- 1Nanobiotechnology Department, Faculty of Innovative Science and Technology, Razi University, Kermanshah, Iran
- 2Department of Surgical Sciences, Division of Functional Pharmacology and Neuroscience, Uppsala University, Uppsala, Sweden
- 3Biosensor Research Center, Endocrinology and Metabolism Molecular–Cellular Sciences Institute, Tehran University of Medical Sciences, Tehran, Iran
- 4Sensor and Biosensor Research Center (SBRC), Faculty of Chemistry, Razi University, Kermanshah, Iran
- 5Endocrinology and Metabolism Research Center, Endocrinology and Metabolism Clinical Sciences Institute, Tehran University of Medical Sciences, Tehran, Iran
Diabetes mellitus (DM) is a prevalent disorder with an urgent need for continuous, precise, and on-site biomarker monitoring devices. The continuous monitoring of DM biomarkers from different biological matrices will become routine in the future, thanks to the promising biosensor design. Lately, employing different nanomaterials in biosensor receptor parts has had a great impact on smart DM monitoring. Among them, gold nanostructures (AuNSs) have arisen as highly potential materials in fabricating precise DM biosensors due to their unique properties. The present study provides an update on the applications of AuNSs in biosensors for detecting glucose as well as other DM biomarkers, such as glycated hemoglobin (HbA1c), glycated albumin (GA), insulin, insulin antibodies, uric acid, lactate, and glutamic acid decarboxylase antibodies (GADA), with a focus on the most important factors in biosensor performance such as sensitivity, selectivity, response time, and stability. Specified values of limit of detection (LOD), linear concentrations, reproducibility%, recovery%, and assay time were used to compare studies. In conclusion, AuNSs, owing to the wide electrochemical potential window and low electrical resistivity, are valuable tools in biosensor design, alongside other biological reagents and/or nanomaterials.
Highlights
1. AuNSs presented outstanding properties such as chemical stability, high electrical conductivity, a large specific surface area, low cost of synthesis, and ease of functionalization.
2. The utilization of AuNSs in biosensors is an excellent opportunity to detect all diabetes biomarkers, such as glucose, HbA1c, GA insulin, IAA, and GADA.
3. AuNSs can improve the efficiency, sensitivity, specificity, and stability of the diabetes biosensors.
4. AuNS properties can increase the efficiency, sensitivity, and specificity of the biosensor through intrinsic specific molecule recognition capacity, different signal transduction amplification methods, fast electron transfer, and the ability to stabilize GOx structures and/or antibodies over proper immobilization on electrodes, serving as fluorescent and colorimetric materials.
5. Further consideration is required to translate AuNS-based technologies into routine and functional biosensors, as the properties of AuNSs are dependent on their size, shape, and spatial arrangement.
1 Introduction
Diabetes mellitus (DM) is a chronic metabolic disorder recognized by persistently elevated blood glucose levels, commonly due to defects in insulin secretion, function, or absorption mechanisms (Association, 2014). It is the ninth leading cause of death globally, as reported by the World Health Organization (WHO) (Khan et al., 2020). The number of people with DM is predicted to increase by 700 million by the year 2045 worldwide because of the modern lifestyle (Saeedi et al., 2019). DM can lead to other long-term adverse health effects, including the escalated risk of cardiovascular disease (Damaskos et al., 2020), hypokalemia (Frier, 2014), nephropathy (Reutens and Atkins, 2011), retinopathy (Shah et al., 2021), blindness (Stratton et al., 2001), foot damage (Vas et al., 2018), falls (Macgilchrist et al., 2010), amputation (Khalil et al., 2023), cerebral edema (Durr et al., 1992), dementia (Pasquier et al., 2006), skin conditions (Wollina et al., 2020), and disability (Yoon and Kim, 2019) which profoundly affect patients’ quality of life (Jitendra et al., 2024). Although DM has no certain cure, continuous follow-up of the body’s glucose levels for appropriate management can minimize the disease complications and reduce its severity (Alam et al., 2021).
The current standard of DM monitoring is through the invasive blood pricking technique for the detection of glucose (Kirk and Stegner, 2010). Typically, this method is reliable; however, the repetitive pricking in the long term is inconvenient for patients and can simply cause irritation and infections (Reddy et al., 2022). The current limitations of existing continuous monitoring biosensors are issues related to reliability, accessibility, complexity, cost, and time (Pullano et al., 2022). In contrast, the non-invasive, real-time, and continuous monitoring of glucose from different biological matrices (i.e., blood, urine, saliva, breath, interstitial fluids, tears, and sweat) has the potential to become routine in the near future (Laha et al., 2022). Furthermore, detecting other DM biomarkers such as insulin, insulin antibodies, HbA1c, GA, and acetone from different biological matrices using novel biosensor technology has remained an active subject of research (Reddy et al., 2022). This offers advantages such as low cost, the ability to detect low concentrations of biomarkers, and time and labor efficiency (Ahmadi et al., 2020; Laha et al., 2022; Wang et al., 2022; Jadhav et al., 2023; Mandali et al., 2023; Psoma and Kanthou, 2023). Different types of advanced point-of-care (POC) platforms make the monitoring of the DM biomarkers more precise, straightforward, safe, and less uncomfortable for patients (Teymourian et al., 2020; Liu Y. et al., 2022; Khor et al., 2022; Li and Chen, 2023; Safarkhani et al., 2023; Hu et al., 2024) (Figure 1).
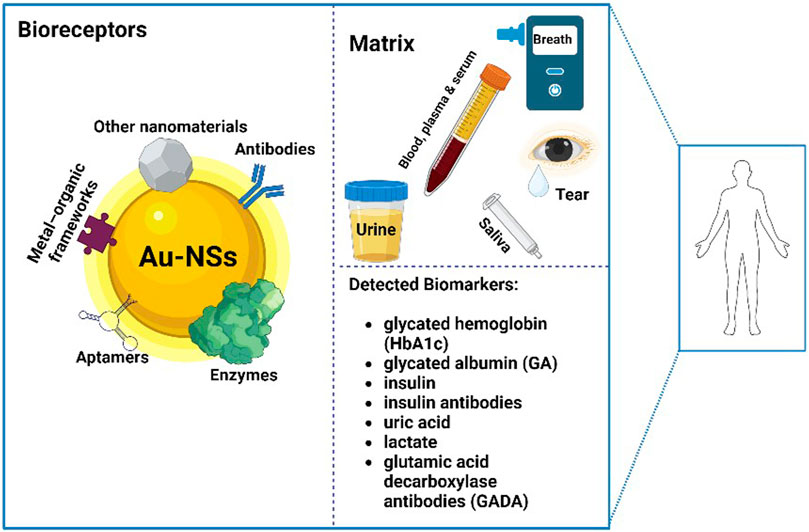
Figure 1. The principles of detecting diabetes mellitus biomarkers from different matrices in the human body using various bioreceptors and gold nanostructures.
Recently, advances in nanomaterials have prompted the designing of promising receptors for the development of cost-effective, on-site, and smart biosensors for DM monitoring (Shoaib et al., 2023). For instance, the surface area of the biosensors can be increased via nanomaterials, which results in generating greater current, more rapid reactions, and improved catalytic activity (Cho et al., 2020). Signal-to-noise ratio improvement is possible due to the amplifying properties of nanomaterials (Kumar et al., 2019). In addition, when designing multifunctional bioreceptors, different nanomaterials and/or biomolecules can be used in the biosensor simultaneously (Theyagarajan and Kim, 2023), or a susceptible biological substance can be replaced by mimicking materials entirely (Mohammadpour-Haratbar et al., 2022). These sensors are intended for the rapid detection of DM biomarkers with high selectivity, sensitivity, accuracy, and low detection limits (El-Safty and Shenashen, 2020; Welch et al., 2021). Last but not least, the upgrading of other biosensor properties such as stability, scalability, miniaturization, wearability, and connectivity to smart devices is possible now thanks to nanotechnology advances (Altintas, 2017; Golsanamlou et al., 2023; Mansour et al., 2024). Different kinds of nanomaterials applied in the construction of DM biosensors, including silver, nickel, carbon-based, and quantum dots, bring their own advantages based on their applications and properties (Malik et al., 2023). Depending on the specific requirements of the biosensors, such as desired sensitivity, stability, cost, and time, gold nanostructures (AuNSs) show great potential in this regard (Dahan et al., 2023).
