- 1Holzforschung Austria, Bioenergy and Analytical Chemistry, Vienna, Austria
- 2Technisches Büro Für Chemie – Dr. Karl Dobianer, Vienna, Austria
Indoor air quality (IAQ) was investigated in 13 newly-built, occupied timber houses on a long-term basis. The study included a comparison of the construction types timber-frame (TF) and solid wood (SF), in addition two different ventilation types, controlled vs. window ventilation, were contrasted. The emission progression of volatile organic compounds (VOCs) including formaldehyde, was recorded and compared with the subjective well-being of the residents, which was identified by use of standardized questionnaires. This was completed with toxicological assessment and repeated measurements of specific medical parameters of health indicative character. It was found that VOC-emissions were initially elevated regardless of construction and ventilation type. However, after a period of up to 8 months emissions mostly decreased to an average level. Whereas, the SW constructions released distinctly more terpenes compared to the TF houses, there was no significant difference regarding the total concentration of emitted VOCs. The use of controlled ventilation systems resulted in lower VOC-concentrations and thus in higher IAQ compared to window ventilation. From a toxicological point of view the major part of the investigated houses were unobtrusive and IAQ was considered as “high” or “satisfactory.” Residents were continuously very satisfied with their health and quality of life. This perception was confirmed by the results gained from the accompanying medical examinations, giving no indication for physical impairments.
Introduction
Interior spaces and IAQ significantly affect human physical and mental well-being, especially in working and living environments, where a considerable amount of time over lifetime is spent (Mølhave et al., 1986; Skov et al., 1990). IAQ is strongly affected by the presence of organic pollutants, such as VOCs or formaldehyde. These substances can be related to material sources e.g., building materials, consumer products, or human activities (Wolkoff, 1995; Brown, 2009).
Considering recent health-related European requirements on the release of dangerous substances from construction products into indoor air (CPR, 2011), the competitiveness of wood, representing a naturally VOC-emitting material, could be restricted.
Wood-specific emissions have been studied by various authors. Few studies involve toxicological or health-related issues. Mersch-Sundermann (2007) performed comprehensive health assessment of α-pinene in indoor air. Gminski et al. (2011) evaluated irritation and pulmonary effects of acute exposure to the emissions from oriented strand boards. Fürhapper et al. (2011) investigated the emissions of several wood species and wood-products focussing toxicological aspects. However, most studies are based on standardized testing conditions and do not consider the overall interior-situation where many different emission-sources are present. Furthermore, long-term investigations in a real-life environment are rare. Wallner et al. (2015) investigated IAQ in occupied buildings at two dates (3 months and 1 year after move-in), but this study has no relationship to neither wood nor health-issues. Low-energy wooden houses were evaluated by Patkó et al. (2013). Schulte-Hubbert et al. (2013) studied indoor air emissions as occurring during the construction of a timber frame building. Indoor air measurements were accompanied by cellbiological tests, showing no emission related cytotoxic effects. Nyrud et al. (2012) evaluated the impact of wood-use in a hospital environment with respect to VOC-emissions and IAQ. Therein, the equipment of hospital rooms with hardwood wall panels had no effect on the interior VOC-concentration, as measured 8 months after installation.
Central issue of the present study is the investigation of the actual IAQ in newly-built wooden houses and its potential impact on the house's occupants. Initial VOC emissions, as occurring immediately after construction work, are expected to be elevated. Main objective is to gather information on the extent and progression of these emissions within the first months after occupation and to evaluate if short-term exposure to such concentrations has detectable health-related effects. Overall the research shall provide new knowledge on the living conditions in timber constructions.
Materials and Methods
Testing Sites and Test Persons
Thirteen testing sites were randomly chosen depending on their availability in the eastern part of Austria and Bavaria. All testing sites were private homes. They included six TF constructions, six SW constructions, and one concrete building, representing a non-wooden reference. Aim of the comparison of construction types was to evaluate a potential correspondence between wood proportion in the building structure and wood specific emissions indoors.
Nine houses were equipped with constantly operated controlled ventilation systems, whereas four sites were vented by window ventilation, performed several times a day. Eleven sites had wooden floorings, mostly designed in oak, with various coatings. Non-wooden floorings consisted of tile and vinyl. A detailed overview of the investigated testing site is accessible as Supplementary Material to this article.
