy controls (15).
Lectin-like oxidized low-density lipoprotein receptor 1 (LOX-1) is physiologically expressed in low amounts on endothelial cells, and sits upstream of several matrix metalloproteinases and pro-inflammatory pathways implicated in atherosclerosis (49). Receptor levels markedly increase in states of inflammation, with expression also spreading to smooth muscle and macrophages. Further, inflammatory stimuli such as TNF-α, CRP, and various interleukins trigger shedding of LOX-1 into a soluble form (sLOX-1) which has been used as a marker for a number of cardiac and vascular endpoints. sLOX-1 has been correlated with complexity of coronary lesions, number of vessels with disease, and is elevated in ACS compared to stable coronary disease (16).
The prospect of developing an atherosclerotic stability marker or series of markers detectable in peripheral blood may open the door to applying risk stratification or population-based screening estimating risk of plaque rupture or other myocardial event. It remains to be investigated whether these markers used in combination may give additive insight into predictability of short-term or long-term plaque rupture.
In addition to its key role in the development of atherosclerosis and vascular dysfunction, inflammation is also seen early in response to myocardial ischaemia. Crucially, these states may be present prior to ACS or within the first minutes from onset, making inflammatory mediators particularly promising cardiovascular biomarkers for detection of acute cardiac events (50).
Macrophage migration inhibitory factor (MIF) is an inflammatory cytokine involved in the early myocardial response to ischaemia (51). In a mouse infarct model, MIF levels were elevated at the earliest recorded point – within 15 min of occlusion and prior to evidence of infarction – and remained elevated at the 60 min mark. In a human study of 374 ST-elevation myocardial infarction (STEMI) patients, of all biomarkers measured on admission including troponins, only MIF was correlated with infarct size, ejection fraction, and ventricular volumes on cardiac MRI at the 3-day and 3-month mark (17).
Nourin is a potent chemoattractant peptide released by ischaemic myocardium within 5 min of onset (52). In a cohort of patients being investigated for inducible ischaemia with stress echo or stress ECG testing, a battery of Nourin-dependent miRNAs were measured and compared to both healthy controls and a STEMI group. Two particular miRNAs, miR-137 and miR-106b-5p both showed marked elevation at baseline in those with positive stress tests, with >1,000- and 100-fold changes respectively (18). In the same study, levels of miR-137 were significantly higher in STEMI patients compared to the positive stress test group, suggesting capacity to stratify between normal, ischaemic, and infarcting myocardium. The role of these miRNAs in the heart is a matter of ongoing investigation, but there is established evidence for miR-137 as anti-inflammatory and anti-oxidant in neuronal tissue during ischaemic stroke (53).
The established inflammatory “prodrome” preceding acute myocardial events presents an opportunity to detect – and subsequently manage – myocardial ischaemic events in the earliest stages of injury (50). As this inflammatory picture shifts to mirror chronic disease processes, other markers such as phenylalanine and novel miRNAs above may provide prognostic value in the non-acute ischaemic heart.
The heart's response to ischaemic insult through fibrosis and subsequent remodeling is a principal determinant of it's long-term function (54). The ability to stratify or predict this response prior to its development will become increasingly important in guiding management as promising anti-fibrotic cardiac therapies move closer to the clinical sphere.
Osteopontin (OPN) is a is a glycoprotein expressed in various cardiac cells that may exist in either an “immobilized” state bound to the extracellular matrix, or as a soluble cytokine mediating inflammatory integrin activation (55). This ability to translocate may imply a messenger role in the remodeling response to physiological and pathological stimuli – accordingly, OPN has been found to be elevated in several animal models in response to biomechanical cardiac stress. As a biomarker, OPN showed diagnostic utility in a cohort of 420 HFrEF patients as well as a strong correlation with NYHA disease severity classification (19). Further, multivariate analyses in the same study found elevated levels to carry a hazard ratio of 2.3 for prediction of 4-year mortality even after accounting for clinical and biochemical features including NT-proBNP.
Osteopontin also triggers the secretion of Galectin-3 (Gal-3), a pro-fibrotic agent released from macrophages (56). In fact, Gal-3 has already seen recognition clinically by the FDA in the prognostication of chronic heart failure (57). Serial echocardiography in 240 advanced heart failure patients (NYHA 3/4) showed Gal-3 predicted change in LV end-diastolic volumes at 3 months compared to baseline, while no such similar significant trend was identified for NT-proBNP (20). Thus Gal-3 has a putative role in detection of both heart failure and acute myocardial infarction – the latter being more controversial in published literature (58).
High sensitivity cardiac troponin is one of the most widely used cardiac biomarkers in current clinical practice (59). Its role in diagnosis of myocardial infarction, myocarditis, and other acute cardiac events is well established. More recently, measurement of post-translation modification of troponins has been shown to reflect the degree of compromise in their function as contractile elements. In particular, phosphorylation of troponin T at serine Ser-207 has been shown to correlate with degree of left ventricular remodeling on echocardiography at 1 year follow up after myocardial infarction (22). Importantly, these blood biomarker changes were observable within the first week post-infarct, well before the echocardiographic changes that they predicted. A separate patent is held for nitrated cardiac troponin I, the result of reactive nitrogen species-driven post-translational modification which purportedly predicts myocardial ischaemia with or without infarction [patent US10175250B2]. It is reportedly detectable immediately after reperfusion of balloon-induced ischaemia in a pig model of left circumflex occlusion, however further publications on this beyond patent data are lacking.
The essential amino acid phenylalanine has been shown play a role in normal cardiac aging, cellular senescence, and fibrosis. Cell culture studies suggest a causative effect between phenylalanine and heart disease in mouse and human models through promoting aging-related redox and epigenetic changes (60). A recent human study in patients admitted to hospital with critical heart failure showed that elevated phenylalanine predicted 1 year all-cause mortality independent of several traditional risk factors (age, atrial fibrillation, cholesterol), mortality scores (SOFA, APACHE II), and inflammatory markers (CRP, transferrin, IL-8, IL-10) (21). Levels >112 μM carried a mortality hazard ratio of 5.06, and a hazard ratio of 2.57 after accounting for the above risk factors. Interestingly, inflammatory states have been shown to attenuate phenylalanine catabolism, suggesting a causative link between pro-inflammatory states and phenylalanine-mediated fibrotic risk (61).
Cardiac fibrosis is classically an irreversible step in the progression of disease with therapies only indirectly managing hemodynamics and fluid status (62). However emerging antifibrotic therapies suggest a growing role for quantifying and characterizing the degree of an individual's fibrotic state, potentially guiding therapy as well as providing prognostic value (63, 64).
Compromised myocardial contractility and/or relaxation is a hallmark of established cardiac disease (65). In fact, it is the sole finding in asymptomatic left ventricular dysfunction (ALVD), or “pre-heart failure,” a condition lacking appropriate screening tools and known to progress to true heart failure (66). ALVD has been found to have a prevalence of 6.8% in a random population of 75 year olds, and more commonly in men and those with coronary artery disease, hypertension, or ECG changes (67). With ALVD carrying a four-fold increase in mortality over 6 years, and cardiac contractility being a driving force behind heart failure symptoms particularly in HFrEF, quantification of contractility and the molecular mechanisms underpinning it are paramount.
Circular RNAs are particularly promising blood biomarkers due to their resistance to degradation compared to other elements of the blood transcriptome (68). Myocardial infarction-associated circular RNA (MICRA) is a circular RNA (circRNA) elevated during myocardial infarction that has shown particular prognostic value. The functional or pathophysiological role of MICRA remains to be fully elucidated, but experimental evidence is promising – in a cohort of 472 acute MI patients, MICRA levels at reperfusion correlated with ejection fraction at 4 months (23). A number of further circRNAs have been implicated in ischaemia/reperfusion, cardiac senescence, fibrosis, and remodeling, making for a promising area for future exploration (69).
ALVD is a recognized precursor to symptomatic heart failure, diagnosable through imaging studies such as via the application of echocardiography and tissue doppler techniques that are impractical to apply at an asymptomatic population screening level (70). A panel of seven genes characterized in peripheral blood may provide a biomarker signature of pre-clinical heart failure (24). Analysis of the leucocyte transcriptome in 294 patients without overt heart failure revealed that levels of FECH, TMEM79, FBXW7, NGFB, ALK, UBN1, and SLC43A2 in peripheral blood were able to predict ALVD with 87% accuracy and 100% precision. These genes encode for membrane proteins, mitochondrial components, and transcription factors not previously associated with heart disease prognosis. In contrast, NT-proBNP is useful in ruling out ALVD but shows poor positive predictive value (71).
The protein angiopoietin-2 (Ang2) is a complex mediator of angiogenesis with seemingly mixed pro- and anti-angiogenic activity depending on its molecular context (72, 73). Ang2 also mediates pericyte detachment to alter vascular permeability in states of inflammation. Conversely, thrombospondin-2 (TSP2) is a potent anti-angiogenic factor with additional function in regulating myocardial matrix integrity (74, 75).
