- 1Department of Oceanography and Coastal Sciences, College of the Coast and Environment, Louisiana State University, Baton Rouge, LA, United States
- 2Bert S. Turner Department of Construction Management, Louisiana State University, Baton Rouge, LA, United States
- 3Coastal Studies Institute, Louisiana State University, Baton Rouge, LA, United States
- 4Eustis Engineering, Baton Rouge, LA, United States
The physical properties of soil can affect the stability of construction. In particular, soil swelling potential (a term which includes swelling/shrinking) is often overlooked as a natural hazard. Similar to risk assessment for other hazards, assessing risk for soil swelling can be defined as the product of the probability of the hazard and the value of property subjected to the hazard. This research utilizes past engineering and geological assessments of soil swelling potential, along with economic data from the U.S. Census, to assess the risk for soil swelling at the census-block level in Louisiana, a U.S. state with a relatively dense population that is vulnerable to expansive soils. Results suggest that the coastal parts of the state face the highest risk, particularly in the areas of greater population concentrations, but that all developed parts of the state have some risk. The annual historical property loss, per capita property loss, and per building property loss are all concentrated in southeastern Louisiana and extreme southwestern Louisiana, but the concentration of wealth in cities increases the historical property loss in most of the urban areas. Projections of loss by 2050 show a similar pattern, but with increased per building loss in and around a swath of cities across southwestern and south-central Louisiana. These results may assist engineers, architects, and developers as they strive to enhance the resilience of buildings and infrastructure to the multitude of environmental hazards in Louisiana.
Introduction
Soil that tends to swell or shrink as moisture content changes is known as expansive soil. Hazardous “swelling” is closely related to heaving when moisture is added to the soil. Problematic “shrinking” occurs when the soil becomes extremely dry. No matter which mechanism of movement occurs, swelling or shrinking, the hazard is known as “expansive soil” (Holtz and Hart, 1978). Expansive soils represent a separate process of soil movement from subsidence, and the two are generally not associated with each other. Subsidence is gradual sinking of landforms to a lower level because of earth movement from the long term consolidation of soft clays due to historical fill placement/surface loading, lowering groundwater tables or natural long-term consolidation.
Expansive soils present a hazard to lightweight buildings and other infrastructure. Uneven settling and shifting in such structures may occur, causing cracks in foundations, walls, streets, driveways, and sidewalks; ruptured pipes; and windows and doors that do not open and close properly. In the 1970s, sixty percent of the 250,000 new homes built on expansive soils each year in the U.S. experienced minor loss and 10 percent sustained significant damage (Jones and Holtz, 1973; Holtz and Hart, 1978). The U.S. Department of Agriculture estimates that 50 percent of the households in the U.S. are constructed on expansive soils, and the American Society of Civil Engineers estimates that one-quarter of all homes in the U.S. are affected (Virginia Department of Mines, Minerals and Energy, 2021). In a typical year, expansive soils cause a greater financial loss to property owners than earthquakes, floods, hurricanes, and tornadoes combined, at a cost of up to $9 billion/year in the U.S. in the 1980s (Jones and Jones, 1987) and perhaps up to $15 billion by the 1990s (Nelson and Miller, 1992). FEMA (1982) projected that residential building losses related to property and income would be $997.1 million by 2000 (1970$). Unlike many other environmental hazards, the effects of expansive soil are insidious in that they are not revealed suddenly or caused by a single event, but rather become increasingly evident and destructive over time. Unfortunately, recent comprehensive studies on the risk attributable to the expansive soil hazard are lacking in the literature.
Given the wide range of cost estimates and the lack of recent analysis, the purpose of this research is to introduce a more elaborate, transparent, updated, data-intensive method of calculating the risk associated with expansive soils in Louisiana, a U.S. state with relatively dense population that is vulnerable to the effects of expansive soils. Because soil features and development are highly heterogeneous across space, the scale of analysis is at the census-block level. Specifically, the three primary objectives are to: 1) characterize the expansive soil swelling potential–the percentage of soil swell from optimum to saturated moisture content (Çimen et al., 2012; Fattah et al., 2021) across Louisiana; 2) project the future swelling potential of expansive soil in Louisiana; and 3) assess future property loss in Louisiana due to expansive soil, considering anticipated changes to climate and population. The results will benefit developers, property owners, and mitigation specialists within and beyond Louisiana as they seek new and improved ways to characterize, anticipate, and prepare for the expansive soil hazard.
Background
Modern, scientific, soil swelling measurements and characterization date back for over a half century, when Seed et al. (1962) evaluated the utility of the plasticity index [“liquid limit” percentage minus “plastic limit” percentage (Coleman and Douglas 2008)] for such purposes, but the plasticity index was later shown to be impractical in humid environments (Jones 2012). Tripathy et al. (2004) characterized swelling of clays, such as bentonites (Bharat and Gapak, 2018) used as barrier materials for storing radioactive waste, and Watanabe and Yokoyama (2021) did similar work with clay/sand mixtures. Rao et al. (2004) suggested that free swell index, identified experimentally using the ratio of the difference between the oven-dried soil volume in water vs. in kerosene to the final volume of soil in kerosene (Holtz and Gibbs, 1954), can circumvent the need for considering the many other soil properties when estimating swelling potential. Ferber et al. (2009) examined the effects of water (or liquid limit) and density on swelling potential in clays. Frikha et al. (2013) measured the lateral motion of kaolin clay when reinforced by stone column. A new instrument was developed recently (Hobbs et al., 2014) and tested (Hobbs et al., 2019) for measuring shrinkage of clays.
In addition to measuring expansive soils, substantial research has been invested in recent years in modeling swelling potential. For example, Çimen et al. (2012) developed and validated a simple multiple regression model to calculate the potential for expansive clays based on water content and plasticity index. Lim and Siemens (2016) identified an upper-bound called the swelling equilibrium limit (SEL) and developed a predictive model for SEL in various soils. Yang et al. (2019) used this parameter in a numerical model. The soil water retention curve has also been found to be useful as a predictive tool for swelling (Tu and Vanapalli, 2016). Eyo et al. (2019) developed and validated a model for characterizing swelling of clays by core mineralogy, microfabrics, grain size, and suction response. Abbey et al. (2020) continued along this research track by characterizing the swelling potential for high-plasticity clays blended with cement. Neural network approaches have also been taken (e.g., Erzin, 2007).