Accordingly, in recent years, progress has been made in AuNS applications in the construction of biosensors (Naresh and Lee, 2021). More specifically, the AuNSs have emerged as potent tools in the fabrication of highly sensitive DM biosensors (Siciliano et al., 2023). Utilizing gold alongside other nanomaterials or biomolecules, such as enzymes and antibodies, to modify electrodes and fabricate AuNS-based biosensors can lead to improved sensitivity and lower LOD compared to different types of biosensors (Xu et al., 2023). The well-known properties of gold, such as the relatively simple synthesis methods of AuNSs, the ability to serve as an effective nanomaterial for biomolecule immobilization, and integration with other nanomaterials to fabricate effective biosensors with an increased load of biomolecules per unit mass of particles, make it a promising choice for biosensor applications (Oliveira et al., 2023; Patil et al., 2023; Zdarta et al., 2023). Additionally, the potential of AuNSs in electron transfer between the electrode surface and the biomolecules highlights the remarkable applications of AuNSs in DM biosensor construction (Deepa et al., 2023).
Some minor drawbacks need to be taken into consideration while designing a DM biosensor based on AuNSs, such as the tendency of the AuNSs to aggregate as well as the detachment or degradation of the surface attachments due to the potential non-specific interactions with biomolecules, oxidation, leaching, and/or corrosion (Demir et al., 2024). These might happen over changes in sample matrix conditions, such as pH, temperature, light, and chemical interactions, which can lead to a loss of AuNS properties and cause potential toxicity (Ngernpimai et al., 2024a). Addressing these challenges requires careful design and optimization of AuNSs, including using stabilizing agents, optimizing surface chemistry, and implementing strategies to minimize environmental conditions (Ngernpimai et al., 2024b). Additionally, a comprehensive study of the impact of relevant conditions is essential to ensure the long-term stability, reliability, and biocompatibility of AuNS-based DM biosensors.
In this regard, Yi et al. reviewed the advances in gold nanomaterial-implemented wearable sensors in general healthcare-related applications with a focus on the gold nanomaterial fabrication method, the working mechanism, and the performance of electrochemical sensors, humidity/gas sensors, strain/pressure sensors, and colorimetric sensors (Yi and Xianyu, 2022). More recently, Patra et al. reviewed previous studies on the applications of Au nanocomposites in enzymatic and non-enzymatic glucose sensing mechanisms (Patra et al., 2024). Several methods of immobilizing glucose oxidase (GOx) on gold nanoparticles (AuNPs) for electrochemical glucose biosensors were described by Lipińska et al. (2021). However, an objective review of AuNS applications in biosensors for all forms of DM biomarker monitoring is needed. Here, we provide an update on the applications of AuNSs in DM monitoring biosensors with a focus on the key factors in real-life biosensor performance. These factors are conductivity potential, linearity, sensitivity, response time, and selectivity when analyzing the DM biomarkers in different biofluid samples. The types of AuNS-based biosensors reviewed here, on the basis of the transduction method, are electrochemical, optical, chemiluminescence, and calorimetric detection in the second and third generation of glucose biosensors. Fourth-generation sensors are not addressed. Other diabetes biomarkers, such as HbA1c, GA, insulin, insulin antibodies, uric acid, lactate, and GADA, are discussed in detail.
2 Gold nanostructure (AuNS) applications in glucose detection
Along with the development of several glucose detection techniques and biosensors, novel practical AuNSs continue to emerge in the non-invasive skin biosensing platforms (Pour et al., 2023). In this regard, Dervisevic et al. proposed a glucose-sensing skin patch applying a high-density silicon micropillar array (MPA) for electrochemically monitoring glucose levels in human sweat (Dervisevic et al., 2021). In their study, the working electrode was modified by depositing a Prussian blue (PB) layer and gold nanoparticle clusters embedded in chitosan (Ch-AuNP) to increase the active surface area, followed by the immobilization of GOx (Dervisevic et al., 2021). The role of the jointed MPAs was physical protection of the immobilized GOx (Dervisevic et al., 2021), which made it possible to detect the glucose from perspiration effectively (Dervisevic et al., 2021). Another wearable biosensing platform was designed by combining a three-dimensional hierarchical porous Au hydrogel-enzyme electrode with soft-MEMS technologies, using GOx with good durability over 15 days and a suitable selectivity (Li et al., 2021). Interestingly, the same study showed that with the assistance of a wireless or a methylene blue Bluetooth module, this wearable sensing platform achieved real-time and non-invasive glucose monitoring on human skin as well (Li et al., 2021). Similarly, continuous lactic acid monitoring was accomplished using lactate oxidase immobilized on the same sensing platform, further verifying the universality of the designed sensing platform (Li et al., 2021). Frajpour et al. developed two high-performance glucose biosensors based on the immobilization of GOx on PB-modified TiO2 nanotube arrays functionalized by Au and AgO NPs, which exhibited satisfactory sensitivity toward glucose (Farajpour et al., 2020). They used AuNPs because of their excellent conductivity, simplicity of fabrication, and cost efficiency (Farajpour et al., 2020). Another PB-based sensing platform was established via the cross-linking enzyme aggregates method (GOxEA@PB/Au/CC) (Yan et al., 2021). The coral-like gold micro/nanostructures were formed onto carbon cloth, followed by a PB electrochemical deposition to construct an electrochemical biosensor to detect both H2O2 and glucose (Yan et al., 2021) (see Table 1).
In addition to electrochemical wearable glucose biosensors, research has been focusing on developing technologies to enable surface-enhanced Raman scattering (SERS) biosensors (Pan et al., 2021). For instance, Pan et al. reported a two-step seed-mediated synthesis of gold core@silver shell nanoparticles (Au@Ag NPs) for detecting diabetes over the etching effect of H2O2 generated from glucose oxidation (Pan et al., 2021). They used Au@Ag NPs as SERS substrates and 4-mercaptobenzoic acid (4-MBA) as the Raman tag to detect glucose concentration (Pan et al., 2021). The role of Au@Ag NPs was to improve the electromagnetic field of SERS owing to their strong plasmonic properties (Pan et al., 2021). Another flexible SERS substrate composed of AuNPs and two-dimensional MXene Ti3C2TX nanosheets has been designed to detect tear glucose (Cui et al., 2022). In the same way, when AuNPs were present, the combination of electromagnetic and chemical enhancement of AuNPs and MXene greatly enhanced the SERS signal (Cui et al., 2022). The GMXeP SERS substrates were used to detect glucose conveniently from diabetic tears with the significant correlation between tear and blood glucose, suggesting that the designed system was suitable for non-invasive and sensitive detection of blood glucose (Cui et al., 2022) (see Table 1).
In another study, for amperometric detection of glucose, GOx was immobilized on AuNPs with the adsorption on a polytyramine layer (AuNPs/Pty) (Khumngern et al., 2021). Then, GOx/AuNP/Pty was coated on a PB-modified screen-printed carbon electrode (SPCE) to produce the GOx/AuNP/Pty/PB/SPCE biosensor (Khumngern et al., 2021). In the same study, the developed amperometric glucose biosensor response was measured through the reduction current of the PB mediator in a flow injection analysis system, displaying a low value for the Michaelis constant (Khumngern et al., 2021). The immobilization of GOx with high affinity was accomplished via AuNPs thanks to the thiol- and amino-functional groups of the enzyme (Khumngern et al., 2021). The immobilization via AuNPs improved the enzyme loading and sensor response without disturbing the enzymatic activity (Khumngern et al., 2021). In the same way, a graphite rod (GR) electrode was modified by AuNPs and PB with GOx to develop an amperometric glucose biosensor in another study, using Nafion (Nf) to produce an Nf-GOx/PB/AuNP/GR biosensor (Sakalauskiene et al., 2023). The AuNPs increased the electrochemically active surface area, improved the GOx immobilization, and yielded a 1.86-fold improvement in analytical signal strength (Sakalauskiene et al., 2023). This study showed AuNSs could be interesting nanomaterials in the interface construction of biosensors due to their low electrical resistivity, relatively wide electrochemical potential window, improved electrooxidation, and fast electron transfer that can enhance the sensitivity and stability of biosensors (Sakalauskiene et al., 2023) (see Table 1).
