- 1School of Grain Science and Technology, Jiangsu University of Science and Technology, Zhenjiang, China
- 2College of Forestry, Nanjing Forestry University, Nanjing, China
As an agricultural waste, crop straw enriched with a variety of nutrients is regarded as an important fertilizer resource. In the past, crop straw return-to-field played a key role in the sustainability of agricultural environment, but some problems, such as ammonia loss in ammoniation, low rate of straw decomposition, and high carbon footprint, attracted researchers’ attentions. In this paper, we propose three technical routes, including cyanobacteria-based ammonia assimilation, microorganisms-based crop straw pretreatment, and microalgae-based carbon capture, to address the aforementioned problems. Besides, challenges which may hinder the practical application of these technical routes as well as the potential solutions are discussed in detail. It is expected that this paper could provide new ideas to the practical application of crop straw return-to-field.
1 Introduction
As a large country with the highest population in the world, China puts a priority on crop production, which is accompanied by the yield of a huge amount of crop straws (Yan et al., 2019; Zhang et al., 2020; Wang et al., 2022). At present, the average annual straw production in China is higher than 1 billion tons, accounting for about one-third of the global straw production (He et al., 2022). In the past, crop straw, which was regarded as an agricultural waste, was often burned, resulting in serious atmospheric pollution and waste of resources (Singh et al., 2021). Recently, the return of crop straw to field as fertilizer is conceived and intensively studied as a promising technology to support the sustainable development of modern agriculture (Yan et al., 2019). Compared to the straw burning, return of crop straw to field has no detrimental effects on air quality and is able to improve soil fertility, maintain soil biodiversity, and promote plant growth (Ma et al., 2020a; Wang et al., 2021; Islam et al., 2022). For instance, after returning straw to fields, with the release of nutritional compositions in crop straw, soil organic carbon content increased significantly by 13.97% (Wang et al., 2020; Wang et al., 2021), while soil nitrogen (N) content and crop yield increased by 11% and 7%, respectively (Wang et al., 2015). Fast development of biotechnologies are promoting the wide application of crop straw return-to-field (Hans et al., 2019; Zhang B. et al., 2022). Owing to the positive effects of returning crop straw to fields on soil fertility, microbial diversity, and plant growth, Chinese government has made policies to support the return-to-field of crop straws (Yin et al., 2018).
Although a variety of methods and technologies were developed to promote the return-to-field of crop straw (Wang et al., 2013; Sun et al., 2014; Ma et al., 2020b), there are still some serious problems occurred in the return-to-field of crop straw (Supplementary Figure S1).
1.1 Waste of nitrogen resource in ammoniation process
As a process of treating crop straw with anhydrous ammonia, ammoniation, which has been widely applied before the return-to-field of crop straw, can improve the N content of straw and the nutritional values of straw-based fertilizer (Gordon and Chesson, 1983; Li et al., 2022). However, ammoniation of crop straw is accompanied with the emission of a huge amount of ammonia (Borhami et al., 1982; Gordon and Chesson, 1983). Gordon and Chesson (1983) reported that treatment of straw with anhydrous ammonia (40 kg t−1 straw dry matter) only increased the N content of straw by 13.9 g kg−1, suggesting that over 65% of ammonia was wasted in the ammoniation process. Hence, it is of importance to further improve the N conversion ratio of ammoniation process.
1.2 Low rate of straw decomposition
Optimization of tillage methods, in some cases, could not effectively accelerate the decomposition of crop straw (Yin et al., 2018). Wang et al. (2013) compared the effects of two tillage methods, including plowing tillage and no-tillage, on soil property in straw return-to-field experiment, discovering that the difference between these two methods in the improvement of soil total organic carbon (TOC) was not significant (Wang et al., 2013). Similar result was observed in the experiment which compared the effects of rotary tillage and plowing tillage on soil property (Zhu et al., 2014). Incomplete decomposition of crop straw can negatively impact the plant root penetration and the seedling growth (Su et al., 2016; Su et al., 2020).
1.3 Invasive emission of CO2
The increase of CO2 emission caused by the return-to-field of crop straw has not been fully addressed (Li H. et al., 2018; Ren et al., 2019; Ma et al., 2020a). Straw return-to-field inputs additional carbon sources to soil and increases microbial activity in the soil, resulting in significantly higher CO2 and methane emissions from the soil (Wang et al., 2018). It was reported that the rate of methane emission from rice fields increased by 210% due to straw return-to-field (Yan et al., 2005). In addition, Cui et al. (2017) pointed out that straw returning to the field has a significantly higher global warming potential (GWP) than conventional fertilization systems (Cui et al., 2017). Therefore, contribution of crop straw return-to-field to global warming has attracted researchers’ attentions (Hong et al., 2016; Liu et al., 2019; Ma et al., 2019).
Herein, we would like to focus on the recent progresses of employing algae and microorganisms to solve the aforementioned problems. In this work, a technical road-map for the sustainable development of straw return-to-field is presented and an in-depth discussion of the application of practically-feasible biotechnology is provided then. It is expected that this work can spur researchers to focus on the environmental problems occurred in the straw return-to-field and provide potential solutions to those problems for the development of eco-friendly agriculture.