Treating expansive soils has also received attention in the scholarly literature. Geotechnical engineers typically include cementitious additives [e.g., lime (Kasangaki and Towhata, 2009; Jung and Santagata, 2014) and fly ash (Puppala et al., 2001; Nalbantoğlu 2004; Hozatlıoğlu and Yilmaz, 2021)], non-cementitious additives [e.g., stone dust (Reddy et al., 2015)], chemical additives [e.g., calcium chloride or magnesium hydroxide (Bhuvaneshwari et al., 2020) or sodium silicate (Reddy et al., 2015)], or gypsum (e.g., Yilmaz and Civelekoglu, 2009) as a stabilizing agent. Guar gum biopolymers (Acharya et al., 2017), commercially available polymers (Taher et al., 2020), wood/paper industry waste (Ijaz et al., 2020), hydrophobic polyurethane foam (Al-Atroush and Sebaey, 2021), along with physical methods such as granulated tire rubber (Patil et al., 2011) and pile anchoring systems (Sfoog et al., 2020) have also been suggested. Comprehensive experiments on expansion rates and treatment options for expansive soils are provided in Al-Rawas and Goosen (2006) and Zumrawi et al. (2017). It should be noted that adding stabilizing agents or any foreign substance to soils can occasionally increase the shrink/swell potential of the subsoils. While the current study does not account for this possibility, it is recommended that the use of additives be evaluated carefully prior to their mixture with existing soils.
Collectively, the research is rich regarding engineering aspects of the expansive soil hazard, including measurement, modeling, and mitigation, but there is a dearth of research on a data-driven link between the hazard and the historical and probable future loss. This paper will be the first to project future property loss at the micro-scale, considering the changing swelling potential due to climate change, property value, and population.
Methods and Materials
Study Area
Louisiana, U.S., is selected for this analysis because its propensity for natural hazards has inspired improvements in its state hazard mitigation plan. The expansive soils hazard, while formidable in its own right in Louisiana, can exacerbate and be exacerbated by changes in other Louisiana hazards, such as intense precipitation, flooding, and extreme heat and cold.
Swelling soils in Louisiana have received little scholarly attention. While Mississippi’s löess bluffs [and presumably, Louisiana’s as well (Heinrich, 2008)] have been found to have little shrinking or swelling and low plasticity (Krinitzsky and Turnbull, 1967; Snowden and Priddy, 1968), Coleman and Douglas (2008) suggested that since so much of Louisiana has swelling potential that is only on the fringe of being hazardous, engineers have typically ignored the problem in the past. This increases the potential for loss, especially as the soils in the southeastern U.S., including Louisiana, have undergone increasingly frequent extremes of wet (Carter et al., 2014) and dry (Schubert et al., 2021) conditions in recent years, with both extreme precipitation and drought expected to become more commonplace in the future (Wehner et al., 2017). Coleman and Douglas (2008) cautioned that some lean clay soils in Louisiana can have dangerous swelling potential even at water contents near or below the plastic limit. Vertisols in Louisiana and elsewhere have been noted to impact the distribution of organic matter because of swelling and other motions (Kovda et al., 2010). Montmorillonite mineral content in the northern section of the state has been particularly problematic for swelling (Khan et al., 2017). Coastal Louisiana marsh soils are also known to swell and shrink due to high-frequency variability in local hydrology and groundwater features (Cahoon et al., 2011).
Wang et al. (2017) developed a contour map of swelling potential based on data from Seed et al. (1962). The map produced by Olive et al. (1989) also includes Louisiana. But the spatial distribution of property loss due to expansive soils has received even less attention. Despite the focus on Louisiana here, the method is applicable in other locations.
Data
Wang’s (2016) point-based swelling potential map is used here to represent the spatial distribution of historical expansive soil conditions–the natural component of the risk. That map had been developed based on data measured by Seed et al. (1962). Future projection of the hazard here relies on information from the fourth National Climate Assessment (NCA4; U.S. Global Change Research Program, 2017). The human component of the risk relies on Louisiana census-block shapefiles, which are downloadable from the United States Census Bureau (2010), and population projections based on data from United States Census Bureau (2020).
Method
Historical Hazard Intensity
Swelling potential by Louisiana census block (
Future Hazard Intensity
The soil structure remains largely unchanged on anthropogenic time scales. However, long-term changes in the freeze-thaw, extreme heat, and/or precipitation climatology could impact the stability of the soil structure for supporting construction. The anticipated decrease in number of freezing-temperature days as temperature increases (Vose et al., 2017; their Figure 6.9), at least under the highest-CO2-emission scenario, would diminish the future expansive soil hazard due to a decrease in freeze-thaw expansion/contraction. However, the likelihood of an increasing number of extreme hot days (Vose et al., 2017; their Figure 6.9) and heavier precipitation by 2050 interrupted by lengthening dry periods (Wehner et al., 2017), albeit again under the highest-CO2-emission scenario, may overcompensate, causing a net increase expansion/contraction. The net effect of these forces leads to a projection in this study of an increase in the expansive soil hazard of 15 percent (i.e.,
Population Projection
The technique for population (
The
Mostafiz et al. (2020b) tested other methods for projecting population but found the technique described above to be superior. Extrapolation of a regression-based trend line of Louisiana parish populations to 2050 proved disadvantageous because of low explained variance and insignificant trend lines for some parishes. Extrapolating the growth rate trend line to estimate the 2050 population was problematic for the same reason. The abrupt, sizeable, and temporary population redistributions both within and beyond Louisiana resulting from significant hurricanes (most notably Katrina in 2005) are likely contributors to the low explained variance. The technique selected is least sensitive to these issues and was also used successfully in Mostafiz et al. (2021a; 2021b).