There has been a boom in developing novel analytic devices that are miniature, user-friendly, rapid, and reliable by combining biosensors with new technologies. Zhang et al. designed a biofuel cell-type sensor device consisting of a glucose biofuel cell, a power management system (PMS), and an indicator module to detect glucose in urine (Zhang et al., 2021). The enzymatic biofuel cells (EBFCs) were manufactured on a flexible substrate by screen printing technology (Zhang et al., 2021). The carbon nanotube (CNT)/hybrids were immobilized on the anode to promote the electron transfer between the active site of enzymes and the electrode surface to improve the output performance of EBFCs (Zhang et al., 2021). The PMS circuit enabled collecting the energy and drive of the light-emitting diode (LED) indicator, whose flash frequency was related to the urine glucose level (Zhang et al., 2021). The designed device was self-powered and held potential application prospects in wearable monitoring systems such as diapers (Zhang et al., 2021). Promsuwan et al. introduced a glucose biosensor that included a smartphone and a battery-less near-field communication (NFC) potentiostat connected to an SPCE modified with a PB-graphene ink and functionalized with AuNP-embedded poly (3,4 ethylene dioxythiophene): polysulfonic acid coated with GOx ((GOx)-AuNP-PEDOT:PSS/PB-G) for glucose detection using an amperometric method (Promsuwan et al., 2023). The PEDOT:PSS was a conductive gel for the entrapment of the GOx-AuNPs and the improvement of electron transfer to the PB-G mediator (Promsuwan et al., 2023). The PB-G was used as a redox mediator to electro-catalyze the reduction of H2O2, which was a byproduct of the GOx reaction (Promsuwan et al., 2023) (Table 1).
The photoluminescence properties of Au in an enzymatic fluorescent probe were developed for the selective detection of glucose using bovine serum albumin stabilized gold nanoclusters (BSA-AuNCs), modified with GOx (Abraham et al., 2024). The red fluorescence exhibited by the probe was quenched by the production of H2O2 on the addition of glucose via a static quenching mechanism, providing UV-visible absorption and fluorescence-lifetime-based glucose sensing (Abraham et al., 2024). In the mentioned study, the fluorescent enzymatic sensing probe served as an off-switch with the production of H2O2, resulting in the selective and sensitive detection of glucose (Abraham et al., 2024). In another work, Zhao et al. demonstrated a glucose electrochemical biosensor through the synergetic labeling strategy utilizing PbS colloidal quantum dots (CQDs) and Au nanospheres (AuNSs) (Zhao et al., 2023). The PbS CQD/AuNS/GOx mixture was immobilized on the carbon electrode surface via the one-step dip-coating method (Zhao et al., 2023). Colorimetric glucose sensors using enzyme-coronated AuNPs have been developed for high-throughput assays (Jang et al., 2022). To increase the selectivity and stability in detecting blood glucose, the biosensors were functionalized with an erythrocyte membrane, which facilitates the permeation of glucose, thanks to glucose-selective membrane proteins (Jang et al., 2022). The performance of the biosensor is represented in Table 1.
3 Au-nanostructure-based biosensors for other diabetes biomarkers
Glycated hemoglobin (HbA1c) is an established DM biomarker, according to the World Health Organization and the American Diabetes Association. HbA1c can be used for the practical long-term diagnosis of the disease in clinical practice as an alternative to glucose (Sherwani et al., 2016). In this regard, an electrochemical HbA1c biosensor with good efficiency was designed based on the electrochemical immune principle. The reproducibility and conductivity of the electrode are improved by depositing AuNPs on the surface of the screen-printed electrode (SPE) (Zhao et al., 2022). The experimental results showed a sensitivity of 0.0938 μA/μg·mL−1 (Zhao et al., 2022). The sensor delivered satisfactory repeatability, stability, and anti-interference performance (Zhao et al., 2022). Additionally, Boonprasert et al. developed multiwalled nanotubes incorporated with gold nanoparticles (POC-HbA1cMWCNTs/AuNPs), used as a routine POC for the detection of HbA1c (Boonprasert et al., 2023). They compared their developed biosensor to the standard HPLC method and showed the accuracy of the POC-HbA1cMWCNTs/AuNPs was 94.18% (Boonprasert et al., 2023). Likewise, a sandwich paper-based electrochemiluminescence (ECL) biosensor was developed using the zirconium metal–organic framework/Fe3O4 (trimethyl chitosan)/gold nanocluster (Zr-MOF/Fe3O4(TMC)/AuNCs) as a tracing tag to label anti-HbA1c monoclonal antibodies and used reduced graphene oxide (rGO) as an immobilization platform for the sensing element (Ahmadi et al., 2021). The fabricated immunosensor demonstrated a desirable assay performance for HbA1c (Ahmadi et al., 2021). Furthermore, a thiol-modified aptamer containing AuNPs bound to HbA1c with high affinity in whole blood samples was synthesized by Devi et al. to produce a stable aptasensor (Devi et al., 2023). The results showed that the thiol groups enhanced the stability of aptamers adsorbed on the surface of AuNPs effectively (Devi et al., 2023) (see Table 2).
However, HbA1c detection is not recommended for specific conditions such as pregnancy, chronic kidney disease, and hemoglobinopathies (Yazdanpanah et al., 2017). In such situations, glycated albumin (GA) can be used as an alternative DM biomarker without the interference of other health issues (Mahobiya et al., 2023). Accordingly, Mahobiya et al. measured the level of GA instead of HbA1c with microscreen-printed electrodes (μSPE) coated with bi-metallic gold-platinum (AuPt) nanomaterial with a synergistic effect (Mahobiya et al., 2023). The developed sensing platform showed an improved response compared to singular Pt nanoparticles (Mahobiya et al., 2023) (see Table 2).
Precise insulin detection is crucial for managing DM through regulated insulin dosage (Turner and Pickup, 1985). Common laboratory analytical methods for insulin detection are usually cost- and time-consuming and lack a real-time and continuous monitoring potential (Psoma and Kanthou, 2023). Therefore, research efforts are aiming toward insulin biosensors to offer a more accurate estimation of insulin (Psoma and Kanthou, 2023). Consequently, a simple sandwich-type electrochemical immunosensor was fabricated using AuNP-adhered metal–organic framework-derived copper–zinc hollow porous carbon nanocubes (Au@Cu5Zn8/HPCNC) and AuNP-deposited nitrogen-doped holey graphene (NHG) was used as a dual functional label and sensing platform (Sakthivel et al., 2022). Similarly, Liu et al. designed an electrochemical aptasensor to detect insulin using laser-scribed graphene electrodes (LSGEs) (Liu J. et al., 2022). The aptasensor was based on using Exonuclease I (Exo I) (Liu J. et al., 2022). The results showed using the aptamer, AuNPs, MB, and Exo I, the signal could be well-correlated to the concentrations of insulin (Liu J. et al., 2022) (see Table 2).
The presence of antibodies against insulin can be part of diagnosing people with type 1 diabetes (Katsarou et al., 2017). In this regard, an electrochemical biosensor for rapid detection of insulin antibodies was developed (Farrokhnia et al., 2022). The fabrication process was based on the optimized sequential electropolymerization of polyaniline and electrodeposition of AuNPs on the surface of the functionalized gold electrode (Farrokhnia et al., 2022). After immobilizing the insulin antigen and blocking with BSA, the biosensor was successfully used to determine different concentrations of insulin antibodies under optimal conditions (Farrokhnia et al., 2022). In addition, an SERS-based biosensor using polyvinylidene fluoride (PVDF) membranes as a flexible support for the detection of GADA and insulin autoantibodies (IAA) was developed (Wang et al., 2023). In the same study, two kinds of silver–gold core-shell nanotags embedded with Raman probes and attached with GADA or IAA were synthesized to capture the targets (GADA and IAA) (Wang et al., 2023). Results showed the probes sandwiched between silver and gold layers guaranteed spectral stability and reliability (Wang et al., 2023). Another electrochemical aptasensor on SPCE was developed for real-time detection of insulin and glucose in saliva (Liu S. et al., 2022). Two specific aptamers for insulin and glucose were fabricated on AuNP SPCEs to form the sensing platform that terminated with methylene blue redox probes (Liu S. et al., 2022) (See Table 2).
