- 1R2M Solution s.r.l, Pavia, Italy
- 2Department of Architectural Engineering and Technology, Delft University of Technology, Faculty of Architecture and the Built Environment, Delft, Netherlands
- 3Department of Environmental Sciences, Informatics and Statistics, University of Venice, Ca’ Foscari, Venice, Italy
- 4Department of Architecture, Korea University, Seoul, Republic of Korea
- 5Department of Engineering and Architecture, University of Parma, Parma, Italy
- 6Institute of Architectural Technology, Royal Danish Academy, Copenhagen, Denmark
- 7Anita Lawrence Chair in High Performance Architecture, School of Built Environment, University of New South Wales, Sydney, NSW, Australia
In recent decades, a new generation of “green indoor” spaces has fulfilled the latest regulations and guidelines for a carbon-neutral society. Their targets are reachable through certifications embracing sets of measures and the adverse effects on occupants. Notwithstanding this, it has constituted a significant step forward in building design. However, the challenges given by climate change and the ecological crises lead to the need for new disruptive approaches to indoor design and function, enhancing human health and adopting regenerative design” at the forefront of buildings’ conception. Besides the positive energy-performance attributes, the creation of a Regenerative Indoor Environment utilizes appropriate construction technologies and systems, to reinforce human health, and enhance users’ experience. This regenerative paradigm shift foresees putting ecosystems at the centre and the users’ psycho-physiological wellbeing, thus magnifying their collaboration. Despite regenerative design gaining some attention, a framework towards its implementation promoting the actual performances of the indoor environment is still missing, and designers do navigate among guidelines with no apparent performance indicators to be achieved, technologies to be implemented, or methodologies for post-occupancy evaluations. These three levels, constituting a stepwise methodology, are addressed by the authors within the sections of this paper, and validated as an example of the office buildings’ typology. i) What characterizes a Regenerative Indoor Environment? ii) What technical solutions underpin the realization of a Regenerative Indoor Environment? iii) What methods or standards are crucial for its evaluation? With these premises, the paper contributes to supporting the creation of a regenerative indoor’ design, by sampling and outlining regenerative indoor performances to be obtained, describing the adequate tools to implement them, as well as by displaying approaches and solutions for their final verification.
1 Introduction
In the last decades, “green” buildings have spread widely, offering an environmental performance higher than average buildings. When it comes to newly constructed buildings, “green indoors” are currently relatively common (Edwards and Naboni, 2012), and green buildings’ certification tools are often characterized by a set of Key Performance Indicators (KPIs) accessible and achievable. As a result, the adopted design, often branded as “green” or “eco-friendly,” has led to a broad market transformation. Nevertheless, these buildings primarily aim to reduce the negative impacts on the environment and humans (Cole, 2012). However, in this historical period, with climate change emerging as one of our age’s most pressing and transformative challenges, the traditional approaches to sustainable building design are found lacking.
These approaches focus on energy efficiency, resource conservation, and reduced carbon footprints. While these aspects are undeniably critical, they only address a fragment of the larger, intricate puzzle of global environmental change. Ecosystems, for instance, are vast interconnected webs of life, that provide essential services like water purification, crop pollination, and carbon dioxide sequestration. Simplified “green” building practices might not account for local ecosystems, potentially disrupting habitats or the larger environmental balance in the area.
Biodiversity, another pillar of a healthy environment, is threatened globally, with species extinction rates soaring. A building that is energy-efficient but located in a biodiversity hotspot, for example, can contribute to habitat fragmentation or loss, undermining the very purpose of its “green” label. Lastly, human health, an intricate combination of physical, mental, and emotional wellbeing, encompasses everything from exposure to natural light, which can regulate our circadian rhythms, to the need for green spaces and natural views, known to reduce stress and promote mental health. The auditory environment, air quality, and even the materials we meet, all influence our health in subtle and profound ways.
Therefore, in an era where the complexities of climate change demand comprehensive solutions, a mere focus on energy efficiency or carbon neutrality is insufficient. A holistic perspective that encompasses the intricate interplay between ecosystems, biodiversity, and the multi-faceted aspect of human health is imperative to truly address the challenges we face. It is becoming clear that buildings need to go beyond the mere reduction of their environmental impacts and should aim at positive environmental benefits. While current sustainability practices focus on moving from the conventional practice of degeneration to a neutral impact, regenerative design aims at allowing human and natural ecosystems to evolve (Figure 1).
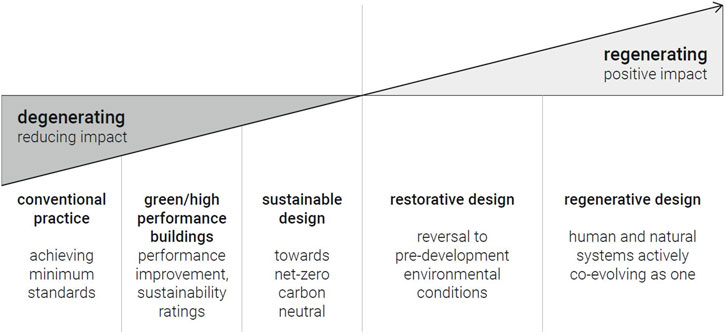
FIGURE 1. Conceptual path from conventional to regenerative practice in buildings’ design and operation. Adapted from (Brown et al., 2018).
To respond to these issues, in the 1970s J.T. Lyle pioneered the term regenerative design (Lyle, 1994). To improve Reed and R. Cole creating definitions and showing their application. The topic of regenerative design has gained importance, and it is applied in several fields linked to various disciplines. However, a body of research focused on the design of a regenerative indoor is still missing, whereas there is an increasing application of the concept of regenerative design within research that is related to the built environment.
Revisiting a broader definition (Naboni et al., 2019), a Regenerative Indoor Environment (RIE) is defined as an approach that aims to create a new set of relationships that reinforce the state of human health and natural ecosystems, utilising appropriate construction and technology targeted to indoor environments. In this perspective, the publication “Regenerative design in digital practice” (Naboni et al., 2019), presents an overview of the regenerative thinking, putting ecosystems at the centre (Ecological gain), together with the users’ psychophysiological wellbeing (Salutogenesis), to the final extent of magnifying their collaboration (Biophilia). Their definitions are proposed hereafter.
- Ecological gain. Instead of minimizing the negative impact of buildings on nature, regenerative design aims to design indoor spaces so that nature -including humans- is connected to them and they can co-evolve: every building should be considered an equivalent dynamic organism that harmoniously gives and takes from the environment, adding value to it. In this way, restoring the ecosystem capital is also a benefit to occupants.
- Salutogenesis. The term, invented by A. Antonovsky, means “generation of health” (Naboni et al., 2019) and, in this direction, the salutogenic focus is on making wellbeing part of the regenerative paradigm rather than the reductionist approach of sustainable design that targets the absence of ill health. Health-promoting environments are the new target and, as stated by J. Golembiewski, “Simple concepts like comfort, joy and aesthetics […]” become “[…] the psychological bricks and mortar of all healthy buildings” (Golembiewski, 2012).
- Biophilia. Highlighting the innate human relationship with nature, it is “the theory, science, and practice of creating buildings inspired by nature, with the aim to continue the individual’s connection with nature in the environments in which we live and work every day” (Kellert et al., 2011).
In this realm of sustainable design, indoor environments have typically focused on achieving baseline levels of comfort and health. This has often translated to minimum ventilation requirements to maintain adequate Indoor Air Quality (IAQ), ensuring thermal comfort through Heating, Ventilation, and Air Conditioning (HVAC) systems, and providing energy-efficient artificial lighting to compensate for potentially insufficient daylight. While these interventions are undoubtedly beneficial, they operate on a paradigm of “do less harm.” The energy conservation efforts, in this respect, have primarily centred around minimizing consumption—be it through efficient appliances or reducing wastage. The recurrent concept of an indoor environment results from a design and construction process meant to create a space for human beings adequate to satisfy specific needs.
Regenerative design, however, reconceptualises the indoor environment. Here, indoor spaces are not just habitats; they are ecosystems. In a regenerative paradigm, ensuring IAQ goes beyond reducing pollutants to actively revitalizing the air. This could be achieved through advanced biophilic integrations, where indoor plants play a role not just as aesthetic elements but as active air purifiers, or through architectural innovations that facilitate better natural ventilation, reducing dependency on mechanically conditioned air and thus conserving energy. Thermal comfort, too, evolves in its definition. This approach can create indoor environments that consume less energy and provide enhanced thermal comfort through passive means.
This intricate process of “making an indoor environment” must deal with the technologies and with a selection process. Here, the driver consisted in the statement that proper technology solution sets can enable a Regenerative Indoor Environment for building users and key technologies have a pivotal role in promoting a paradigm shift in building design from “less bad” to “more regenerative.” Solution sets should be meant to be resilient against the contextual dynamics, and at the same time, balanced between positive effects on the indoor environment and the use of natural sources and energy. As underlined in (Lollini and Pasut, 2020), technologies must guarantee regenerative buildings, as well as bio-aesthetics (enlivening happy and healthy people in different contexts), with favourable environmental (e.g., energy) and social impacts. By definition, the concept of a regenerative indoor is harmonized in the contest, so the amount and types of resources needed for the technology must be sourced according to circular principles.