Assessing Building Value
Following Mostafiz et al. (2021a; 2021b), evaluation of current and future building value in each census block is done under the assumption that unpopulated areas have no residential or commercial property value, for the purpose of this analysis. Of course, in reality they do have property value, but the loss of an unoccupied barn, camp, or other dwelling to the hazard would be unlikely to impact in the same way that a loss of a primary residence would. The number of buildings by census block in 2010
The number of buildings in 2050 by census block (
Projecting Future Property Loss
Property loss due to expansive soil
To quantify the uncertainty involved in this calculation, a sensitivity test using the bounds of 5 and 10 percent for
Similarly, the historical annual property loss (
Results
Historical Hazard Intensity
The southeastern and southwestern parts of the state have the highest swelling potential for expansive soil (Figure 1A). Historical expansive soil swelling potential ranges from 3.5 in northwestern and central Louisiana census blocks to 58.0 percent in both Cameron Parish in the extreme coastal southwest and in some census blocks to the west of New Orleans in Lafourche, St. Charles, and St. John the Baptist parishes (Figure 1A; Supplementary Appendix SA). Because planning is done at the parish level, it is also worthwhile to note that St. Charles Parish is the most vulnerable parish on the whole, where the mean historical expansive soil swelling potential is 42.9 percent (Supplementary Appendix SA).
Future Hazard Intensity
Because of the assumption of uniformity in the future environmental effects on soil features across the state, the expansive soil hazard is projected to remain concentrated in the same geographical areas of the state as in the historical record, but with swelling potential projected to increase by 15 percent by 2050. Such an assumption is necessary due to the scale of model output by the National Climate Assessment. Projected soil swelling potential is anticipated to range from 4.0 in northwestern and central Louisiana to 66.7 percent in Cameron, Lafourche, St. Charles, and St. John the Baptist parishes (Figure 1B; Supplementary Appendix SB) by 2050. St. Charles will remain the most vulnerable parish, where the mean projected expansive soil swelling potential is 49.4 percent (Supplementary Appendix SB). Nine of the ten most vulnerable parishes will remain in the southeastern part of the state (i.e., St. Charles, Orleans, St. John the Baptist, Assumption, St. James, Jefferson, Plaquemines, Lafourche, and St. Mary), with only Cameron (ranking seventh) in the extreme southwest (Supplementary Appendix SB). The east-central parish of East Feliciana is and is projected to be the least vulnerable parish, followed by the six northern Louisiana parishes of Bossier, Caddo, Claiborne, De Soto, Sabine, and Webster (Supplementary Appendix SB).
Historical and Projected Population
The population is most densely concentrated around New Orleans, Baton Rouge, and Shreveport (Figure 2A), the state’s three largest cities and metropolitan areas. By 2050, increasing density will be in and near Lafayette and Baton Rouge, and in east-central Louisiana (Figure 2B). The greatest population losses, expressed in terms of population density, are projected to be in rural areas of northeastern Louisiana and the inhabited areas along the Red River from north of Shreveport to southeast of Alexandria, in the New Orleans area, and elsewhere (Figure 2B). Population, population density, and their 2050-projected values by parish are shown in Supplementary Appendix SC.
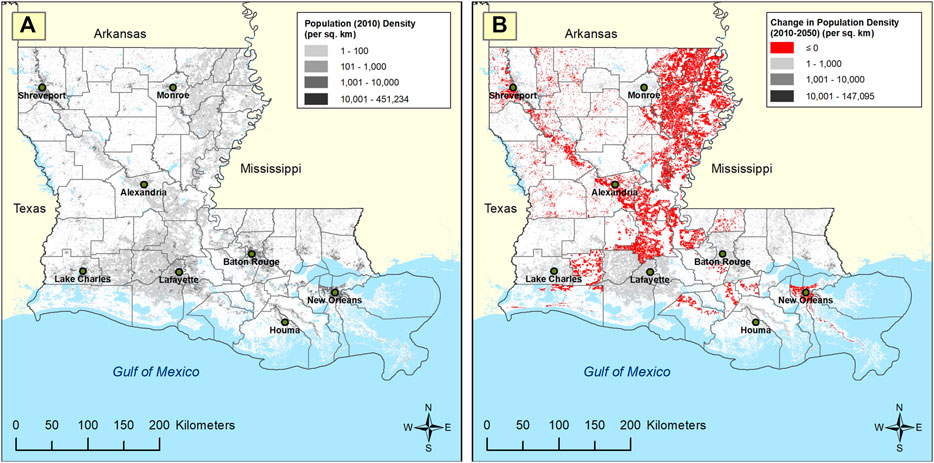
FIGURE 2. Population density by Louisiana census block: (A) 2010, and (B) projected change from 2010 to 2050.
Historical and Projected Property Loss
The historical average annual statewide property loss due to expansive soil is $66,231,136 (2010$), and the loss will increase by 2050 as the product of the determinants of loss–hazard intensity (in this case, expansive soil swelling potential) and population–increase in most parts of the state. Statewide property loss is projected to be $91,753,149 (2010$) by 2050 (Supplementary Appendix SD), a growth of 39 percent. The maximum estimated property losses will remain concentrated near their present locations, namely, southern urban centers (i.e., Baton Rouge, Houma, Lafayette, Lake Charles, and New Orleans), Shreveport, and the east-central parishes (Figures 3A,B).
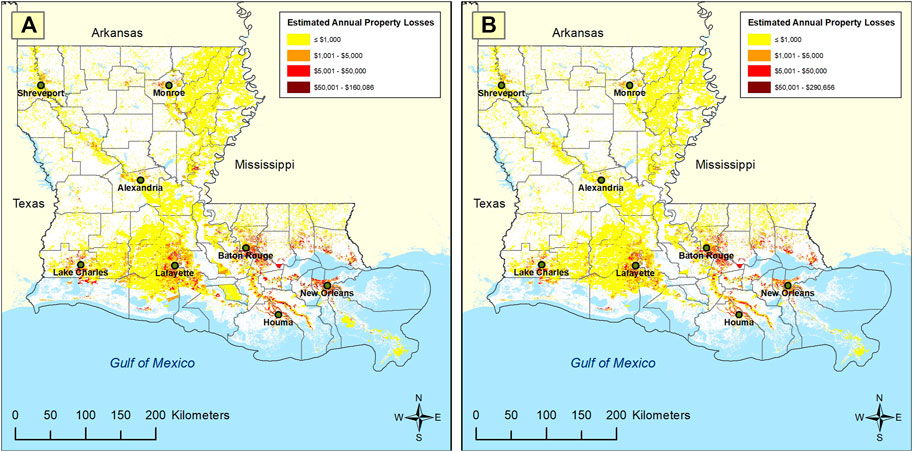
FIGURE 3. Estimated annual property loss (2010$) due to expansive soil by Louisiana census block: (A) historical, and (B) projection for 2050.