In another combinational work, a gold nanopine needle (AuNN)-programmed flexible sweat sensor was developed for real-time monitoring of glucose and lactate levels in human sweat (Yu et al., 2021). The AuNNs were grown on the flexible gold substrate by electrochemical deposition for signal amplification (Yu et al., 2021). The corresponding enzymes were immobilized on the chip via a cross-linker poly (ethylene glycol) diglycidylether (PEGDE) (Yu et al., 2021). Zhou et al. designed a luminescent wearable sweat tape (LWST) biosensor that can be attached to a smartphone (Zhou et al., 2021). It embedded multi-component nanoprobes onto microwell-patterned paper substrates of hollowed-out double-side tapes consisting of responsive luminophores, enzyme-loaded gold nanoclusters (AuNCs), which were wrapped by the switch and MnO2 nanosheets (Zhou et al., 2021). The responsive luminophores were constructed using three substitutable components: first, uricase, GOx, and alcohol dehydrogenase enzymes for molecular target recognition of uric acid, glucose, and alcohol, respectively (Zhou et al., 2021). Second, glutathione-protected AuNCs (yellow, red, and green) for luminescent signal output, and third, polycation PAH (poly (allylamine hydrochloride)) for integration (Zhou et al., 2021). MnO2 NSs as the switch could quench the emission of the AuNCs but be degraded by the reductive product of the incorporated enzymes (Zhou et al., 2021). The results showed the targeting analysts could be detected through a “turn-on” luminescence approach (Zhou et al., 2021) (see Table 2).
4 Challenges and drawbacks in the commercialization of AuNS-based DM biosensors
Commercializing AuNS-based DM biosensors involves navigating several hurdles, including regulatory challenges, manufacturing scalability, cost-effectiveness, and user adaptation (Kumar and Mahajan, 2024). Accordingly, proving the safety and biocompatibility of the biosensor requires comprehensive studies to ensure that AuNS-based biosensors are safe (Lu et al., 2023). Potential toxicity, long-term stability, and environmental impact need thorough evaluation (Lu et al., 2023). In this context, obtaining approval from regulatory authorities could be stringent and time-consuming (Marimuthu et al., 2024). It usually involves extensive preclinical and clinical testing, quality control, and documentation (Marimuthu et al., 2024). Additionally, defining exclusive and universal standardization protocols for testing and validating the performance, safety, and quality control of heterogeneous AuNS-based biosensors can complicate regulatory approval (Marimuthu et al., 2024). Furthermore, manufacturing with consistent quality and performance at a large scale is challenging (Kumalasari et al., 2024). Variability in AuNS size, shape, and surface functionalization can affect biosensor translation to large-scale production (Chakraborty et al., 2024). Moreover, integration with smart devices in a reproducible and scalable manner requires advanced manufacturing techniques and robust quality control processes, which can lead to high production costs (Ghobashy et al., 2024). To address this challenge, collaborative efforts are needed between researchers, manufacturers, and regulatory bodies to help streamline the development and approval process (Ghobashy et al., 2024). This includes advancements in integration, automation, chemical synthesis, and process optimization to reduce costs (Zou et al., 2024). In summary, while AuNS-based biosensors hold significant promise for improving diabetes management, addressing these hurdles is critical for successful commercialization. Through collaborative efforts, innovative manufacturing, and cost-reduction strategies, these challenges can be overcome (Kumar and Mahajan, 2024).
5 Future perspective and emerging trends in AuNS-based DM biosensors
Emerging trends in the application of AuNSs are one of the most successful examples of biosensor innovations for DM detection, offering exceptional biocompatibility, stability, and conductivity, paving the way for significant breakthroughs in POC (Haider et al.). Currently, AuNSs enhance the sensitivity and precision of glucose monitoring devices, enabling real-time, non-invasive glucose detection with higher accuracy (Arafa et al., 2024). Their ability to operate in various environmental conditions without the limitations associated with enzyme degradation makes them ideal for continuous glucose monitoring systems in non-enzymatic biosensors (Tehrani et al., 2024). Additionally, they enhance the sensitivity and specificity of biosensors for other DM biomarkers, facilitating real-time, non-invasive, and multi-detection DM monitoring through smart devices (Kim et al., 2024). As an emerging trend, the integration of AuNSs with advanced technologies like artificial intelligence and machine learning can improve data analysis and predictive capabilities (Ahmad and Muhmood, 2024; Darwish et al., 2024; Eswaran et al., 2024; Gupta et al., 2024; Zhou et al., 2024). Future perspectives also include the development of hybrid biosensors combining nanomaterials with other nano- and micro-structures to simultaneously monitor a broader range of biomarkers and the release of a drug in a closed loop to improve the overall management of DM (Wang et al., 2024) (Figure 2). This offers a more comprehensive health assessment and personalized treatment strategies in the future.
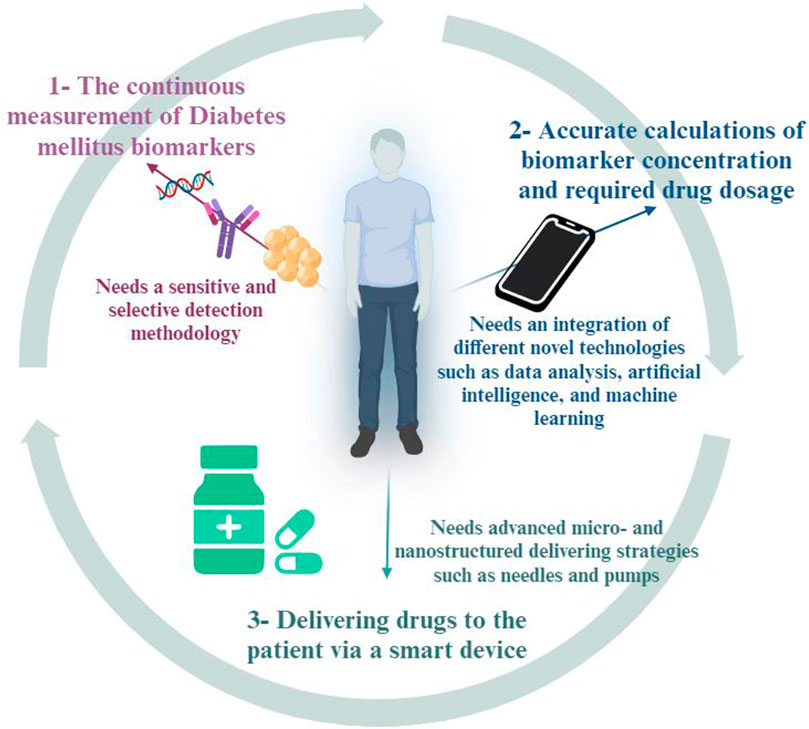
Figure 2. Closed loop structures can improve the overall management of diabetes mellitus (DM) by providing simultaneous biomarker detection and drug release.
6 Discussion
This study aimed to shed light on the recent advances in Au-nanostructured-based biosensors in detecting glucose and other diabetes biomarkers, such as HbA1c, GA insulin, IAA, and GADA. This is imperative because DM has no certain cure to date, and a real-time measurement of the biomarkers for proper management of the disease can lower the risk of further complications. Previous studies showed that AuNSs exhibited excellent properties, including high electrical conductivity, a large specific surface area, relatively low cost of synthesis, and high biocompatibility. Thus, they are suitable options in the construction of biosensors for DM monitoring. AuNSs are valuable in biosensor construction due to their established low electrical resistivity and wide electrochemical potential window. In addition, they can enhance the sensitivity and stability of biosensors through properties such as intrinsic specific molecule recognition capacity, signal transduction amplification in different methods, fast electron transfer, and the ability to stabilize structures of GOx and/or antibodies on electrodes. They also can serve as fluorescent and colorimetric materials, benefiting from their excellent optical features based on an aggregation/dispersion switch.
The explanation for the wide applications of the Au-nanostructures in DM biosensor construction is their strong properties, such as chemical stability and consistent morphology and size, while detecting various biomarkers. Another important characteristic is the ease of functionalization of biosensors with biomaterials such as GOx and antibodies, which can increase the efficiency, sensitivity, and specificity of the biosensor. The high surface-to-volume ratio of the Au nanostructures allows for the attachment of many biomaterials and chemicals. Outstanding electrical conductivity enables a reliable signal response for various biomarkers in different biological matrices. Last but not least, good biocompatibility, in addition to affordable price warranty the mass production of the DM biosensors. The designed biosensors based on biocompatibility and stability will be safe for medical applications that contact body fluids. These properties are crucial for designing stable, cost-effective, efficient, and fast DM biosensors, which are vital in DM monitoring.