There are a few regenerative design frameworks that attempt to embrace such a broader approach to the building design (e.g., The Living building Challenge at https://living-future.org/lbc/), The WELL Building Standard at https://www.wellcertified.com/), and many standards and guidelines have been released. All these sources prescribe performance indicators to be met, and for each indicator a value is recommended. These parameters and values differ one from another and guidelines are often controversial; thresholds are often targeting sustainable rather than Regenerative Indoor Environments, and designers have no clear framework to follow. Despite regenerative indoors being currently a common goal for many building standards and green-rating systems, a comprehensive framework to design buildings promoting health, wellbeing, high-quality indoor conditions, and kinship to nature’s tangible outcomes, is yet to be achieved. Basic principles need to be linked with real performance, new targets, development, and assessment of innovative technologies. In this direction, a framework has necessarily to start defining the aspects that make an indoor environment regenerative for its users so that, in a second phase, the technologies, with their characteristics, can be selected to cover specific regenerative needs, and ends with a methodology for its post-occupancy assessment.
In addressing the gap in regenerative indoor design, this paper seeks to elucidate regenerative indoor performances and provide designers with the appropriate tools for their execution. Central to this discourse is the overarching research question: how can designers render Regenerative Indoor Environments? The paper delineates three sub-questions to deepen our exploration: i) What characterizes a Regenerative Indoor Environment? ii) What technical solutions underpin the realization of a Regenerative Indoor Environment? iii) What methods or standards are crucial for its evaluation? Collectively, these questions form the bedrock of our methodological framework, serving as an explanatory outline and a guiding trajectory for designers navigating this field.
2 Aims and objectives
The paper is meant to support practitioners in implementing forward-thinking regenerative design by setting a framework for conscious selection of technologies within a comprehensive decision-making process. In this direction, the three main research questions provide practical answers for designers and are unfolded in the different chapters of this paper. This first phase of the work has developed a methodology to characterize a Regenerative Indoor Environment (RIE) and has validated it by focusing on office buildings. The choice of this building typology relies on the fact that the intrinsic characteristics of such facilities include all the aspects that can be then extended and adapted to other building types. Our investigation is guided by three foundational questions, each one correlating to a primary objective, in order to understand the nuances of Regenerative Indoor Environments. Each question serves as a milestone, charting the path from conceptualizing a Regenerative Indoor Environment to its realization and subsequent evaluation.
i) What characterizes a Regenerative Indoor Environment? The inception of this inquiry does not merely seek a definition but a comprehensive understanding of RIE. Key Performance Indicators (KPIs) are crucial in this exploration. While they do not provide a standalone definition of RIE, they serve as essential metrics, setting regenerative thresholds. KPIs are designers who navigate the complex terrain of crafting spaces promoting health and wellbeing without sidelining the goal of building system energy efficiency. The latter is not the centre of the paper but will be mentioned when relevant.
ii) What technical solutions underpin the realization of Regenerative Indoor Environment? With the attributes of RIE and their respective KPIs, we approach this question by examining the technological and design strategies that can facilitate such an environment. This stage is an intricate matter of innovation and integration. It mandates the interplay between various building sub-systems, ranging from construction techniques and spatial configurations to nuanced operational controls. Each element, in this mosaic, is geared towards echoing and amplifying the regenerative design ambitions.
iii) What methods or standards are crucial for its evaluation? The embodiment of a regenerative space prompts another pertinent inquiry: its effectiveness. Evaluating an RIE necessitates a blend of post-occupancy analyses and experiential feedback. Objective methodologies juxtapose with subjective evaluations to gauge the building’s performance against the established KPIs. Post-occupancy evaluations (POEs) are critical in this phase. POEs are vital instruments that refine and recalibrate design and operational strategies, foregrounding occupant comfort, satisfaction, health, and wellbeing.
3 What characterizes a Regenerative Indoor Environment? Key Performance indicators for its definition and assessment
As a primary driver of a Regenerative Indoor Environment is to demonstrably enhance occupants’ comfort and wellbeing, it is pivotal to implement a holistic approach that considers multiple aspects connected with the human-building co-existence and interactions. Focusing on office buildings, as stated in (Bergs, 2002), indoor environmental quality conditions in such facilities can affect occupants’ productivity to a high degree and impair the performance of different tasks and activities. According to (Wyon and Wargocki, 2013), workers’ general wellbeing and health, and consequently productivity and performance, are affected by quality of indoor spaces, thermal, visual, acoustic and Indoor Air Quality (IAQ) aspects, as well as availability of personal control (Fisk, 2002; Fisk et al., 2011). Different typologies of discomfort issues have the power to invalidate task-related capabilities, to the final extent of severely long-term adverse health effects (Edwards and Naboni, 2012). Indoor Air Quality and thermal comfort are recognized to be the main responsible for a worsening in productivity (Wargocki and Wyon, 2017), which implies a loss in levels of attention and a subsequent increase in cognitive effort, significantly impacting decision-making, information processing and writing capabilities. Such aspects can also lead to ill health symptoms (Wyon, 2004; Lan et al., 2011). Del Ama Gonzalo et al. (ref) performed an assessment of IAQ in residential buildings, revealing that for the 25% of a specific timeframe, the surveyed occupants were exposed to potentially hazardous concentration levels of CO2, i.e., above 1,000 parts per million (ppm). Van Tran et al. (Tran et al., 2020), in their work, provide a critical review and assessment of the major sources of pollutant emissions, pointing out their health effects and issues related to sick building syndrome (SBS) and building-related illness (BRI). In addition, the work summarizes strategies for controlling and reducing pollutant concentrations, highlighting advantages and potentials.
Besides thermal and IAQ features, which are usually the most considered, the visual environment is a key aspect in office spaces, due to its importance for conducting desk and screen reading tasks, as well as for its connection to wider psychological aspects like visual privacy. In this direction, the overall working experience can be enhanced by a good space layout design (Webb, 2006; Konstantzos and Tzempelikos, 2017), as also highlighted by the increasingly fundamental role that the visual environment is gaining in design and verification protocols (Court, 2010). According to (Heschong Mahone Group, 2003; Blume et al., 2019), the presence of windows with a view to the outdoors, an aspect that is often neglected or poorly considered, could enhance occupants’ productivity even if only by an improvement of their psychological mood, mind wellbeing and, nonetheless, by a better regulation of circadian rhythms due to the exposure to natural daylight. To investigate the contribution of daylight to occupants’ comfort, Hosseini and Ansari (Hosseini and Ansari, 2022) performed a daylight assessment of visual and non-visual effects of light shelves as daylight redirecting systems to enhance indoor daylight conditions, including the effects on circadian stimulus, visual comfort, and task performance. The study pointed out that the enhancements were minor compared to a conventional window. In another work, Moyano and al. (Moyano et al., 2020). investigated the potential hazardous effects on the retina given by blue light from artificial lighting sources. The results showed that, despite the blue light emission of the measured devices did not show high values that should be a cause for concern for eye diseases, the levels could influence circadian rhythms and sleep quality.
Finally, acoustic performance of indoor spaces is another important feature. Acoustic conditions are often associated with individual health and wellbeing issues, as reported in (Lee and Aletta, 2019), since they directly impact on auditive effort, sound privacy, communication, and task performances. High psychological distress for office workers is stated to be linked to acoustical discomfort, resulting from co-workers’ presence-related noises, lack of privacy, but also outdoor noises or in-space system noises. Workers are found to be irritated or distracted by the background noises in their workplace, e.g., noises coming from heating/cooling and ventilation systems, which can hinder their activities. For example, in (Karmann et al., 2017), the authors present IEQ survey results from 60 office buildings located in North America, including the comparison on acoustic satisfaction between radiant and all-air systems. In (Moyano et al., 2022), the possible effects on health of ultrasound exposure in working environments were reviewed, pointing out the necessity of more investigation studies on the ultrasound emissions that can be absorbed by the human body and how this can affect humans. In addition to this, both poor visual and acoustic conditions are demonstrated to potentially result in visual and acoustic pathologies, which can also negatively aggravate psychological mood and working attitudes (Boyce, 2010; Park et al., 2018).
