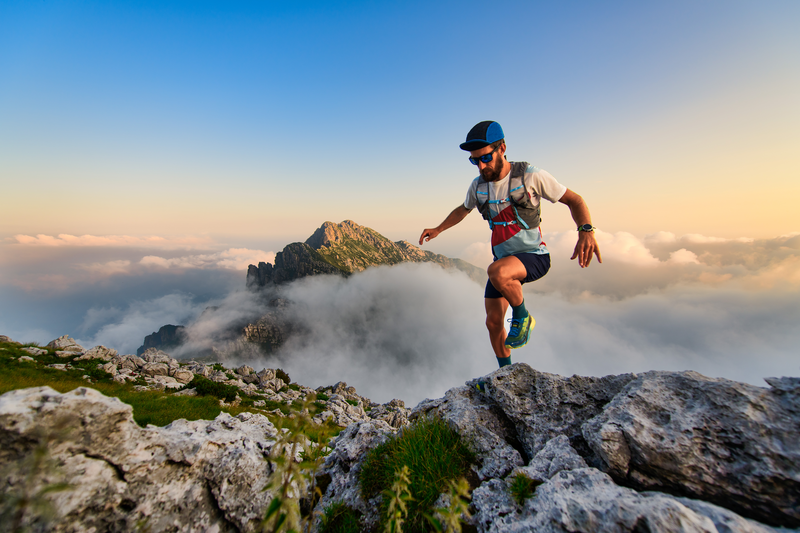
94% of researchers rate our articles as excellent or good
Learn more about the work of our research integrity team to safeguard the quality of each article we publish.
Find out more
CLINICAL TRIAL article
Front. Cardiovasc. Med. , 16 November 2022
Sec. Cardiovascular Surgery
Volume 9 - 2022 | https://doi.org/10.3389/fcvm.2022.1000761
Objective: The aim of this study was to test the hypothesis whether serum level of calpains could become a meaningful biomarker for diagnosis of acute lung injury (ALI) in clinical after cardiac surgery using cardiopulmonary bypass (CPB) technology.
Methods and results: Seventy consecutive adults underwent cardiac surgery with CPB were included in this prospective study. Based on the American-European Consensus Criteria (AECC), these patients were divided into ALI (n = 20, 28.57%) and non-ALI (n = 50, 71.43%) groups. Serum level of calpains in terms of calpains’ activity which was expressed as relative fluorescence unit (RFU) per microliter and measured at beginning of CPB (baseline), 1 h during CPB, end of CPB as well as 1, 12, and 24 h after CPB. Difference of serum level of calpains between two groups first appeared at the end of CPB and remained different at subsequent test points. Univariate and multivariate logistic regression analysis indicated that serum level of calpains 1 h after CPB was an independent predictor for postoperative ALI (OR 1.011, 95% CI 1.001, 1.021, p = 0.033) and correlated with a lower PaO2/FiO2 ratio in the first 2 days (The first day: r = -0.389, p < 0.001 and the second day: r = -0.320, p = 0.007) as well as longer mechanical ventilation time (r = 0.440, p < 0.001), intensive care unit (ICU) length of stay (LOS) (r = 0.419, p < 0.001) and hospital LOS (r = 0.297, p = 0.013).
Conclusion: Elevated serum level of calpains correlate with impaired lung function and poor clinical outcomes, indicating serum level of calpains could act as a potential biomarker for postoperative ALI following CPB in adults.
Clinical trial registration: [https://clinicaltrials.gov/show/NCT05610475], identifier [NCT05610475].
More than two million patients worldwide receive heart surgery every year, majority of these surgical patients will undergo cardiopulmonary bypass (CPB) (1). With the improvement of surgical technology and CPB technology, the incidence of complications after CPB is getting lower. However, the incidence of postoperative acute lung injury (ALI) due to CPB is still as high as 20–35% compared with non-CPB surgery (2, 3). Postoperative lung injury usually leads to the extension of Intensive Care Unit (ICU) and total hospital stay, thereby increasing the mental and economic burden of patients. If severe cases evolve into acute respiratory distress syndrome (ARDS), the mortality rate is close to 30% (4, 5). According to clinical experience, the earlier lung damage is detected, the more successful the treatment will be (6–8). Therefore, in-depth study of the mechanism of CPB-related lung injury, discovery, and validation of certain alarming biomarker of CPB-related lung injury in cardiac surgery patients will greatly contribute to early diagnosis and effective treatment decisions making.