In a large population of 1,315 patients, proteomic analyses identified Ang2 and TSP2 as two particularly promising biomarker candidates across multiple cohorts. In dyspnoeic patients presenting to the emergency department, both were independently useful in distinguishing acute heart failure from alternative causes of dyspnoea after accounting for NT-proBNP level (25). Secondly, in a longitudinal study of 768 patients in the community without known heart disease, both markers predicted development of heart disease at 20 year follow up with hazard ratios of 1.36 (Ang2) and 1.29 (TSP2) per standard deviation of biomarker level, again independent of NT-proBNP (25). Interestingly, in the same study levels of both biomarkers declined significantly – by approximately 80% – in a third cohort of post-cardiac transplant patients; implying these are true biomarkers of local cardiac disease rather than surrogates reflective of systemic illness or indicative of comorbidities.
Growth hormone (GH) and insulin-like growth factor 1 (IGF-1) are involved in intracellular calcium handling, myocardial contractile proteins, and systemic vascular function (76). IGF-1 binding protein 2 (IGFBP2) is a downstream regulator of IGF-1 at both the tissue and blood level, and in contrast to the more abundant IGFBP1, levels of IGFBP2 exhibit less post-prandial fluctuation (77). Analysis of plasma from 870 patients across multiple cohorts – two cohorts of outpatient chronic stable HF and 1 of patients presenting to the emergency department with acute decompensated HF – revealed positive associations with mortality, cardiac transplant, or need for LVAD (26). Importantly, a combined model of IGFBP2 and NT-proBNP produced stronger associations with these endpoints than using NT-proBNP alone. IGFBP2's role in CVD may be multifactorial, with associations also shown with intima-media thickness and myocardial infarction, while data on cardiometabolic endpoints such as obesity and metabolic syndrome paradoxically show negative correlations with IGFBP2 in literature (78–80).
Taken together, these markers are promising as a means to assess ventricular function across a range of patient groups. The above trials demonstrate potential in asymptomatic, known heart failure, and post-infarct cohorts. Further exploration of these molecules may also provide mechanistic insights into phenotypes such as ALVD and HFpEF, which are poorly understood (81).
Metabolic syndrome is a key culprit in the modern-day heart disease phenotype (82). Diabetes, obesity, and subjective assessment of lifestyle factors capture only a subset of cardiometabolic disease and may be undetected before established cardiovascular damage. The intracellular manifestations of these changes center around mitochondrial dynamics, energy handling, and lipid modifications, and are promising candidates for metabolic biomarkers.
Lipids are implicated in atherosclerosis both locally in foam cells and the necrotic lipid core, and systemically as mediators of inflammation (83). In a cohort of 220 that compared healthy patients to those with stable and unstable angina, liquid-chromatography mass-spectrometry generated a panel of 105 lipids which improved predictive power in disease state classification when used alongside traditional risk factors (27). Sub-analysis of component lipids revealed that some may correlate with plaque burden, whilst others relate to plaque instability. For example, the lipid groups alkylphosphatidylethanolamine [PE(O)] and phosphatidylethanolamine plasmalogen [PE(P)] were only significant in the unstable CAD group with no difference seen between the control and stable CAD groups.
Mitochondria account for almost a third of the cardiomyocyte by volume – in keeping with their essential role in both cardiac health and disease (84). LIPCAR is a mitochondrial long non-coding RNA (lncRNA) shown to be elevated in chronic HFrEF, and is positively correlated with cardiovascular mortality in this cohort (28). Interestingly, LIPCAR levels were lower at baseline following infarction in patients who underwent more significant LV remodeling. This trend reversed in the following 1–12 month period, with increased LIPCAR in extensively-remodeled patients implying a dynamic role of LIPCAR in the natural history of cardiac remodeling. Non-coding mitochondrial microRNAs (mitomiRs) have also shown promise as biomarkers, possibly secondary to their post-transcriptional regulation of mitochondrial functions including apoptosis, calcium homeostasis, and energy metabolism (85, 86). Plasma profiling studies of 206 patients demonstrated 7 mitomiRs (miR-18a-5p, miR-26b-5p, miR-27a-3p, miR-30e-5p, miR-106a-5p, miR-199a-3p, and miR-652-3p) that both predicted 180-day mortality in heart failure, and differentiated acute HF from acute exacerbation of COPD (29).
Oxidative stress and lipid peroxidation have been implicated in atherogenesis in multiple studies by contributing to endothelial dysfunction and being a strong predictor of CAD events (87, 88). However, measurements of dysregulated oxidative signaling have been challenging. This has substantial implications for the translation of any novel antioxidant supplements or therapies (89), as an ideal clinical trial would be targeting individuals with high “oxidative stress,” and have a biomarker that could measure response to therapy. Biomarkers of dysregulated oxidative signaling may also have advantages for detection of early cardiometabolic disease given its integral role as a downstream mediator not only of many traditional risk factors but also of many emerging atypical drivers of disease (87). Malondialdehyde (MDA) is formed during lipid peroxidation and has been associated with endothelial dysfunction and CAD events (30, 90, 91). MDA breakdown can form malondialdehyde-acetaldehyde (MAA) adducts which contribute to atherogenesis through its proinflammatory, cytotoxic and potential protein modulating effect (30, 92). A cross-sectional study of 236 patients demonstrated that detection of different antibody isotypes to MAA adducts were associated with separate CAD states; for example, higher levels of anti-MAA IgM and IgG antibodies were associated with acute myocardial infarction, whilst CABG patients had comparatively higher anti-MAA IgA antibodies (30).
Homoarginine is a nonproteinogenic amino acid that is suspected to have a protective cardiometabolic effect (31, 93, 94). The mechanism underlying this effect is unknown, although it is suspected that this association is driven by homoarginine-mediated nitric oxide production and subsequent protective vascular effects. Several studies have supported the potential the protective cardiometabolic effect of homoarginine through correlation (93), most notably in a cohort study of 3,305 patients finding low homoarginine levels were associated with increased cardiovascular and all-cause mortality (31). The study also demonstrated homoarginine levels were inversely related to markers of endothelial dysfunction (e.g., ICAM-1 and VCAM-1).
A more recent study of 2,106 patients reported several of the same correlations, however, when a Mendelian randomization approach was instituted, no significant causal associations of cardiometabolic or vascular protective effects were observed (95). Although the evidence surrounding homoarginine's role as a cardiac biomarker is debated (95), recent reviews suggest there may still be scope for its clinical use (93).
These markers stand to carry increasing importance given the growing recognition of multiple phenotypes within cardiometabolic disease (96). As such, characterizing the specific mechanisms involved in a given patient's disease alongside their genetic predispositions may become a larger part of clinical practice, prognostication, and management of CVD.
Biomarkers are applied across the full spectrum of clinical cardiovascular care. In the case of coronary artery disease and ischaemic heart disease, this extends from primary prevention to the diagnosis and risk assessment of acute coronary syndrome, to secondary prevention. Currently, screening in primary care is focused on traditional risk factors such as hypertension and dyslipidaemia, but there is an unmet need for markers that integrate these with the host response. Screening for risk factors and coronary artery disease may help target efforts in patient health education and behavior modification early in disease processes. In the setting of an acute coronary syndrome, some markers are focused on accurate diagnosis (97, 98). Currently, troponin, reflecting heart muscle damage, lacks the specificity to diagnose an atherosclerotic mechanism that would benefit from invasive angiography and percutaneous intervention, vs. potential mimic conditions such as Takotsubo cardiomyopathy or myocarditis. Improvements in single or combination biomarkers may help avoid unnecessary coronary angiography, particularly if combined with non-invasive imaging modalities (99). In general, for secondary prevention for IHD markers are currently similar to those used in primary prevention screening, although clinical action is more rigorous, with aggressive targets for pharmacological management of LDL cholesterol and BP in patients who have already suffered a major adverse cardiovascular event. Biomarkers may also be beneficial in tertiary prevention settings where the clinical application of said biomarker is related to therapeutic monitoring, such as in the case of BNP to monitor heart failure management (97, 98). Many of these scenarios open the opportunity for allied health professionals, such as clinical nurse consultants, to utilize biomarkers within the scope of their outpatient clinical practice, following guidelines for adjusting therapies and identifying warning signs.
The classical “one size fits all” approach to CVD treatment based on early epidemiological data stands to be replaced by precision medicine, with therapies tailored to the individual patient (100). The growing field of pharmacogenomics emphasizes the need for therapy informed by both patient and disease factors, and with this comes the need to move toward utilizing integrated biomarker tools as adjuncts to clinical decision making. This section discusses biomarker panels which generate clinical scores to assist in developing personalized therapeutic regimens.