With these premises, in (Pistore et al., 2023a) the authors identified and collected the multiple benefits that can, on the contrary, result from an improved new generation of buildings and indoor spaces. Despite their economic quantification is still research in progress, all these positive effects should be considered in an overall building performance evaluation. According to some preliminary quantifications, in (Fisk, 2002) reduced respiratory illness can account for productivity gains/annual savings around USD 6–14 billion, reduced allergies and asthma for USD 1–4 billion, reduced sick building syndrome symptoms for USD 10–30 billion, and worker performance can improve from changes in thermal environment and lighting for an estimation of USD 20–160 billion. In the EU CRAVEzero Project (CRAVEzero project 2020), an increase in productivity is the natural result of a decrease of health effects, especially when considering office environments, with reduced sick leaves estimated around 10% for and additional investment of 70 EUR/m2, and an estimated profit of 154 EUR/m2 over 30 years. According to (Almeida et al., 2017) health benefits from improved indoor climate can be monetised as a permanent annual benefit to society around EUR 42–88 billion. The authors estimate that for an additional investment of 170 EUR/m2, an increased productivity of +1% can be achieved, with a profit estimated over 30 years of around 347 EUR/m2. As the main objective of this work is to deliver a framework for the design and verification of RIEs, as a first step, a set of Key Performance Indicators (KPIs) for the indoor environment has been identified. Secondly, to each KPI, appropriate values and suitable thresholds were attributed after a proper review of the main documents and guidelines in the field, to provide designers with a comprehensive tool for the definition of a regenerative space.
Considering the wellbeing theories’ state-of-the-art over the past decades, four main conventional areas are generally considered and assessed in the context of indoor environmental conditions, i.e.,: air quality, hygro-thermal environment, visual environment, acoustic environment, which are recognized to affect both occupants’ health and energy/costs demands. These aspects can be assessed through objectively measured indoor parameters and users’ subjective responses, which are both equally significant and to be integrated in a robust overall Post-Occupancy Evaluation (POE) of indoor conditions (Zhang and Altan, 2011; Heinzerling et al., 2013; Pei et al., 2015; Leccese et al., 2021; Mujan et al., 2021). Regarding the verification of indoor conditions, national and international standards report indicators and thresholds to be fulfilled both for objective and subjective methodologies. However, often different guidelines contain different requirements and values for the same environmental aspect, and this leads to an uncertainty that complicates the navigation of such regulations, the interpretation of specifications, and does not allow generalization and real standardization. The result is a generalized controversial understanding of the required performance levels among professionals.
To delineate a structured framework for the fulfilment of a Regenerative Indoor Environment, a stepwise methodological approach was outlined referring to office buildings. The final goal was to provide a structured and repeatable tool, easily expandable to other building typologies, to be efficiently implemented in everyday practice for the design and evaluation of a RIE. As a first step, the office building environment was characterized using important environmental aspects and sub-aspects to be taken into consideration. After this identification, a literature review on state-of-the-art standards and thresholds currently applied was conducted. Results are presented in Table 1.
As it can be observed, to fulfil the initial aim and definition of a “regenerative” design, some human-nature related values were included besides the environmental aspects, which are key not only to preserve, but also to boost occupants’ satisfaction levels, health, and wellbeing. External view and right to light, together with biophilia and access to nature, were considered, to embed new potentialities to IEQ and create inspiring indoor environments. In fact, during the 21st century, one major issue resulting from artificial environments was the subsequent disconnection between nature and people. Current common design approaches usually fail in providing breakthrough solutions to the problem, since they perpetuate an anthropocentric design and thus the human-nature dichotomy. On the contrary, building’s technologies should consider all habitats’ needs, and lead to a significant improvement of occupants’ wellbeing by reconnecting their bond with other forms of life through exposure to natural elements (Browning et al., 2014).
According to the key aspects highlighted in Table 1, a list of KPIs was proposed to promote the creation of a Regenerative Indoor Environment that overcomes the limited concept of a merely sustainable building, but to achieve conditions that improve occupants’ experiences, health, wellbeing, attitudes, and psychological mood. The approach conducted to draft this list of KPIs has consisted neither of nullifying nor of erasing the regulatory requirements, instead, it has been intended as a step forward towards the achievement of a better indoor environment and reconnection with natural elements. A total of 10 KPIs were proposed, considered as the main aspects to be addressed, and for each KPI a regenerative threshold was decided. Results are shown in Table 2. Specific values were determined after an accurate evaluation of the ones gathered in the state-of-the-art documents reported in Table 1, aiming at clarifying the controversy of different sources proposing different thresholds and finally embrace an overall new perspective.
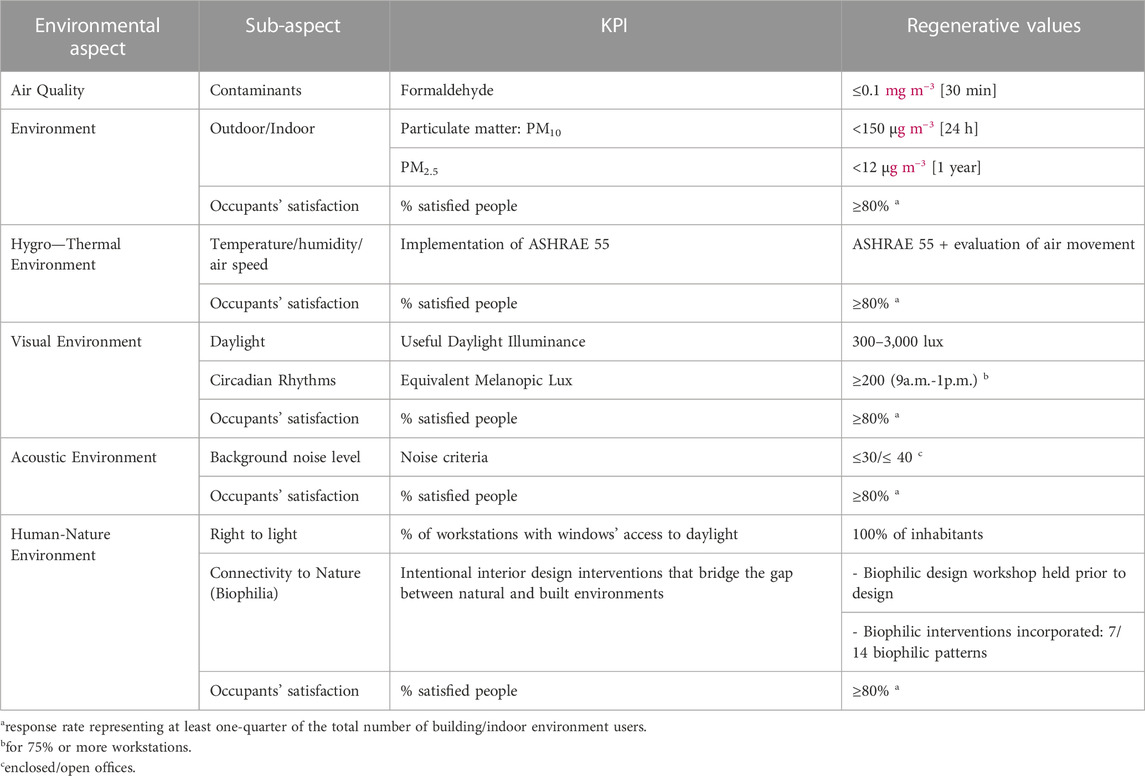
TABLE 2. List of KPIs for a Regenerative Indoor Environment and proposed regenerative values. Source (Lollini and Pasut, 2020, Table 8, p. 44).
For each KPI in Table 2, a definition is introduced hereafter.
3.1 Air quality environment
O. Fanger in his paper “What is IAQ?” (Fanger, 2006), stated that: “A paradigm shift is required, and further future shifts are foreseen where we learn how to make indoor air equally fresh and pleasant as outdoors when it is best.” In this perspective, three KPIs were selected regarding this environmental aspect, i.e., formaldehyde, PM10 and PM2.5. According to the United States Environmental Protection Agency (EPA, 2023; United States Environmental Protection Agency, 2023), formaldehyde is defined as a colourless and potentially flammable gas at room temperature. Occupants exposed to this chemical hazard may manifest different health issues, lie skin, eyes, throat, and nose irritation. A prolonged time of exposure may lead to cancer. In buildings, primarily, people are exposed to it by breathing air with off-gassed formaldehyde, coming from resins used in wood products, building materials, insulation, household and paper products. According to the World Health Organization (WHO) (World Health Organization, 2020), a short-term exposure of 0.1 mg m−3 is associated with throat and nose irritation in humans, but sensible targets can sense its presence also at lower concentrations. With these premises, an air-quality guideline value of 0.1 mg m−3 is recommended as a 30-min average, to avoid any significant sensory irritation in the average population.
PM10 (United States Environmental Protection Agency, 2023) consist in particles that are smaller than 10 microns in diameter and exposure to high concentrations of them in the air can irritate the eyes and throat. PM10 particles can be generated by sea salt, pollen, and combustion activities such as motor vehicles and industrial processes in general, but also mainly by dirt roads. In people with existing heart or lung diseases, e.g., asthma and other breathing problems, an increase in symptoms has been shown, leading to wheezing, chest tightness and difficulty breathing. PM2.5 (United States Environmental Protection Agency, 2023) particles are smaller than 2.5 μm in diameter and can therefore be inhaled deeply into the lungs, which can lead to health problems, especially in children, people over 65, pregnant women and people with existing heart or lung diseases. These particles result from the combustion of fossil fuels, organic matter, and most other materials such as rubber and plastic, and automobiles, power plant emissions, and bush fires are all major sources of fine particles. Symptoms may include wheezing, chest tightness, and difficulty breathing.