The pathophysiological changes of lung injury caused by CPB are mainly manifested in the inflammatory reaction and ischemia-reperfusion injury (9–12). Systemic inflammation causes the aggregation and activation of neutrophils in small pulmonary vessels. At the same time, vascular permeability increases, and pulmonary interstitial edema occurs, which affects oxygen diffusion, leads to lung dysfunction (11, 13). Two sets of blood supply systems exist in the lungs, including the pulmonary artery system and the bronchial artery system. The former is the main blood supply to the lungs which accounts for 97% of the pulmonary blood flow, while bronchial artery system accounts for only 3% of pulmonary blood flow. Complete cessation of the pulmonary circulatory system during CPB results in a significant decrease in pulmonary blood flow, a feature that makes lung tissue more vulnerable to ischemia-reperfusion injury (14). It is worth mentioning that both pathological processes can activate calpains (15, 16).
Calpain family is a large protease family composed of at least 15 different subtypes, some members are expressed widely in organisms, known as traditional calpains, while other members show tissue specificity (17). According to the level of Ca2+ activation concentration, traditional calpains are divided into calpain-1 (micromolar level) and calpain-2 (millimolar level), which are the most characteristic members of calpain family and ubiquitously expressed (17). Under physiological conditions, the macromolecules of calpains prevent them from freely passing through the cell membrane. Therefore, calpains play a biological role primarily in the cytoplasm (18). However, more and more studies reported that actived calpains were found extracellularly and affected cellular activities (19). The purpose of this prospective study was to examine changes in serum level of traditional calpains in patients following CPB and to explore whether serum calpains could serve as a potential biological marker for predicting the incidence of postoperative ALI.
This prospective study was performed in Affiliated Hospital of Yangzhou University, China. A total of 70 patients beyond the age of 18 who underwent cardiac surgery using CPB technology at cardiovascular surgery department between February 2022 and July 2022 were enrolled in this trial (Supplementary Figure 1). The study protocols were approved by the Ethics Committee of Affiliated Hospital of Yangzhou University (2022-YKL03-SKJ013). Exclusion criteria: Presence of abnormal liver, kidney or other organ function, pulmonary inflammation, COPD or tumors, postoperative need for extracorporeal membrane oxygenation support, or patients who refused to participate were excluded.
Demographic data contains patient’s gender, age, body mass index (BMI), smoking, hypertension, diabetes mellitus, cardiac function and left ventricular ejection fraction (LVEF) were recorded. Routine hemodynamics were performed in all patients during the surgical procedure. Arterial blood gas surveillance was performed every 30 min after mechanical ventilation until the end of the operation. The ratio of inspiratory oxygen fraction to oxygen pressure (PaO2/FiO2) was calculated. Intraoperative data such as surgery type, operation time, CPB time, clamp time, and ultrafiltered volume removal (UVR), as well as serum samples at different time points were collected. After operation, the patients were transferred to the ICU immediately and given sedation, intubation, and mechanical ventilation. Laboratory parameters of all postoperative patients including blood cell analysis, blood gas surveillance, liver, and kidney function alone with chest radiograph and echocardiography were dynamically monitored. A structured tutorial was used to establish consensus in the interpretation of radiographs for radiographic lung-injury scores (LISs) (20, 21). In addition, mechanical ventilation (MV) time together with length of ICU stay and hospital stay were followed up.
Based on the American-European Consensus Conference (AECC) definition (22). Enrolled patients (n = 70) were divided into the ALI group (n = 20) and non-ALI group (n = 50). ALI was defined as follows: Oxygenation: PaO2/FiO2 < 300 mmHg (ignoring positive end-expiratory pressure); Chest radiograph: Visible bilateral infiltration; Pulmonary artery occlusion pressure (PAOP): Below 18 mmHg and no cardiogenic pulmonary edema (CPE). (CPE can be determined by PAOP over 18 mmHg or by the presence of central venous pressure over 14 mmHg with LVEF below 45%).
