- Department of Biomedical Engineering, Tufts University, Medford, MA, United States
Bioactive glasses and Calcium Phosphate bioceramics have emerged as promising scaffold biomaterials for bone tissue engineering. These materials possess inherent osteoinductive properties that work to create a more suitable environment for bone tissue formation. Additionally, these scaffolds exhibit dissolution properties when submerged in physiological fluids in vivo and therefore can release different ions. Incorporating therapeutic ion-modifiers that have independently demonstrated their osteogenic favorability to these scaffolds can further increase environmental suitability. This review discusses the favorable properties of bioactive glasses and Calcium Phosphate bioceramics in the context of Bone Tissue Engineering as well as potential incorporable metal ion-modifiers.
1 Introduction
Bone tissue engineering (BTE) is an emerging subsect of regenerative medicine that offers an alternative treatment to bone fractures and segmental defects—both of which pose as a notable source of patient morbidity. Current treatments comprise mostly of allografts or synthetic grafts, and are estimated at a staggering annual cost of $5 billion in the US alone (Perez et al., 2018). To circumvent the common limitations of graft rejection or infection, BTE has been continually explored as a novel method in tissue repair.
Current approaches to BTE typically use mesenchymal stem cells [MSCs] and specific bioactive molecules to develop and promote the formation of new bone tissue, a process otherwise known as osteogenesis. Tissues produced by BTE must be able to fully integrate with host bone while also performing native functions like locomotion, electrolyte maintenance, load-bearing etc (Amini et al., 2012). In recent years, different inorganic ions have been researched to have therapeutic effects and hold significance in bone tissue formation (Schatkoski et al., 2021). While still not fully understood, certain osteogenic properties have been validated and their incorporation with an ECM-like scaffold help promote bone repair and regeneration (Motamedian et al., 2015). Although exogenous scaffolds primarily provide mechanical support and nutrient perfusion, to be in a stable in vivo environment they must also mimic normal ECM cues that accurately modulate cell behavior (Echeverria Molina et al., 2021). Various methodologies have already been established to yield functional scaffolds; further osteogenic optimization must also focus on specific biomaterials targeting these properties.
“Smart” scaffolds in BTE are comprised to act as environmentally sensitive vehicles for different osteogenic biomolecules. They can exert stimulating effects on tissues to increase stem cell attachment, differentiation, and continued proliferation (Motamedian et al., 2015). Among researched biomaterials, Calcium phosphate (CaP) bioceramics have emerged as suitable for BTE due to their osteoinductive abilities and structural similarity to native bone (Wei et al., 2022). The abundance of CaP crystals in native bone indicates their favorable properties and has thus become a central focus of bioceramic development. Similarly, bioactive glasses [BGs] have also emerged as an extremely promising biomaterial and are able to rapidly form a continuous bond with host bone. This bond allows seeded MSCs and other proteins to begin integrating with the native bone environment.
Additionally, the smooth incorporation both biomaterials have with inorganic metal ions as a bioactive molecule favors their use further. These ions and their displayed properties are critical in establishing a stable environment. This study reviews the attributes of CaP bioceramics and bioactive glasses as a scaffold material for BTE and discusses different osteogenic properties of therapeutic ions to improve bioactivity.
2 Biomaterials of BTE smart scaffolds
2.1 Calcium phosphate bioceramics
“Bioceramics” is an umbrella term to encompass a vast range of biocompatible inorganic non-metallic materials (Brunello et al., 2020). They vary from inert ceramic oxides to resorbable materials that are replaced by tissue following a period of time. Bioceramics are under consideration as a scaffold material mainly due to their mechanical and load-bearing properties, which are important attributes for hard tissue engineering (bone, teeth, etc.). However, in recent years large strides have been made in the development of bioceramics possessing intrinsic osteoinductive properties (Ginebra et al., 2018).
In bone ECM, calcium phosphate crystals act as a mineral phase to reinforce a collagen fiber network (Ginebra et al., 2018). Many different CaPs have demonstrated their presence can trigger differentiation of osteoprogenitor cells and lead to bone formation (Ginebra et al., 2018). Moreover, CaP bioceramics partially dissolve in vivo, resulting in higher local calcium and phosphate ion concentrations. This property contributes to a better osteogenic capacity and has been established in studies since the 1990s (Daculsi et al., 1989; Klein et al., 1994; Eliaz and Metoki, 2017). This dissolution can also release other ion-modifiers present within the biomaterial.