Two types of national air quality standards are established in the Clean Air Act and the EPA’s 1990s amendments to the National Ambient Air Quality Standards (U.S. Environmental Protection Agency, 2023). Primary standards provide public health protections, including protection of the health of vulnerable populations such as asthmatics, children, and the elderly. Secondary standards provide protections for the common good, including protection from reduced visibility and damage to animals, plants, crops, and buildings. Primary requirements were selected for the scope of this work aiming at a RIE and limit values of less than 150 μg m−3 for PM10 were set, averaged over 24 h, and not exceeded more than once a year on average over 3 years; less than 12 μg m−3 for PM2.5 in annual average, averaged over 3 years.
3.2 Hygro-thermal environment
The ANSI/ASHRAE Standard 55: Thermal Environmental Conditions for Human Occupancy (American Society of Heating Refrigerating and Air-Conditioning Engineers, 2020) provides the methods to be followed for the evaluation of thermal comfort conditions in accordance with the type of environment, integrated HVAC system and ventilation type. Recommended values are to comply with this standard. While the ANSI/ASHRAE Standard 55 in a sustainable approach aims to maintain conditions that preserve comfort, the regenerative interpretation of the same standard seeks to transform and elevate those conditions to spaces that are not just comfortable but are also health-generating. It suggests evolving from a reactive stance, which addresses discomfort once it arises, to a proactive stance, which anticipates and optimizes the environment for enhanced wellbeing, aligning the built environment more closely with its natural surroundings and the inherent needs of its inhabitants. To this extent, the trend of creating constant, comfortable indoor environments in buildings is today considered not healthy. Over-reliance on such environments weakens humans’ ability to handle temperature changes and can harm health. Instead, allowing more temperature variation indoors can and improve human resilience and health (reduce CO2 emissions, save resources (Pallubinsky et al., 2023).
3.3 Visual environment
In the domain of daylighting, regenerative design focuses on not just providing sufficient light, but ensuring that the light actively contributes to the wellbeing and health of occupants. The selection of the Useful Daylight Illuminance (UDI) (Nabil and Mardaljevic, 2005) as a Key Performance Indicator (KPI) embodies this philosophy. By gauging the annual occurrence of illuminances that lie within the 300–3,000 lux range on the work plane, the UDI ensures that natural light is harnessed to optimize visual comfort without resorting to excessive artificial illumination. This not only conserves energy but also creates spaces that are in harmony with their natural surroundings.
The incorporation of the Equivalent Melanopic Lux (EML) (International Well Building Institute, 2016) takes this regenerative approach a step further. Rather than just measuring light in terms of visual utility, the EML quantifies how light impacts human health at a physiological level, specifically the melanopsin-encoded response, which is crucial for regulating our circadian rhythms. By aligning with the WELL Building Standard, the emphasis shifts from illumination to the holistic wellbeing of occupants, ensuring that daylighting strategies provide visibility, while actively contributing to the physical and psychological health of building users.
3.4 Acoustic environment
Embracing the Noise Criteria (NC) as set out by the International Well Building Institute (International Well Building Institute, 2016) represents a marked shift towards a regenerative approach in indoor acoustic design. Unlike traditional strategies which simply aim to minimize or insulate against noise, the NC method actively shapes the acoustic environment. By assessing sound pressure levels and adhering to criteria curves across octave band spectra ranging from 63 to 8,000 Hz, the NC ensures that every frequency is accounted for, creating a balanced soundscape.
What makes this approach inherently regenerative is its human-centric focus. Using the WELL Building Standard as a guide, specific thresholds have been chosen: a “confidential” speech privacy level with NC ≤ 30 for private offices, ensuring intimate conversations remain private, and a “normal” speech secrecy level with NC ≤ 40 for open-plan offices, which balances openness with acoustic comfort. These values not only reduce noise, but also cultivate an environment that actively supports the activities and interactions taking place within.
By adhering to these NC values, the design not only mitigates potential acoustic disturbances but actively crafts an environment that promotes wellbeing, understanding, and effective communication. In this regenerative framework, noise is not merely a challenge to be addressed but an element to be sculpted, ensuring spaces not only sound good but feel good for their occupants.
3.5 Human-nature environment
The awareness that humans and the built environment co-exist together within natural systems is a pillar of regenerative design, intending to restore ecosystems and allowing them to thrive and evolve along with the human system. This nature-environment-human connection is an intricate relationship reflecting the profound and inherent bond between humans and their natural surroundings. This connection has roots in evolutionary biology, where early humans evolved in close harmony with nature, leading to a deep-seated affinity towards natural environments—a concept termed “biophilia” by Edward O. Wilson (use same reference used above). This connection is not just aesthetic or romantic; it has tangible physiological and psychological impacts on human health and wellbeing. To enhance the connectivity between occupants and the natural environment, two KPIs were here selected.
The percentage (%) of workstations with windows’ access to daylight (International Well Building Institute, 2016) reflects results of research showing that access to daylight and exterior views increase mood and productivity in indoor office environments (Heschong Mahone Group, 2003). This indicator consists of the percentage of all workstations within a specified distance from an atrium or window facing the outside. To significantly enhance a regenerative space, its recommended value refers to the Well Building Standard, but it was decided to extend it to a total of 100%. Connectivity to nature can be measured using the visual connection to nature (Kellert et al., 2011) as a viewing factor from the workplace. This indicator is based on evidence from visual preference research, which suggests that the preferred view is looking down a slope to a scene that includes shady clumps of trees, flowering plants, calm non-threatening animals, evidence of human habitation, and clean water. The recommendations were adapted from (Browning et al., 2014).
3.6 Occupants’ satisfaction
The percentage (%) of satisfied people, determined from the subjective answers from the users, is meant to be rated for the five selected environmental aspects, i.e., indoor air quality, hygrothermal environment, visual environment, acoustics, and human nature. Despite a value of 100% of satisfied users is desirable, a share of occupants would most certainly never be satisfied due to the intrinsic personal nature of subjective responses. For this reason, we aim at a value that is 80% or higher (American Society of Heating Refrigerating and Air-Conditioning Engineers, 2020), with a response rate representing at least one-quarter of the total number of building/indoor environment users. It can be determined by handing out subjective questionnaires to building occupants during POE campaigns. In conclusion, using the percentage of satisfied users as a key metric embodies the essence of regenerative design by centring on human wellbeing, fostering continuous engagement, and emphasizing iterative improvement.
4 What technical solutions underpin the realization of a Regenerative Indoor Environment? Solution sets for building’s sub-systems
Regenerative design, in its essence, surpasses mere sustainability by not only reducing harm but also by actively enhancing and restoring the environment it interacts with. In this context, the technologies and approaches selected should not only serve their primary functional purpose but also contribute to the holistic wellbeing of the occupants, the self-sufficiency of the building, and a positive feedback loop with the surrounding ecosystem. This means that when considering technologies, it is not just about immediate utility but also about the long-term impact, adaptability, and the potential to foster a deeper connection between the occupants and their environment. The aim is to create dynamically responsive spaces, that grow and evolve, and that heal their inhabitants over time: this necessitates new types of building components.
Building components, when viewed through a regenerative lens, embody qualities of being living, regenerative, restorative, and adaptive. “Living” components integrate biological elements, creating a symbiotic relationship between the built environment and nature. Biophilic design is an important aspect of this approach, as tamis can be pursued by the design of interiors inspired by nature and imbued with positive emotional experiences, to continue the individual’s connection with nature in the environments in which we live and work every day (Kellert et al., 2011). “Regenerative” elements actively renew and enhance resources beyond mere conservation. Regenerative features aim to reintroduce or lastly, “adaptive” components dynamically respond to evolving conditions, ensuring buildings remain resilient to climatic shift ensuring indoor thermal comfort (Romano et al., 2018). These components are pivotal to regenerative design because they transform structures from static entities into dynamic ecosystems, ensuring buildings not only coexist with nature but actively participate in nurturing and enhancing their occupant experience (Gambato and Zerbi, 2019).
To discern the optimal technologies that align with the principles of regenerative design for indoor environments and to meet the stipulated KPIs, it is paramount to elucidate the interconnectedness between identified environmental facets and their nuanced sub-elements. This involves harmonizing these facets with the intrinsic functionalities of building systems, components, and their symbiotic technologies. Core to these functionalities are the modulation of air quality, thermal regulation and resilience, illumination and glare equilibrium, acoustic harmony and mitigation, and the integration of biophilic elements to foster holistic wellbeing (Konstantinou et al., 2020).