Serial serum samples were obtained at the following time points: beginning of CPB (baseline), 1 h during CPB, the end of CPB as well as 1, 12, and 24 h after CPB. After centrifugation at 3,000 rpm for 15 min at 4°C, serum was aliquoted and frozen at −80°C until assayed. Serum level of calpains reacted with calpains’ activity, which was measured by using a fluorometric kit (Abcam, Cambridge, UK) according to the manufacturer’s protocol. Calpains’ activity was analyzed using a fluorometer equipped with a 400 nm excitation filter and a 505 nm emission filter. Laboratory staff were blinded to clinical parameters, and researchers involved in collecting demographic and preoperative data were blinded to the level of calpains. The activity of calpains was expressed as relative fluorescence unit (RFU) per microliter of serum per sample.
The data were expressed as mean (standard deviation) or median (25th percentile, 75th percentile). All statistical analyses in this study were performed with SPSS 26.0 for Windows (SPSS Inc., Chicago, IL, USA). After normal distribution analysis by one sample Kolmogorov–Smirnov test. Unpaired student’s t test was devoted to further analyze the normally distributed data while Mann–Whitney U test was used to further analyze the abnormally distributed data. χ2 test was employed to compare categorical data as appropriate. Pearson or Spearman correlation test were performed to access the correlation between serum level of calpains and clinical parameters such as CPB time, PaO2/FiO2 ratio, MV time, ICU LOS, and hospital LOS. Receiver operating characteristic curve (ROC) was computed, and area under the curve (AUC) was calculated to evaluate the significance of calpains’ level in diagnosing ALI. Risk factors associated to the development of ALI including cardiac function, operation time, CPB time, clamp time, serum level of calpains at the end of CPB and serum level of calpains at 1 h after CPB were introduced into further univariate analysis. Multivariate logistic regression analysis (Enter) was then performed on statistically significant univariate predictors to determine the independent risk factor for postoperative ALI. Statistical significance was identified as p < 0.05.
A total of 70 patients met the inclusion criteria. Among them, postoperative ALI occurred in 20 cases (28.57%). The demographic and perioperative characteristics of all participants are shown in Table 1. Demographic parameters of gender, age, BMI, smoking, hypertension, diabetes mellitus, and LVEF were not significantly different between the two groups, as were the intraoperative parameters of surgery type and UFV. However, the cardiac function in basic parameters, operation time, CPB time and clamp time in intraoperative parameters emerged huge increase in ALI patients by comparing with non-ALI counterparts. Further, the perioperative risk factors including MV time, ICU LOS, and hospital LOS were evidently prolonged in ALI patients.
Postoperative serum calpains’ concentrations are shown in Figure 1 and Table 2. Difference in serum level of calpains between the two groups first appeared at the end of CPB, and at the following detection time points within 24 h, the serum level of calpains in ALI patient was obviously higher than that in non-ALI counterparts.
Figure 1. Mean serum concentration of serum level of calpains at baseline level and different time points perioperative period. (*p < 0.05, **p < 0.01, and ****p < 0.0001).
Table 2. Serum calpains’ activity relative fluorescence unit (RFU/μl) at baseline level and different time points after cardiopulmonary bypass (CPB).
Univariate logistic regression analysis including cardiac function, operation time, CPB time, clamp time as well as serum level of calpains at the end of CPB and 1 h after CPB. It revealed that cardiac function, operation time, CPB time, clamp time, and serum level of calpains at 1 h after CPB were conspicuous related with an increased risk of ALI. After adjustment by multivariable logistic analysis, cardiac function, operation time, CPB time, and serum calpains at 1 h after CPB were highly associated with the incidence of postoperative ALI (Table 3).
Table 3. Univariate and multivariate analysis of serum level of calpains after cardiopulmonary bypass (CPB) for prediction of acute lung injury (ALI).
Serum level of calpains at 1 h after CPB correlated with CPB time (Figure 2), and in ROC analysis, the AUC of serum level of calpains at 1 h after CPB for ALI was 0.738 (Figure 3). Derived sensitivity, specificity, and predictive cutoff value of serum level of calpains at 1 h after CPB was listed in Table 4. The serum level of calpains at 1 h after CPB had the highest sensitivity and specificity at the cutoff value of 33.52 (RFU/μl).