One adult test person was appointed per household, whereby an equal gender distribution (seven males, six females) was considered. The age of the test persons was between 28 and 42 years. By their own account the test persons were in good health conditions and did not show medical peculiarities at the beginning of the study.
Sampling Procedure
Up to eight samplings were carried out in the sleeping rooms of each testing site at regular intervals (mostly monthly). Initial samplings took place at a late state of construction, hence served as reference measurements for building product emissions. Subsequent periodical sampling events started shortly after move-in.
Indoor Air Parameters
VOCs were analyzed according to ISO 16000-6 by active sampling on Tenax TA® tubes and thermal desorption (Markes Int. Ltd., Unity2, UltraTD) coupled with a gas chromatography—mass spectrometry (GC-MS) system (Agilent GC6890N, Agilent MSD 5973), operated with a Phenomenex Zebron 5 MSi column (60 m × 0.25 mm × 0.25 μm). Sampling took place at a fixed height of 1.5 m with a minimum distance of 1.0 m from walls by use of an automated sampling pump (Chematec FLEC air pump 1001) at an air flow rate of 100 ml/min (sampling volume: 6 l). Thermal desorption operating parameters: 5 min desorption at 280°C, 5 min cold trapping at −3°C, abrupt trap desorption at 300°C and split ratio of 1:1; temperature program: start temperature 40°C, 10°C/min until 100°C, 25 min at 300°C; MS parameters: electron ionization (EI) mode, scan-mass-range from 30 to 300, analysis via target and qualifying ions and total ion current (TIC). Qualitative data assessment was carried out via comparison of the specific mass spectra with commercial spectra libraries (NIST MS Search 2.0) and substance specific retention times. Quantitative assessment was based on a substance specific calibration (including up to 75 typical indoor air substances) considering the peak areas of the target ions, cyclodecane was used as internal standard. The calibration-range was between 1 and 250 μg/m3. Substances not included in the specific calibration were evaluated as toluene-equivalents.
Formaldehyde and other short-chain carbonyl-compounds were analyzed according to ISO 16000-3 by active sampling on 2,4-dinitrophenylhydrazine (DNPH) cartridges (Supelco 54278-U), subsequent elution and measurement by use of a high-performance liquid chromatography (HPLC) system with diode array detection (Thermo Scientific, Dionex Ultimate3000), operated with a C18 column (Thermo Scientific, Dionex Acclaim™ Carbonyl C18, 3 μm, 120 Å 3.0 × 150 mm). Sampling took place according to the VOC-conditions by use of an automated sampling pump (KNF Laboport®) at an air flow rate of 1.2 l/min (sampling volume: 99 l). Gradient elution was performed with mixtures of acetonitrile (A) and ultrapure water (B) at a flow of 0.6 ml/min; Gradient program: 0–4.5 min: equilibration at 53% A, 47% B; 4.5–12.5 min: 53% A, 47% B, 16.5–20 min: 100% A, 100% B. Detection wavelength: 360 nm. Qualitative data assessment was carried out via the retention times of the substances. Quantitative assessment was based on a calibration considering the peak areas of the specific compounds. The calibration range was between 1 and 610 μg/m3.
Medical Parameters
Recording of medical data was solely based on non-invasive diagnostic methods. Upper arm blood pressure and pulse monitors (Braun, ExactFit™3 BP6000) were distributed to all participating households. As an indication for their general health status, the test persons were instructed to measure and record blood pressure and pulse once a week.
As wood-specific-emissions like terpenes or aldehydes can cause irritations affecting the mucous membranes and respiratory system (Sagunski and Heinzow, 2003; Ad-hoc-Arbeitsgruppe, 2009), these substance-groups were considered of special relevance. In order to assess potential burdens regarding the respiratory system, the pulmonary function of the testing persons was measured at each sampling event using a spirometer (Geratherm Respiratory GmbH, Spirostik™). Recorded parameters included vital capacity (VC), forced expiratory volume in 1 s (FEV1) and forced vital capacity (FVC).
Changes in the eye blink frequency can indicate insufficient IAQ, e.g., caused by terpene oxidation products (Klenø and Wolkoff, 2004). Therefore, the test persons were filmed during a consistent activity (mostly while fulfilling the questionnaires) using a camera with video function (Sony DSC RX100). Blinking per minute was counted afterwards by video analysis.