The historical average annual per capita property loss due to expansive soil is $14.61 (2010$) in Louisiana but will grow to $16.21 by 2050 (2010$), an increase of 11 percent (Supplementary Appendix SD). The same general spatial distribution of per capita property losses (Figures 4A,B) occurs and is projected to occur by 2050 as was shown for absolute losses, but with slight increases near Lake Charles and slight decreases in the Lafayette, Baton Rouge, and Monroe areas.
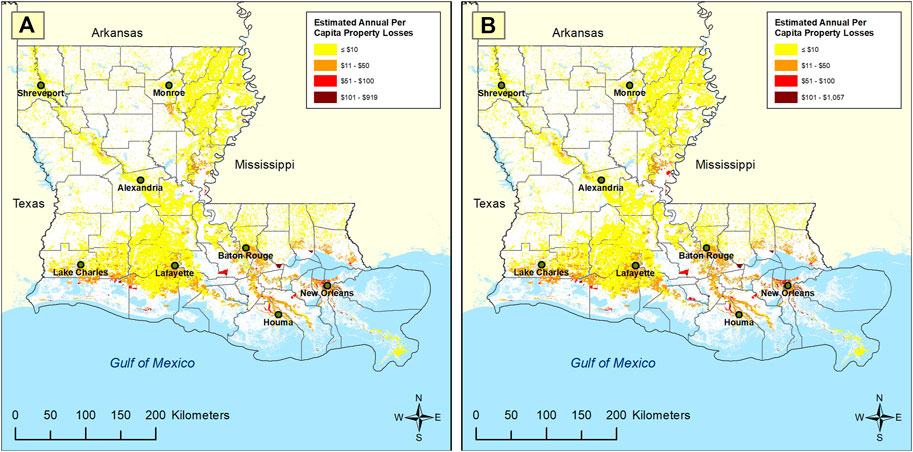
FIGURE 4. Estimated annual per capita property loss (2010$) due to expansive soil by Louisiana census block: (A) historical, and (B) projection for 2050.
The historical average annual per building property loss is $33.71 (2010$) with an increase to $38.10 (2010$) expected by 2050 (Supplementary Appendix SD), for an increase of 13 percent statewide. The Alexandria, Baton Rouge, Lafayette, Lake Charles, and Monroe areas all show a greater propensity for current and future per building annual losses than they do for annual current and future property loss and per capita property loss (compare Figures 5A,B to Figures 3A,B, and 4A,B).
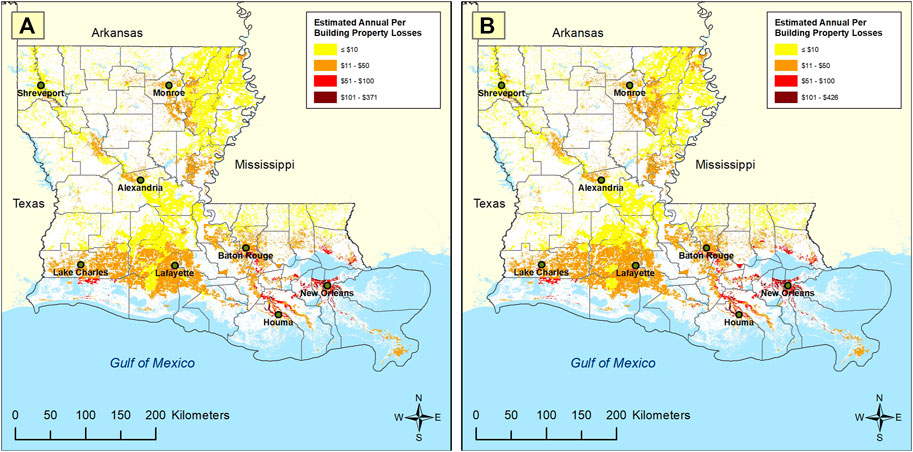
FIGURE 5. Estimated annual per building property loss (2010$) by Louisiana census block: (A) historical, and (B) projection for 2050.
At the parish level, Orleans has the highest historical overall expansive soil annual property loss ($16,908,448), per capita property loss ($49.18), and per building property loss ($89.04) among the parishes (Supplementary Appendix SD). Although changes in the expansive soil swelling potential and population are projected to change the expansive soil risk by 2050, the greatest annual expansive soil property loss ($17,479,776), per capita property loss ($56.36), and per building property loss ($102.59) are expected to remain in Orleans Parish through 2050 (Supplementary Appendix SD).
At the census-block level, the largest historical average annual property loss due to expansive soil is in block 220510205171002 of Jefferson Parish ($160,086). The maximum historical annual per capita property loss in the state is $918 in census block 220710094004011, in Orleans Parish. The highest historical average annual per building property loss ($370) is in census block 220510226001000, in Jefferson Parish. By 2050, the greatest annual property loss due to expansive soil is projected to be in census block 221030408035041, in St. Tammany Parish ($290,655). The maximum annual per capita property loss ($1,056) will be in census block 220710094004011 of Orleans Parish. The highest annual per building property loss ($425) is projected to be in census block 220510226001011, in Jefferson Parish.
Sensitivity Analysis
The sensitivity analysis demonstrates the impact of different model assumptions regarding expansive soil swelling potential by 2050 (

TABLE 1. Sensitivity analysis of 2050 projections of Louisiana statewide annual property loss (i.e., risk) due to expansive soil, by parameter (2010$).