Here, we emphasized that using AuNSs accompanied by other nanomaterials and biological agents can improve the most influential aspects of successful DM biosensors, namely, sensitivity, specificity, assay time, and stability. This work covers AuNS applications in simple and easy-to use detection of many different DM biomarkers. Researchers are attempting to discover possible transformations to achieve stability and high sensitivity and selectivity. Substantial advances are expected. However, the approaches described in this review are based on experimental research that has as yet no significant commercialized application. Further consideration is required to translate these technologies into routine and functional biosensing devices. Another vital concern is that the existing glucose biosensors are not continuous, wearable, or implantable equipment. Developing non-invasive, continuous, and wearable biosensors can eliminate the discomfort associated with finger-prick tests, improving patient compliance and providing comprehensive DM management throughout the day. Meanwhile, challenges such as regulatory hurdles, scalability, cost, and user adaptation must be addressed. Another limitation of this work is the exclusion of the fourth-generation category of reagent-less glucose biosensors incorporating AuNSs. In conclusion, AuNSs, with their unique properties, can greatly improve the ability to detect DM biomarkers in biosensors. In this context, they are valuable nanomaterials for DM diagnosis, but further research and development are needed.
Author contributions
TJ-T: investigation, methodology, and writing–original draft. SK: conceptualization, resources, supervision, validation, and writing–review and editing. KO: conceptualization, resources, supervision, validation, and writing–review and editing. HS: funding acquisition, resources, supervision, validation, visualization, and writing–review and editing.
Funding
The author(s) declare that financial support was received for the research, authorship, and/or publication of this article. HS was supported by the Swedish Research Council.
Acknowledgments
All authors gratefully acknowledge support from the Endocrinology and Metabolism Research Institute of Tehran University of Medical Sciences in Iran. Additionally, we want to extend our special gratitude to Uppsala University for its valuable assistance and guidelines.
Conflict of interest
The authors declare that the research was conducted in the absence of any commercial or financial relationships that could be construed as a potential conflict of interest.
Publisher’s note
All claims expressed in this article are solely those of the authors and do not necessarily represent those of their affiliated organizations, or those of the publisher, the editors, and the reviewers. Any product that may be evaluated in this article, or claim that may be made by its manufacturer, is not guaranteed or endorsed by the publisher.
References
Abraham, M. K., Madanan, A. S., Varghese, S., Rs, L., Shkhair, A. I., George, S., et al. (2024). Fluorescent enzymatic sensor based glucose oxidase modified bovine serum albumin-gold nanoclusters for detection of glucose. ChemPlusChem 89, e202300601. doi:10.1002/cplu.202300601
Ahmad, F., and Muhmood, T. (2024). Clinical translation of nanomedicine with integrated digital medicine and machine learning interventions. Colloids Surfaces B Biointerfaces 241, 114041. doi:10.1016/j.colsurfb.2024.114041
Ahmadi, A., Kabiri, S., and Omidfar, K. (2020). Advances in HbA1c biosensor development based on field effect transistors: a review. IEEE Sensors J. 20 (16), 1–21. doi:10.1109/jsen.2020.2987836
Ahmadi, A., Khoshfetrat, S. M., Kabiri, S., Dorraji, P. S., Larijani, B., and Omidfar, K. (2021). Electrochemiluminescence paper-based screen-printed electrode for HbA1c detection using two-dimensional zirconium metal-organic framework/Fe3O4 nanosheet composites decorated with Au nanoclusters. Microchim. Acta 188 (9), 296. doi:10.1007/s00604-021-04959-y
Alam, S., Hasan, M. K., Neaz, S., Hussain, N., Hossain, M. F., and Rahman, T. (2021). Diabetes Mellitus: insights from epidemiology, biochemistry, risk factors, diagnosis, complications and comprehensive management. Diabetology 2 (2), 36–50. doi:10.3390/diabetology2020004
Altintas, Z. (2017). Biosensors and nanotechnology: applications in health care diagnostics. John Wiley and Sons.
Arafa, F. N., Fahmy, H. M., Elshafiey, M., and Elshenawy, M. A. (2024) “Nanostructured highly sensitive and selective electrochemical sensors and their applications,” in Handbook of nanosensors: materials and technological applications. Springer, 1529–1575.
Association, A. D. (2014). Diagnosis and classification of diabetes mellitus. Diabetes care 37 (Suppl. ment_1), S81–S90. doi:10.2337/dc14-s081
Boonprasert, K., Tharavanij, T., Pechyen, C., Ponsanti, K., Tangnorawich, B., Viyanant, V., et al. (2023). Validation of an electrochemical sensor based on gold nanoparticles as a point-of-care test for quantitative determination of glycated hemoglobin. PLoS One 18 (6), e0276949. doi:10.1371/journal.pone.0276949
Chakraborty, S., Mal, S., Halder, A., Das, S., Sen, K. K., Mukherjee, A., et al. (2024). Nano-dimensional gold synthesis for biomedical applications: upscaling and challenges. Part. Sci. Technol. 42 (1), 145–163. doi:10.1080/02726351.2023.2211954
Cho, I.-H., Kim, D. H., and Park, S. (2020). Electrochemical biosensors: perspective on functional nanomaterials for on-site analysis. Biomaterials Res. 24 (1), 6–12. doi:10.1186/s40824-019-0181-y
Cui, X., Li, J., Li, Y., Liu, M., Qiao, J., Wang, D., et al. (2022). Detection of glucose in diabetic tears by using gold nanoparticles and MXene composite surface-enhanced Raman scattering substrates. Spectrochimica Acta Part A Mol. Biomol. Spectrosc. 266, 120432. doi:10.1016/j.saa.2021.120432
Dahan, K. A., Li, Y., Xu, J., and Kan, C. (2023). Recent progress of gold nanostructures and their applications. Phys. Chem. Chem. Phys. 25 (28), 18545–18576. doi:10.1039/d3cp01549a
Damaskos, C., Garmpis, N., Kollia, P., Mitsiopoulos, G., Barlampa, D., Drosos, A., et al. (2020). Assessing cardiovascular risk in patients with diabetes: an update. Curr. Cardiol. Rev. 16 (4), 266–274. doi:10.2174/1573403x15666191111123622
Darwish, M. A., Abd-Elaziem, W., Elsheikh, A., and Zayed, A. A. (2024). Advancements in nanomaterials for nanosensors: a comprehensive review. Nanoscale Adv. 6, 4015–4046. doi:10.1039/d4na00214h
Deepa, K., Sridhar, A., and Panda, T. (2023). Biogenic gold nanoparticles: current applications and future prospects. J. Clust. Sci. 34 (3), 1163–1183. doi:10.1007/s10876-022-02304-8
Demir, E., Kırboga, K. K., and Işık, M. (2024) “An overview of stability and lifetime of electrochemical biosensors,” in Novel nanostructured materials for electrochemical bio-sensing applications, 129–158.