Repeated surveys were conducted to evaluate the subjective well-being and health condition of the test persons. The questionnaires included specific questions in the fields of respiratory reactions, mental pressure, quality of sleep, quality of life, and general well-being and were designed specifically for this study.
Data Evaluation
The assessment of the detected TVOC-concentrations refers to the Austrian guideline for the assessment of indoor air quality (BMLFUW, 2005) and to the guide- and reference-values published by the German committee on indoor guide values (Ad-hoc-Arbeitsgruppe der IRK/AGLMB, 2007), respectively, both focusing on the TVOC-value (Total Volatile Organic Compounds, sum of all volatile compounds eluting between n-hexane and n-hexadecane on a non-polar or slightly-polar GC-column). In the Austrian guideline a total concentration of <0.25 mg/m3 is considered “low,” whereas 0.25 to 0.5 mg/m3 represent an “average” range. Up to 1 mg/m3 the concentration is regarded as “slightly elevated,” representing an expected condition immediately after construction work when using solvent-free materials. A concentration range between 1 and 3 mg/m3 is considered “distinctly elevated,” indicating the presence of VOC-sources. According to the German committee on indoor guide values human exposure is tolerable for a period of up to 12 months. Concentrations exceeding 3 mg/m3 represent a “strongly elevated” and “hygienically concerning” TVOC-level, that is to be expected immediately after construction work when using solvent-based materials. Whereas, human exposure is tolerable for a period of up to 1 month at a level of 3–10 mg/m3, habitat use shall be avoided if 10 mg/m3 are exceeded, thus representing inacceptable conditions (Ad-hoc-Arbeitsgruppe der IRK/AGLMB, 2007).
The assessment of the detected formaldehyde-concentration refers to the air quality guidelines for Europe published by the World Health Organization (WHO, 2000).
When comparing construction and ventilation types, statistical significance was evaluated using an independent t-test. Due to the low sample size (n = 12 for construction types and n = 13 for ventilation types), only highly statistical effects were recognized.
The toxicological evaluation is based on the substance specific VOC-concentrations that were detected in the houses. It refers to the TIAC-system (Fürhapper et al., 2011), which stands for Tolerable Indoor Air Concentration and represents the indoor air concentration of a substance that is tolerated even by very sensitive persons over a lifetime. Apart from characteristic toxicological values like NOAEL (No Observed Adverse Effect Level), LOAEL (Lowest Observed Adverse Effect Level), NIK (Niedrigst interessierende Konzentration) and occupational exposure concentrations, the TIAC-system further considers odor threshold values. In general, TIAC-assessment refers to the lowest available, plausible reference values, hence occasionally leading to stricter or else more lenient limiting values compared to EU-LCIs (European-Lowest Concentration of Interest, as of Dec. 2016). Substance-mixtures are evaluated by dividing the detected single substance concentrations (ci) by their corresponding TIACs. Added up this leads to the specific hazard index (HI) of the mixture.
While HIs < 1 are considered toxicologically safe, HIs between 1 and 4 are increasingly conspicuous, whereas HIs > 4 require remedial actions.
The questionnaires were digitalized and evaluated from a medical point of view by descriptive statistics based on progressive graphs and calculation of scattering and location parameters like arithmetic mean, quartiles, minimum, maximum, and standard deviation.
Results
Long-Term Emission Development
Initial VOC-emissions, as observed during construction state and shortly after moving-in, were elevated regardless of construction and ventilation type. Considering all 12 wooden constructions, 1 month after the date of move-in a TVOC mean value of 2.2 mg/m3 was measured. In two of the investigated sites a TVOC-concentration of 3 mg/m3 was exceeded, thus implying a hygienic concern.
In the further course emission curves showed a decreasing tendency until, after 6–8 months, a slightly elevated or average level, in dependence of the construction type, was reached. Seven months after move-in the SW sites showed a TVOC mean value of 0.75 mg/m3. As for the TF constructions, a TVOC mean value of 0.43 mg/m3 was calculated.
This decline of emissions contrasts with the detected formaldehyde-concentrations, that on average stayed continuously on a low level, undercutting the recommended 24 h average value of 60 μg/m3 of the WHO air quality guidelines for Europe (WHO, 2000). Only one SW testing site showed an elevated formaldehyde-concentration of 345 μg/m3 during construction state.