Discussion
When approximating the economic impact of expansive soils nationwide, FEMA (1982) projection for 2000 of $997.1 million nationwide would equate to $5.60 billion in 2010$ (CPI Inflation Calculator 2021). The $66,231,136 (2010$) loss calculated here for Louisiana represents approximately 1.18 percent of this national total. Given the extent of the hazard and the property value in the state, this percentage compares favorably with Louisiana’s 2010 share of the U.S. population (1.46 percent) and GDP (1.65 percent). Even closer estimates may be provided by using the 10 percent value for
The Louisiana government sector would be wise to invest in mitigation mechanisms, at least for government-owned buildings. Possibilities include requiring structures to be pile supported, or incorporating swell potential foundation design elements into foundations (e.g., void space under slabs, vapor barriers between foundation and soil, weather conditioning the soils under building prior to construction, and perimeter drainage systems around structures to drain moisture away from foundations). Detailed geotechnical exploration can be performed to address swelling in the subsoils, to minimize the projected increases in property loss for much of Louisiana by 2050. Property owners should be aware of and plan for expected increases in maintenance costs during the useful life span of their homes and businesses. The good news is that mitigation can be accomplished relatively easily in most cases. Furthermore, the sensitivity analysis shows clearly that maintenance cost is a more sensitive variable for predicting future loss due to expansive soils than the change in expansive soil hazard intensity. Thus, mitigation strategies to reduce the maintenance costs, which is more in the control of the homeowner than changes in hazard intensity, may be effective in avoiding major losses.
Limitations
The assumption that the expansive soil hazard intensity will change equally across the state, necessitated by a lack of confidence in higher-resolution climate data output for 2050, calls for caution to be exercised in the interpretation of results. Also, as in Mostafiz et al. (2021a; 2021b), limitations of this research involve the population projection methodology. Sudden, unpredictable shifts in future population, such as those caused by disasters, economic conditions, or other extreme events would alter the results. Likewise, the assumption that census blocks within a parish have the same population growth rate and that the population growth follows an exponential curve may further limit the interpretation of results. The cost associated with maintenance and repair of property does not differentiate other potential source of damage from other factors such as subsidence, settlement, and poor foundations. In addition, the projected damage by 2050 does not account for future potential technologies and design mitigation measures that may reduce the future shrink/swell damage, especially with differential application measures across space. Finally, the absence of real-world data against which to calibrate the model and assess its utility is a limitation at this time.
Summary and Conclusion
The hazard and risk due to expansive soils is often overlooked when tabulating natural hazard risk, vulnerability, and resilience. This study introduces a method for assessing the property risk due to expansive soils at the census-block and parish (county) level in Louisiana, a U.S. state with substantial impacts of this hazard. Risk is assigned as the product of exposure to the hazard and the potential loss, the latter of which is a function of the population and building value. Results suggest that the annual historical property loss, per capita property loss, and per building property loss are all greatest in southeastern Louisiana and extreme southwestern Louisiana, but the concentration of wealth in cities increases the property loss in most of the urban areas. Projections of loss by 2050 show a similar pattern, but with increased per building loss in and around a swath of cities across southwestern and south-central Louisiana. Despite some limitations, these results are based on the most thorough analysis to date on the economic risk due to expansive soils, and the method may be applied elsewhere.
Future research should be undertaken to “fine tune” the future estimates of loss, which are currently limited by the lack of sophisticated geophysical model output, demographic model-based projections in Louisiana, robust estimates of “real world” losses for validation, and knowledge of any existing mitigation techniques that have been implemented. Regardless, care must be taken to ensure that home renovations are not mischaracterized as remediation from the hazard. Application in other states or regions with more abundant, high-quality demographic projections might yield enhanced results. Collection of data via surveys/interviews of homeowners in different markets, along with data from foundation contractors and perhaps insurance companies, including upfront costs and retrofit mitigation costs, is a substantial future research effort. Regardless, imminent improvements in climate model output, at finer resolutions, will improve our ability to anticipate and mitigate the risk of expansive soils.
Data Availability Statement
The original contributions presented in the study are included in the article/Supplementary Material, further inquiries can be directed to the corresponding author.
Author Contributions
RM developed the detailed methodology, collected and analyzed the data, and developed the initial text. CF conceptualized the hazard quantification and revised the text. RR developed the atmospheric projections and edited early and late drafts of the text. NB provided oversight on analysis, particularly regarding the population projections, and revised the text. CH reviewed and provided insight and recommendation for project evaluation from a geotechnical engineering perspective.
Funding
This project resulted from the 2019 Louisiana State Hazard Mitigation Plan update, for which CF and RR received funding from FEMA, via GOHSEP, grant number: 2000301135. Any opinions, findings, conclusions, and recommendations expressed in this manuscript are those of the authors and do not necessarily reflect the views of FEMA or GOHSEP. Publication of this article was subsidized by the Louisiana State University (LSU) Libraries Open Access Author Fund and the Performance Contractors Professorship in the College of Engineering at LSU.
Conflict of Interest
The authors declare that the research was conducted in the absence of any commercial or financial relationships that could be construed as a potential conflict of interest.
Publisher’s Note
All claims expressed in this article are solely those of the authors and do not necessarily represent those of their affiliated organizations, or those of the publisher, the editors and the reviewers. Any product that may be evaluated in this article, or claim that may be made by its manufacturer, is not guaranteed or endorsed by the publisher.
Acknowledgments
The authors warmly appreciate the overall project support from Jeffrey Giering of Louisiana’s Governor’s Office of Homeland Security and Emergency Preparedness (GOHSEP).
Supplementary Material
The Supplementary Material for this article can be found online at: https://www.frontiersin.org/articles/10.3389/fbuil.2021.754761/full#supplementary-material
References
Abbey, S. J., Eyo, E. U., and Ng’ambi, S. (2020). Swell and Microstructural Characteristics of High-Plasticity clay Blended with Cement. Bull. Eng. Geol. Environ. 79 (4), 2119–2130. doi:10.1007/s10064-019-01621-z
Acharya, R., Pedarla, A., Bheemasetti, T. V., and Puppala, A. J. (2017). Assessment of Guar Gum Biopolymer Treatment toward Mitigation of Desiccation Cracking on Slopes Built with Expansive Soils. Transportation Res. Rec. 2657 (1), 78–88. doi:10.3141/2657-09
Al-Atroush, M. E., and Sebaey, T. A. (2021). Stabilization of Expansive Soil Using Hydrophobic Polyurethane Foam: A Review. Transportation Geotechnics 27, 100494. doi:10.1016/j.trgeo.2020.100494
Al-Rawas, A. A., and Goosen, M. F. (2006). Expansive Soils: Recent Advances in Characterization and Treatment. London, UK: Taylor & Francis.