In a regenerative framework, it is essential to recognize the interconnectedness of building components and their potential multi-functionality. Single technological solutions, when optimally leveraged, can serve more purposes. A regenerative design matches the internal conditions—encompassing a blend of ambient and surface temperatures, humidity, and airflow reminiscent of natural outdoor settings—with avenues for personal agency over these conditions, whether through manual interventions, digital adjustments, or spatial reconfigurations (Browning et al., 2014). Realizing these functions necessitates the integration of technologies across the building’s various subsystems, enveloping the building shell, internal elements, and dynamic services and controls. Therefore, our solution spectrum adopts an integrated approach, interweaving environmental considerations, functions, and subsystems. In this integrative narrative, three predominant building subsystems emerge as focal points: i) the building’s envelope, ii) adaptive interior elements and finishes, and iii) the building’s active and responsive systems, inclusive of HVAC, RES, and control mechanisms.
4.1 Building envelope
The building envelope is one of the main building components to regulate the indoor environment (Konstantinou et al., 2020) and is designed to fulfil multiple functions (Klein, 2013). Regenerative building envelopes can be defined as technological bio-based solutions, inspired by nature, adaptive, and capable of interacting with the external environment and user requirements to improve indoor comfort (Kuru et al., 2019).
The regenerative building envelopes must be able to control one or more of the environmental aspects, as outlined in Table 1. For instance, they should prevent contaminants from entering from the outside, thus promoting good Indoor Air Quality, while also providing adequate thermal resistance to address the required hygrothermal performance. In addition, the openings of the building envelope affect the visual and the acoustic environment. Furthermore, these must positively influence health, wellbeing, and quality of life through building envelope solutions that follow the natural patterns and features. By following natural patterns and features, and considering biomimetic properties, regenerative envelope solutions have the potential to revolutionize product development and have biophilic and regenerative impact.
A variety of regenerative envelope systems have already been developed, designed to react to changes in external factors and promote regenerative sustainability for the built environment. Adaption is key to achieve the regenerative impact on the indoor environment. Two main categories can be distinguished in such adaptive technologies: 1) adaptive technologies, which rely on passive design to improve indoor comfort and building energy efficiency, and 2) active technologies that include renewable harvesting (Mazzucchelli et al., 2018).
4.2 Interior elements and finishes
Several scientific studies show that we spend most of the day inside the buildings where we work and live. Great attention must be paid to the design and selection of interior elements (materials and furniture), choosing natural and eco-compatible ones, to reduce the environmental impact of the built environment and to improve indoor comfort. In addition, to decrease users stress, it is important to create inside the building indirect experience, involving contact with nature that requires ongoing human input, such as views of the nature, shapes, forms, patterns, and colour palette that feels connected to nature, along with natural light, live plants, greenery, and water features. It has been shown (Appleton, 1996) that users react positively not only towards head-on exposure to the natural environment but also, they have responded with certainty to the artificial imitation of nature and its forms in fractal patterns, and cases of organic and conceptual mimicry of the natural entities.
4.3 Active building systems
In the framework of regenerative design, thermal comfort must be achieved primarily through a proper control of thermal fluxes within the building envelope, and secondly through a well-designed and efficiently operated HVAC system (Konstantinou and Prieto Hoces, 2018). Moreover, regenerative buildings need to be designed as nearly zero-energy and nearly carbon-neutral buildings. In other words, regenerative buildings must produce all or in part the renewable energy necessary to its energy requirements (heating, cooling, electricity, hot water, etc.) and must be designed to minimize their carbon footprint across their entire life cycle span. The objective is the on-site production of the renewable energy required by the HVAC equipment and integrating in the building envelope some of its components to transform the whole building in an interactive organism able to react in a dynamic way with the external environment and to satisfy, at the same time, the user requirements. Additionally, buildings must provide on-site energy storage for resilience.
Finally, since comfort is inherently subjective, and strongly varies between people, it is important to give a degree of control to individuals, which can manifest architecturally or mechanically. Interior monitoring devices can contribute to improving indoor environmental quality. These systems continuously monitor and assess Indoor Air Quality and adjust as needed.
4.4 Framework for solution sets enabling a RIE
The sub-systems as described in the previous paragraphs and their link to the function and environmental aspect constitute a framework, which was used to collect information on existing technologies. Table 3 provides an overview of the required functions, in relation to the environmental aspect. Moreover, it shows the respective technologies that address the required function. They are grouped into categories of technologies and solutions. At the time these guidelines were drawn up, about 73 technical solutions had been collected and analysed, almost equally spread among those referring to the building envelope sub-system, to active system components, and to the interior sub-system and building finishes.
The developed framework presents technical solutions that underpin the creation of a Regenerative Indoor Environment. It is not meant to be exhaustive but to direct the designers towards integrated solutions sets that address different environmental aspects and different building subsystems. However, the decisions on which and how to apply the combinations of technologies during designing for a RIE are determined by the specific building context and the project’s objectives.
The first step is understanding the link between the environmental aspect that the design addresses and the respective function. Prioritizing the environmental aspects that contribute to Regenerative Indoor Environment it is, in the end, a design choice. This choice can be determined by the designer’s ambitions and the project objectives. It is often related to standards and regulations that require certain function and performance to reach a KPI, as discussed in the previous section. The application of regenerative technology can achieve more than one functions. For example, a green wall can remove pollutants, to cater for improved air quality, while at the same time improving thermal conductivity and bringing natural elements into the building. Table 3 presents an overview of the solutions sets and how they influence the different environmental aspect. It has been adapted from (Konstantinou et al., 2020), restructuring the tables’ content to highlight the function of the building’s sub-systems.
Furthermore, the subsystems, as defined by the discussed framework complement each other. Thus, the designer should seek combinations and synergies within the technologies. There are several passive solutions (e.g., natural ventilation, envelope shape, window coatings and manipulators) and active ways (e.g., HVAC delivery) to create inside the building the variability of natural spaces. An integrated design combines both strategies to create variability, mainly because most environments are unable to use solely natural methods due to impracticality. For example, natural ventilation has limits in very high temperature, high humidity, or high pollution periods, which has led to the development of mixed-mode cooling and ventilation HVAC systems (Kellert et al., 2011).
The interrelations between various subsystems play a pivotal role in creating RIEs. These connections emerge from a holistic approach to construction, emphasizing the use of natural materials in both the building envelope and interior spaces. For instance, incorporating materials like straw bales, clay walls, and rammed-earth façade elements not only provides insulation but also promotes the use of natural and regenerative materials. Moreover, natural materials used as interior finishes, such as bio lime and natural clay plaster, contribute to both Indoor Air Quality.
Effective HVAC control systems with Building Management Systems (BMS) and sensor integration further reinforce these interrelations. These systems ensure pollutant control and optimize window operations, thus promoting air quality and energy efficiency. Innovative materials like photocatalytic concrete and pollutant-absorbing plasterboard partitions enhance air quality and environmental sustainability.
In addition, the incorporation of green elements, such as green walls, vertical gardens, and green roofs, underscores the connection to nature. These features, when paired with intelligent irrigation systems controlled by BMS, enhance not only the wellbeing of occupants but also the building’s harmony with its surroundings, reflecting regenerative principles in architectural design.
5 What methods or standards are crucial for its evaluation? Learning from existing methodologies
The quality of indoor environments has gained increasing attention over the last decades, focusing on adapting the operational strategies of the building to the needs and attitudes of occupants and increase user confidence in energy-saving strategies. Despite the importance of addressing occupants’ comfort and health conditions, POEs are still rarely implemented in everyday professional practice, remaining a tool within the research field, even though they consist of a structured and repeatable way to assess the performance of a building after its occupation, able to deliver to designers, buildings’ owners and managers critical information for a constant verification and improvement.
During POEs, data can be collected using different tools and instrumentation (Elsayed et al., 2023), to identify the intrinsic problems of the building, operational malfunctioning, wrong management strategies, and users’ needs, sensations, and preferences. The final goal is elaborating possible solutions and the creating future knowledge. Another critical aspect, identifiable by POEs, is the occupant’s behaviour and interaction with the building: to achieve a condition of comfort in the indoor environment, the user takes action to meet their convenience. These interactions are driven by numerous diversity-driving factors influencing users’ expectations and adaptation (Pistore and Pasut, 2023), ranging from users’ cultural and social background to more momentary needs and preferences, and they affect the buildings’ performance, being the cause of the well-known gap between actual and predicted energy use (Yoshino et al., 2017).
In literature, different approaches can be found to evaluate environmental conditions within indoor spaces. Regarding the comprehensiveness of the studies, two main approaches are usually applied, whether they consider single aspects, with a focus on just one IEQ area at a time or, with a more comprehensive approach, consist in a simultaneous assessment of all IEQ areas. Despite the difficulty and the number of resources needed, a complete review of indoor environmental conditions is better achieved through a comprehensive approach, which implies the simultaneous evaluation of the thermal, olfactory, visual, and acoustic qualities perceived by occupants. Any discomfort in each one of these areas could compromise the experience of users within the whole indoor environment.