The hierarchical porous structure of bone, ranging in size from 20 to 400 μm (Vallet-Regí et al., 2011), is necessary for proper cell adherence and proliferation; CaP bioceramics have traditionally been macroporous (∼100 μm) to accommodate osteocytes and allow for bone tissue ingrowth. Recent studies conducted on scaffold porosity have demonstrated that an increase of pore volume and specific surface area may significantly accelerate bone formation by enhancing protein and cell adhesion (Eliaz and Metoki, 2017). Studies conducted on high porosity CaP particles have suggested that their pore distribution does enhance protein adsorption qualities (Li et al., 2008; Zhu et al., 2010).
Similar to increased porosity, cell adhesion is also influenced by the inherent surface properties of CaP bioceramics. Attributes like surface roughness, surface charge, and percent of crystallinity all play a role in cell attachment and can be modified with other derivatives (Samavedi et al., 2013; Poli et al., 2019). Surface roughness mainly influences attachment by the grain size of CaP crystallites and particle size (Samavedi et al., 2013). This roughness differs depending on bioceramic composition, but most provide a favorable environment for adhesion and continued proliferation.
Although numerous studies have been conducted to verify the advantageous nature of CaP bioceramics, its main problem limiting clinical applications originate from its poor mechanical properties. CaP crystals are commonly characterized as brittle and possess relatively low tensile strength (Ambard and Mueninghoff, 2006). This incurs significant limitations of bone tissue grafts, where mechanical properties are of the utmost importance. Because of this, steps have been taken to try to adjust certain mechanical features. One strategy is to explore the development of composite materials with contrasting ratios of bioceramics and polymers (Brunello et al., 2020). Preliminary studies using polymers like polyethers and ketones, or acids like PLGA have shown to increase load bearing capacity and strain, but more research needs to be conducted for definitive conclusions to be stated (Zong et al., 2014; Yu H. et al., 2018). Once an optimized CaP bioceramic material is created, normal scaffolding methodologies can be used, substituting the CaP bioceramic as the main biomaterial.
2.2 Bioactive glass
Like bioceramics, bioactive glasses are an extremely attractive material for BTE due to their ability to attach to bone and induce bone formation. These materials are categorized as silicate-based materials with a Si-O-Si bonding network compatible with human tissue. With the addition of different ion modifiers, new derivatives of the Si-O-Si network can be constructed (Jones et al., 2010). A unique property of BGs allows for the release of various metallic ions from its glass structure, and with this researchers can obtain favored biological effects from tissue samples (Kargozar et al., 2019; Westhauser et al., 2020a). These ionic dissolution products permeate the native bone environment and affect osteoinductive ability, seemingly upregulating multiple gene families associated with bone production in vivo (Crush et al., 2021).
The osteogenic properties of BGs and their ability to release significant amounts of ions into the surrounding medium is a result of their inherent atomic structure. When submerged in physiological fluids present in the body, BGs become partially soluble (Vallet-Regi and Salinas, 2021). This causes an ionic exchange between BG material and protons in the solution, forming hydroxyl groups as a product (Crush et al., 2021). Further ion exchange also releases silicon from the BG and leads to the formation of a preliminary silica gel layer. Incorporation of hydroxyl groups and carbonate groups atop the silica gel layer creates a hydroxyl carbonated apatite [HCA] layer (Crush et al., 2021). This biologically compatible layer establishes as a continuous bond and rapidly interfaces with living bone in vivo (Yu Y. et al., 2018). Proteins and osteoprogenitor MSCs within the BG scaffold are drawn to the bone surface after the formation of the HCA layer, and start to integrate with native bone (Crush et al., 2021). This process is highlighted below in Figure 1.
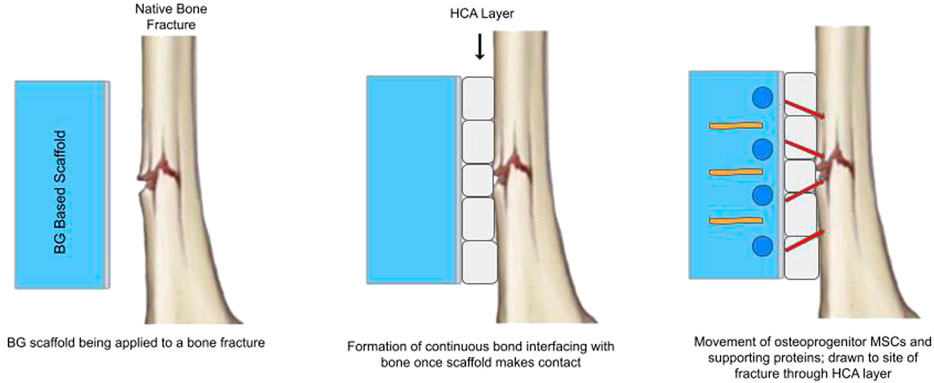
FIGURE 1. Simplified schematic of Bioactive Glass establishing continuous HCA bond, allowing for bioactive modifier and osteoprogenitor stem cell interfacing.