Figure 2. Scatterplot displaying the relation between cardiopulmonary bypass (CPB) time and serum level of calpains 1 h after CPB.
Figure 3. Receiver operating characteristic curves displaying the ability of serum level of calpains 1 h after cardiopulmonary bypass (CPB) to predict the occurrence of acute lung injury (ALI). The area under the curve (AUC) of serum calpains’ level 1 h after CPB for ALI was 0.738 (95% CI 0.615, 0.861).
Table 4. Performance of serum level of calpains 1 h after cardiopulmonary bypass (CPB) (RFU/μl) for diagnosis of acute lung injury (ALI).
Higher serum calpains’ level at 1 h followed CPB were significantly related with more a severe ALI, as reflected by the lower PaO2/FiO2 ratio measured in the first two postoperative days (Figures 4A,B) and higher radiographic LIS (Figure 4C). Likewise, elevated serum calpains were correlated with poor clinical outcomes, as reflected by the longer MV time (Figure 5A), ICU LOS (Figure 5B), and hospital LOS (Figure 5C).
Figure 4. Scatterplot displaying the relation between serum level of calpains 1 h after cardiopulmonary bypass (CPB) and the inspiratory oxygen fraction to oxygen pressure (PaO2/FiO2) ratio at first 2 days (A,B), and radiographic lung-injury scores (LIS) (C).
Figure 5. Scatterplot displaying the relation between serum level of calpains 1 h after cardiopulmonary bypass (CPB) and the clinical outcomes. The mechanical ventilation time (A), the intensive care unit (ICU) length of stay (LOS) (B), and the hospital LOS (C) are shown.
In current prospective pilot study of perioperative serum level of calpains in patients undergoing cardiac surgery using CPB technique, serum calpains’ concentrations were remarkably elevated after CPB and were paralleled with the development of ALI as well as clinical outcomes. According to the dynamic measurements, serum calpains’ level in the ALI patients present a prominent increase by comparing with non-ALI counterparts within 24 h after CPB, indicating that serum calpains’ level may serve as a potential biomarker for postoperative ALI.
Dynamic detection of serum calpains was started from the beginning of CPB. With the progress of CPB, the serum calpains’ level increased in all patients. The significant difference between two groups appeared at the end of CPB. Further, the difference has been maintained based on detection results at test time points up to 24 h after CPB. We selected two indicators of the earliest difference time points (serum level of calpains at the end of CPB and 1 h after CPB) for further logistic regression analysis, and the results suggested that only the serum level of calpains at 1 h after CPB has predictive value. Thus, the serum level of calpains at 1 h after CPB could serve as the first predictor of postoperative ALI. In the following analysis, serum calpains were related with the impaired lung function such as lower PaO2/FiO2 and LIS as well as poor clinical outcomes such as longer MV time, ICU LOS, and hospital LOS.
Mechanisms of CPB related lung injury include inflammation and ischemia-reperfusion injury. Both pathological processes can lead to the upregulation of intracellular Ca2+ (15, 16), which implies the activation of calpains. Calpains can also give positive feedback to the above two pathological processes. On one hand, calpains play an important role in inflammation. By participating in the activation of NF-κB signaling pathway, calpain could promote the expression of pro-inflammatory cytokines and adhesion molecules (23, 24). In addition, cleavage of junctional proteins by calpains promote the infiltration of inflammatory cells (18–25). Finally, calpains can be externalized during inflammation and function in the microenvironment (18). On the other hand, the ischemic process during CPB leads to mechanical stress loss of pulmonary vessels, resulting in a large influx of Ca2+ (13). In the subsequent reperfusion phase, oxygenated blood re-influxes into the lung tissue, leading to the massive production and release of oxygen free radicals and more severe calcium overload (26), processes that activate calpains and amplifies cellular damage (27, 28). The increase of calpains has been proved and to exert a negative effect during ischemia-reperfusion (28–31). In an animal model of CPB, inhibition of calpains is beneficial, which reduces endothelin-1 expression and maintains eNOS levels and, therefore, results in lower pulmonary vascular resistance (32). The role of calpains in endothelial barrier is highlighted (25, 33), up-regulation of calpains could proteolysis the juxta-membrane domain of VE-cadherin, thereby destabilizing the adhesive junction and promoting the infiltration of inflammatory cells (33), which is an important hallmark of ALI (34, 35). Notably, calpains were also found in lung microvascular cells to hydrolyze VE-cadherin, disrupting the endothelial barrier, and promoting pulmonary edema (36).