Figure 1 shows the emission development based on the TVOC and formaldehyde as a comparison of construction types. Whereas, TVOC-emission-maxima were mostly reached around the date of move-in, formaldehyde emissions followed a steady course on average. Concerning the TVOC-progression as shown in Figure 1A, no significant difference was found between the construction types SW and TF construction (p = 0.225). Compared to the concrete reference construction, both wooden construction types showed higher TVOC-emissions in construction state and at the beginning of utilization phase. Emissions declined significantly with time (p < 0.001) and reached an emission level in the range of the concrete site after ~7 months. Regarding the formaldehyde-emissions (Figure 1B) SW construction types were found to release significantly higher formaldehyde concentrations than TF constructions (p = 0.022). When comparing the wooden construction types to the concrete site no obvious difference in concentration range was found.
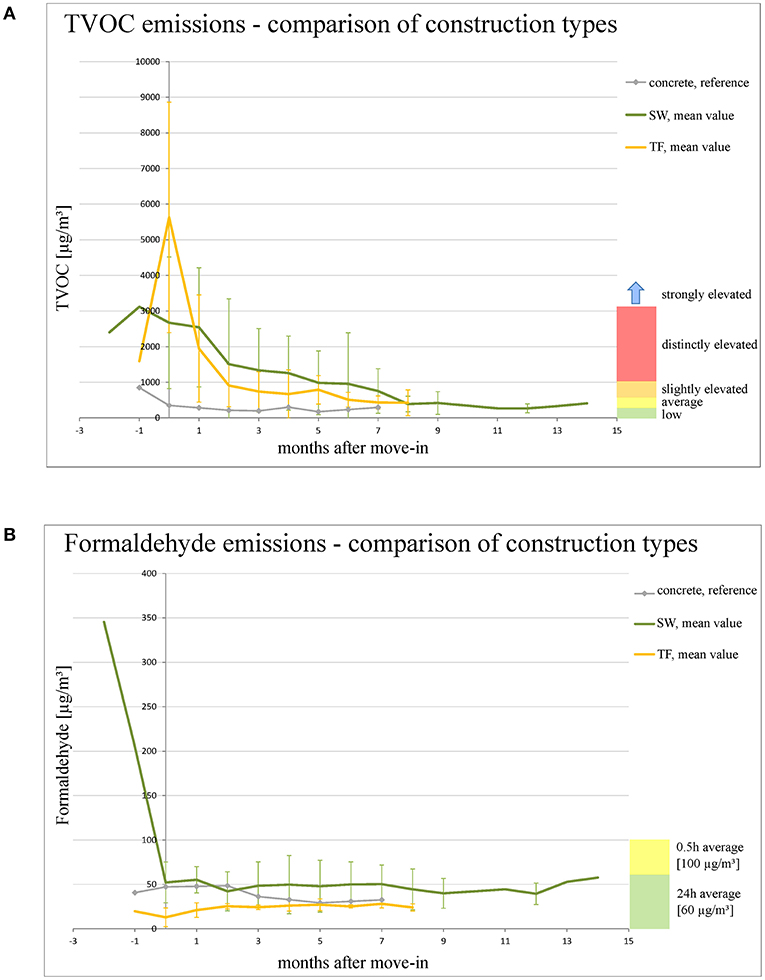
Figure 1. Long-term progression of TVOC (A) and formaldehyde (B) emissions—comparison of construction types.
Figure 2 presents a comparison of the emission course of manually vented houses and testing sites equipped with controlled ventilation systems. The first data points of controlled ventilated sites are not considered relevant, as the ventilation systems were subject to adjustments during the first weeks after installation. The figures show clearly that the use of controlled ventilation systems resulted in lower pollutant-concentrations. Although not statistically significant (p = 0.059), probably caused by the small sample number, TVOC-emissions as shown in Figure 2A are distinctly higher in testing sites with window ventilation. The same is valid for formaldehyde (Figure 2B), manually vented testing sites showed a significant (p = 0.013) higher release.