Although preliminary bioactive glasses displayed improved bioactivity, its chemical/structural limitations and crystallization tendencies oftentimes prevent viable BTE scaffolds from being fabricated. Factors like temperature and viscosity range may break certain bonds in the glass network and allow it to rearrange to a weaker crystalline structure (Arstila et al., 2007). Like CaP bioceramics, BGs tend to display weak mechanical and stress bearing properties. To accommodate native bones’ requirement of increased porosity, mesoporous bioactive glasses [MBGs] have recently garnered much attention. MBGs possess a similar composition to traditional BGs and can still be modified into different derivatives by ion substitution processes. However, their highly ordered mesoporous structure and high pore volume allow them to display unique structural characteristics, further improving biocompatibility and presenting great potential for future applications (Zhang et al., 2016; Wang et al., 2019). In addition, different fabrication techniques can affect the mechanical properties of MBGs; novel 3-D printing methods have shown to increase the mechanical strength of MBG scaffolds by almost 200 fold (Wu and Chang, 2012).
3 Various osteogenic inorganic-ion modifiers
Ion-functionalized scaffolds are composed of ion-doped (ion-modified) materials conducive to bone tissue regeneration. Incorporating different ion-doped bioceramic or BG materials in a novel scaffold may enhance an osteoinductive and stable environment for tissue proliferation. Current ions being researched presently were discovered at a higher concentration in native bone tissue or in localized trace elements observed in vivo. The effects of some ions are more known, while others are still under observation.
3.1 Calcium
Many different bioactive metal ions have been tested in the context of bone repair, with calcium being a prime example (Jiang et al., 2021). Being a core component of CaP bioceramics, its osteoinductive properties have been studied extensively. As the most abundant mineral in the body, it has a well-defined critical role; its extracellular presence is detected by the calcium sensing receptor (CaSR) and serves as a stimulus for differentiating MAPK signaling pathways (González-Vázquez et al., 2014). CaSR is a G-protein coupled receptor whose expression in osteoblasts plays a vital role in cell regulation (Ye et al., 2016); an increased concentration of Ca2+ ions significantly promotes osteogenic differentiation and bone marrow stem cell proliferation (Ye et al., 2016; Jiang et al., 2021). Multiple in vitro studies demonstrate that calcium loading into scaffolds encourages osteoinduction in MSCs (Motamedian et al., 2015; Aquino-Martínez et al., 2017; Lim et al., 2017; Lee et al., 2018).
3.2 Silver
Silver ions are known for antibacterial properties and have been shown to inhibit bacterial growth when released as a dissolution product (Hoppe et al., 2011). In a recent study conducted employing a rabbit model, preparation of a 3D-porous composite scaffold utilizing Ag+ ions was shown to have effectively eliminated bacterial infection and inhibit biofilm formation while still promoting bone repair (Weng et al., 2020). Furthermore, additional studies conducted using silver nanoparticles show significant enhancement of bone cell mineralization and differentiation (Deng et al., 2018; Qing et al., 2018). This indicates minimal interference with osteogenesis markers, and thus harbors a strong future potential for the use of silver as an antibacterial agent.
3.3 Strontium
Due to its physical and chemical properties similar to calcium, strontium has been widely researched in relation to bone regeneration. In osteoporosis, a strontium based drug derivative has been commonly used for treatment; this element can be substituted in lieu of calcium in certain regions of bone mineral (Kargozar et al., 2019). By upregulating osteoblast marker genes, strontium itself has shown to enhance osteogenic differentiation while also inhibiting osteoclast activity (Yang et al., 2011; O’Neill et al., 2018). Several iterations of BGs and CaP bioceramics have been developed where calcium is partially substituted with strontium, and most studies conclude a greater osteoconductive effect on new bone formation (Kargozar et al., 2019; Fredholm et al., 2012; Hoppe et al., 2014; Bellucci et al., 2018). However, this substitution does little to change the poor mechanical properties of these biomaterials and is a separate concern that still needs to be addressed.
3.4 Zinc
Zinc ions are an essential trace element required for normal skeletal growth; its ability to induce collagen synthesis and bone mineralization notably help enhance osteogenesis and has been demonstrated in vitro using fibrous scaffolds (Yang et al., 2011; Lim et al., 2017; Deng et al., 2018). Like strontium it helps support osteogenic activity while also simultaneously suppressing osteoclast activity (O’Neill et al., 2018). Additionally, like silver zinc is able to provide anti-bacterial activity and is also recognized as an antioxidant and inflammatory agent (O’Neill et al., 2018; Neščáková et al., 2019). Direct effects of zinc on bone cells are still not completely understood, yet its substantial impact in relation to bone growth have prompted future avenues of research employing Zn-containing BGs and bioceramics.