Calpains are normally perform regulatory functions intracellularly. The discovery of calpains externalization further refines their biological functions in organisms (17). Recent studies suggest that calpains are externalized through two distinct mechanisms: autonomous secretion and leakage from dying cells. Letavernier et al. noted that externalization of calpains’ activity increased when endothelial cells were exposed to VEGF-A and norepinephrine (37). Secretory modalities include cellular vesicles (38) or, via the ATP-binding cassette transporter A1 (ABCA1) (39). In the other way of externalization, calpains released from dying cells. The mechanism of externalized calpain is ambiguous. Externalized calpain could promote angiogenesis by cleaving fibronectin, accelerating the organ repair (37). However, in Limaye et al. study on acute liver injury, externalized calpain was found mediating damage to surrounding normal hepatocytes through hydrolyzing cytoskeleton proteins (40). The ubiquitously expressed of calpains make it difficult to trace the origin. In the present research, we monitored the parameters of other organs such as liver and kidney to evaluate whether high level of serum calpains could also reflect liver and kidney injury after CPB. However, the data showed that hyperactive calpains did not cause similar damage to other organs except lungs (Supplementary Table 1), which partly confirmed the specificity of serum calpains on lung injury.
The limitations of this study should be acknowledged. First, based on a single-center study, the insufficient number of enrolled patients may limit the application of our relatively correlated findings in other institutions. Hence, larger cohort studies to further validate these results were needed. Second, the detection of inflammatory cytokines was not quantified in this trial, which limited our research on the correlation between calpains and inflammatory cytokines. Third, it is difficult to determine whether elevated serum level of calpains is a cause of ALI or a consequence of CPB which leading to ALI. In this case, ALI people who have not experienced CPB need to be included in the comparison.
In current clinical trial, the potential role of serum calpains in predicting the incidence of postoperative ALI followed CPB was identified. The predictive value of which was shown as early as 1 h after CPB. At the same time, serum calpains were associated with poorer clinical outcomes. Afterall, an in-depth understanding of the role of calpains in clinical manifestations and development has the potential to better understanding of CPB-induced ALI.
The original contributions presented in this study are included in the article/Supplementary material, further inquiries can be directed to the corresponding authors.
The studies involving human participants were reviewed and approved by Medical Ethical Committee of Affiliated Hospital of Yangzhou University. The patients/participants provided their written informed consent to participate in this study. Written informed consent was obtained from the individual(s) for the publication of any potentially identifiable images or data included in this article.
WW, QW, and XW formulated the idea of the manuscript and supervised the research. CY, FC, and RM performed the research and wrote the manuscript. CY, ZF, WW, and QW carried out the operations. RM, LC, and XT collected the clinical samples. MS, QZ, QS, and GZ participated in preparing the figures, tables, and data analyzing. PL and BL provided comments and technical advice. WW and XW revised the manuscript and provided comments. All authors reviewed the manuscript.
This work was supported by National Natural Science Foundation of China (grant numbers: 82100110 and 82000106), Yangzhou Science and Technology Development Plan (grant number: YZ2022105), and Yangzhou Science and Technology Bureau (award number: YZ2022105).
The authors declare that the research was conducted in the absence of any commercial or financial relationships that could be construed as a potential conflict of interest.
All claims expressed in this article are solely those of the authors and do not necessarily represent those of their affiliated organizations, or those of the publisher, the editors and the reviewers. Any product that may be evaluated in this article, or claim that may be made by its manufacturer, is not guaranteed or endorsed by the publisher.