Bharat, T. V., and Gapak, Y. (2018). Hydration Kinetics of Bentonite Buffer Material: Influence of Vapor Pressure, Bentonite Plasticity, and Compaction Density. Appl. Clay Sci. 157, 41–50. doi:10.1016/j.clay.2018.02.029
Bhuvaneshwari, S., Robinson, R. G., and Gandhi, S. R. (2020). Effect of Functional Group of the Inorganic Additives on index and Microstructural Properties of Expansive Soil. Int. J. Geosynth. Ground Eng. 6 (4), 1–15. doi:10.1007/s40891-020-00235-w
Cahoon, D. R., Perez, B. C., Segura, B. D., and Lynch, J. C. (2011). Elevation Trends and Shrink-Swell Response of Wetland Soils to Flooding and Drying. Estuarine, Coastal Shelf Sci. 91 (4), 463–474. doi:10.1016/j.ecss.2010.03.022
Carter, L. M., Jones, J. W., Berry, L., Burkett, V., Murley, J. F., Obeysekera, J., et al. (2014). “Southeast and the Caribbean,” in Climate Change Impacts in the United States: The Third National Climate Assessment. Editors J. M. Melillo, T. C. Richmond, and G. W. Yohe, Washington, DC: U.S. Global Change Research Program, 396–417. doi:10.7930/J0N-P22CB
Çimen, Ö., Keskin, S. N., and Yıldırım, H. (2012). Prediction of Swelling Potential and Pressure in Compacted clay. Arab J. Sci. Eng. 37 (6), 1535–1546. doi:10.1007/s13369-012-0268-4
Coleman, D. M., and Douglas, S. C. (2008). “Swell Potential of Near Surface Soils in Mississippi and Louisiana,” in Proceedings of the Geosustainability and Geohazard Mitigation: Proceedings of Sessions of Geocongress 2008, New Orleans, Louisiana, March 9‒12, 2008, 279–286. doi:10.1061/40971(310)35
Cpi Inflation Calculator, (2021). CPI Inflation Calculator. Available at: https://www.in2013dollars.com (Last accessed 9 14, 2021).
United States Global Change Research Program (2017). “Fourth National Climate Assessment,” in Climate Science Special Report: Fourth National Climate Assessment, Volume I. Editors D. J. Wuebbles, D. W. Fahey, K. A. Hibbard, D. J. Dokken, B. C. Stewart, and T. K. Maycock Washington, D.C., United States: U.S. Global Change Research Program, 470. doi:10.7930/J0J964J6
Erzin, Y. (2007). Artificial Neural Networks Approach for Swell Pressure versus Soil Suction Behaviour. Can. Geotech. J. 44 (10), 1215–1223. doi:10.1139/T07-052
Eyo, E. U., Ng'ambi, S., and Abbey, S. J. (2019). Effect of Intrinsic Microscopic Properties and Suction on Swell Characteristics of Compacted Expansive Clays. Transportation Geotechnics 18, 124–131. doi:10.1016/j.trgeo.2018.11.007
Fattah, M. Y., Salim, N. M., and Irshayyid, E. J. (2021). Swelling Behavior of Unsaturated Expansive Soil. Transp. Infrastruct. Geotech. 8 (1), 37–58. doi:10.1007/s40515-020-00112-z
FEMA (1982). Special Statistical Summary – Deaths, Injuries, and Property Loss by Type of Disaster 1970-1980. Available at: https://apps.dtic.mil/dtic/tr/fulltext/u2/a127645.pdf (Last accessed 9 14, 2021).
Ferber, V., Auriol, J.-C., Cui, Y.-J., and Magnan, J.-P. (2009). On the Swelling Potential of Compacted High Plasticity Clays. Eng. Geology 104 (3‒4), 200–210. doi:10.1016/j.enggeo.2008.10.008
Frikha, W., Bouassida, M., and Canou, J. (2013). Observed Behaviour of Laterally Expanded Stone Column in Soft Soil. Geotech. Geol. Eng. 31 (2), 739–752. doi:10.1007/s10706-013-9624-8
Heinrich, P. V. (2008). Loess Map of Louisiana. Public Information Series No. 12, Baton Rouge, Louisiana, United States: Louisiana Geological Survey. Available at: https://www.lsu.edu/lgs/publications/products/Free_publications/Loess-Map-LA.pdf (Last accessed 9 14, 2021).
Hobbs, P. R. N., Jones, L. D., Kirkham, M. P., Gunn, D. A., and Entwisle, D. C. (2019). Shrinkage Limit Test Results and Interpretation for clay Soils. Q. J. Eng. Geology Hydrogeology 52 (2), 220–229. doi:10.1144/qjegh2018-100
Hobbs, P. R. N., Jones, L. D., Kirkham, M. P., Roberts, P., Haslam, E. P., and Gunn, D. A. (2014). A New Apparatus for Determining the Shrinkage Limit of clay Soils. Géotechnique 64 (3), 195–203. doi:10.1680/geot.13.P.076
Holtz, W. G., and Gibbs, H. J. (1954). Engineering Properties of Expansive Clays. Proc. Am. Soc. Civil Eng. 80, 516-1–516-28.
Holtz, W. G., and Hart, S. S. (1978). Home Construction on Shrinking and Swelling Soils. Colo. Geol. Surv. 11.