3.5 Other ions
Many more ions have been subject to extensive scientific examination in the context of BTE. In a microenvironment as complex as native bone tissue, an abundance of ions is of little surprise. However, research is still being conducted on the most impactful ions that would best be incorporated into a functional scaffold. Table 1 summarizes the biological responses of other common inorganic ions currently being explored in the context of BTE. Notably, many of these ions have only been explored preliminarily to identify their effect on osteogenic activity and no definitive conclusions with modified scaffolds have been made yet. The possibility of heavy metal poisoning with different ion concentrations is one significant concern that still needs proper evaluation, along with others. Because of these concerns and also due to the preliminary stage the research is in, most studies have been done in an in vitro environment.
4 Conclusion and future perspectives
In the pursuit of an optimized scaffold for bone tissue regeneration, many of these ion-doped materials could serve as an addition to a novel scaffold to further promote a stable osteogenic environment. Recent experiments over the last decade have successfully created distinct ion doped BGs and CaP bioceramics as scaffold materials. Many of these were created using existing fabrication techniques that facilitate ion deposition onto the surface of the biomaterial. Depending on the specific ion used and its effect on osteogenesis, different optimization properties were observed. Although current studies are still in preliminary stages, the osteogenic potential demonstrated using inorganic ions strongly indicate its future potential and various clinical applications.
Multiple future directions of research are available to translate these in vitro results into functional scaffolds capable of supporting grafts for clinical use. Primarily, biomaterial enhancement with polymer-based materials needs to be investigated more thoroughly. Although BGs and CaP bioceramics have proven to be a bone conducive scaffold material, severe mechanical and structural limitations prevent them from ever becoming a truly functional bone tissue scaffold. Identifying directions of structural enhancement can help change their inherent mechanical properties and increase stress bearing capabilities.
Additionally, the use of biomaterials with multiple functional ion-modifiers characterizes a promising direction for research. Current scaffolds have only utilized one ion at a time to generate osteoinductive effects. An ideal scaffold would incorporate multiple therapeutic ions, with each modifier factoring their own unique properties. Multiple ion modifiers would also better mimic the bone microenvironment and increase the overall functionality of the scaffold. Once a suitable scaffold has been created, the next step is to resolve the long-term direct effects of ions. This can be studied upon in vivo animal implantation with a functional scaffold to ensure the safety of inorganic metal ions in long-term grafts. While the prospect of fabricating a fully optimized scaffold for BTE may seem far-fetched, the promise of so many potential avenues of research present it as an attainable goal that scientists around the world can set their sights on.
Author contributions
The author confirms being the sole contributor of this work and has approved it for publication.
Funding
The author(s) declare financial support was received for the research, authorship, and/or publication of this article. This paper was funded by Tisch Library OA Publishing Fund from Tufts University.
Acknowledgments
Tufts University Department of Biomedical Engineering, Nisha Iyer, Justin Wang, Maddy Porter Ariana Barreiro, Ryanne Barrett.
Conflict of interest
The author declares that the research was conducted in the absence of any commercial or financial relationships that could be construed as a potential conflict of interest.
Publisher’s note
All claims expressed in this article are solely those of the authors and do not necessarily represent those of their affiliated organizations, or those of the publisher, the editors and the reviewers. Any product that may be evaluated in this article, or claim that may be made by its manufacturer, is not guaranteed or endorsed by the publisher.
Abbreviations
BTE, Bone tissue engineering; ECM, Extracellular Matrix; HCA, Hydroxycarbonate Apatite; MSC, Mesenchymal Stem Cell; BG, Bioactive Glass; HCA, Hydroxyl Carbonated Apatite; MBG, Mesoporous Bioactive Glass; CaP, Calcium Phosphate.