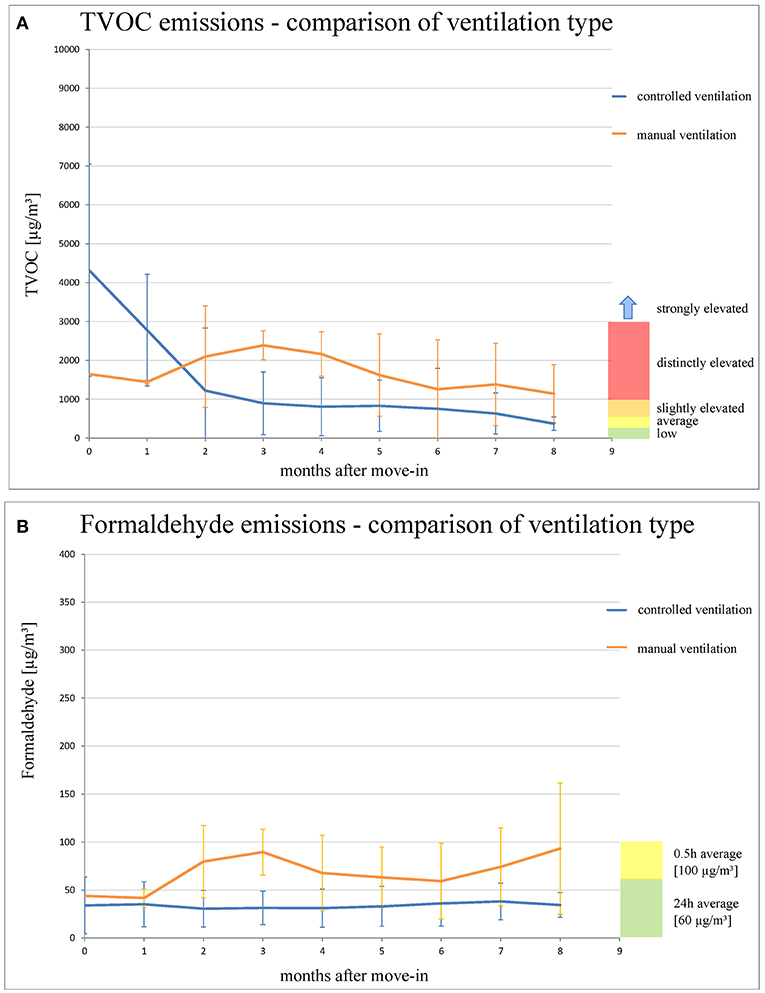
Figure 2. Long-term progression of TVOC (A) and formaldehyde (B) emissions—comparison of ventilation types.
Apart from the TVOC, emissions were also evaluated according to their substance specific composition with special focus on wood-related compounds. Therefore, TVOC was subdivided into the substance classes terpenes, aldehydes, and organic acids, representing the most important emission groups associated with wood and wood-based materials (Risholm-Sundman et al., 1998; Makowski et al., 2005). In addition, the category “other” summarizes all other detected substances, consisting of alkanes, ketones, alcohols, esters, aromatic compounds, and siloxanes. A comparison of the average TVOC-composition in the investigated construction types over the entire investigation period is given in Figure 3. With 44% of the totally detected VOCs, terpenes represented the major emissions in SW constructions. Aldehydes contributed with 29% and organic acids with 13% to the TVOC, leaving 14% of “other” substances. TF constructions showed a rather equal allocation between terpenes, aldehydes and “other” substances (30/34/28%), organic acids amounted to 8%. The remarkable difference in terpene-emissions between the two construction types is statistically not significant (p = 0.084). The concrete-built reference construction emitted mostly aldehydes and “other” substances (41/35%), terpenes accounted for 17% and organic acids for 7% of the total emissions.
When examining chronological trends, it became apparent that the TVOC composition shifted during the observation period. While initial emissions could be related to building products, flooring, and new furniture for the most part, assignment of the detected VOCs became more difficult at a later stage, as the impact of user habits gained in importance.
Toxicological Assessment of Indoor Environment
Except for the construction-state, most of the investigated testing sites were considered toxicologically unobtrusive using the TIAC-system. Figure 4 shows an exemplary comparison of the calculated HIs 8 months after move-in. As an exception, for the testing sites 7, 8, and 12, where samplings were completed at an earlier stage (6 and 7 months, respectively), the HI of the last sampling-date is presented. Ten testing sites exhibit a HI < 1, implying toxicologically safe conditions. Two sites showed HIs between 1 and 2, indicating slight conspicuities. In one site the HI was calculated as 3.4, marking distinctly conspicuous conditions that were in all likelihood linked to the presence of specific building products.