Ijaz, N., Dai, F., and Rehman, Z. u. (2020). Paper and wood Industry Waste as a Sustainable Solution for Environmental Vulnerabilities of Expansive Soil: A Novel Approach. J. Environ. Manage. 262, 110285. doi:10.1016/j.jenvman.2020.110285
Jones, L. D. (2012). “Expansive Soils,” in ICE Manual of Geotechnical Engineering. Volume 1, Geotechnical Engineering Principles, Problematic Soils and Site Investigation. Editor J. Buland (London, UK: ICE Publishing), 413–441. Available at: http://nora.nerc.ac.uk/id/eprint/17002/ (Last accessed 9 14, 2021).
Jung, S., and Santagata, M. (2014). Expansive Behavior of Coal Mine Spoils Treated with Calcium-Based Stabilizers. Eng. Geology 175, 46–57. doi:10.1016/j.enggeo.2014.03.013
Kasangaki, G. J., and Towhata, I. (2009). Wet Compaction and Lime Stabilization to Mitigate Volume Change Potential of Swelling Clayey Soils. Soils and Foundations 49 (5), 813–821. doi:10.1016/j.trgeo.2020.10038710.3208/sandf.49.813
Khan, M. A., Wang, J. X., and Patterson, W. B. (2017). A Study of the Swell-Shrink Behavior of Expansive Moreland clay. Int. J. Geotechnical Eng. 13 (3), 205–217. doi:10.1080/19386362.2017.1351744
Kovda, I., Morgun, E., and Boutton, T. W. (2010). Vertic Processes and Specificity of Organic Matter Properties and Distribution in Vertisols. Eurasian Soil Sc. 43 (13), 1467–1476. doi:10.1134/S1064229310130065
Krinitzsky, E. L., and Turnbull, W. J. (1967). Loess Deposits of Mississippi. Special Paper 94. Boulder, Colorado, United States: Geological Society of America.
Lim, B. F., and Siemens, G. A. (2016). Unifying Framework for Modeling Swelling Soil Behaviour. Can. Geotech. J. 53 (9), 1495–1509. doi:10.1139/cgj-2015-0049
Mostafiz, R. B., Friedland, C. J., Rohli, R. V., and Bushra, N. (2021a). Property Risk Assessment of Sinkhole Hazard in Louisiana, U.S.A. Front. Environ. Sci. 9, 780870. doi:10.3389/fenvs.2021.780870
Mostafiz, R. B., Friedland, C. J., Rohli, R. V., and Bushra, N. (2021b). “Censusblock-Level Property Risk Assessment for Wildfire in Louisiana, U.S.A.,” in Review at Frontiers in Forests and Global Change Since 7/13/2021. Lausanne, Switzerland: Frontiers Media. Available at: https://www.essoar.org/doi/abs/10.1002/essoar.10508248.2 (Accessed October 22, 2021).
Mostafiz, R. B., Friedland, C. J., Rohli, R. V., Gall, M., Bushra, N., and Gilliland, J. M. (2020a). Census-block-level Property Risk Estimation Due to Extreme Cold Temperature, Hail, Lightning, and Tornadoes in Louisiana, United States. Front. Earth Sci. 8, 601624. doi:10.3389/feart.2020.601624
Mostafiz, R. B., Friedland, C. J., Rohli, R. V., and Bushra, N. (2020b). “Assessing Property Loss in Louisiana, U.S.A., to Natural Hazards Incorporating Future Projected Conditions,” in American Geophysical Union Conference. Available at: https://ui.adsabs.harvard.edu/abs/2020AGUFMNH0150002M/abstract (Accessed August 27, 2021).
Nalbantoğlu, Z. (2004). Effectiveness of Class C Fly Ash as an Expansive Soil Stabilizer. Construction Building Mater. 18 (6), 377–381. doi:10.1016/j.conbuildmat.2004.03.011
Nelson, J. D., and Miller, D. J. (1992). Expansive Soils—Problems and Practice in Foundation and Pavement Engineering. New York, USA: Wiley Department of Civil Engineering, Colorado State University.
Olive, W. W., Chleborad, A. F., Frahme, C. W., Schlocker, J., Schneider, R. R., and Schuster, R. L. (1989). “Swelling Clays Map of the Conterminous United States,” in USGS Miscellaneous Investigations Series Map I-1940 (Reston, Virginia: USGS). Available at: https://ngmdb.usgs.gov/Prodesc/proddesc_10014.htm (Last accessed 9 14, 2021). doi:10.3133/i1940
Patil, U., Valdes, J. R., and Evans, T. M. (2011). Swell Mitigation with Granulated Tire Rubber. J. Mater. Civil Eng. 23 (5), 721–727. doi:10.1061/(ASCE)MT.1943-5533.0000229
Puppala, A., Hoyos, L., Viyanant, C., and Musenda, C. (2001). Fiber and Fly Ash Stabilization Methods to Treat Soft Expansive Soils. Soft Ground Technology Conf., 136–145. doi:10.1061/40552(301)11
Rao, A. S., Phanikumar, B. R., and Sharma, R. S. (2004). Prediction of Swelling Characteristics of Remoulded and Compacted Expansive Soils Using Free Swell index. Q. J. Eng. Geology Hydrogeology 37 (3), 217–226. doi:10.1144/1470-9236/03-052
Reddy, N. G., Tahasildar, J., and Rao, B. H. (2015). Evaluating the Influence of Additives on Swelling Characteristics of Expansive Soils. Int. J. Geosynth. Ground Eng. 1 (1). 7, doi:10.1007/s40891-015-0010-x
Schubert, S. D., Chang, Y., DeAngelis, A. M., Wang, H., and Koster, R. D. (2021). On the Development and Demise of the Fall 2019 Southeast U.S. Flash Drought: Links to an Extreme Positive IOD. J. Clim. 34 (5), 1701–1723. doi:10.1175/JCLI-D-20-0428.1
Seed, H. B., Woodward, R. J., and Lundgren, R. (1962). Prediction of Swelling Potential for Compacted Clays. J. Soil Mech. Foundations Division 88 (3), 53–87. doi:10.1061/jsfeaq.0000431
Sfoog, E. H., Siang, A. J. L. M., Siang, A. J., Naji, N., Yi, S. S., and Guntor, N. A. (2020). Foundation Failures Mitigation under Expansive clay by Using Granular Pile Anchor System. Ijie 12 (9), 36–44. doi:10.30880/ijie.2020.12.09.005
Snowden, J. O., and Priddy, R. R. (1968). Loess Investigations in Mississippi. Bulletin 111 (Jackson, Mississippi: Mississippi Geological, Economic and Topographical Survey), 40–46. Available at: https://www.mdeq.ms.gov/geology/work-areas/publications-and-map-sales/categories/bulletins/loess-investigations-in-mississippi-19386/ (Accessed 9 14, 2021).