References
Ali Akbari Ghavimi, S., Allen, B. N., Stromsdorfer, J. L., Kramer, J. S., Li, X., and Ulery, B. D. (2018). Calcium and phosphate ions as simple signaling molecules with versatile osteoinductivity. Biomed. Mat. 13, 055005. doi:10.1088/1748-605x/aac7a5
Ambard, A. J., and Mueninghoff, L. (2006). Calcium phosphate cement: review of mechanical and biological properties. J. Prosthodont. 15, 321–328. doi:10.1111/j.1532-849x.2006.00129.x
Amini, A. R., Laurencin, C. T., and Nukavarapu, S. P. (2012). Bone tissue engineering: recent advances and challenges. Crit. Rev. Biomed. Eng. 40, 363–408. doi:10.1615/critrevbiomedeng.v40.i5.10
Aquino-Martínez, R., Angelo, A. P., and Pujol, F. V. (2017). Calcium-containing scaffolds induce bone regeneration by regulating mesenchymal stem cell differentiation and migration. Stem Cell Res. Ther. 8, 265. doi:10.1186/s13287-017-0713-0
Arstila, H., Vedel, E., Hupa, L., and Hupa, M. (2007). Factors affecting crystallization of bioactive glasses. J. Eur. Ceram. Soc. 27, 1543–1546. doi:10.1016/j.jeurceramsoc.2006.04.017
Barrioni, B. R., Naruphontjirakul, P., Norris, E., Li, S., Kelly, N. L., Hanna, J. V., et al. (2019a). Effects of manganese incorporation on the morphology, structure and cytotoxicity of spherical bioactive glass nanoparticles. J. Colloid Interface Sci. 547, 382–392. doi:10.1016/j.jcis.2019.04.016
Barrioni, B. R., Norris, E., Li, S., Naruphontjirakul, P., Jones, J. R., and Pereira, M. de M. (2019b). Osteogenic potential of sol–gel bioactive glasses containing manganese. J. Mat. Sci. Mat. Med. 30, 86. doi:10.1007/s10856-019-6288-9
Beck, G. R., Zerler, B., and Moran, E. (2000). Phosphate is a specific signal for induction of osteopontin gene expression. Proc. Natl. Acad. Sci. 97, 8352–8357. doi:10.1073/pnas.140021997
Bellucci, D., Cannillo, V., Anesi, A., Salvatori, R., Chiarini, L., Manfredini, T., et al. (2018). Bone regeneration by novel bioactive glasses containing strontium and/or magnesium: A preliminary in-vivo study. Materials 11, 2223. doi:10.3390/ma11112223
Bernhardt, A., Bacova, J., Gbureck, U., and Gelinsky, M. (2021). Influence of Cu2+ on osteoclast formation and activity in vitro. Int. J. Mol. Sci. 22, 2451. doi:10.3390/ijms22052451
Brunello, G., Panda, S., Schiavon, L., Sivolella, S., Biasetto, L., and Del Fabbro, M. (2020). The impact of bioceramic scaffolds on bone regeneration in preclinical in vivo studies: A systematic review. Materials 13, 1500. doi:10.3390/ma13071500
Choi, S., Kim, K.-J., Cheon, S., Kim, E.-M., Kim, Y.-A., Park, C., et al. (2020). Biochemical activity of magnesium ions on human osteoblast migration. Biochem. Biophys. Res. Commun. 531, 588–594. doi:10.1016/j.bbrc.2020.07.057
Crush, J., Hussain, A., Seah, K. T. M., and Khan, W. S. (2021). Bioactive glass: methods for assessing angiogenesis and osteogenesis. Front. Cell Dev. Biol. 9, 643781. doi:10.3389/fcell.2021.643781
Daculsi, G., Legeros, R. Z., Nery, E., Lynch, K., and Kerebel, B. (1989). Transformation of biphasic calcium phosphate ceramicsin vivo: ultrastructural and physicochemical characterization. J. Biomed. Mat. Res. 23, 883–894. doi:10.1002/jbm.820230806
Dang, W., Li, T., Li, B., Ma, H., Zhai, D., Wang, X., et al. (2018). A bifunctional scaffold with CuFeSe2 nanocrystals for tumor therapy and bone reconstruction. Biomaterials 160, 92–106. doi:10.1016/j.biomaterials.2017.11.020
Deng, Y., Yang, L., Huang, X., Chen, J., Shi, X., Yang, W., et al. (2018). Dual Ag/ZnO-decorated micro-/nanoporous sulfonated polyetheretherketone with superior antibacterial capability and biocompatibility via layer-by-layer self-assembly strategy. Macromol. Biosci. 18, 1800028. doi:10.1002/mabi.201800028
Drynda, A., Drynda, S., Kekow, J., Lohmann, C. H., and Bertrand, J. (2018). Differential effect of cobalt and chromium ions as well as CoCr particles on the expression of osteogenic markers and osteoblast function. Int. J. Mol. Sci. 19, 3034. doi:10.3390/ijms19103034