Post-occupancy evaluations can be based on two different assessment methods: i) subjective, which implies the administration of response survey questionnaires to occupants, and ii) objective, with short/long term monitoring campaigns of indoor environmental parameters. Best practices foresee the integration of both these two approaches. Subjective responses are essential to integrate assumptions made on objective measurements that cannot ultimately return the multifaceted aspects related to the stochastic nature of human subjects. Vice versa, subjective feedback, for their intrinsic nature, are different from one another, e.g., due to climatic background differences when it comes to thermal comfort preferences (Pistore et al., 2023b), and thus they can be better explained and investigated with the help of measured parameters. Various approaches can be implemented to collect all the environmental parameters to be assessed with a POE: i) transversal, ii) point in time, iii) longitudinal.
The transversal approach consists of administering survey questionnaires to the occupants through an online link. The questionnaire is usually short (within 15 min), and the answers are provided based on a satisfaction score from an evaluation scale recommended by standards. Questions shall be standardised to enable the repetition of the same survey over time and to allow benchmarking among different buildings. The point-in-time method is used to identify specific complaints and their causes, and they envisage the integration of surveys with monitoring campaigns. Information on users’ perceptions is collected with a short survey, while measurements are taken using sensors installed throughout the building. Due to the time-consuming and cost-intensive use of sensors, robust large-scale zoning of the building is usually very demanding and, therefore, restricted to a limited time per day. Longitudinal studies are typically conducted to monitor the evolution of IEQ parameters over time. Questions usually require quick feedback from the user using simple rating scales. Surveys can be integrated with objective measurements or dynamic simulation campaigns. However, the survey questionnaire typology may not be sufficient for an accurate analysis of IEQ and complaints due to its simplification and would also require an extensive installation of fixed sensors to correlate subjective and objective information over an extended period (Lollini and Pasut, 2020).
A substantial limitation of POEs is that, despite the numerous examples in literature, consistent and robust methodologies are often hard to find, and there is still the need to outline a standardised method, to implement IEQ evaluations using an efficient, structured, and repeatable approach. Although standards offer recommendations on ways of gathering user feedback in practice and performing energy audits, systematic and uniform methods are still missing and this results in limitations to an overall univocal application, to the final extent that POEs are carried out to the best knowledge of the researchers, based on experience, limited by the existing constraints (Elsayed et al., 2023). Thus, the data obtained is challenging to integrate into a comprehensive database. Nowadays, different POEs protocols are available in the market (Lollini and Pasut, 2020), and accredited certifications are being developed, contributing to the spread of such evaluations as a professional tool to investigate the performance of a building after occupancy.
6 Discussion
Regenerative design holds promise in this realm, aiming to enrich human health and nature through informed construction and technology. Our paper seeks to bridge this gap, offering insights leveraged by a framework of questions.
- What characterizes a Regenerative Indoor Environment?
The Regenerative Indoor Environment represents a holistic design paradigm that seeks to enhance human experience within built environments. It is characterized by Key Performance Indicators (KPIs) that address human perception, environmental connectivity, and holistic wellbeing. These indicators, totalling ten as identified in the research, serve as metrics that push boundaries beyond sustainability standards, aiming for a regenerative space.
RIE takes a multi-dimensional approach, emphasising objective and subjective assessments of space. While it hinges on tangible environmental factors such as air quality, acoustics, and visual elements, it prioritises occupants’ experiences, gauged through Post-Occupancy Evaluation (POE) surveys. Specific KPIs such as the Useful Daylight Illuminance (UDI) and the Equivalent Melanopic Lux (EML) demonstrate the regenerative philosophy. The former ensures optimal harnessing of natural light, while the latter quantifies light’s physiological impact, particularly its influence on circadian rhythms. Furthermore, the Noise Criteria (NC) KPI exemplifies a regenerative approach to acoustics. It does not just aim to suppress unwanted noise; it actively shapes an acoustic environment conducive to wellbeing, collaboration, and privacy.
Central to RIE is the human-nature nexus. An emphasis on biophilia, or our innate connection to nature, guides design strategies. KPIs, such as the percentage of workstations with access to natural daylight and views, validate studies pointing to enhanced mood and productivity when this connection is fostered. Moreover, the inclusion of nature further augments this bond. In conclusion, the RIE approach, validated primarily within office contexts but adaptable to other spaces, transitions from merely mitigating harm to actively fostering spaces that nourish human health and productivity.
- What technical solutions underpin the realization of a Regenerative Indoor Environment?
Achieving a RIE necessitates a profound shift from traditional sustainable building practices to a comprehensive integration of advanced technologies and architectural strategies. This design paradigm goes beyond sustainability, to actively enhancing users experience and connection to nature.
The air quality environment plays a critical role. Strategic architectural choices in the building envelope, such as green façades, double-skin façades, and state-of-the-art photocatalytic components, serve as frontline defenders against pollutants. Integrating operable windows, wind towers, and wind-directional chimneys facilitates passive air purification. Inside the building, incorporating green walls, combined with advanced coatings like photocatalytic and antibacterial TiO2, further cleanses the air. An environment augmented with atria filled with plants and natural elements is a living purifier. Complementing these passive systems, advanced ventilation systems equipped with heat recovery and a sensor-driven Building Management System (BMS) actively maintain and enhance this environment.
In the hygro-thermal environment, it is essential to harmonise passive and active measures. Natural ventilation, green walls and roofs, and double-skin façades are foundational architectural elements. Employing natural and ecological materials, combined with certified insulation materials, ensures a controlled thermal and humidity profile. When paired seamlessly with a responsive BMS and innovative envelope technologies, modern HVAC systems dynamically adjust, providing the indoor environment mirrors the natural rhythms and conditions of the outdoors. The visual environment demands a comprehensive approach. Internal shading devices, such as solar shelves, drapes, curtains, and blinds, offer architects the tools to modulate light, maximizing daylighting while controlling glare. The innovative use of switchable glazing, particularly for internal partitions, provides further adaptability. Beyond visual comfort, the auditory environment is vital. Incorporating 3D-printed sound-absorbing panels and tailored interior wall and ceiling treatments ensures a harmonious acoustic environment.
Lastly, fostering the human-nature environment bridges indoor spaces’ physical and psychological aspects. Techniques such as green façades, greenhouses crafted from ecological materials, and spaces enriched with living entities like atria filled with plants or water features serve as biophilic anchors, enhancing the innate human-nature connection. In conclusion, to achieve a Regenerative Indoor Environment, innovative technologies must be intertwined with evolved architectural practices. Every element, from the building envelope to the HVAC systems, is meticulously designed to coexist and actively regenerate the environment, crafting technologically advanced and profoundly natural spaces.
- What methods or standards are crucial for its evaluation?
Evaluating a RIE necessitates an integrated approach, and one of the most effective methods for this is the post-occupancy evaluation (POE). Regenerative design is distinct in its philosophy, aiming not just to reduce harm but to enhance the environment it encompasses actively. This emphasis makes the POE especially crucial; it does not just measure tangible metrics but holistically appraises the lived experiences and interactions between occupants and they are indoors.
The value of POE lies in its ability to combine measurable indoor metrics with pivotal feedback from building occupants. This lens of objective measurements and subjective experiences helps understand if the design regenerates and establishes a harmonious relationship between the inhabitants and their environment. When employing POE in the context of regenerative design, researchers can opt for two primary methodologies: i) a specialised approach focusing intensively on individual indoor environmental quality (IEQ) areas or ii) a comprehensive strategy that holistically assesses all IEQ domains simultaneously. Given the all-encompassing nature of regenerative design, the comprehensive approach is often more suitable. It offers a well-rounded assessment, capturing the thermal, olfactory, visual, and acoustic facets intrinsic to the regenerative logic.
Data collection methodologies, such as transversal, point-in-time, or longitudinal approaches, play a role in evaluating regenerative attributes. These methods provide insights into the design’s adaptability, resilience, and evolving regenerative qualities. Structured questionnaires are crucial to considering regenerative design via a POE. These evaluative tools, rooted in industry standards and academic literature, ensure the design’s regenerative principles are validated and refined, guaranteeing that the environment remains dynamically responsive and regenerative throughout its operations.
7 Conclusion
The transition to regenerative indoors underscores the need to minimise environmental harm and actively regenerate both human and natural ecosystems within indoor spaces. The RESTORE publication (Naboni et al., 2019) has delineated some primary contours of regenerative design. Still, a cohesive framework translating these theoretical edifices into tangible building components and practical design remains elusive. This research strives to bridge that gap. The main findings are related to the primary question that framed the paper, which centre around the definition of Regenerative Indoor Environment the solution sets for the building components that enable realising RIE, and, finally, the methodologies to evaluate RIE.
The emphasis is on the indoor built environment and its close relationship with human experience. This study offers a layered exploration of the RIE, enhancing the existing literary fabric while highlighting areas for future exploration and introducing measurable constructs. Key Performance Indicators like the Useful Daylight Illuminance (UDI), the Equivalent Melanopic Lux (EML) and several others have been revisited within the specific context to operationalise the theoretical underpinnings of RIE. Furthermore, a framework was crafted to ensure the theories find actionable ground. It systematically identifies the nexus between technologies, environmental paradigms, their performance, and the function of resultant solutions. This analytical lens categorically peruses three building subsystems: the building envelope, interior elements and finishes, and active systems. Regarding evaluating RIE, Post-Occupancy Evaluation emerges as one of the most effective methods. While POE’s pedigree in architectural research is well-established, its nuanced application in the RIE framework is novel. Here, POE internally incorporates quantifiable environmental metrics and human feedback according to an expanded scope.