Taher, Z. J., Scalia IV, J., and Bareither, C. A. (2020). Comparative Assessment of Expansive Soil Stabilization by Commercially Available Polymers. Transportation Geotechnics 24, 100387. doi:10.1016/j.trgeo.2020.100387
Toksöz Hozatlıoğlu, D., and Yılmaz, I. (2021). Shallow Mixing and Column Performances of Lime, Fly Ash and gypsum on the Stabilization of Swelling Soils. Eng. Geology 280, 105931. doi:10.1016/j.enggeo.2020.105931
Tripathy, S., Sridharan, A., and Schanz, T. (2004). Swelling Pressures of Compacted Bentonites from Diffuse Double Layer Theory. Can. Geotech. J. 41 (3), 437–450. doi:10.1139/T03-096
Tu, H., and Vanapalli, S. K. (2016). Prediction of the Variation of Swelling Pressure and One-Dimensional Heave of Expansive Soils with Respect to Suction Using the Soil-Water Retention Curve as a Tool. Can. Geotech. J. 53 (8), 1213–1234. doi:10.1139/cgj-2015-0222
United States Census Bureau (2010). TIGER/Line Shapefiles. Available at: https://www.census.gov/geographies/mapping-files/time-series/geo/tiger-line-file.2010.html (Last accessed 9 14, 2021).
United States Census Bureau (2020). Programs-surveys. Popest. Datasets. Available at: https://www2.census.gov/programs-surveys/popest/datasets/(Last accessed 9 14, 2021).
Virginia Department of Mines, Minerals and Energy (2021). Division of Geology and Mineral Resources. Expansive Soils and Frost Heaves. Available at: https://www.dmme.virginia.gov/dgmr/expansivesoils.shtml# (Last accessed 9 14, 2021).
Vose, R. S., Easterling, D. R., Kunkel, K. E., LeGrande, A. N., and Wehner, M. F. (2017). “Ch. 6: Temperature Changes in the United States. Climate Science Special Report: Fourth National Climate Assessment, Volume I,” in Climate Science Special Report: Fourth National Climate Assessment, Volume I. Editors D. J. Wuebbles, D. W. Fahey, K. A. Hibbard, D. J. Dokken, B. C. Stewart, and T. K. Maycock (Washington D.C.: U.S. Global Change Research Program), 185–206. doi:10.7930/J0N29V45
Wang, J. X. “Expansive Soils and Practice in Foundation Engineering,” in A presentation delivered at the 2016 Louisiana Transportation Conference, Baton Rouge, Louisiana, 03 March 2016. Available at: http://www.ltrc.lsu.edu/ltc_16/pdf/presentations/10-University%20Transportation%20Centers%20(Part%201)-Characterization%20of%20Expansive%20Soils%20in%20Northern%20Louisiana.pdf (Last accessed 9 14, 2021).
Wang, J. X., Khan, M. A., and Ikra, B. A. (2017). Impact of Severe Drought on the Compacted Expansive Clays (Subgrade) in Northern Louisiana. Transportation Research Board Weekly. Washington, D.C., United States: National Academies of Science, Engineering, and Medicine. Available at: https://trid.trb.org/view/1500364 (Last accessed 9 14, 2021).
Watanabe, Y., and Yokoyama, S. (2021). Self-sealing Behavior of Compacted Bentonite-Sand Mixtures Containing Technological Voids. Geomechanics Energ. Environ. 25, 100213. doi:10.1016/j.gete.2020.100213
Wehner, M. F., Arnold, J. R., Knutson, T., Kunkel, K. E., and LeGrande, A. N. (2017). “Ch. 8: Droughts, Floods, and Wildfires. Climate Science Special Report: Fourth National Climate Assessment, Volume I,” in Climate Science Special Report: Fourth National Climate Assessment, Volume I. Editors D. J. Wuebbles, D. W. Fahey, K. A. Hibbard, D. J. Dokken, B. C. Stewart, and T. K. Maycock (Washington, DC, USA: U.S. Global Change Research Program), 231–256. doi:10.7930/J0CJ8BNN
Yang, R., Xiao, P., and Qi, S. (2019). Analysis of Slope Stability in Unsaturated Expansive Soil: A Case Study. Front. Earth Sci. 7, 292. doi:10.3389/feart.2019.00292
Yilmaz, I., and Civelekoglu, B. (2009). Gypsum: An Additive for Stabilization of Swelling clay Soils. Appl. Clay Sci. 44 (1‒2), 166–172. doi:10.1016/j.clay.2009.01.020
Keywords: shrinking soil potentiality, swelling soil potentiality, soil subsidence, soil stability, environmental hazards, natural hazards, census block, Gulf of Mexico coast
Citation: Mostafiz R, Friedland C, Rohli R, Bushra N and Held C (2021) Property Risk Assessment for Expansive Soils in Louisiana. Front. Built Environ. 7:754761. doi: 10.3389/fbuil.2021.754761
Received: 07 August 2021; Accepted: 30 September 2021;
Published: 29 October 2021.
Edited by:
Mounir Bouassida, Tunis El Manar University, TunisiaCopyright © 2021 Mostafiz, Friedland, Rohli, Bushra and Held. This is an open-access article distributed under the terms of the Creative Commons Attribution License (CC BY). The use, distribution or reproduction in other forums is permitted, provided the original author(s) and the copyright owner(s) are credited and that the original publication in this journal is cited, in accordance with accepted academic practice. No use, distribution or reproduction is permitted which does not comply with these terms.
*Correspondence: Rubayet Bin Mostafiz, cmJpbm1vMUBsc3UuZWR1