Dervisevic, M., Alba, M., Esser, L., Tabassum, N., Prieto-Simon, B., and Voelcker, N. H. (2021). Silicon micropillar array-based wearable sweat glucose sensor. ACS Appl. Mater. and Interfaces 14 (1), 2401–2410. doi:10.1021/acsami.1c22383
Devi, R., Chauhan, A., Yadav, S., Kumar, P., and Pundir, C. (2023). Development of aptamer based colorimetric assay for whole blood glycated hemoglobin (HbA1c) estimation using gold nanoparticles. Biosens. Bioelectron. X 13, 100304. doi:10.1016/j.biosx.2023.100304
Durr, J. A., Hoffman, W. H., Sklar, A. H., El Gammal, T., and Steinhart, C. M. (1992). Correlates of brain edema in uncontrolled IDDM. Diabetes 41 (5), 627–632. doi:10.2337/diab.41.5.627
El-Safty, S., and Shenashen, M. (2020). Nanoscale dynamic chemical, biological sensor material designs for control monitoring and early detection of advanced diseases. Mater. Today Bio 5, 100044. doi:10.1016/j.mtbio.2020.100044
Eswaran, U., Eswaran, V., Murali, K., and Eswaran, V. (2024). Elevating Healthcare: the synergy of AI and biosensors in disease management. i-Manager's J. Artif. Intell. and Mach. Learn. (JAIM) 2 (1), 73. doi:10.26634/jaim.2.1.20190
Farajpour, N., Deivanayagam, R., Phakatkar, A., Narayanan, S., Shahbazian-Yassar, R., and Shokuhfar, T. (2020). A novel antimicrobial electrochemical glucose biosensor based on silver–Prussian blue-modified TiO2 nanotube arrays. Med. Devices and Sensors 3 (2), e10061. doi:10.1002/mds3.10061
Farrokhnia, M., Amoabediny, G., Ebrahimi, M., Ganjali, M., and Arjmand, M. (2022). Ultrasensitive early detection of insulin antibody employing novel electrochemical nano-biosensor based on controllable electro-fabrication process. Talanta 238, 122947. doi:10.1016/j.talanta.2021.122947
Frier, B. M. (2014). Hypoglycaemia in diabetes mellitus: epidemiology and clinical implications. Nat. Rev. Endocrinol. 10 (12), 711–722. doi:10.1038/nrendo.2014.170
Ghobashy, M. M., Alkhursani, S. A., Alqahtani, H. A., El-damhougy, T. K., and Madani, M. (2024). Gold nanoparticles in microelectronics advancements and biomedical applications. Mater. Sci. Eng. B 301, 117191. doi:10.1016/j.mseb.2024.117191
Golsanamlou, Z., Mahmoudpour, M., Soleymani, J., and Jouyban, A. (2023). Applications of advanced materials for non-enzymatic glucose monitoring: from invasive to the wearable device. Crit. Rev. Anal. Chem. 53 (5), 1116–1131. doi:10.1080/10408347.2021.2008227
Gupta, S., Sharma, N., Arora, S., and Verma, S. (2024). Diabetes: a review of its pathophysiology, and advanced methods of mitigation. Curr. Med. Res. Opin. 40 (5), 773–780. doi:10.1080/03007995.2024.2333440
Haider, S., Saira, F., Siddiq, A., Vishnu, D. S. M., Al Sibani, M., Naureen, Z., et al. “Introduction to nanomaterials for point of care diagnostics: technologies and applications,” in Nanomaterials and point of care technologies. CRC Press, 1–24.
Hu, Y., Chatzilakou, E., Pan, Z., Traverso, G., and Yetisen, A. K. (2024). Microneedle sensors for point-of-care diagnostics. Adv. Sci. 11, 2306560. doi:10.1002/advs.202306560
Jadhav, M. R., Wankhede, P., Srivastava, S., Bhargaw, H. N., and Singh, S. (2023). Breath-based biosensors and system development for noninvasive detection of diabetes: a review. Diabetes and Metabolic Syndrome Clin. Res. and Rev. 18, 102931. doi:10.1016/j.dsx.2023.102931
Jang, J. W., Kim, H., Kim, I., Lee, S. W., Jung, H. G., Hwang, K. S., et al. (2022). Surface functionalization of enzyme-coronated gold nanoparticles with an erythrocyte membrane for highly selective glucose assays. Anal. Chem. 94 (17), 6473–6481. doi:10.1021/acs.analchem.1c04541
Jitendra, D., Sharma, D. J., Dutta, D. S., Batra, D. J., Dey, D. S., and Rai, D. R. (2024). An in-depth analysis of anti-diabetic medication prescribing patterns, utilization trends, and patient compliance: a cross-sectional study at santosh medical college and hospital, Delhi NCR. Korean J. Physiology Pharmacol. 28 (1), 133–139. doi:10.25463/kjpp.28.1.2024.1
Katsarou, A., Gudbjörnsdottir, S., Rawshani, A., Dabelea, D., Bonifacio, E., Anderson, B. J., et al. (2017). Type 1 diabetes mellitus. Nat. Rev. Dis. Prim. 3 (1), 17016–17017. doi:10.1038/nrdp.2017.16
Khalil, S. H. A., Deeb, HMAE, Ajang, M. O. D., Osman, N. A., and Amin, N. G. (2023). Impact of diabetic peripheral neuropathy on gait abnormalities in patients with type 2 diabetes mellitus. Diabetol. Int. 15, 58–66. doi:10.1007/s13340-023-00652-y
Khan, M. A. B., Hashim, M. J., King, J. K., Govender, R. D., Mustafa, H., and Al Kaabi, J. (2020). Epidemiology of type 2 diabetes–global burden of disease and forecasted trends. J. Epidemiol. Glob. health 10 (1), 107. doi:10.2991/jegh.k.191028.001
Khor, S. M., Choi, J., Won, P., and Ko, S. H. (2022). Challenges and strategies in developing an enzymatic wearable sweat glucose biosensor as a practical point-of-care monitoring tool for type II diabetes. Nanomaterials 12 (2), 221. doi:10.3390/nano12020221
Khumngern, S., Jirakunakorn, R., Thavarungkul, P., Kanatharana, P., and Numnuam, A. (2021). A highly sensitive flow injection amperometric glucose biosensor using a gold nanoparticles/polytyramine/Prussian blue modified screen-printed carbon electrode. Bioelectrochemistry 138, 107718. doi:10.1016/j.bioelechem.2020.107718
Kim, T. Y., De, R., Choi, I., Kim, H., and Hahn, S. K. (2024). Multifunctional nanomaterials for smart wearable diabetic healthcare devices. Biomaterials 310, 122630. doi:10.1016/j.biomaterials.2024.122630
Kirk, J. K., and Stegner, J. (2010). Self-monitoring of blood glucose: practical aspects. J. diabetes Sci. Technol. 4 (2), 435–439. doi:10.1177/193229681000400225
Kumalasari, M. R., Alfanaar, R., and Andreani, A. S. (2024). Gold nanoparticles (AuNPs): a versatile material for biosensor application. Talanta Open 9, 100327. doi:10.1016/j.talo.2024.100327
Kumar, A., Purohit, B., Maurya, P. K., Pandey, L. M., and Chandra, P. (2019). Engineered nanomaterial assisted signal-amplification strategies for enhancing analytical performance of electrochemical biosensors. Electroanalysis 31 (9), 1615–1629. doi:10.1002/elan.201900216
Kumar, P. P. P., and Mahajan, R. (2024). Gold polymer nanomaterials: a promising approach for enhanced biomolecular imaging. Nanotheranostics 8 (1), 64–89. doi:10.7150/ntno.89087
Laha, S., Rajput, A., Laha, S. S., and Jadhav, R. (2022). A concise and systematic review on non-invasive glucose monitoring for potential diabetes management. Biosensors 12 (11), 965. doi:10.3390/bios12110965
Li, G., Hao, J., Li, W., Ma, F., Ma, T., Gao, W., et al. (2021). Integrating highly porous and flexible au hydrogels with soft-mems technologies for high-performance wearable biosensing. Anal. Chem. 93 (42), 14068–14075. doi:10.1021/acs.analchem.1c01581
Li, Y., and Chen, Y. (2023). Review of noninvasive continuous glucose monitoring in diabetics. ACS sensors 8 (10), 3659–3679. doi:10.1021/acssensors.3c01538
Lipińska, W., Grochowska, K., and Siuzdak, K. (2021). Enzyme immobilization on gold nanoparticles for electrochemical glucose biosensors. Nanomaterials 11 (5), 1156. doi:10.3390/nano11051156
Liu, J., Zhu, B., Dong, H., Zhang, Y., Xu, M., Travas-Sejdic, J., et al. (2022b). A novel electrochemical insulin aptasensor: from glassy carbon electrodes to disposable, single-use laser-scribed graphene electrodes. Bioelectrochemistry 143, 107995. doi:10.1016/j.bioelechem.2021.107995
Liu, S., Shen, Z., Deng, L., and Liu, G. (2022c). Smartphone assisted portable biochip for non-invasive simultaneous monitoring of glucose and insulin towards precise diagnosis of prediabetes/diabetes. Biosens. and Bioelectron. 209, 114251. doi:10.1016/j.bios.2022.114251
Liu, Y., Luo, X., Yu, Q., Ye, L., Yang, L., and Cui, Y. (2022a). Review of point-of-care platforms for diabetes:(1) sensing. Sensors Actuators Rep. 4, 100113. doi:10.1016/j.snr.2022.100113
Lu, T., Ji, S., Jin, W., Yang, Q., Luo, Q., and Ren, T.-L. (2023). Biocompatible and long-term monitoring strategies of wearable, ingestible and implantable biosensors: reform the next generation healthcare. Sensors 23 (6), 2991. doi:10.3390/s23062991
Macgilchrist, C., Paul, L., Ellis, B., Howe, T. E., Kennon, B., and Godwin, J. (2010). Lower-limb risk factors for falls in people with diabetes mellitus. Diabet. Med. 27 (2), 162–168. doi:10.1111/j.1464-5491.2009.02914.x
Mahobiya, S. K., Balayan, S., Chauhan, N., Rosario, W., Kuchhal, N. K., Islam, S., et al. (2023). Fabricating a rapid and low-cost electrochemical biosensor with imprints of glycated albumin molecules to detect diabetes using bimetallic Au-Pt nanoparticles on μSPE. Appl. Surf. Sci. Adv. 16, 100425. doi:10.1016/j.apsadv.2023.100425
Malik, S., Singh, J., Goyat, R., Saharan, Y., Chaudhry, V., Umar, A., et al. (2023). Nanomaterials-based biosensor and their applications: a review. Heliyon 9, e19929. doi:10.1016/j.heliyon.2023.e19929
Mandali, P. K., Prabakaran, A., Annadurai, K., and Krishnan, U. M. (2023). Trends in quantification of HbA1c using electrochemical and point-of-care analyzers. Sensors 23 (4), 1901. doi:10.3390/s23041901
Mansour, M., Darweesh, M. S., and Soltan, A. (2024). Wearable devices for glucose monitoring: a review of state-of-the-art technologies and emerging trends. Alexandria Eng. J. 89, 224–243. doi:10.1016/j.aej.2024.01.021
Marimuthu, M., Kanagaraj, R., Velayutham, R., Joseph, J., and Veerapandian, M. (2024). Commercialization and safety aspects of biosensing technologies. Health and environmental applications of biosensing technologies. Elsevier, 425–438.