Where many major cardiac risk factors including diabetes, hypertension, and dyslipidaemia all have observable outcomes to guide treatment regimens, current standard of practice lacks the ability to practically measure case-by-case potency of antiplatelet therapy (101, 102). The epidemiology of clinically relevant resistance is controversial and poorly characterized but ‘laboratory' resistance has been found in meta-analyses to be present in 23%−40% of cases, based on tools such as light transmission aggregometry, impedance aggregometry, or platelet function analysers (103). Thus aspirin resistance often goes undetected, which is particularly concerning given its association with other comorbidities such as insulin resistance (104). A panel of 65 proteins or their associated RNAs measured in peripheral blood has been developed as an “aspirin response signature,” six of which were incorporated into a platelet function score reflecting response to aspirin (32). This signature was also correlated with adverse outcomes of myocardial infarction and death independent of other risk factors, implying identification of an aspirin-resistant population with a subsequent increase in adverse outcomes.
Another panel proposes 16 biomarkers to guide the choice of antihypertensive pharmacotherapy in the context of heart failure. Several of these have already been shown to have prognostic value such as BNP, NT-proBNP, ST2, Gal-3 or cardiac troponins (105, 106). An individual patient data meta-analysis of 2,000 individuals found that heart failure therapy agent or dose guided by natriuretic peptide levels improved all-cause mortality in patients under 75 when compared to standard clinical care (107). Whilst the strength of this evidence is debated (105), the potential utility of multi-marker approaches is being explored to improve prognostic accuracy (108). For example, a cohort study of 195 patients found a combination of sST2 and BNP improved prognostic accuracy in HFrEF patients (109). The merits of using a multimarker approach thus appears sound as a method to guide the intensity of heart failure therapy (105).
Furthermore, there may be scope for this biomarker panel to guide the choice of pharmacotherapy. An analysis of 499 patients from the TIME-CHF study showed the relationship between different therapies and biomarkers (33). One of the significant findings included that high cystatin C levels were associated with improved outcomes (mortality and hospitalization) in patients treated with higher spironolactone doses. The combination of all these biomarkers as part of broader multimarker panel may present significant clinical utility in progressing personalized heart failure therapies.
Cardiac resynchronisation therapy (CRT) – when successful – may improve quality of life, mortality, hospitalization, and NYHA class in select HF patients, and even partially reverses detrimental cardiac remodeling (110, 111). A number of trials have attempted to identify demographic factors that predict response to CRT, with modest success (112–114). A panel made up of 4 markers: soluble ST2 (sST2), soluble tumor necrosis factor receptor-II (sTNFr-II), matrix metalloproteinase-2 (MMP-2), and CRP have been combined into the Biomarker-CRT score to predict likelihood of a favorable response from peripheral blood (34). Just over half of study participants had a positive CRT response, while those with the lowest score of 0 were more than five times more likely to respond than those with the maximum score of 4. This effect was independent and additive to clinical and demographic factors.
Lipid-lowering statin therapy is widely used in cardiovascular secondary prevention, making for one of the most widely prescribed drugs in the world (115). A lipidomic analysis of over 10,000 samples from 5,000 individual in the Long-Term Intervention with Pravastatin in Ischaemic Disease (LIPID) study, a large clinical trial of statin efficacy in secondary prevention, identified a two lipid signature, PI(36:2)/PC(38:4), that could stratify people into those who received a reduction in risk in response to statin treatment and those who did not (116). With this study also demonstrating as many as 25% of patients not receiving any measurable cardiovascular risk reduction from pravastatin therapy, these types of risk markers hold promise for a more personalized approach to risk stratification within the existing risk management framework.
Commercialisation pathways play a critical role in allowing for the rigorous translation of biomarkers or other medical innovations to the clinic (117). Ultimately, the pathway ensures clinical efficacy and utility, optimizes methods for measurement, defines standard operating protocols for the assay, identifies the market, and provides the service. Partnership between researchers in academia and industry is critical for the success of this translational pipeline. Traditionally patents were awarded for individual biomarkers and their application to diagnosis or risk prediction in particular conditions. However, increasingly patents covering the measurement of naturally occurring molecules have been challenged.
The ability to patent biomarkers and consequently enforce said patents is a complex issue that has undergone significant recent regulatory changes, and vary between countries and regulatory bodies. Notably in the United States, three key decisions in Mayo v Prometheus, AMP v Myriad Genetics and Alice Corp. v CLS Bank International placed significant restrictions on what biomarker claims could be patented (118).
• The Mayo decision of 2012 highlighted how natural processes (in this case being the measurement of a metabolite to titrate drug dose) cannot be patented (119)
• The Myriad decision of 2013 demonstrated that naturally occurring products (in this case the detection of DNA fragments) cannot be patented (120).
• The Alice decision of 2014 demonstrated that abstract ideas (in this case being the use of technology to exchange financial information) cannot be patented (121).
Thus, current and future claims will need to pass three tests to be patent eligible: first, that it differs from nature or natural processes; second, that it consists of inventive concepts (i.e., not abstract ideas or natural laws); and third, that it differs significantly from routine clinical practice (122). Despite these barriers, subsequent decisions demonstrate patents are still achievable, albeit with claims requiring increased specificity to be granted – for example, patents granted between 2014 and 2018 (the time since these landmark decisions) in personalized oncology diagnostics have increased (118). Interestingly, there has been a shift in the presentation of these patents with the majority of new claims in this field being tied to therapeutic methods (53%). The trend of expanding patents to also include changes to treatment algorithms rather than only including diagnostic biomarkers has been rising since 2012, reflecting the changing legal precedence, particularly after the Mayo decision and the resulting requirements put on prospective patent holders (118). This trend in patent claim construction is also demonstrated in the field of cardiac biomarkers as detailed in Table 1.
In addition to patents, there are alternative pathways for a novel marker being implemented in the clinic that include licensing of “know how” and platforms or tools to rigorously measure the marker and communicate results to physicians and patients. As detailed in Table 1, some of these measures utilized in the field of cardiac biomarkers include decision matrices (MMP-9, adiponectin), computerized biomarker risk algorithms (angiopoeitin-2, anti-hypertensive panel), means of monitoring therapeutic effectiveness (phenylalanine, MICRA, mitomiR panel, aspirin response signature) and small molecules implicated in cardiovascular pathophysiology (galectin-3, thrombospondin-2, homoarginine). However, more complex biomarker panels particularly in the fields of metabolomics and lipidomics where panels may contain dozens to hundreds of individual metabolites are likely to also depend heavily on “know how” of both the measurement and the algorithm, to drive the commercialization process (123). Whether or not the more complex algorithms themselves will represent a patentable form of intellectual property remains to be determined as this field develops over coming years. In either event, it will be important to develop a viable commercialization pathway for these developments if we are to maximize the health benefits from this technology.
Despite restrictions on patenting biological processes, there remain a number of pathways to commercially incentivise emerging biomarkers. Patents may still be taken out on inventions that utilize biomarkers to produce clinically relevant results, including assays. An example of this is the cardiac troponin – no single patent is held on its detection but a number of “high sensitivity” assays have been patented by separate groups over the years, resulting in increasing sensitivity through a competitive commercial environment while still providing financial incentive (124). This has implications not only in earlier detection of acute myocardial infarctions but also opens the door for its use in a wide range of diseases involving myocardial strain or insult including heart failure, pulmonary embolism, and sepsis (125).
Similarly, novel platforms for detection and quantification emphasizing rapid delivery of results are also grounds for patenting. Aptasensors are a form of biosensors using highly specific oligonucleotides or short peptides to bind to a target analyte and produce a signal through a transducer that can then be quantified (126). Aptasensors provide several advantages over classical antibody assays including a broader range of potential analytes, portability, and the potential for point-of-care testing designs (127). Again, troponins are a chief candidate for timely detection in point-of-care or out-of-hospital settings (128), and other markers such as BNP, IL-6, and CRP have also been had aptasensor patents developed [WO2017062349A1]. More conventional lateral flow assays have also shown promise in both qualitative and quantitative point-of-care applications (129).
Translation of biomarkers from the laboratory into the clinical sphere brings with it a number of technical challenges. The most obvious of these is validation of laboratory findings in relevant clinical cohorts in a reproducible manner, but there are several other hurdles to consider (130). Detection of a given marker should be timely and financially practical – this is particularly important in markers of ischaemia and infarction where there is a well-recognized correlation between time-to-treatment and clinical outcomes (131). To this end, the development of a routinely available assay that is feasible to use outside of a research setting requires significant assay optimisation, while a number of point-of-care technologies such as aptasensors and lateral flow tests show promise in rapid quantification of biomarkers including troponins (129, 132, 133).