Taking into account all calculated HIs throughout the entire investigation period, seven testing sites showed constantly good or very good IAQ (HI around or below 1), while four sites were classified as satisfactory. In two cases the toxicological assessment showed constant or temporary peculiarities, which are probably related to either specific building materials or certain user habits (e.g., the usage of air refreshers).
Medical Evaluation
As an outcome from the questionnaires, it appeared that health-related self-perception of the test persons remained rather constant within the entire investigation period. Well-being was rated as excellent; no abnormal symptoms or impairments were reported. The quality of sleep (falling asleep, sleeping through the night) was perceived highly satisfying. Concerning respiratory health, no evident symptoms or impairments were detected. Neither eye- nor mucosal irritations were reported at any time. Health and quality of life was generally rated as highly positive.
The pulmonary function parameters VC, FEV1, and FVC are dependent on age and sex of the test persons. Therefore, evaluation was based on a comparison to the reference values of an age- and sex-matched normal control group. None of the test persons showed substantial changes of their pulmonary function over time. Even if the initial level was below or above 100% compared to the reference values, no changes were detected during the investigation period.
The assessment of systolic and diastolic blood pressure and pulse measurements showed in no case peculiarities, results were in accordance with standard values for healthy adults.
Depending on environmental conditions and activities, the natural variation range of the blinking frequency is large. Literature values range between 1 and 22 blinks per minute during reading (Bentivoglio et al., 1997). Most measured values were within this range but showed distinctive interindividual differences. Overall no conspicuities were determined.
Discussion
VOC-emissions measured in newly built and occupied timber houses were initially elevated, as is often the case in newly established buildings (Brown, 2002). At this stage the occurrence of increased emissions was independent of construction and ventilation type. Building products, flooring and new furniture could be accounted for the major sources. However, after a period of up to 8 months, emissions decreased mostly down to an average level. This chronological emission-decline coincides with the observations of Stratev et al. (2016) who investigated the long-term emission progress of softwood-constructions based on real-life measurements and a modeling approach.
Comparing the TVOC emission-course of the construction types SW and TF, no significant differences were found. In qualitative terms, the investigated SW constructions resulted in remarkably higher terpene-release. Considering the higher proportion of timber in SW constructions and terpenes representing the major emissions of softwood (Risholm-Sundman et al., 1998), which is mainly used as construction timber in European regions, this finding complies with previously generated hypotheses. Compared to the concrete construction, both timber construction types showed higher TVOC-emissions within the first months but decreased to a similar concentration range subsequently.
Even if constantly on a safe level, SW constructions showed higher formaldehyde-release compared to TF and the concrete construction. This can also be explained by the higher proportion of timber in solid wood construction.
The equipment of buildings with controlled ventilation systems resulted in lower VOC concentrations and thus in higher IAQ compared to window ventilation. This corresponds to the observations of Wallner et al. (2015), who found that in buildings with mechanical ventilation, TVOC- and formaldehyde-emissions were significantly lower compared to manually vented houses.
Toxicologically, the major part of the investigated houses appeared unremarkable except for the construction-state, where a large number of different substances, mainly originating from building products, was detected. While being occupied, indoor air of the houses was classified as of high or satisfactory quality. Temporary peculiarities in one site could be explained by certain user habits.
The medical evaluation revealed a very positive self-assessment of the study participants regarding their health and well-being. This perception was confirmed by accompanying orienting medical examinations, giving no indication for physical impairments with respect to the respiratory and cardiovascular system, even at an early state, when emissions were still elevated.
Due to its descriptive character, this study does not claim absolute statistical robustness. However, results demonstrate realistic trends and provide an important data base for future research under real-life conditions.
Data Availability Statement
The datasets for this study will not be made publicly available because Emission-testing and medical measurements were performed in occupied private houses. Due to confidentiality agreements and obligations toward the study participants we are not able to publish raw datasets.