Mohammadpour-Haratbar, A., Mohammadpour-Haratbar, S., Zare, Y., Rhee, K. Y., and Park, S.-J. (2022). A review on non-enzymatic electrochemical biosensors of glucose using carbon nanofiber nanocomposites. Biosensors 12 (11), 1004. doi:10.3390/bios12111004
Naresh, V., and Lee, N. (2021). A review on biosensors and recent development of nanostructured materials-enabled biosensors. Sensors 21 (4), 1109. doi:10.3390/s21041109
Ngernpimai, S., Puangmali, T., Kopwitthaya, A., Tippayawat, P., Chompoosor, A., and Teerasong, S. (2024a). Enhanced stability of gold nanoparticles with thioalkylated carboxyl-terminated ligands for applications in biosensing. ACS Appl. Nano Mater. 7 (11), 13124–13133. doi:10.1021/acsanm.4c01631
Ngernpimai, S., Puangmali, T., Kopwitthaya, A., Tippayawat, P., Chompoosor, A., and Teerasong, S. (2024b). Enhanced stability of gold nanoparticles with thioalkylated carboxyl-terminated ligands for applications in biosensing. ACS Appl. Nano Mater. 7, 13124–13133. doi:10.1021/acsanm.4c01631
Oliveira, B. B., Ferreira, D., Fernandes, A. R., and Baptista, P. V. (2023). Engineering gold nanoparticles for molecular diagnostics and biosensing. Wiley Interdiscip. Rev. Nanomedicine Nanobiotechnology. 15 (1), e1836. doi:10.1002/wnan.1836
Pan, Z., Yang, J., Song, W., Luo, P., Zou, J., Peng, J., et al. (2021). Au@ Ag nanoparticle sensor for sensitive and rapid detection of glucose. New J. Chem. 45 (6), 3059–3066. doi:10.1039/d0nj04489j
Pasquier, F., Boulogne, A., Leys, D., and Fontaine, P. (2006). Diabetes mellitus and dementia. Diabetes and Metabolism 32 (5), 403–414. doi:10.1016/s1262-3636(07)70298-7
Patil, T., Gambhir, R., Vibhute, A., and Tiwari, A. P. (2023). Gold nanoparticles: synthesis methods, functionalization and biological applications. J. Clust. Sci. 34 (2), 705–725. doi:10.1007/s10876-022-02287-6
Patra, S., Sahu, K. M., Reddy, A. A., and Swain, S. K. (2024). Polymer and biopolymer based nanocomposites for glucose sensing. Int. J. Polym. Mater. Polym. Biomaterials 73 (6), 490–521. doi:10.1080/00914037.2023.2175824
Pour, S. R. S., Calabria, D., Emamiamin, A., Lazzarini, E., Pace, A., Guardigli, M., et al. (2023). Electrochemical vs. optical biosensors for point-of-care applications: a critical Review. Chemosensors 11 (10), 546. doi:10.3390/chemosensors11100546
Promsuwan, K., Soleh, A., Samoson, K., Saisahas, K., Wangchuk, S., Saichanapan, J., et al. (2023). Novel biosensor platform for glucose monitoring via smartphone based on battery-less NFC potentiostat. Talanta 256, 124266. doi:10.1016/j.talanta.2023.124266
Psoma, S. D., and Kanthou, C. (2023). Wearable insulin biosensors for diabetes management: advances and challenges. Biosensors 13 (7), 719. doi:10.3390/bios13070719
Pullano, S. A., Greco, M., Bianco, M. G., Foti, D., Brunetti, A., and Fiorillo, A. S. (2022). Glucose biosensors in clinical practice: principles, limits and perspectives of currently used devices. Theranostics 12 (2), 493–511. doi:10.7150/thno.64035
Reddy, V. S., Agarwal, B., Ye, Z., Zhang, C., Roy, K., Chinnappan, A., et al. (2022). Recent advancement in biofluid-based glucose sensors using invasive, minimally invasive, and non-invasive technologies: a review. Nanomaterials 12 (7), 1082. doi:10.3390/nano12071082
Reutens, A. T., and Atkins, R. C. (2011). Epidemiology of diabetic nephropathy. Diabetes Kidney 170, 1–7. doi:10.1159/000324934
Saeedi, P., Petersohn, I., Salpea, P., Malanda, B., Karuranga, S., Unwin, N., et al. (2019). Global and regional diabetes prevalence estimates for 2019 and projections for 2030 and 2045: results from the international diabetes federation diabetes atlas, 9th edition. Diabetes Res. Clin. Pract. 157, 107843. doi:10.1016/j.diabres.2019.107843
Safarkhani, M., Aldhaher, A., Heidari, G., Zare, E. N., Warkiani, M. E., Akhavan, O., et al. (2023). Nanomaterial-assisted wearable glucose biosensors for noninvasive real-time monitoring: pioneering point-of-care and beyond. Nano Mater. Sci. 6, 263–283. doi:10.1016/j.nanoms.2023.11.009
Sakalauskiene, L., Brasiunas, B., Popov, A., Kausaite-Minkstimiene, A., and Ramanaviciene, A. (2023). The development of reagentless amperometric glucose biosensor based on gold nanostructures, prussian blue and glucose oxidase. Biosensors 13 (10), 942. doi:10.3390/bios13100942
Sakthivel, R., Prasanna, S. B., Tseng, C. L., Lin, L. Y., Duann, Y. F., He, J. H., et al. (2022). A sandwich-type electrochemical immunosensor for insulin detection based on Au-adhered Cu5Zn8 hollow porous carbon nanocubes and AuNP deposited nitrogen-doped holey graphene. Small 18 (35), 2202516. doi:10.1002/smll.202202516
Shah, S., Feher, M., McGovern, A., Sherlock, J., Whyte, M. B., Munro, N., et al. (2021). Diabetic retinopathy in newly diagnosed Type 2 diabetes mellitus: prevalence and predictors of progression; a national primary network study. Diabetes Res. Clin. Pract. 175, 108776. doi:10.1016/j.diabres.2021.108776
Sherwani, S. I., Khan, H. A., Ekhzaimy, A., Masood, A., and Sakharkar, M. K. (2016). Significance of HbA1c test in diagnosis and prognosis of diabetic patients. Biomark. insights 11, BMI.S38440. BMI. S38440. doi:10.4137/bmi.s38440
Shoaib, A., Darraj, A., Khan, M. E., Azmi, L., Alalwan, A., Alamri, O., et al. (2023). A nanotechnology-based approach to biosensor application in current diabetes management practices. Nanomaterials 13 (5), 867. doi:10.3390/nano13050867
Siciliano, G., Alsadig, A., Chiriacò, M. S., Turco, A., Foscarini, A., Ferrara, F., et al. (2023). Beyond traditional biosensors: recent advances in gold nanoparticles modified electrodes for biosensing applications. Talanta 268, 125280. doi:10.1016/j.talanta.2023.125280
Stratton, I., Kohner, E., Aldington, S., Turner, R., Holman, R., Manley, S., et al. (2001). UKPDS 50: risk factors for incidence and progression of retinopathy in Type II diabetes over 6 years from diagnosis. Diabetologia 44, 156–163. doi:10.1007/s001250051594
Tehrani, M. A., Ahmadi, S. H., Alimohammadi, S., Sasanpour, P., Batvani, N., Kazemi, S. H., et al. (2024). Continuous glucose monitoring using wearable non-enzymatic sensors in a physiological environment. Biosens. Bioelectron. X 18, 100482. doi:10.1016/j.biosx.2024.100482