Broadly speaking, a given biomarker should provide additional predictive value over established tests, typically requiring improved sensitivity and/or specificity to be demonstrated (134). However given the heterogenicity of pathophysiology in cardiac disease, markers that are less sensitive or specific than established markers may still complement them by providing information on different mechanisms of disease and prognosticate, stratify, or otherwise guide treatment in particular subgroups that are otherwise missed (135). Thus “panel” based markers provide an opportunity for combining biomarkers into a multi-mechanism approach. Beyond this, biomarkers guiding treatment necessitate not only improved predictive value but also a demonstration of improved outcomes for patients in a clinical setting. This is particularly challenging given the timeframes required to observe disease progression and long-term consequences of therapy. Researchers and research groups equipped with the skillset and resources for biomarker discovery and assay development may not be the same as those with access to clinical populations and driving therapeutic decision-making over time, thus leading to a point of fragmentation along this translational pipeline.
Finally, there is the hurdle of approval for clinical use by governing regulatory bodies. Indeed, of the biomarkers discussed in this paper only galectin-3, sST2, and hsCRP have recognized cardiovascular indications by the FDA despite the promising human clinical data presented above (58, 136, 137). This appears to be largely due to the lack of a clearly defined roadmap linking scientific, industrial, and regulatory bodies in a collaborative fashion (117).
There remains a considerable unmet need for an improved armament of blood-based biomarkers in early detection and prevention of a variety of common cardiovascular disease states. Against this, hurdles to the development and commercialization of cardiovascular biomarkers remain. The markers presented above carry promising prognostic and predictive value that suggests applicability in guiding therapy, however longitudinal data demonstrating a reflection in improved patient outcomes over time remains limited and should be an area of future attention. Improved molecular phenotyping with more advanced blood-based biosignatures will likely permit enhanced stratification of patients in subgroups where distinct biology may be shared, and subsequently drive personalized treatment regimens. Further, the growing understanding of these disease phenotypes provided by emerging biomarkers may implicitly lead to the development of therapies specifically targeting these subgroups. This has the potential to assist in accelerating translation of biologically relevant therapies – such as novel small molecules and targeted RNA therapies – in groups enriched with relevant signaling abnormalities. Ongoing collaboration between academic and clinical leaders with industry and regulatory bodies are required to overcome hurdles in biomarker translation. Looking forward, exciting opportunities abound in this area that will enable the practice of cardiology to better embrace the precision medicine strategies of the future.
SS and JG drafted the initial version under the supervision of GF, with all other authors contributing additional sections and editing. All authors were significantly involved in the writing and editing of this manuscript.
The authors declare that the research was conducted in the absence of any commercial or financial relationships that could be construed as a potential conflict of interest.
All claims expressed in this article are solely those of the authors and do not necessarily represent those of their affiliated organizations, or those of the publisher, the editors and the reviewers. Any product that may be evaluated in this article, or claim that may be made by its manufacturer, is not guaranteed or endorsed by the publisher.
ACS, acute coronary syndrome; ALK, anaplastic lymphoma receptor tyrosine kinase; APACHE II, acute physiology and chronic health evaluation II; Ang2, angiopoietin-2; ALVD, asymptomatic left ventricular dysfunction; BNP, brain natriuretic peptide; CABG, coronary artery bypass graft; CAD, coronary artery disease; circRNA, circular RNA; CRP, c-reactive protein; CRT, cardiac resynchronisation therapy; CVD, cardiovascular disease; ICAM, intercellular adhesion molecule; ICU, intensive care unit; IGF-1, insulin-like growth factor; IGFBP, IGF-1 binding protein; IgG, immunoglobulin G; IgM, immunoglobulin M; IL-8, IL-10, interleukin-8, interleukin 10; FBXW7, f-box and WD repeat domain containing 7; FECH, ferrochelatase; Gal-3, galectin-3; GH, growth hormone; HF, heart failure; HFrEF, heart failure with reduced ejection fraction; hsCRP, high sensitivity c-reactive protein; LIPCAR, long intergenic non-coding RNA predicting cardiac remodeling; lncRNA, long non-coding RNA; LOX-1, lectin-like oxLDL (oxidized low-density lipoprotein) receptor 1; LV, left ventricle; LVAD, left ventricular assist device; MAA, malondialdehyde-acetaldehyde adduct; MDA, malondialdehyde; MICRA, myocardial infarction-associated circular RNA; miR/miRNA, microRNA; mitomiR, mitochondrial miRNA; MMP, matrix metalloproteinase; ncRNA, non-coding RNA; NGFB, nerve growth factor (beta polypeptide); NT-proBNP, N terminal-pro BNP; NYHA, New York Heart Association; OPN, osteopontin; PSGL-1, p-selectin glycoprotein ligand-1; RNA, ribonucleic acid; SLC43A2, solute carrier family 43, member 2; sLOX-1, soluble LOX-1; SOFA, sequential organ failure assessment; sST2, soluble suppression of tumorigenesis-2; STEMI, ST-elevation myocardial infarction; sTNFr-II, soluble tumor necrosis factor receptor-II; TMEM79, transmembrane protein 79; TSP2, thrombospondin-2; UBN1, ubinuclein 1; VCAM, vascular cell adhesion molecule; VEGF, vascular endothelial growth factor.
1. Roth GA, Mensah GA, Johnson CO, Addolorato G, Ammirati E, Baddour LM, et al. Global burden of cardiovascular diseases and risk factors, 1990-2019: update from the GBD 2019 study. J Am Coll Cardiol. (2020) 76:2982–3021. doi: 10.1016/j.jacc.2020.11.010
2. Vasan RS. Biomarkers of cardiovascular disease. Circulation. (2006) 113:2335–62. doi: 10.1161/CIRCULATIONAHA.104.482570
3. Figtree GA, Vernon ST, Hadziosmanovic N, Sundstrm J, Alfredsson J, Arnott C, et al. Mortality in STEMI patients without standard modifiable risk factors: a sex-disaggregated analysis of SWEDEHEART registry data. Lancet. (2021) 397:1085–94. doi: 10.1016/S0140-6736(21)00272-5
4. Topel ML, Kim JH, Mujahid MS, Ko Y, Vaccarino V, Mubasher M, et al. Individual characteristics of resilience are associated with lower-than-expected neighborhood rates of cardiovascular disease in blacks: results from the Morehouse-Emory Cardiovascular (MECA) Center for Health Equity Study. J Am Heart Assoc. (2019) 8:e011633. doi: 10.1161/JAHA.118.011633
5. Kott KA, Vernon ST, Hansen T, Yu C, Bubb KJ, Coffey S, et al. Biobanking for discovery of novel cardiovascular biomarkers using imaging-quantified disease burden: protocol for the longitudinal, prospective, BioHEART-CT cohort study. BMJ Open. (2019) 9:e028649. doi: 10.1136/bmjopen-2018-028649
6. Figtree GA, Vernon ST. Coronary artery disease patients without standard modifiable risk factors (SMuRFs)- a forgotten group calling out for new discoveries. Cardiovasc Res. (2021) 117:e76–8. doi: 10.1093/cvr/cvab145
7. Smith JA, Ware EB, Middha P, Beacher L, Kardia SLR. Current applications of genetic risk scores to cardiovascular outcomes and subclinical phenotypes. Curr Epidemiol Rep. (2015) 2:180–90. doi: 10.1007/s40471-015-0046-4