Ethics Statement
As this study is of non-interventional character, approval through an ethical commission is not obligatory as per Austrian legislation. The herein presented data are not identifiable and do not allow any conclusion about individual study participants. All participants freely gave informed consent in writing for the anonymous use of their personal data. Appropriate data protection measures were taken, and the project was registered at the Austrian data protection authority (assigned DVR-number: 1005316). Complying with good scientific practice, participants were informed about general outcomes and results of the study at the end of the project.
Author Contributions
As project leader, CF was responsible for the overall-evaluation and wrote the manuscript. EH and DS performed and evaluated indoor air measurements. MW was involved in project planning and coordination. KD was responsible for the toxicological evaluation.
Funding
This research was an exclusive part of the European project Wood2New, Competitive wood based interior materials and systems for modern wood construction. Funding was provided by WoodWisdomNet+ via the Austrian Federal Ministry of Agriculture, Forestry, Environment and Water management and the European Confederation of the Woodworking Industries–CEI Bois.
Conflict of Interest
KD operates the company “Technisches Büro für Chemie.”
The remaining authors declare that the research was conducted in the absence of any commercial or financial relationships that could be construed as a potential conflict of interest.
Acknowledgments
The authors express their gratitude to Thomas Waldhör, who supported this research by contributing to the statistical evaluation. H.P.-Hutter is thanked for provision of his medical experience, on which the medical evaluation of this research is based.
Supplementary Material
The Supplementary Material for this article can be found online at: https://www.frontiersin.org/article/10.3389/fbuil.2019.00151/full#supplementary-material
Supplementary Material: Overview of the investigated testing sites including construction types, ventilation systems and flooring details.
References
Ad-hoc-Arbeitsgruppe (2009). Richtwerte für gesättigte azyklische aliphatische C4- bis C11-Aldehyde in der Innenraumluft. Bundesgesundheitsbl Gesundheitsf Gesundheitsschutz 52, 650–659. doi: 10.1007/s00103-009-0860-2
Ad-hoc-Arbeitsgruppe der IRK/AGLMB (2007). Beurteilung von Innenraumluftkontaminationen mittels Referenz- und Richtwerten. Bundesgesundheitsbl Gesundheitsf Gesundheitsschutz 50, 990–1005. doi: 10.1007/s00103-007-0290-y
Bentivoglio, A. R., Bressman, S. B., Cassetta, E., Carretta, D., Tonali, P., and Albanese, A. (1997). Analysis of blink rate patterns in normal subjects. Mov. Disord. 12, 1028–1034. doi: 10.1002/mds.870120629
BMLFUW (2005). Richtlinie zur Bewertung der Innenraumluft. Arbeitskreis Innenraumluft im Bundesministerium für Land und Forstwirtschaft, Umwelt und Wasserwirtschaft. Wien: Kommission für Reinhaltung der Luft der Österreichischen Akademie der Wissenschaften.
Brown, S. K. (2002). Volatile organic pollutants in new and established buildings in Melbourne, Australia. Indoor Air 12, 55–63. doi: 10.1034/j.1600-0668.2002.120107.x
Brown, S. K. (2009). “Building products as sources of indoor organic pollutants,” in Organic Indoor Air Pollutants. Occurrence, Measurement, Evaluation, eds T. Salthammer and E. Uhde (Braunschweig: Wiley VCH), 373–404. doi: 10.1002/9783527628889.ch16
Fürhapper,C Dobianer, K., Aschacher, G., Eichholzer, A., and Prantl, S. (2011). VOC-Emissionen und Toxikologische Aspekte. Endbericht. Projektnr.: 818628. Wien: Holzforschung Austria. Available online at: https://www.researchgate.net/publication/331488749_VOC-Emissionen_aus_Holz_und_toxikologische_Aspekte (accessed March, 2019).
Gminski, R., Marutzky, R., Kevekordes, S., Fuhrmann, F., Bürger, W., Hauschke, D., et al. (2011). Chemosensory irritations and pulmonary effects of acute exposure to emissions from oriented strand board. Hum. Exp. Toxicol. 30, 1204–1221. doi: 10.1177/0960327110388537
Klenø, J., and Wolkoff, P. (2004). Changes in eye blink frequency as a measure of trigeminal stimulation by exposure to limonene oxidation products, isoprene oxidation products and nitrate radicals. Int. Arch. Occup. Environ. Health 77, 235–243. doi: 10.1007/s00420-003-0502-1
Makowski, M., Ohlmeyer, M., and Meier, D. (2005). Long-term development of VOC emissions from OSB after hot-pressing. Holzforschung 59, 519–523. doi: 10.1515/HF.2005.086
Mersch-Sundermann, V. (2007). Gesundheitliche Bewertung von alpha-Pinen in der Innenraumluft-aktueller Erkenntnisstand. Umweltmed Forsch Prax 12, 129–151.