Echeverria Molina, M. I., Malollari, K. G., and Komvopoulos, K. (2021). Design challenges in polymeric scaffolds for tissue engineering. Front. Bioeng. Biotechnol. 9, 617141. doi:10.3389/fbioe.2021.617141
Eliaz, N., and Metoki, N. (2017). Calcium phosphate bioceramics: A review of their history, structure, properties, coating technologies and biomedical applications. Materials 10, 334. doi:10.3390/ma10040334
Foroutan, F., McGuire, J., Gupta, P., Nikolaou, A., Kyffin, B. A., Kelly, N. L., et al. (2019). Antibacterial copper-doped calcium phosphate glasses for bone tissue regeneration. ACS Biomater. Sci. Eng. 5, 6054–6062. doi:10.1021/acsbiomaterials.9b01291
Fredholm, Y. C., Karpukhina, N., Brauer, D. S., Jones, J. R., Law, R. V., and Hill, R. G. (2012). Influence of strontium for calcium substitution in bioactive glasses on degradation, ion release and apatite formation. J. R. Soc. Interface. 9, 880–889. doi:10.1098/rsif.2011.0387
Fu, Q., Rahaman, M. N., Fu, H., and Liu, X. (2010). Silicate, borosilicate, and borate bioactive glass scaffolds with controllable degradation rate for bone tissue engineering applications. I. Preparation and in vitro degradation. J. Biomed. Mat. Res. A 95A, 164–171. doi:10.1002/jbm.a.32824
Ginebra, M.-P., Espanol, M., Maazouz, Y., Bergez, V., and Pastorino, D. (2018). Bioceramics and bone healing. EFORT Open Rev. 3, 173–183. doi:10.1302/2058-5241.3.170056
González-Vázquez, A., Planell, J. A., and Engel, E. (2014). Extracellular calcium and CaSR drive osteoinduction in mesenchymal stromal cells. Acta Biomater. 10, 2824–2833. doi:10.1016/j.actbio.2014.02.004
He, L. Y., Zhang, X. M., Liu, B., Tian, Y., and Ma, W. H. (2016). Effect of magnesium ion on human osteoblast activity. Braz. J. Med. Biol. Res. Rev. Bras. Pesqui. Medicas E Biol. 49, e5257–S0100. doi:10.1590/1414-431x20165257
Hoppe, A., Güldal, N. S., and Boccaccini, A. R. (2011). A review of the biological response to ionic dissolution products from bioactive glasses and glass-ceramics. Biomaterials 32, 2757–2774. doi:10.1016/j.biomaterials.2011.01.004
Hoppe, A., Sarker, B., Detsch, R., Hild, N., Mohn, D., Stark, W. J., et al. (2014). In vitro reactivity of Sr-containing bioactive glass (type 1393) nanoparticles. J. Non-Cryst. Solids. 387, 41–46. doi:10.1016/j.jnoncrysol.2013.12.010
Jiang, S., Wang, M., and He, J. (2021). A review of biomimetic scaffolds for bone regeneration: toward a cell-free strategy. Bioeng. Transl. Med. 6, e10206. doi:10.1002/btm2.10206
Jones, J. R., Lin, S., Yue, S., Lee, P. D., Hanna, J. V., Smith, M. E., et al. (2010). Bioactive glass scaffolds for bone regeneration and their hierarchical characterisation. Proc. Inst. Mech. Eng. H. 224, 1373–1387. doi:10.1243/09544119jeim836
Kargozar, S., Montazerian, M., Fiume, E., and Baino, F. (2019). Multiple and promising applications of strontium (Sr)-Containing bioactive glasses in bone tissue engineering. Front. Bioeng. Biotechnol. 7, 161. doi:10.3389/fbioe.2019.00161
Klein, C., de Groot, K., Chen, W., Li, Y., and Zhang, X. (1994). Osseous substance formation induced in porous calcium phosphate ceramics in soft tissues. Biomaterials 15, 31–34. doi:10.1016/0142-9612(94)90193-7
Lee, M. N., Hwang, H.-S., Oh, S.-H., Roshanzadeh, A., Kim, J.-W., Song, J. H., et al. (2018). Elevated extracellular calcium ions promote proliferation and migration of mesenchymal stem cells via increasing osteopontin expression. Exp. Mol. Med. 50, 1–16. doi:10.1038/s12276-018-0170-6
Lepry, W. C., Naseri, S., and Nazhat, S. N. (2017). Effect of processing parameters on textural and bioactive properties of sol–gel-derived borate glasses. J. Mat. Sci. 52, 8973–8985. doi:10.1007/s10853-017-0968-y
Li, J., Li, J., Wei, Y., Xu, N., Li, J., Pu, X., et al. (2021a). Ion release behavior of vanadium-doped mesoporous bioactive glass particles and the effect of the released ions on osteogenic differentiation of BMSCs via the FAK/MAPK signaling pathway. J. Mat. Chem. B 9, 7848–7865. doi:10.1039/d1tb01479j