The Supplementary Material for this article can be found online at: https://www.frontiersin.org/articles/10.3389/fcvm.2022.1000761/full#supplementary-material
1. Zheng XM, Yang Z, Yang GL, Huang Y, Peng JR, Wu MJ. Lung injury after cardiopulmonary bypass: alternative treatment prospects. World J Clin Cases. (2022) 10:753–61. doi: 10.12998/wjcc.v10.i3.753
2. Ball L, Costantino F, Pelosi P. Postoperative complications of patients undergoing cardiac surgery. Curr Opin Crit Care. (2016) 22:386–92.
3. Huffmyer JL, Groves DS. Pulmonary complications of cardiopulmonary bypass. Best Pract Res Clin Anaesthesiol. (2015) 29:163–75.
4. Piquilloud L. ARDS after cardiac surgery: is it a problem, a problem of definition, or both? Respiration. (2019) 97:495–7.
5. Kogan A, Segel MJ, Ram E, Raanani E, Peled-Potashnik Y, Levin S, et al. Acute respiratory distress syndrome following cardiac surgery: comparison of the American-European consensus conference definition versus the Berlin definition. Respiration. (2019) 97:518–24. doi: 10.1159/000495511
6. Turnbull AE, Ruhl AP, Lau BM, Mendez-Tellez PA, Shanholtz CB, Needham DM. Timing of limitations in life support in acute lung injury patients: a multisite study. Crit Care Med. (2014) 42:296–302. doi: 10.1097/CCM.0b013e3182a272db
7. Ohata K, Chen-Yoshikawa TF, Menju T, Miyamoto E, Tanaka S, Takahashi M, et al. Protective effect of inhaled rho-kinase inhibitor on lung ischemia-reperfusion injury. Ann Thorac Surg. (2017) 103:476–83. doi: 10.1016/j.athoracsur.2016.07.067
8. Liu X, Chen Q, Luo Y, Hu Y, Lai D, Zhang X, et al. Plasma levels of alarmin HNPs 1-3 associate with lung dysfunction after cardiac surgery in children. BMC Pulm Med. (2017) 17:218. doi: 10.1186/s12890-017-0558-4
9. Goto Y, Hiramatsu Y, Ageyama N, Sato S, Mathis BJ, Kitazawa S, et al. Rolipram plus sivelestat inhibits bone marrow-derived leukocytic lung recruitment after cardiopulmonary bypass in a primate model. J Artif Organs. (2019) 22:44–52. doi: 10.1007/s10047-018-1071-0
10. Zhu Y, Peng W, Zhen S, Jiang X. Postoperative neutrophil-to-lymphocyte ratio is associated with mortality in adult patients after cardiopulmonary bypass surgery: a cohort study. Med Sci Monit. (2021) 27:e932954.
11. Pak O, Sydykov A, Kosanovic D, Schermuly RT, Dietrich A, Schröder K, et al. Lung ischaemia-reperfusion injury: the role of reactive oxygen species. Adv Exp Med Biol. (2017) 967:195–225.
12. Sun H, Zhao X, Tai Q, Xu G, Ju Y, Gao W. Endothelial colony-forming cells reduced the lung injury induced by cardiopulmonary bypass in rats. Stem Cell Res Ther. (2020) 11:246.
13. den Hengst WA, Gielis JF, Lin JY, Van Schil PE, De Windt LJ, Moens AL. Lung ischemia-reperfusion injury: a molecular and clinical view on a complex pathophysiological process. Am J Physiol Heart Circ Physiol. (2010) 299:H1283–99. doi: 10.1152/ajpheart.00251.2010
14. Friedman M, Sellke FW, Wang SY, Weintraub RM, Johnson RG. Parameters of pulmonary injury after total or partial cardiopulmonary bypass. Circulation. (1994) 90:Ii262–8.