The technical solutions of the framework that has been developed underpin the creation of a regenerative, indoor environment. Moreover, it does not aim at offering ready-made solutions sets, but rather directs designers towards integrated solution-sets that address different environmental aspects and separate building’s subsystems. Recommendations for designers lay in the link between aspects for RIE and the functional requirement of the solution per component, as well as the interrelation between the functions.
While the study aims to offer holistic insights, it also has constraints. Practical challenges in implementing regenerative designs, potential economic implications, trade-offs, or conflicts between diverse indoor environmental quality metrics remain outside this study’s purview. The research also stops short of offering empirical validation of the efficacy of regenerative design or a granular guide to POE’s practical application. Future research could explore these areas, offering more pragmatic insights.
In conclusion, this manuscript emerges as a reference for professionals navigating the terrains of architecture, engineering, and building design, elucidating regenerative design’s practical contours. It also underscores the pivotal role of subjective perceptions and KPIs in gauging indoor regenerative ambience. The framework serves as a blueprint, guiding professionals and researchers through the maze of regenerative design, offering both a theoretical and actionable roadmap. Though the regenerative design of indoors is robust in its philosophy, the regenerative design demands continual refinement and iteration. While this research offers foundational insights, it is but a step in the expansive journey of Regenerative Indoor Environments. The challenge and opportunity, for future researchers and practitioners is to further these discussions, evolve the paradigms, and continue the quest for regenerative indoors.
Data availability statement
The original contributions presented in the study are included in the article/supplementary material, further inquiries can be directed to the corresponding authors.
Author contributions
All authors contributed to conceptualization and methodology of the study, to the writing of original draft, review and editing. All authors read and approved the submitted version.
Funding
This work was conducted within the European Cooperation in Science and Technology COST Action CA16114 RESTORE.
Acknowledgments
The work presented in this paper is part of the COST Action RESTORE WG4. The authors would like to thank all the COST Action Participants, who have contributed to the work, particularly Roberto Lollini (co-leader of WG4), Francesco Fiorito, Rosa Romano and Antonia Sore who contributed to the development of the Repository of Regenerative Technological Solutions, and Martin Brown for his precious insights on the KPIs regarding the human-nature relationship and biophilic aspects.
Conflict of interest
Author LP was employed by R2M Solution s.r.l.
The remaining authors declare that the research was conducted in the absence of any commercial or financial relationships that could be construed as a potential conflict of interest.
Publisher’s note
All claims expressed in this article are solely those of the authors and do not necessarily represent those of their affiliated organizations, or those of the publisher, the editors and the reviewers. Any product that may be evaluated in this article, or claim that may be made by its manufacturer, is not guaranteed or endorsed by the publisher.
References
Almeida, M., Ferreira, M., Rodrigues, A., Höfler, K., Maydl, J., Venus, D., et al. (2017). Co-benefits of energy related building renovation-demonstration of their impact on the assessment of energy related building renovation (annex 56) energy in buildings and communities programme author of parametric calculations of generic buildings. Avaliable at: www.iea-ebc.org.
American Society of Heating Refrigerating and Air-Conditioning Engineers (ASHRAE) (2020). ANSI/ASHRAE standard. Atlanta: ASHRAE.
Appleton, J. (1996). The experience of landscape. Wiley. https://books.google.nl/books?id=ucafAAAAMAAJ.
ASHRAE (2022). ANSI/ASHRAE standard 62-1 ventilation for acceptable indoor air quality. Atlanta: ASHRAE.
Bergs, J. (2002). The effect of healthy workplaces on the well-being and productivity of office workers. Int. Plants People Symposium 2, 1–12.
Blume, C., Garbazza, C., and Spitschan, M. (2019). Effects of light on human circadian rhythms, sleep and mood. Somnologie 23 (3), 147–156. doi:10.1007/s11818-019-00215-x
Boyce, P. R. (2010). Review: the impact of light in buildings on human health. Indoor Built Environ. 19 (1), 8–20. doi:10.1177/1420326X09358028
Brown, M., Haselsteiner, E., Apró, D., Kopeva, D., Lucas, E., Pulkkinen, K., et al. (2018). Sustainability, restorative to regenerative. COST action CA16114 RESTORE, working Group one report: restorative sustainability. Bolzano, Italy: Urbanity - Architektur, Kunst, Kultur & Sprache.
Browning, W. D., Bryan, C. O., and Clancy, J. O. (2014). 14 patterns of biophilic design. New York: Terrapin Bright Green llc. Avaliable at: https://www.terrapinbrightgreen.com/reports/14-patterns/#content.
Building Research Establishment (BRE) (1990). BREEAM, building research establishment environmental assessment method. Avaliable at: https://bregroup.com/products/breeam/.
Cole, R. J. (2012). Transitioning from green to regenerative design. Build. Res. Inf. 40 (1), 39–53. doi:10.1080/09613218.2011.610608
Court, A. (2010). The health risks associated with prolonged sitting: a systematic review. JBI Libr. Syst. Rev. 8 (16), 1–19. doi:10.11124/jbisrir-2010-626
Edwards, B. W., and Naboni, E. (2012). Green buildings pay, design, productivity and ecology. London and New York: Routledge.
Elsayed, M., Pelsmakers, S., Pistore, L., Castaño-Rosa, R., and Romagnoni, P. (2023). Post-occupancy evaluation in residential buildings: a systematic literature review of current practices in the EU. Build. Environ. 236, 110307. doi:10.1016/j.buildenv.2023.110307
EPA (2023). Particulate matter (PM) pollution. Avaliable at: https://www.epa.gov/pm-pollution.
European Committee for Standardization (CEN) (2006). EN ISO 7730: ergonomics of the thermal environment – analytical determination and interpretation of thermal comfort using calculation of the PMV and PPD indices and local thermal comfort criteria. Brussels: ISO.
European Committee for Standardization (CEN) (2019). EN 16798-1/2: energy performance of buildings – ventilation for buildings – Part 1: indoor environmental input parameters for design and assessment of energy performance of buildings addressing indoor air quality, thermal environment, lighting and acousti. Brussels: ISO.
European Committee for Standardization (CEN) (2021). EN 12464-1: light and lighting - lighting of work places - Part 1: indoor work places. Brussels: ISO.
Fisk, W. J., Black, D., and Brunner, G. (2011). Benefits and costs of improved IEQ in U.S. Offices. Indoor Air 21 (5), 357–367. doi:10.1111/j.1600-0668.2011.00719.x
Gambato, C., and Zerbi, S. (2019). The regenerative building: a concept of total sustainability. IOP Conf. Ser.: Earth Environ. Sci. 323, 12061. doi:10.1088/1755-1315/323/1/012061
Golembiewski, J. (2012). There’s something in my head (but it’s not me): the complex relationship between the built environment and schizophrenia. Sydney University.
Heinzerling, D., Schiavon, S., Webste, T., and Arens, E. (2013). Indoor environmental quality assessment models: a literature review and a proposed weighting and classification scheme. Build. Environ. 70, 210–222. doi:10.1016/j.buildenv.2013.08.027
Heschong Mahone Group (2003). Appendix of windows and offices: a study of office worker performance and the indoor environment. Calif. Energy Commision 37 (4), 414–435.
Hosseini, S. N., and Ansari, I. S. (2022). A daylight assessment on visual and nonvisual effects of light shelves: a human-centered simulation-based approach. J. Daylighting 9 (1), 28–47. doi:10.15627/jd.2022.3
International Living Future Institute (ILFI) (2022). The living building challenge. Avaliable at: https://living-future.org/lbc/.
International Well Building Institute (IWBI) (2016). WELL building standard. Avaliable at: https://standard.wellcertified.com/well.
Karmann, C., Schiavon, S., Graham, L. T., Raftery, P., and Bauman, F. (2017). Comparing temperature and acoustic satisfaction in 60 radiant and all-air buildings. Build. Environ. 126, 431–441. doi:10.1016/j.buildenv.2017.10.024
Kellert, S. R., Heerwagen, J., and Mador, M. (2011). Biophilic design: the theory, science and practice of bringing buildings to life. Wiley. Avaliable at: https://books.google.nl/books?id=FyNer_nQrW4C.
Klein, T. (2013). Integral Facade Construction: towards a new product architecture for curtain walls. TU Delft.
Konstantinou, T., and Prieto Hoces, A. (2018). “Environmental design principles for the building envelope and more _: passive and active measures,” in Editors T. Konstantinou, N. Cukovic, and M. Zbasnik (TU Delft Open), 147–180.