Teymourian, H., Barfidokht, A., and Wang, J. (2020). Electrochemical glucose sensors in diabetes management: an updated review (2010–2020). Chem. Soc. Rev. 49 (21), 7671–7709. doi:10.1039/d0cs00304b
Theyagarajan, K., and Kim, Y.-J. (2023). Recent developments in the design and fabrication of electrochemical biosensors using functional materials and molecules. Biosensors 13 (4), 424. doi:10.3390/bios13040424
Turner, A. P., and Pickup, J. C. (1985). Diabetes mellitus: biosensors for research and management. Biosensors 1 (1), 85–115. doi:10.1016/0265-928x(85)85006-9
Vas, P. R., Edmonds, M., Kavarthapu, V., Rashid, H., Ahluwalia, R., Pankhurst, C., et al. (2018). The diabetic foot attack:tis too late to retreat. Int. J. Low. Extrem. wounds 17 (1), 7–13. doi:10.1177/1534734618755582
Wang, J., Liu, S., Meng, Z., Han, X. X., Cai, L., Xu, B., et al. (2023). Flexible SERS biosensor based on core-shell nanotags for sensitive and multiple detection of T1DM biomarkers. Anal. Chem. 95 (38), 14203–14208. doi:10.1021/acs.analchem.3c01791
Wang, T.-T., Huang, X.-F., Huang, H., Luo, P., and Qing, L.-S. (2022). Nanomaterial-based optical-and electrochemical-biosensors for urine glucose detection: a comprehensive review. Adv. Sens. Energy Mater. 1 (3), 100016. doi:10.1016/j.asems.2022.100016
Wang, X., Wang, Z., Xiao, M., Li, Z., and Zhu, Z. (2024). Advances in biomedical systems based on microneedles: design, fabrication, and application. Biomaterials Sci. 12, 530–563. doi:10.1039/d3bm01551c
Welch, E. C., Powell, J. M., Clevinger, T. B., Fairman, A. E., and Shukla, A. (2021). Advances in biosensors and diagnostic technologies using nanostructures and nanomaterials. Adv. Funct. Mater. 31 (44), 2104126. doi:10.1002/adfm.202104126
Wollina, U., Bujok, V., Fürll, C., and Hansel, G. (2020). Deep skin and soft tissue infection of the neck–first sign of unrecognized diabetes mellitus. Our Dermatol Online 11, 312–313. doi:10.7241/ourd.20203.21
Xu, J., Ma, J., Peng, Y., Cao, S., Zhang, S., and Pang, H. (2023). Applications of metal nanoparticles/metal-organic frameworks composites in sensing field. Chin. Chem. Lett. 34 (4), 107527. doi:10.1016/j.cclet.2022.05.041
Yan, L., Miao, K., Ma, P., Ma, X., Bi, R., and Chen, F. (2021). A feasible electrochemical biosensor for determination of glucose based on Prussian blue – enzyme aggregates cascade catalytic system. Bioelectrochemistry 141, 107838. doi:10.1016/j.bioelechem.2021.107838
Yazdanpanah, S., Rabiee, M., Tahriri, M., Abdolrahim, M., Rajab, A., Jazayeri, H. E., et al. (2017). Evaluation of glycated albumin (GA) and GA/HbA1c ratio for diagnosis of diabetes and glycemic control: a comprehensive review. Crit. Rev. Clin. laboratory Sci. 54 (4), 219–232. doi:10.1080/10408363.2017.1299684
Yi, J., and Xianyu, Y. (2022). Gold nanomaterials-implemented wearable sensors for healthcare applications. Adv. Funct. Mater. 32 (19), 2113012. doi:10.1002/adfm.202113012
Yoon, S.-J., and Kim, K.-i. (2019). Frailty and disability in diabetes. Ann. geriatric Med. Res. 23 (4), 165–169. doi:10.4235/agmr.19.0036
Yu, M., Li, Y.-T., Hu, Y., Tang, L., Yang, F., Lv, W.-L., et al. (2021). Gold nanostructure-programmed flexible electrochemical biosensor for detection of glucose and lactate in sweat. J. Electroanal. Chem. 882, 115029. doi:10.1016/j.jelechem.2021.115029
Zdarta, J., Kołodziejczak-Radzimska, A., Bachosz, K., Rybarczyk, A., Bilal, M., Iqbal, H. M., et al. (2023). Nanostructured supports for multienzyme co-immobilization for biotechnological applications: achievements, challenges and prospects. Adv. Colloid Interface Sci. 315, 102889. doi:10.1016/j.cis.2023.102889
Zhang, J., Liu, J., Su, H., Sun, F., Lu, Z., and Su, A. (2021). A wearable self-powered biosensor system integrated with diaper for detecting the urine glucose of diabetic patients. Sensors Actuators B Chem. 341, 130046. doi:10.1016/j.snb.2021.130046
Zhao, Y., Huang, J., Huang, Q., Tao, Y., Gu, R., Li, H.-Y., et al. (2023). Electrochemical biosensor employing PbS colloidal quantum dots/Au nanospheres-modified electrode for ultrasensitive glucose detection. Nano Res. 16 (3), 4085–4092. doi:10.1007/s12274-022-5138-0
Zhao, Y., Zhang, H., Li, Y., Wang, X., Zhao, L., Xu, J., et al. (2022). Glycated hemoglobin electrochemical immunosensor based on screen-printed electrode. Biosens. (Basel). 12 (10), 902. doi:10.3390/bios12100902
Zhou, Z., Shu, T., Sun, Y., Si, H., Peng, P., Su, L., et al. (2021). Luminescent wearable biosensors based on gold nanocluster networks for “turn-on” detection of Uric acid, glucose and alcohol in sweat. Biosens. Bioelectron. 192, 113530. doi:10.1016/j.bios.2021.113530
Zhou, Z., Xu, T., and Zhang, X. (2024). Empowerment of AI algorithms in biochemical sensors. TrAC Trends Anal. Chem. 173, 117613. doi:10.1016/j.trac.2024.117613
Keywords: diabetes mellitus, biosensor, gold nanostructures, biomarker, glucose detection
Citation: Jamshidnejad-Tosaramandani T, Kashanian S, Omidfar K and Schiöth HB (2024) Recent advances in gold nanostructure-based biosensors in detecting diabetes biomarkers. Front. Bioeng. Biotechnol. 12:1446355. doi: 10.3389/fbioe.2024.1446355
Received: 09 June 2024; Accepted: 08 August 2024;
Published: 17 September 2024.
Edited by:
Aylin Marz, Norfolk State University, United StatesReviewed by:
Mahdieh Darroudi, The University of Texas at Dallas, United StatesCongnyu Che, Sanofi Genzyme, United States
Copyright © 2024 Jamshidnejad-Tosaramandani, Kashanian, Omidfar and Schiöth. This is an open-access article distributed under the terms of the Creative Commons Attribution License (CC BY). The use, distribution or reproduction in other forums is permitted, provided the original author(s) and the copyright owner(s) are credited and that the original publication in this journal is cited, in accordance with accepted academic practice. No use, distribution or reproduction is permitted which does not comply with these terms.
*Correspondence: Soheila Kashanian, a2FzaGFuaWFuc0BnbWFpbC5jb20=; Helgi B. Schiöth, aGVsZ2kuc2NoaW90aEBuZXVyby51dS5zZQ==