8. Montaner J, Ramiro L, Simats A, Tiedt S, Makris K, Jickling GC, et al. Multilevel omics for the discovery of biomarkers and therapeutic targets for stroke. Nat Rev Neurol. (2020) 16:247–64. doi: 10.1038/s41582-020-0350-6
9. Figtree GA, Broadfoot K, Casadei B, Califf R, Crea F, Drummond GR, et al. A call to action for new global approaches to cardiovascular disease drug solutions. Eur Heart J. (2021) 42:1464–75. doi: 10.1093/eurheartj/ehab068
10. Kott KA, Vernon ST, Hansen T, de Dreu M, Das SK, Powell J, et al. SingleCell immune profiling in coronary artery disease: the role of StateoftheArt immunophenotyping with mass cytometry in the diagnosis of atherosclerosis. J Am Heart Assoc. (2020) 9:e017759. doi: 10.1161/JAHA.120.017759
11. Ezhov M, Safarova M, Afanasieva O, Mitroshkin M, Matchin Y, Pokrovsky S. Matrix metalloproteinase 9 as a predictor of coronary atherosclerotic plaque instability in stable coronary heart disease patients with elevated lipoprotein(a) levels. Biomolecules. (2019) 9:129. doi: 10.3390/biom9040129
12. Ntzouvani A, Fragopoulou E, Panagiotakos D, Pitsavos C, Antonopoulou S. Reduced circulating adiponectin levels are associated with the metabolic syndrome independently of obesity, lipid indices and serum insulin levels: a cross-sectional study. Lipids Health Dis. (2016) 15:140–140. doi: 10.1186/s12944-016-0311-7
13. Maahs DM, Ogden LG, Kinney GL, Wadwa P, Snell-Bergeon JK, Dabelea D, et al. Low plasma adiponectin levels predict progression of coronary artery calcification. Circulation. (2005) 111:747–53. doi: 10.1161/01.CIR.0000155251.03724.A5
14. Ridker PM, Buring JE, Rifai N. Soluble P-selectin and the risk of future cardiovascular events. Circulation. (2001) 103:491–5. doi: 10.1161/01.CIR.103.4.491
15. George R, Bhatt A, Narayani J, Thulaseedharan JV, Sivadasanpillai H, Tharakan JA. Enhanced P-selectin expression on platelet-a marker of platelet activation, in young patients with angiographically proven coronary artery disease. Mol Cell Biochem. (2016) 419:125–33. doi: 10.1007/s11010-016-2756-4
16. Hofmann A, Brunssen C, Wolk S, Reeps C, Morawietz H. Soluble LOX1: a novel biomarker in patients with coronary artery disease, stroke, and acute aortic dissection? J Am Heart Assoc. (2020) 9:e013803. doi: 10.1161/JAHA.119.013803
17. Chan W, White DA, Wang X, Bai R, Liu Y, Yu H, et al. Macrophage migration inhibitory factor for the early prediction of infarct size. J Am Heart Assoc. (2013) 2:e000226. doi: 10.1161/JAHA.113.000226
18. Elgebaly SA, Christenson RH, Kandil H, Ibrahim M, Rizk H, El-Khazragy N, et al. Nourin-dependent miR-137 and miR-106b: novel biomarkers for early diagnosis of myocardial ischemia in coronary artery disease patients. Diagnostics. (2021) 11:703. doi: 10.3390/diagnostics11040703
19. Rosenberg M, Zugck C, Nelles M, Juenger C, Frank D, Remppis A, et al. Osteopontin, a new prognostic biomarker in patients with chronic heart failure. Circ Heart Fail. (2008) 1:43–9. doi: 10.1161/CIRCHEARTFAILURE.107.746172
20. Lok DJ, Lok SI, Bruggink-Andr de la Porte PW, Badings E, Lipsic E, van Wijngaarden J, et al. Galectin-3 is an independent marker for ventricular remodeling and mortality in patients with chronic heart failure. Clin Res Cardiol. (2013) 102:103–10. doi: 10.1007/s00392-012-0500-y
21. Chen WS, Wang CH, Cheng CW, Liu MH, Chu CM, Wu HP, et al. Elevated plasma phenylalanine predicts mortality in critical patients with heart failure. ESC Heart Fail. (2020) 7:2884–93. doi: 10.1002/ehf2.12896
22. Dubois E, Richard V, Mulder P, Lamblin N, Drobecq H, Henry JP, et al. Decreased Serine207 phosphorylation of troponin T as a biomarker for left ventricular remodelling after myocardial infarction. Eur Heart J. (2011) 32:115–23. doi: 10.1093/eurheartj/ehq108
23. Salgado-Somoza A, Zhang L, Vausort M, Devaux Y. The circular RNA MICRA for risk stratification after myocardial infarction. Int J Cardiol Heart Vasc. (2017) 17:33–6. doi: 10.1016/j.ijcha.2017.11.001
24. Smih F, Desmoulin F, Berry M, Turkieh A, Harmancey R, Iacovoni J, et al. Blood signature of pre-heart failure: a microarrays study. PLoS ONE. (2011) 6:e20414–e20414. doi: 10.1371/journal.pone.0020414
25. Wells Quinn S, Gupta Deepak K, Smith JG, Collins Sean P, Storrow Alan B, Ferguson J, et al. Accelerating biomarker discovery through electronic health records, automated biobanking, and proteomics. J Am Coll Cardiol. (2019) 73:2195–205. doi: 10.1016/j.jacc.2019.01.074
26. Barutaut M, Fournier P, Peacock WF, Evaristi MF, Caubre C, Turkieh A, et al. Insulin-like growth factor binding protein 2 predicts mortality risk in heart failure. Int J Cardiol. (2020) 300:245–51. doi: 10.1016/j.ijcard.2019.09.032
27. Meikle PJ, Wong G, Tsorotes D, Barlow CK, Weir JM, Christopher MJ, et al. Plasma lipidomic analysis of stable and unstable coronary artery disease. Arterioscler Thromb Vasc Biol. (2011) 31:2723–32. doi: 10.1161/ATVBAHA.111.234096
28. Kumarswamy R, Bauters C, Volkmann I, Maury F, Fetisch J, Holzmann A, et al. Circulating long noncoding RNA, LIPCAR, predicts survival in patients with heart failure. Circ Res. (2014) 114:1569–75. doi: 10.1161/CIRCRESAHA.114.303915
29. Ovchinnikova ES, Schmitter D, Vegter EL, ter Maaten JM, Valente MAE, Liu LCY, et al. Signature of circulating microRNAs in patients with acute heart failure. Eur J Heart Fail. (2016) 18:414–23. doi: 10.1002/ejhf.332
30. Anderson DR, Duryee MJ, Shurmur SW, Um JY, Bussey WD, Hunter CD, et al. Unique antibody responses to malondialdehyde-acetaldehyde (MAA)-protein adducts predict coronary artery disease. PLoS ONE. (2014) 9:e107440. doi: 10.1371/journal.pone.0107440
31. Mrz W, Meinitzer A, Drechsler C, Pilz S, Krane V, Kleber ME, et al. Homoarginine, cardiovascular risk, and mortality. Circulation. (2010) 122:967–75. doi: 10.1161/CIRCULATIONAHA.109.908988
32. Voora D, Cyr D, Lucas J, Chi JT, Dungan J, McCaffrey TA, et al. Aspirin exposure reveals novel genes associated with platelet function and cardiovascular events. J Am Coll Cardiol. (2013) 62:1267–76. doi: 10.1016/j.jacc.2013.05.073
33. Davarzani N, Sanders-van Wijk S, Maeder MT, Rickenbacher P, Smirnov E, Karel J, et al. Novel concept to guide systolic heart failure medication by repeated biomarker testing-results from TIME-CHF in context of predictive, preventive, and personalized medicine. EPMA J. (2018) 9:161–73. doi: 10.1007/s13167-018-0137-7
34. Spinale FG, Meyer TE, Stolen CM, Van Eyk JE, Gold MR, Mittal S, et al. Development of a biomarker panel to predict cardiac resynchronization therapy response: results from the SMART-AV trial. Heart Rhythm. (2019) 16:743–53. doi: 10.1016/j.hrthm.2018.11.026
35. Li T, Li X, Feng Y, Dong G, Wang Y, Yang J. The role of matrix metalloproteinase-9 in atherosclerotic plaque instability. Mediators Inflamm. (2020) 2020:3872367. doi: 10.1155/2020/3872367
36. Maurovich-Horvat P, Ferencik M, Voros S, Merkely B, Hoffmann U. Comprehensive plaque assessment by coronary CT angiography. Nat Rev Cardiol. (2014) 11:390–402. doi: 10.1038/nrcardio.2014.60
37. Leber AW, von Ziegler F, Becker A, Becker CR, Reiser M, Steinbeck G, et al. Characteristics of coronary plaques before angiographic progression determined by Multi-Slice CT. Int J Cardiovasc Imaging. (2008) 24:423–8. doi: 10.1007/s10554-007-9278-9
38. Voros S, Rinehart S, Qian Z, Joshi P, Vazquez G, Fischer C, et al. Coronary atherosclerosis imaging by coronary CT angiography: current status, correlation with intravascular interrogation and meta-analysis. JACC Cardiovasc Imaging. (2011) 4:537–48. doi: 10.1016/j.jcmg.2011.03.006