Konstantinou, T., Romano, R., and Fiorito, F. (2020). “Solution-sets for a regenerative environment,” in Regenerative technologies for the indoor environment: inspirational guidelines for practitioners. Editors R. Lollini, and W. Pasut (Bolzano: European Cooperation in Science and Technology COST), 137–164. Avaliable at: https://www.eurestore.eu/publications-and-articles/.
Konstantzos, I., and Tzempelikos, A. (2017). A holistic approach for improving visual environment in private offices. Procedia Environ. Sci. 38, 372–380. doi:10.1016/j.proenv.2017.03.104
Kuru, A., Oldfield, P., Bonser, S., and Fiorito, F. (2019). Biomimetic adaptive building skins: energy and environmental regulation in buildings. Energy Build. 205. doi:10.1016/j.enbuild.2019.109544
Lan, L., Wargocki, P., Wyon, D. P., and Lian, Z. (2011). Effects of thermal discomfort in an office on perceived air quality, SBS symptoms, physiological responses, and human performance. Indoor Air 21 (5), 376–390. doi:10.1111/j.1600-0668.2011.00714.x
Leccese, F., Rocca, M., Salvadori, G., Belloni, E., and Buratti, C. (2021). Towards a holistic approach to indoor environmental quality assessment: weighting schemes to combine effects of multiple environmental factors. Energy Build. 245, 111056. doi:10.1016/j.enbuild.2021.111056
Lee, Y., and Aletta, F. (2019). Acoustical planning for workplace health and well-being: a case study in four open-plan offices. Build. Acoust. 26 (3), 207–220. doi:10.1177/1351010X19868546
Lollini, R., and Pasut, W. (2020). Regenerative technologies for the indoor environment. Inspirational guidelines for practitioners. Avaliable at: www.cost.eu/COST_Actions/ca/CA16114.
Lyle, J. T. (1994). “Regenerative design for sustainable development,” in Wiley series in sustainable design (New York: John Wiley). Avaliable at: https://worldcat.org/title/1245774116.
Mardaljevic, J., Andersen, M., Roy, N., and Christoffersen, J. (2012). Daylighting, artificial lighting and non-visual effects study for a residential building. Loughborough, United Kingdom. Avaliable at: http://infoscience.epfl.ch/record/181055/files/JM-MA-VeluxReport-FINAL_1.pdf.
Mazzucchelli, E. S., Romano, R., Aelenei, L., Gomes, M. da G., Karlessi, T., Alston, M., et al. (2018)“Passive Adaptive Façades – Examples from COST TU1403 Working Group 1,” in Facade 2018–Adaptive! TU Delft Open.
Moyano, D. B., Arranz Paraiso, D., and Alonso González-Lezcano, R. (2022). Possible effects on health of ultrasound exposure, risk factors in the work environment and occupational safety review. Healthcare 10 (3), 423. doi:10.3390/healthcare10030423
Moyano, D. B., Sola, Y., and Alonso González-Lezcano, R. (2020). Blue-light levels emitted from portable electronic devices compared to sunlight. Energies 13 (16), 4276. doi:10.3390/en13164276
Mujan, I., Licina, D., Kljajić, M., Čulić, A., and Aleksandar, S. (2021). Development of indoor environmental quality index using a low-cost monitoring platform. J. Clean. Prod. 312, 127846. doi:10.1016/j.jclepro.2021.127846
Nabil, A., and Mardaljevic, J. (2005). Useful daylight illuminance: a new paradigm for assessing daylight in buildings. Light. Res. Technol. 37 (1), 41–57. doi:10.1191/1365782805li128oa
Naboni, E., Chokhachian, A., Finnochiaro, L., and Havinga, L. (2019). Regenerative design in digital practice - a handbook for the built environment. Bolzano, Italy: Eurac Research.
Pallubinsky, H., Kramer, R. P., and van Marken Lichtenbelt, W. D. (2023). Establishing resilience in times of climate change - a perspective on humans and buildings. Clim. Change 176 (10), 135. doi:10.1007/s10584-023-03614-0
Park, S. H., Lee, P. J., and Jeong, J. H. (2018). Effects of noise sensitivity on psychophysiological responses to building noise. Build. Environ. 136, 302–311. doi:10.1016/j.buildenv.2018.03.061
Pei, Z., Lin, B., Liu, Y., and Zhu, Y. (2015). Comparative study on the indoor environment quality of green office buildings in China with a long-term field measurement and investigation. Build. Environ. 84, 80–88. doi:10.1016/j.buildenv.2014.10.015
Pistore, L., and Pasut, W. (2023). “Roots and mechanisms of thermal comfort expectations: from individuals’ own background to adaptation and change BT,” in Proceedings of the 5th international conference on building energy and environment (Singapore: Springer Nature Singapore).
Pistore, L., Tintinaglia, F., Pernetti, R., Stivanello, P., and Pasut, W. (2023b). Indirect effects of high-performance buildings at household and community level: a systematic literature review. Energies 16 (5), 2499. doi:10.3390/en16052499
Pistore, L., Varin, C., and Pasut, W. (2023a). Development of climate-based thermal comfort ranges from existing data: analysis of the smart controls and thermal comfort (SCATS) database. Energy Build. 298, 113509. doi:10.1016/j.enbuild.2023.113509
Romano, R., Aelenei, L., Aelenei, D., and Mazzucchelli, E. S. (2018). What is an adaptive façade? Analysis of Recent Terms and definitions from an international perspective. J. Facade Des. Eng. 6 (3), 65–76. doi:10.7480/jfde.2018.3.2478
The Chartered Institution of Building Services Engineers (CIBSE) (1999). Daylighting and window design. London: CIBSE.
Tran, V. V., Park, D., and Lee, Y. C. (2020). Indoor air pollution, related human diseases, and recent trends in the control and improvement of indoor air quality. Int. J. Environ. Res. Public Health 17 (8), 2927. doi:10.3390/ijerph17082927
United States Environmental Protection Agency (EPA) (2023). Formaldehyde. Avaliable at: https://www.epa.gov/formaldehyde.
U.S. Environmental Protection Agency (EPA) (2023). National ambient air quality standards (NAAQS). Avaliable at: https://www.epa.gov/criteria-air-pollutants/naaqs-table (Accessed March 30, 2023).
U.S. Green Building Council (USGBC) (2021). LEED rating system. Avaliable at: https://www.usgbc.org/leed.
U.S. National Institute for Occupational Safety and Health (NIOSH) (2020). Pocket guide to chemical hazards. Avaliable at: https://www.cdc.gov/niosh/npg/default.html (Accessed March 30, 2023).
U.S. Occupational Safety and Health Administration (OSHA). (2023). Code of federal regulations. Avaliable at: https://www.graphicproducts.com/articles/osha-29-cfr-1910/(Accessed March 30, 2023).
Wargocki, P., and Wyon, D. P. (2017). Ten questions concerning thermal and indoor air quality effects on the performance of office work and schoolwork. Build. Environ. 112, 359–366. doi:10.1016/j.buildenv.2016.11.020
Webb, A. R. (2006). Considerations for lighting in the built environment: non-visual effects of light. Energy Build. 38 (7), 721–727. doi:10.1016/j.enbuild.2006.03.004
World Health Organization (WHO) (2020). Air quality guidelines for europe. 2nd Edition. Copenhagen, Denmark: World Health Organization Regional Office for Europe.
Wyon, D. P. (2004). The effects of indoor air quality on performance and productivity. Indoor Air 14 (s7), 92–101. doi:10.1111/j.1600-0668.2004.00278.x
Wyon, D. P., and Wargocki, P. (2013). How indoor environment affects performance. Ashrae J. 55, 46–52.
Yoshino, H., Hong, T., and Nord, N. (2017). IEA EBC annex 53: total energy use in buildings—analysis and evaluation methods. Energy Build. 152, 124–136. doi:10.1016/j.enbuild.2017.07.038
Keywords: regenerative design, regenerative indoor environment, key performance indicators, technologies, post-occupancy evaluations
Citation: Pistore L, Konstantinou T, Pasut W and Naboni E (2023) A framework to support the design of a regenerative indoor environment. Front. Built Environ. 9:1225024. doi: 10.3389/fbuil.2023.1225024
Received: 18 May 2023; Accepted: 04 December 2023;
Published: 20 December 2023.
Edited by:
Song Pan, Beijing University of Technology, ChinaReviewed by:
Roberto Alonso González-Lezcano, CEU San Pablo University, SpainClaudio Martani, Purdue University, United States
Copyright © 2023 Pistore, Konstantinou, Pasut and Naboni. This is an open-access article distributed under the terms of the Creative Commons Attribution License (CC BY). The use, distribution or reproduction in other forums is permitted, provided the original author(s) and the copyright owner(s) are credited and that the original publication in this journal is cited, in accordance with accepted academic practice. No use, distribution or reproduction is permitted which does not comply with these terms.
*Correspondence: Lorenza Pistore, lorenza.pistore@r2msolution.com; Thaleia Konstantinou, t.konstantinou@tudelft.nl