Li, J., Li, X., Li, J., Pu, X., Wang, J., Huang, Z., et al. (2021b). Effects of incorporated vanadium and its chemical states on morphology and mesostructure of mesoporous bioactive glass particles. Microporous Mesoporous Mater 319, 111061. doi:10.1016/j.micromeso.2021.111061
Li, X., van Blitterswijk, C. A., Feng, Q., Cui, F., and Watari, F. (2008). The effect of calcium phosphate microstructure on bone-related cells in vitro. Biomaterials 29, 3306–3316. doi:10.1016/j.biomaterials.2008.04.039
Lim, S. S., Chai, C. Y., and Loh, H.-S. (2017). In vitro evaluation of osteoblast adhesion, proliferation and differentiation on chitosan-TiO2 nanotubes scaffolds with Ca2+ ions. Mat. Sci. Eng. C 76, 144–152. doi:10.1016/j.msec.2017.03.075
Motamedian, S. R., Hosseinpour, S., Ahsaie, M. G., and Khojasteh, A. (2015). Smart scaffolds in bone tissue engineering: A systematic review of literature. World J. Stem cells. 7, 657–668. doi:10.4252/wjsc.v7.i3.657
Neščáková, Z., Zheng, K., Liverani, L., Nawaz, Q., Galusková, D., Kaňková, H., et al. (2019). Multifunctional zinc ion doped sol – gel derived mesoporous bioactive glass nanoparticles for biomedical applications. Bioact. Mat. 4, 312–321. doi:10.1016/j.bioactmat.2019.10.002
O’Neill, E., Awale, G., Daneshmandi, L., Umerah, O., and Lo, K. W.-H. (2018). The roles of ions on bone regeneration. Drug Discov. Today. 23, 879–890. doi:10.1016/j.drudis.2018.01.049
Perez, J. R., Kouroupis, D., Li, D. J., Best, T. M., Kaplan, L., and Correa, D. (2018). Tissue engineering and cell-based Therapies for fractures and bone defects. Front. Bioeng. Biotechnol. 6, 105. doi:10.3389/fbioe.2018.00105
Perni, S., Yang, L., Preedy, E. C., and Prokopovich, P. (2018). Cobalt and Titanium nanoparticles influence on human osteoblast mitochondrial activity and biophysical properties of their cytoskeleton. J. Colloid Interface Sci. 531, 410–420. doi:10.1016/j.jcis.2018.07.028
Poli, E., Magnaudeix, A., Damia, C., Lalloué, F., Chaleix, V., Champion, E., et al. (2019). Advanced protocol to functionalize CaP bioceramic surface with peptide sequences and effect on murine pre-osteoblast cells proliferation. Bioorg. Med. Chem. Lett. 29, 1069–1073. doi:10.1016/j.bmcl.2019.03.002
Prasadh, S., Gupta, M., and Wong, R. (2022). In vitro cytotoxicity and osteogenic potential of quaternary Mg-2Zn-1Ca/X-Mn alloys for craniofacial reconstruction. Sci. Rep. 12, 8259. doi:10.1038/s41598-022-12490-0
Qing, T., Mahmood, M., Zheng, Y., Biris, A. S., Shi, L., and Casciano, D. A. (2018). A genomic characterization of the influence of silver nanoparticles on bone differentiation in MC3T3-E1 cells. J. Appl. Toxicol. 38, 172–179. doi:10.1002/jat.3528
Samavedi, S., Whittington, A. R., and Goldstein, A. S. (2013). Calcium phosphate ceramics in bone tissue engineering: A review of properties and their influence on cell behavior. Acta Biomater. 9, 8037–8045. doi:10.1016/j.actbio.2013.06.014
Schatkoski, V. M., Larissa do Amaral Montanheiro, T., Canuto de Menezes, B. R., Pereira, R. M., Rodrigues, K. F., Ribas, R. G., et al. (2021). Current advances concerning the most cited metal ions doped bioceramics and silicate-based bioactive glasses for bone tissue engineering. Ceram. Int. 47, 2999–3012. doi:10.1016/j.ceramint.2020.09.213
Tang, G., Liu, Z., Liu, Y., Yu, J., Wang, X., Tan, Z., et al. (2021). Recent trends in the development of bone regenerative biomaterials. Front. Cell Dev. Biol. 9, 665813. doi:10.3389/fcell.2021.665813
Vallet-Regí, M., and Ruiz-Hernández, E. (2011). Bioceramics: from bone regeneration to cancer nanomedicine. Adv. Mat. 23, 5177–5218. doi:10.1002/adma.201101586
Vallet-Regi, M., and Salinas, A. J. (2021). Mesoporous bioactive glasses for regenerative medicine. Mat. Today bio. 11, 100121. doi:10.1016/j.mtbio.2021.100121