15. Weissmann N, Sydykov A, Kalwa H, Storch U, Fuchs B, Mederos Y, et al. Activation of TRPC6 channels is essential for lung ischaemia-reperfusion induced oedema in mice. Nat Commun. (2012) 3:649. doi: 10.1038/ncomms1660
16. Itagaki K, Zhang Q, Hauser CJ. Sphingosine kinase inhibition alleviates endothelial permeability induced by thrombin and activated neutrophils. Shock. (2010) 33:381–6. doi: 10.1097/SHK.0b013e3181c6bb75
18. Miyazaki T, Akasu R, Miyazaki A. Calpain proteolytic systems counteract endothelial cell adaptation to inflammatory environments. Inflamm Regen. (2020) 40:5. doi: 10.1186/s41232-020-00114-x
19. Peltier J, Bellocq A, Perez J, Doublier S, Dubois YC, Haymann JP, et al. Calpain activation and secretion promote glomerular injury in experimental glomerulonephritis: evidence from calpastatin-transgenic mice. J Am Soc Nephrol. (2006) 17:3415–23. doi: 10.1681/ASN.2006050542
20. Liu X, Chen Q, Shi S, Shi Z, Lin R, Tan L, et al. Plasma sRAGE enables prediction of acute lung injury after cardiac surgery in children. Crit Care. (2012) 16:R91. doi: 10.1186/cc11354
21. Murray JF, Matthay MA, Luce JM, Flick MR. An expanded definition of the adult respiratory distress syndrome. Am Rev Respir Dis. (1988) 138:720–3.
22. Bernard GR, Artigas A, Brigham KL, Carlet J, Falke K, Hudson L, et al. The American-european consensus conference on ARDS. Definitions, mechanisms, relevant outcomes, and clinical trial coordination. Am J Respir Crit Care Med. (1994) 149:818–24.
23. Yi C, Wu W, Zheng D, Peng G, Huang H, Shen Z, et al. Targeted inhibition of endothelial calpain delays wound healing by reducing inflammation and angiogenesis. Cell Death Dis. (2020) 11:533.
24. Li X, Luo R, Chen R, Song L, Zhang S, Hua W, et al. Cleavage of IκBα by calpain induces myocardial NF-κB activation, TNF-α expression, and cardiac dysfunction in septic mice. Am J Physiol Heart Circ Physiol. (2014) 306: H833–43.
25. Miyazaki T, Koya T, Kigawa Y, Oguchi T, Lei XF, Kim-Kaneyama JR, et al. Calpain and atherosclerosis. J Atheroscler Thromb. (2013) 20:228–37.
26. Sharma AK, Charles EJ, Zhao Y, Narahari AK, Baderdinni PK, Good ME, et al. Pannexin-1 channels on endothelial cells mediate vascular inflammation during lung ischemia-reperfusion injury. Am J Physiol Lung Cell Mol Physiol. (2018) 315:L301–12. doi: 10.1152/ajplung.00004.2018
27. Zheng D, Cao T, Zhang LL, Fan GC, Qiu J, Peng TQ. Targeted inhibition of calpain in mitochondria alleviates oxidative stress-induced myocardial injury. Acta Pharmacol Sin. (2021) 42:909–20. doi: 10.1038/s41401-020-00526-y
28. Kim H, Zamel R, Bai XH, Lu C, Keshavjee S, Keshavjee S, et al. Ischemia-reperfusion induces death receptor-independent necroptosis via calpain-STAT3 activation in a lung transplant setting. Am J Physiol Lung Cell Mol Physiol. (2018) 315:L595–608. doi: 10.1152/ajplung.00069.2018
29. Chatterjee PK, Brown PA, Cuzzocrea S, Zacharowski K, Stewart KN, Mota-Filipe H, et al. Calpain inhibitor-1 reduces renal ischemia/reperfusion injury in the rat. Kidney Int. (2001) 59:2073–83. doi: 10.1046/j.1523-1755.2001.00722.x
30. Neri M, Riezzo I, Pascale N, Pomara C, Turillazzi E. Ischemia/reperfusion injury following acute myocardial infarction: a critical issue for clinicians and forensic pathologists. Mediators Inflamm. (2017) 2017:7018393. doi: 10.1155/2017/7018393
31. Yue RC, Lu SZ, Luo Y, Wang T, Liang H, Zeng J, et al. Calpain silencing alleviates myocardial ischemia-reperfusion injury through the NLRP3/ASC/Caspase-1 axis in mice. Life Sci. (2019) 233:116631. doi: 10.1016/j.lfs.2019.116631
32. Duffy JY, Schwartz SM, Lyons JM, Bell JH, Wagner CJ, Zingarelli B, et al. Calpain inhibition decreases endothelin-1 levels and pulmonary hypertension after cardiopulmonary bypass with deep hypothermic circulatory arrest. Crit Care Med. (2005) 33:623–8. doi: 10.1097/01.ccm.0000156243.44845.67
33. Miyazaki T, Taketomi Y, Takimoto M, Lei XF, Arita S, Kim-Kaneyama JR, et al. m-Calpain induction in vascular endothelial cells on human and mouse atheromas and its roles in VE-cadherin disorganization and atherosclerosis. Circulation. (2011) 124:2522–32. doi: 10.1161/CIRCULATIONAHA.111.021675