39. Jiang X, Zeng HS, Guo Y, Zhou ZB, Tang BS Li FK. The expression of matrix metalloproteinases-9, transforming growth factor-beta1 and transforming growth factor-beta receptor I in human atherosclerotic plaque and their relationship with plaque stability. Chin Med J. (2004) 117:1825–9. doi: 10.3760/cma.j.issn.0366-6999.2004.12.112
40. Sinclair H, Veerasamy M, Bourantas C, Egred M, Nair A, Calvert PA, et al. The role of virtual histology intravascular ultrasound in the identification of coronary artery plaque vulnerability in acute coronary syndromes. Cardiol Rev. (2016) 24:303–9. doi: 10.1097/CRD.0000000000000100
41. Olson FJ, Schmidt C, Gummesson A, Sigurdardottir V, Hulthe J, Wiklund O, et al. Circulating matrix metalloproteinase 9 levels in relation to sampling methods, femoral and carotid atherosclerosis. J Intern Med. (2008) 263:626–35. doi: 10.1111/j.1365-2796.2008.01927.x
42. Lahdentausta L, Leskel J, Winkelmann A, Tervahartiala T, Sorsa T, Pesonen E, et al. Serum MMP-9 diagnostics, prognostics, and activation in acute coronary syndrome and its recurrence. J Cardiovasc Transl Res. (2018) 11:210–20. doi: 10.1007/s12265-018-9789-x
43. Garvin P, Jonasson L, Nilsson L, Falk M, Kristenson M. Plasma matrix metalloproteinase-9 levels predict first-time coronary heart disease: an 8-year follow-up of a community-based middle aged population. PLoS ONE. (2015) 10:e0138290. doi: 10.1371/journal.pone.0138290
44. Liu Y, Vu V, Sweeney G. Examining the potential of developing and implementing use of adiponectin-targeted therapeutics for metabolic and cardiovascular diseases. Front Endocrinol. (2019) 10:842. doi: 10.3389/fendo.2019.00842
45. Yanai H, Yoshida H. Beneficial effects of adiponectin on glucose and lipid metabolism and atherosclerotic progression: mechanisms and perspectives. Int J Mol Sci. (2019) 20:1190. doi: 10.3390/ijms20051190
46. Mathieu P, Pibarot P, Desprs JP. Metabolic syndrome: the danger signal in atherosclerosis. Vasc Health Risk Manag. (2006) 2:285–302. doi: 10.2147/vhrm.2006.2.3.285
47. Song C, Wu G, Chang S, Bie L. Plasma P-selectin level is associated with severity of coronary heart disease in Chinese Han population. J Int Med Res. (2020) 48:0300060519896437. doi: 10.1177/0300060519896437
48. Merten M, Thiagarajan P. P-selectin in arterial thrombosis. Z Kardiol. (2004) 93:855–63. doi: 10.1007/s00392-004-0146-5
49. Hofmann A, Brunssen C, Morawietz H. Contribution of lectin-like oxidized low-density lipoprotein receptor-1 and LOX-1 modulating compounds to vascular diseases. Vascul Pharmacol. (2018) 107:1–11. doi: 10.1016/j.vph.2017.10.002
50. Bartekova M, Radosinska J, Jelemensky M, Dhalla NS. Role of cytokines and inflammation in heart function during health and disease. Heart Fail Rev. (2018) 23:733–58. doi: 10.1007/s10741-018-9716-x
51. Luedike P, Alatzides G, Papathanasiou M, Heisler M, Pohl J, Lehmann N, et al. Circulating macrophage migration inhibitory factor (MIF) in patients with heart failure. Cytokine. (2018) 110:104–9. doi: 10.1016/j.cyto.2018.04.033
52. Elgebaly SA, Poston R, Todd R, Helmy T, Almaghraby AM, Elbayoumi T, et al. Cyclocreatine protects against ischemic injury and enhances cardiac recovery during early reperfusion. Expert Rev Cardiovasc Ther. (2019) 17:683–97. doi: 10.1080/14779072.2019.1662722
53. Tian R, Wu B, Fu C, Guo K. miR-137 prevents inflammatory response, oxidative stress, neuronal injury and cognitive impairment via blockade of Src-mediated MAPK signaling pathway in ischemic stroke. Aging. (2020) 12:10873–95. doi: 10.18632/aging.103301
54. Frangogiannis NG. Cardiac fibrosis. Cardiovasc Res. (2021) 117:1450–88. doi: 10.1093/cvr/cvaa324
55. Denhardt DT, Noda M, ORegan AW, Pavlin D, Berman JS. Osteopontin as a means to cope with environmental insults: regulation of inflammation, tissue remodeling, and cell survival. J Clin Invest. (2001) 107:1055–61. doi: 10.1172/JCI12980
56. Amin HZ, Amin LZ, Wijaya IP. Galectin-3: a novel biomarker for the prognosis of heart failure. Clujul Med. (2017) 90:129–132. doi: 10.15386/cjmed-751
57. Mueller T, Dieplinger B. Soluble ST2 and galectin-3: what we know and dont know analytically. Ejifcc. (2016) 27:224.
58. Li M, Yuan Y, Guo K, Lao Y, Huang X, Feng L. Value of galectin-3 in acute myocardial infarction. Am J Cardiovasc Drugs. (2020) 20:333–42. doi: 10.1007/s40256-019-00387-9
59. Garg P, Morris P, Fazlanie AL, Vijayan S, Dancso B, Dastidar AG, et al. Cardiac biomarkers of acute coronary syndrome: from history to high-sensitivity cardiac troponin. Intern Emerg Med. (2017) 12:147–55. doi: 10.1007/s11739-017-1612-1
60. Czibik G, Mezdari Z, Murat Altintas D, Brhat J, Pini M, dHumires T, et al. Dysregulated phenylalanine catabolism plays a key role in the trajectory of cardiac aging. Circulation. (2021) 144:559–74. doi: 10.1161/CIRCULATIONAHA.121.054204
61. Murr C, Grammer TB, Meinitzer A, Kleber ME, Mrz W, Fuchs D. Immune activation and inflammation in patients with cardiovascular disease are associated with higher phenylalanine to tyrosine ratios: the Ludwigshafen risk and cardiovascular health study. J Amino Acids. (2014) 2014:783730–783730. doi: 10.1155/2014/783730
62. Murtha LA, Schuliga MJ, Mabotuwana NS, Hardy SA, Waters DW, Burgess JK, et al. The Processes and mechanisms of cardiac and pulmonary fibrosis. Front Physiol. (2017) 8:777. doi: 10.3389/fphys.2017.00777
63. Park S, Nguyen NB, Pezhouman A, Ardehali R. Cardiac fibrosis: potential therapeutic targets. Transl Res. (2019) 209:121–37. doi: 10.1016/j.trsl.2019.03.001
64. Hinderer S, Schenke-Layland K. Cardiac fibrosis A short review of causes and therapeutic strategies. Adv Drug Deliv Rev. (2019) 146:77–82. doi: 10.1016/j.addr.2019.05.011
65. Abebe TB, Gebreyohannes EA, Tefera YG, Abegaz TM. Patients with HFpEF and HFrEF have different clinical characteristics but similar prognosis: a retrospective cohort study. BMC Cardiovasc Disord. (2016) 16:232. doi: 10.1186/s12872-016-0418-9
66. Echouffo-Tcheugui Justin B, Erqou S, Butler J, Yancy Clyde W, Fonarow Gregg C. Assessing the risk of progression fromasymptomatic left ventricular dysfunction to overt heart failure. JACC Heart Fail. (2016) 4:237–48. doi: 10.1016/j.jchf.2015.09.015
67. Zdrojewski T. Prevalence, Incidence and Lifetime Risk of Heart Failure. Berlin, Heidelberg: Springer (2016), p. 3–13. doi: 10.1007/978-3-319-45237-1_1
68. Sun C, Ni M, Song B, Cao L. Circulating circular RNAs: novel biomarkers for heart failure. Front Pharmacol. (2020) 11:560537. doi: 10.3389/fphar.2020.560537
69. Altesha MA Ni T, Khan A, Liu K, Zheng X. Circular RNA in cardiovascular disease. J Cell Physiol. (2019) 234:5588–600. doi: 10.1002/jcp.27384
70. Kuznetsova T, Herbots L, Jin Y, Stolarz-Skrzypek K, Staessen JA. Systolic and diastolic left ventricular dysfunction: from risk factors to overt heart failure. Expert Rev Cardiovasc Ther. (2010) 8:251–8. doi: 10.1586/erc.10.3
71. Betti I, Castelli G, Barchielli A, Beligni C, Boscherini V, De Luca L, et al. The role of N-terminal PRO-brain natriuretic peptide and echocardiography for screening asymptomatic left ventricular dysfunction in a population at high risk for heart failure. The PROBE-HF Study. J Card Fail. (2009) 15:377–84. doi: 10.1016/j.cardfail.2008.12.002
72. Akwii RG, Sajib MS, Zahra FT, Mikelis CM. Role of Angiopoietin-2 in Vascular Physiology and Pathophysiology. Cells. (2019) 8:471. doi: 10.3390/cells8050471
73. Thurston G, Daly C. The complex role of angiopoietin-2 in the angiopoietin-tie signaling pathway. Cold Spring Harb Perspect Med. (2012) 2:a006550–a006550. doi: 10.1101/cshperspect.a006650
74. Schroen B, Heymans S, Sharma U, Blankesteijn WM, Pokharel S, Cleutjens JPM, et al. Thrombospondin-2 is essential for myocardial matrix integrity. Circ Res. (2004) 95:515–22. doi: 10.1161/01.RES.0000141019.20332.3e