Wang, Y., Pan, H., and Chen, X. (2019). The preparation of hollow mesoporous bioglass nanoparticles with excellent drug delivery capacity for bone tissue regeneration. Front. Chem. 7, 283. doi:10.3389/fchem.2019.00283
Wei, H., Cui, J., Lin, K., Xie, J., and Wang, X. (2022). Recent advances in smart stimuli-responsive biomaterials for bone therapeutics and regeneration. Bone Res. 10, 17. doi:10.1038/s41413-021-00180-y
Weng, W., Li, X., Nie, W., Liu, H., Liu, S., Huang, J., et al. (2020). <p>One-Step preparation of an AgNP-nHA@RGO three-dimensional porous scaffold and its application in infected bone defect treatment</p>. Int. J. Nanomedicine. 15, 5027–5042. doi:10.2147/ijn.s241859
Westhauser, F., Hohenbild, F., Arango-Ospina, M., Schmitz, S. I., Wilkesmann, S., Hupa, L., et al. (2020a). Bioactive glass (BG) ICIE16 shows promising osteogenic properties compared to crystallized 45S5-BG. Int. J. Mol. Sci. 21, 1639. doi:10.3390/ijms21051639
Westhauser, F., Wilkesmann, S., Nawaz, Q., Schmitz, S. I., Moghaddam, A., and Boccaccini, A. R. (2020b). Osteogenic properties of manganese-doped mesoporous bioactive glass nanoparticles. J. Biomed. Mat. Res. A 108, 1806–1815. doi:10.1002/jbm.a.36945
Wu, C., and Chang, J. (2012). Mesoporous bioactive glasses: structure characteristics, drug/growth factor delivery and bone regeneration application. Interface Focus 2, 292–306. doi:10.1098/rsfs.2011.0121
Yang, F., Yang, D., Tu, J., Zheng, Q., Cai, L., and Wang, L. (2011). Strontium enhances osteogenic differentiation of mesenchymal stem cells and in vivo bone formation by activating wnt/catenin signaling. Stem Cells 29, 981–991. doi:10.1002/stem.646
Ye, J., Ai, W., Zhang, F., Zhu, X., Shu, G., Wang, L., et al. (2016). Enhanced proliferation of porcine bone marrow mesenchymal stem cells induced by extracellular calcium is associated with the activation of the calcium-sensing receptor and ERK signaling pathway. Stem Cells Int. 2016, 6570671–6570711. doi:10.1155/2016/6570671
Yu, H., Chen, Y., Mao, M., Liu, D., Ai, J., and Leng, W. (2018a). PEEK-biphasic bioceramic composites promote mandibular defect repair and upregulate BMP-2 expression in rabbits. Mol. Med. Rep. 17, 8221–8227. doi:10.3892/mmr.2018.8867
Yu, Y., Bacsik, Z., and Edén, M. (2018b). Contrasting in vitro apatite growth from bioactive glass surfaces with that of spontaneous precipitation. Materials 11, 1690. doi:10.3390/ma11091690
Zhang, X., Zeng, D., Li, N., Wen, J., Jiang, X., Liu, C., et al. (2016). Functionalized mesoporous bioactive glass scaffolds for enhanced bone tissue regeneration. Sci. Rep. 6, 19361. doi:10.1038/srep19361
Zhu, X. D., Zhang, H. J., Fan, H. S., Li, W., and Zhang, X. D. (2010). Effect of phase composition and microstructure of calcium phosphate ceramic particles on protein adsorption. Acta Biomater. 6, 1536–1541. doi:10.1016/j.actbio.2009.10.032
Keywords: smart scaffolds, bone tissue engineering, scaffold optimization, biomaterials, ion-doped modifiers, bioactive glass, extracellular matrix (ECM)
Citation: Basavarajappa Y (2023) Ion—modified optimization of smart scaffolds in bone tissue regeneration. Front. Front. Biomater. Sci. 2:1289382. doi: 10.3389/fbiom.2023.1289382
Received: 05 September 2023; Accepted: 25 September 2023;
Published: 11 October 2023.
Edited by:
Ciara M. Murphy, Royal College of Surgeons in Ireland, IrelandReviewed by:
Iram Maqsood, University of Maryland, United StatesCopyright © 2023 Basavarajappa. This is an open-access article distributed under the terms of the Creative Commons Attribution License (CC BY). The use, distribution or reproduction in other forums is permitted, provided the original author(s) and the copyright owner(s) are credited and that the original publication in this journal is cited, in accordance with accepted academic practice. No use, distribution or reproduction is permitted which does not comply with these terms.
*Correspondence: Yashas Basavarajappa, eWFzaGFzLmJhc2F2YXJhamFwcGFAdHVmdHMuZWR1