34. Grommes J, Soehnlein O. Contribution of neutrophils to acute lung injury. Mol Med. (2011) 17:293–307.
35. Su W, Kowalczyk AP. The VE-cadherin cytoplasmic domain undergoes proteolytic processing during endocytosis. Mol Biol Cell. (2017) 28:76–84. doi: 10.1091/mbc.E16-09-0658
36. Friedrich EE, Hong Z, Xiong S, Zhong M, Di A, Rehman J, et al. Endothelial cell Piezo1 mediates pressure-induced lung vascular hyperpermeability via disruption of adherens junctions. Proc Natl Acad Sci USA. (2019) 116:12980–5. doi: 10.1073/pnas.1902165116
37. Letavernier B, Zafrani L, Nassar D, Perez J, Levi C, Bellocq A, et al. Calpains contribute to vascular repair in rapidly progressive form of glomerulonephritis: potential role of their externalization. Arterioscler Thromb Vasc Biol. (2012) 32:335–42. doi: 10.1161/ATVBAHA.111.240242
38. Nishihara H, Nakagawa Y, Ishikawa H, Ohba M, Shimizu K, Nakamura T. Matrix vesicles and media vesicles as nonclassical pathways for the secretion of m-Calpain from MC3T3-E1 cells. Biochem Biophys Res Commun. (2001) 285:845–53. doi: 10.1006/bbrc.2001.5242
39. Hanouna G, Tang E, Perez J, Vandermeersch S, Haymann JP, Baud L, et al. Preventing calpain externalization by reducing ABCA1 activity with probenecid limits melanoma angiogenesis and development. J Invest Dermatol. (2020) 140:445–54. doi: 10.1016/j.jid.2019.06.148
Keywords: acute lung injury, calpains, cardiopulmonary bypass (CPB), PaO2/FiO2, heart surgery
Citation: Yi C, Chen F, Ma R, Fu Z, Song M, Zhang Z, Chen L, Tang X, Lu P, Li B, Zhang Q, Song Q, Zhu G, Wang W, Wang Q and Wang X (2022) Serum level of calpains product as a novel biomarker of acute lung injury following cardiopulmonary bypass. Front. Cardiovasc. Med. 9:1000761. doi: 10.3389/fcvm.2022.1000761
Received: 22 July 2022; Accepted: 24 October 2022;
Published: 16 November 2022.
Edited by:
Warwick Wolf Butt, Royal Children’s Hospital, AustraliaReviewed by:
Clarke Thuys, Royal Children’s Hospital, AustraliaCopyright © 2022 Yi, Chen, Ma, Fu, Song, Zhang, Chen, Tang, Lu, Li, Zhang, Song, Zhu, Wang, Wang and Wang. This is an open-access article distributed under the terms of the Creative Commons Attribution License (CC BY). The use, distribution or reproduction in other forums is permitted, provided the original author(s) and the copyright owner(s) are credited and that the original publication in this journal is cited, in accordance with accepted academic practice. No use, distribution or reproduction is permitted which does not comply with these terms.
*Correspondence: Wei Wang, d2VpX3dhbmc3OUAxNjMuY29t; Qiang Wang, bmp1d2FuZ3FpYW5nQDE2My5jb20=; Xiaowei Wang, d2FuZ3hpYW93ZWlAbmptdS5lZHUuY24=
†These authors have contributed equally to this work
Disclaimer: All claims expressed in this article are solely those of the authors and do not necessarily represent those of their affiliated organizations, or those of the publisher, the editors and the reviewers. Any product that may be evaluated in this article or claim that may be made by its manufacturer is not guaranteed or endorsed by the publisher.
Research integrity at Frontiers
Learn more about the work of our research integrity team to safeguard the quality of each article we publish.