75. Egerstedt A, Berntsson J, Smith ML, Gidlf O, Nilsson R, Benson M, et al. Profiling of the plasma proteome across different stages of human heart failure. Nat Commun. (2019) 10:5830. doi: 10.1038/s41467-019-13306-y
76. Castellano G, Affuso F, Conza PD, Fazio S. The GH/IGF-1 axis and heart failure. Curr Cardiol Rev. (2009) 5:203–15. doi: 10.2174/157340309788970306
77. Hoeflich A, David R, Hjortebjerg R. Current IGFBP-related biomarker research in cardiovascular disease-we need more structural and functional information in clinical studies. Front Endocrinol. (2018) 9:388–388. doi: 10.3389/fendo.2018.00388
78. Martin RM, Gunnell D, Whitley E, Nicolaides A, Griffin M, Georgiou N, et al. Associations of insulin-like growth factor (IGF)-I, IGF-II, IGF binding protein (IGFBP)-2 and IGFBP-3 with ultrasound measures of atherosclerosis and plaque stability in an older adult population. J Clin Endocrinol Metab. (2008) 93:1331–8. doi: 10.1210/jc.2007-2295
79. Halim SA, Neely ML, Pieper KS, Shah SH, Kraus WE, Hauser ER, et al. Simultaneous consideration of multiple candidate protein biomarkers for long-term risk for cardiovascular events. Circ Cardiovasc Genet. (2015) 8:168–77. doi: 10.1161/CIRCGENETICS.113.000490
80. Lau ES, Paniagua SM, Zarbafian S, Hoffman U, Long MT, Hwang S, et al. Cardiovascular biomarkers of obesity and overlap with cardiometabolic dysfunction. J Am Heart Assoc. (2021) 10:e020215. doi: 10.1161/JAHA.120.020215
81. Bianco CM, Farjo PD, Ghaffar YA, Sengupta PP. Myocardial mechanics in patients with normal LVEF and diastolic dysfunction. JACC Cardiovasc Imaging. (2020) 13(1, Part 2):258–71. doi: 10.1016/j.jcmg.2018.12.035
82. Wende Adam R, Brahma Manoja K, McGinnis Graham R, Young Martin E. Metabolic origins of heart failure. JACC Basic Transl Sci. (2017) 2:297–310. doi: 10.1016/j.jacbts.2016.11.009
83. Shapiro MD, Fazio S. From lipids to inflammation. Circ Res. (2016) 118:732–49. doi: 10.1161/CIRCRESAHA.115.306471
84. Givvimani S, Pushpakumar SB, Metreveli N, Veeranki S, Kundu S, Tyagi SC. Role of mitochondrial fission and fusion in cardiomyocyte contractility. Int J Cardiol. (2015) 187:325–33. doi: 10.1016/j.ijcard.2015.03.352
85. Song R, Hu XQ, Zhang L. Mitochondrial miRNA in cardiovascular function and disease. Cells. (2019) 8:1475. doi: 10.3390/cells8121475
86. Vegter EL, van der Meer P, de Windt LJ, Pinto YM, Voors AA. MicroRNAs in heart failure: from biomarker to target for therapy. Eur J Heart Fail. (2016) 18:457–68. doi: 10.1002/ejhf.495
87. Karimi Galougahi K, Antoniades C, Nicholls SJ, Channon KM, Figtree GA. Redox biomarkers in cardiovascular medicine. Eur Heart J. (2015) 36:1576–82. doi: 10.1093/eurheartj/ehv126
88. Daiber A, Chlopicki S. Revisiting pharmacology of oxidative stress and endothelial dysfunction in cardiovascular disease: evidence for redox-based therapies. Free Radic Biol Med. (2020) 157:15–37. doi: 10.1016/j.freeradbiomed.2020.02.026
89. Brancaccio M, Mennitti C, Cesaro A, Fimiani F, Moscarella E, Caiazza M, et al. Dietary thiols: a potential supporting strategy against oxidative stress in heart failure and muscular damage during sports activity. Int J Environ Res Public Health. (2020) 17:9424. doi: 10.3390/ijerph17249424
90. Walter MF, Jacob RF, Jeffers B, Ghadanfar MM, Preston GM, Buch J, et al. Serum levels of thiobarbituric acid reactive substances predict cardiovascular events in patients with stable coronary artery disease: a longitudinal analysis of the PREVENT study. J Am Coll Cardiol. (2004) 44:1996–2002. doi: 10.1016/j.jacc.2004.08.029
91. Bassu S, Zinellu A, Sotgia S, Mangoni AA, Floris A, Farina G, et al. Oxidative stress biomarkers and peripheral endothelial dysfunction in rheumatoid arthritis: a monocentric cross-sectional case-control study. Molecules. (2020) 25:3855. doi: 10.3390/molecules25173855
92. Nakamura J, Shimomoto T, Collins LB, Holley DW, Zhang Z, Barbee JM, et al. Evidence that endogenous formaldehyde produces immunogenic and atherogenic adduct epitopes. Sci Rep. (2017) 7:10787. doi: 10.1038/s41598-017-11289-8
93. Karetnikova ES, Jarzebska N, Markov AG, Weiss N, Lentz SR, Rodionov RN. Is homoarginine a protective cardiovascular risk factor? Arterioscler Thromb Vasc Biol. (2019) 39:869–75. doi: 10.1161/ATVBAHA.118.312218
94. Pilz S, Meinitzer A, Gaksch M, Grbler M, Verheyen N, Drechsler C, et al. Homoarginine in the renal and cardiovascular systems. Amino Acids. (2015) 47:1703–13. doi: 10.1007/s00726-015-1993-2
95. Seppl I, Oksala N, Jula A, Kangas AJ, Soininen P, Hutri-Khnen N, et al. The biomarker and causal roles of homoarginine in the development of cardiometabolic diseases: an observational and Mendelian randomization analysis. Sci Rep. (2017) 7:1130. doi: 10.1038/s41598-017-01274-6
96. Martin S, Cule M, Basty N, Tyrrell J, Beaumont RN, Wood AR, et al. Genetic evidence for different adiposity phenotypes and their opposing influences on ectopic fat and risk of cardiometabolic disease. Diabetes. (2021) 70:1843–56. doi: 10.2337/db21-0129
97. Pletcher MJ, Pignone M. Evaluating the clinical utility of a biomarker: a review of methods for estimating health impact. Circulation. (2011) 123:1116–24. doi: 10.1161/CIRCULATIONAHA.110.943860
98. Selleck MJ, Senthil M, Wall NR. Making meaningful clinical use of biomarkers. Biomark Insights. (2017) 12:1177271917715236. doi: 10.1177/1177271917715236
99. Iyngkaran P, Noaman S, Chan W, Mahadavan G, Thomas MC, Rajendran S. Non-invasive risk stratification for coronary artery disease: is it time for subclassifications? Curr Cardiol Rep. (2019) 21:87. doi: 10.1007/s11886-019-1174-0
100. Weldy CS, Ashley EA. Towards precision medicine in heart failure. Nat Rev Cardiol. (2021) 18:745–62. doi: 10.1038/s41569-021-00566-9
101. Pinchevsky Y, Butkow N, Chirwa T, Raal FJ. Glycaemic, blood pressure and cholesterol control in 25 629 diabetics: review articles. Cardiovasc J Afr. (2015) 26:188–92. doi: 10.5830/CVJA-2015-050
102. Lordkipanidz M. Platelet function tests. Semin Thromb Hemost. (2016) 42:258–67. doi: 10.1055/s-0035-1564834
103. Van Oosterom N, Barras M, Cottrell N, Bird R. Platelet function assays for the diagnosis of aspirin resistance. Platelets. (2022) 33:329–38. doi: 10.1080/09537104.2021.1942816
104. Paven E, Dillinger JG, Bal dit Sollier C, Vidal-Trecan T, Berge N, Dautry R, et al. Determinants of aspirin resistance in patients with type 2 diabetes. Diabetes Metab. (2020) 46:370–6. doi: 10.1016/j.diabet.2019.11.002
105. Nadar SK, Shaikh MM. Biomarkers in routine heart failure clinical care. Card Fail Rev. (2019) 5:50–6. doi: 10.15420/cfr.2018.27.2
106. Ibrahim NE, Januzzi JL. Established and emerging roles of biomarkers in heart failure. Circ Res. (2018) 123:614–29. doi: 10.1161/CIRCRESAHA.118.312706
107. Troughton RW, Frampton CM, Brunner-La Rocca HP, Pfisterer M, Eurlings LWM, Erntell H, et al. Effect of B-type natriuretic peptide-guided treatment of chronic heart failure on total mortality and hospitalization: an individual patient meta-analysis. Eur Heart J. (2014) 35:1559–67. doi: 10.1093/eurheartj/ehu090
108. Spoletini I, Coats AJS, Senni M, Rosano GMC. Monitoring of biomarkers in heart failure. Eur Heart J Suppl. (2019) 21(Suppl):M5–8. doi: 10.1093/eurheartj/suz215
109. Fries F, Loureno P, Laszczynska O, Almeida PB, Guimares JT, Januzzi JL, et al. Prognostic value of sST2 added to BNP in acute heart failure with preserved or reduced ejection fraction. Clin Res Cardiol. (2015) 104:491–9. doi: 10.1007/s00392-015-0811-x
110. Abraham WT, Hayes DL. Cardiac resynchronization therapy for heart failure. Circulation. (2003) 108:2596–603. doi: 10.1161/01.CIR.0000096580.26969.9A