Mølhave, L., Bach, B., and Pedersen, O. F. (1986). Human reactions to low concentrations of volatile organic compounds. Environ. Int. 12, 167–175. doi: 10.1016/0160-4120(86)90027-9
Nyrud, A. Q., Bringslimark, T., and Englund, F. (2012). Wood use in a hospital environment: VOC emissions and air quality. Eur. J. Wood Prod. 70, 541–543. doi: 10.1007/s00107-011-0578-3
Patkó, C., Patkó, I., and Pásztory, Z. (2013). Indoor air quality testing in low-energy wooden houses: measurements of formaldehyde and VOC-s. Acta Polytech. Hungarica 10, 105–116. doi: 10.12700/APH.10.08.2013.8.6
Risholm-Sundman, M., Lundgren, M., Vestin, E., and Herder, P. (1998). Emissions of acetic acid and other volatile organic compounds from different species of solid wood. Holz als Roh- und Werkstoff 56, 125–129. doi: 10.1007/s001070050282
Sagunski, H., and Heinzow, B. (2003). Richtwerte für die innenraumluft: bicyclische terpene (Leitsubstanz a-Pinen). Bundesgesundheitsblatt Gesundheitsforschung Gesundheitsschutz 46, 346–352. doi: 10.1007/s00103-003-0584-7
Schulte-Hubbert, F., Rehmers, A., Schuster, A., Gminski, R., and Huraß, J. (2013). Messung der Innenraumluftemissionen während der Bauphase eines modernen Holzhauses. Gefahrst. Reinhalt. L. 73, 81–86.
Skov, P., Valbjorn, O., and Pedersen, B. V. (1990). Influence of indoor climate on the sick building syndrome in an office environment. The Danish Indoor Climate Study Group. Scand. J. Work Environ. Health 16, 363–371. doi: 10.5271/sjweh.1772
Stratev, D., Fürhapper, C., Niedermayer, S., Habla, E., Nohava, N., and Weigl, M. (2016). From model rooms towards a modelled indoor environment. Int. Wood Prod. J. 7, 195–201. doi: 10.1080/20426445.2016.1222254
Wallner, P., Munoz, U., Tappler, P., Wanka, A., Kundi, M., Shelton, J. F., et al. (2015). Indoor Environmental quality in mechanically ventilated, energy-efficient buildings vs. conventional buildings. Int. J. Environ. Res. Public Health 12, 14132–14147. doi: 10.3390/ijerph121114132
WHO (2000). Air Quality Guidelines for Europe, 2nd ed. Copenhagen: WHO Regional Publication; European Series.
Keywords: indoor air quality, timber constructions, living comfort, long-term emissions, toxicological assessment
Citation: Fürhapper C, Habla E, Stratev D, Weigl M and Dobianer K (2020) Living Conditions in Timber Houses: Emission Trends and Indoor Air Quality. Front. Built Environ. 5:151. doi: 10.3389/fbuil.2019.00151
Received: 14 March 2019; Accepted: 30 December 2019;
Published: 22 January 2020.
Edited by:
Dimitrios Kraniotis, OsloMet–Oslo Metropolitan University, NorwayReviewed by:
Mohamed F. Yassin, Kuwait Institute for Scientific Research, KuwaitRiina Johanna Muilu-Mäkelä, Natural Resources Institute Finland (Luke), Finland
Copyright © 2020 Fürhapper, Habla, Stratev, Weigl and Dobianer. This is an open-access article distributed under the terms of the Creative Commons Attribution License (CC BY). The use, distribution or reproduction in other forums is permitted, provided the original author(s) and the copyright owner(s) are credited and that the original publication in this journal is cited, in accordance with accepted academic practice. No use, distribution or reproduction is permitted which does not comply with these terms.
*Correspondence: Christina Fürhapper, Yy5mdWVyaGFwcGVyQGhvbHpmb3JzY2h1bmcuYXQ=