111. Gorodeski EZ, Magnelli-Reyes C, Moennich LA, Grimaldi A, Rickard J. Cardiac resynchronization therapy-heart failure (CRT-HF) clinic: a novel model of care. PLoS ONE. (2019) 14:e0222610. doi: 10.1371/journal.pone.0222610
112. Pires LA, Abraham WT, Young JB, Johnson KM. Clinical predictors and timing of New York Heart Association class improvement with cardiac resynchronization therapy in patients with advanced chronic heart failure: results from the Multicenter InSync Randomized Clinical Evaluation (MIRACLE) and Multicenter InSync ICD Randomized Clinical Evaluation (MIRACLE-ICD) trials. Am Heart J. (2006) 151:837–43. doi: 10.1016/j.ahj.2005.06.024
113. Goldenberg I, Moss AJ, Hall WJ, Foster E, Goldberger JJ, Santucci P, et al. Predictors of response to cardiac resynchronization therapy in the Multicenter Automatic Defibrillator Implantation Trial with Cardiac Resynchronization Therapy (MADIT-CRT). Circulation. (2011) 124:1527–36. doi: 10.1161/CIRCULATIONAHA.110.014324
114. Ellenbogen KA, Gold MR, Meyer TE, Fernndez Lozano I, Mittal S, Waggoner AD, et al. Primary results from the SmartDelay determined AV optimization: a comparison to other AV delay methods used in cardiac resynchronization therapy (SMART-AV) trial: a randomized trial comparing empirical, echocardiography-guided, and algorithmic atrioventricular delay programming in cardiac resynchronization therapy. Circulation. (2010) 122:2660–8. doi: 10.1161/CIRCULATIONAHA.110.992552
115. Morival C, Westerlynck R, Bouzill G, Cuggia M, Le Corre P. Prevalence and nature of statin drug-drug interactions in a university hospital by electronic health record mining. Eur J Clin Pharmacol. (2018) 74:525–34. doi: 10.1007/s00228-017-2400-6
116. Jayawardana KS, Mundra PA, Giles C, Barlow CK, Nestel PJ, Barnes EH, et al. Changes in plasma lipids predict pravastatin efficacy in secondary prevention. JCI Insight. (2019) 4:e128438. doi: 10.1172/jci.insight.128438
117. Lavezzari G, Womack A. Industry perspectives on biomarker qualification. Clin Pharmacol Ther. (2016) 99:208–13. doi: 10.1002/cpt.264
118. Ouellette SB. Landscape of granted US patents in personalized diagnostics for oncology from 2014 to 2018. Expert Opin Ther Pat. (2019) 29:191–8. doi: 10.1080/13543776.2019.1575809
119. Haanes EJ, Cnaves JM. Stealing fire: a retrospective survey of biotech patent claims in the wake of Mayo v. Prometheus. Nat Biotechnol. (2012) 30:758–60. doi: 10.1038/nbt.2318
120. Offit K, Bradbury A, Storm C, Merz JF, Noonan KE, Spence R. Gene patents and personalized cancer care: impact of the Myriad case on clinical oncology. J Clin Oncol. (2013) 31:2743. doi: 10.1200/JCO.2013.49.7388
121. Ganske SA. The US Supreme Court decides six patent cases in 2014, culminating in Alice Corp. v CLS Bank International. Tex Intell Prop LJ. (2014) 23:183.
122. Chang BC, Wang SJ. The impact of patent eligibility on biotech patents: a flow chart for determining patent eligibility and an immune therapy case study. Hum Vaccin Immunother. (2015) 11:789–94. doi: 10.1080/21645515.2015.1009344
123. Cirulli ET, Guo L, Leon Swisher C, Shah N, Huang L, Napier LA, et al. Profound perturbation of the metabolome in obesity is associated with health risk. Cell Metab. (2019) 29:488–500.e2. doi: 10.1016/j.cmet.2018.09.022
124. Wu AHB, Christenson R. The era for high-sensitivity cardiac troponin has begun in the US (finally). J Appl Lab Med. (2017) 2:1–3. doi: 10.1373/jalm.2017.023648
125. Harvell B, Henrie N, Ernst AA, Weiss SJ, Oglesbee S, Sarangarm D, et al. The meaning of elevated troponin I levels: not always acute coronary syndromes. Am J Emerg Med. (2016) 34:145–8. doi: 10.1016/j.ajem.2015.09.037
126. Grabowska I, Sharma N, Vasilescu A, Iancu M, Badea G, Boukherroub R, et al. Electrochemical aptamer-based biosensors for the detection of cardiac biomarkers. ACS Omega. (2018) 3:12010–8. doi: 10.1021/acsomega.8b01558
127. Azzouz A, Hejji L, Sonne C, Kim KH, Kumar V. Nanomaterial-based aptasensors as an efficient substitute for cardiovascular disease diagnosis: future of smart biosensors. Biosens Bioelectron. (2021) 193:113617. doi: 10.1016/j.bios.2021.113617
128. Negahdary M, Behjati-Ardakani M, Heli H. An electrochemical troponin T aptasensor based on the use of a macroporous gold nanostructure. Microchimica Acta. (2019) 186:377. doi: 10.1007/s00604-019-3472-z
129. Ouyang M, Tu D, Tong L, Sarwar M, Bhimaraj A, Li C, et al. A review of biosensor technologies for blood biomarkers toward monitoring cardiovascular diseases at the point-of-care. Biosens Bioelectron. (2021) 171:112621. doi: 10.1016/j.bios.2020.112621
130. Weber M, Hamm C. Novel biomarkersthe long march from bench to bedside. Eur Heart J. (2008) 29:1079–81. doi: 10.1093/eurheartj/ehn143
131. Boehm E, Better N. Time is myocardium, but who does best? J Nucl Cardiol. (2021) 28. doi: 10.1007/s12350-021-02820-6. [Epub ahead of print].
132. Regan B, O'Kennedy R, Collins D. Point-of-care compatibility of ultra-sensitive detection techniques for the cardiac biomarker troponin i-challenges and potential value. Biosensors. (2018) 8:114. doi: 10.3390/bios8040114
133. Meirinho SG, Dias LG, Peres AM, Rodrigues LR. Voltammetric aptasensors for protein disease biomarkers detection: a review. Biotechnol Adv. (2016) 34:941–53. doi: 10.1016/j.biotechadv.2016.05.006
134. Drucker E, Krapfenbauer K. Pitfalls and limitations in translation from biomarker discovery to clinical utility in predictive and personalised medicine. EPMA J. (2013) 4:7. doi: 10.1186/1878-5085-4-7
135. Narayan V, Thompson EW, Demissei B, Ho JE, Januzzi JL, Ky B. Mechanistic biomarkers informative of both cancer and cardiovascular disease: JACC State-of-the-Art Review. J Am Coll Cardiol. (2020) 75:2726–37. doi: 10.1016/j.jacc.2020.03.067
136. Roberts WL, Sedrick R, Moulton L, Spencer A, Rifai N. Evaluation of four automated high-sensitivity C-reactive protein methods: implications for clinical and epidemiological applications. Clin Chem. (2000) 46:461–8. doi: 10.1093/clinchem/46.4.461
Keywords: cardiovascular disease, biomarker, patents, personalized medicine, translational medicine, commercialization, risk stratification
Citation: Saleh S, George J, Kott KA, Meikle PJ and Figtree GA (2022) The Translation and Commercialisation of Biomarkers for Cardiovascular Disease—A Review. Front. Cardiovasc. Med. 9:897106. doi: 10.3389/fcvm.2022.897106
Received: 15 March 2022; Accepted: 11 May 2022;
Published: 02 June 2022.
Edited by:
Atsushi Tanaka, Saga University, JapanReviewed by:
Edouard Nice, Monash University, AustraliaCopyright © 2022 Saleh, George, Kott, Meikle and Figtree. This is an open-access article distributed under the terms of the Creative Commons Attribution License (CC BY). The use, distribution or reproduction in other forums is permitted, provided the original author(s) and the copyright owner(s) are credited and that the original publication in this journal is cited, in accordance with accepted academic practice. No use, distribution or reproduction is permitted which does not comply with these terms.
*Correspondence: Gemma A. Figtree, gemma.figtree@sydney.edu.au
Disclaimer: All claims expressed in this article are solely those of the authors and do not necessarily represent those of their affiliated organizations, or those of the publisher, the editors and the reviewers. Any product that may be evaluated in this article or claim that may be made by its manufacturer is not guaranteed or endorsed by the publisher.
Research integrity at Frontiers
Learn more about the work of our research integrity team to safeguard the quality of each article we publish.