2 Technical roadmap for the sustainable development of straw return-to-field
A road-map with three major technical routes is presented in Figure 1.
Firstly, to improve N conversion ratio of crop straw ammoniation, emitted NH3 can be collected and dissolved in water, which is further utilized for cyanobacteria cultivation. In cyanobacterial cells, assimilated ammonia is converted to algal protein (Lu et al., 2019). As the harvested algal biomass is added into soil as fertilizer, ammonia will be released back to soil with the decomposition of algal protein (Zou et al., 2021).
Secondly, microorganisms, particularly bacteria and fungi, are employed to pretreat crop straw. Extracellular enzymes secreted by those microorganisms can promote the decomposition of crops straw (Ji et al., 2018). With the assistance of enzymes-secreting microorganisms, the decomposition rate of crop straw can be improved. Accordingly, nutrients contained in crop straw can be utilized by plants through an efficient way.
Thirdly, microalgae are spread on soil as a potential strategy to attenuate the CO2 emission of return-to-field of crop straw. Microalgae on soil surface can capture CO2 via photosynthesis, creating a huge carbon sink (Lu et al., 2022; Lu and Xiao, 2022). With the photosynthesis of microalgae on soil surface, carbon footprint of crop straw return-to-field can be lowered.
The feasibility of aforementioned strategies is intensively studied. Firstly, cyanobacteria-based ammonia assimilation is applied in wastewater remediation to achieve the nitrogen recovery (Lu et al., 2017; Lu et al., 2019). Besides, cyanobacteria with high content of nitrogen have been proven to be a good resource for organic fertilizer (Acea, 2003; Abinandan et al., 2019). Secondly, mechanisms associated with the bacteria- or fungi-based crop straw decomposition have been intensively studied, demonstrating the possibility of using microorganisms to promote the crop straw return-to-field (Wood et al., 1986; Ji et al., 2018). Thirdly, microalgae have been intensively employed to reduce carbon emission of traditional factories. For example, some microalgal strains perform well in assimilating CO2 in flue gas, contributing to the construction of net-zero carbon factory (Cheah et al., 2015). Besides, mechanisms of algal photosynthesis and critical points enhancing the microalgae-based carbon fixation have been studied (Singh et al., 2016; Lu et al., 2018a; Petrova et al., 2020).
3 Integration of cyanobacteria-based ammonia absorption with ammoniation
3.1 Ammonia emission in ammoniation process
Ammoniation is conducted to generate ammonolysis reaction in crop straw by using ammonia-containing chemicals, such as liquid ammonia, urea, and ammonium bicarbonate (Yu et al., 2015; Doğan and Hayırlı, 2022). Through ammoniation, ester bonds between lignin and polysaccharide in crop straw can be damaged, making straw more decomposable in soil (Datsomor et al., 2022). Up to now, although key parameters, including temperature, moisture level, treatment period, and so on, have been optimized, ammonia emission in ammoniation process is still a serious challenge (Cloete and Kritzinger, 1984; Ma et al., 2020c). According to the study of Ma et al. (2020a) that used 5% urea (46% N content) for ammoniation, crude protein contents in rice straw (350 g) with ammoniation and without ammoniation were 9.27% and 5.79%, respectively (Ma et al., 2020c). If the nitrogen-to-protein conversion factor was set as 6.25, the addition of 5% urea (8.05 g N) in 350 g rice straw (3.24 g N) only increased the total N content of rice straw from 3.24 g to 5.19 g (Lu et al., 2015). In other words, 6.10 g N from urea was wasted in the ammoniation process while only 1.95 g N in urea was combined to rice straw (Ma et al., 2020c). Therefore, in this work, N conversion ratio of ammoniation was only 24.22% while about 75.78% of nitrogen in urea was wasted (Supplementary Figure S1).
Ammonia emission may dramatically increase the ammonia concentration in a closed environment, causing potential explosion under certain conditions (Li H. et al., 2018). Besides, ammonia released into atmosphere can be a promoter of the formation of haze (Ye et al., 2011). Hence, measures must be taken to attenuate the ammonia emission in ammoniation process. As shown in Figure 1, to reduce the ammonia emission in ammoniation process, a potential strategy of recovering the ammonia released from ammoniation process by growing cyanobacteria is proposed. In this strategy, ammonia released from ammoniation is collected to used as a nitrogen source of culture medium for cyanobacteria production. Then, cyanobacterial biomass enriched with nitrogen can be used as organic fertilizer to improve soil fertility.
3.2 Cyanobacteria-based ammonia absorption
Cyanobacteria are a group of blue-green algae with the great capacity of assimilating nitrogen for protein synthesis (Lu et al., 2019). In the metabolisms, nitrate in external environment must be converted to ammonia by nitrate reductase and nitrite reductase while ammonia can be directly assimilated by cyanobacterial cells (Li et al., 2016). Since ammonia in external environment can be efficiently used by cyanobacteria as a nitrogen source, cyanobacteria can be employed for ammonia removal. Previous studies have intensively cultured various species of cyanobacteria for ammonia recovery in different types of wastewater or culture media (Lu et al., 2019; Srimongkol et al., 2019). Cyanobacteria can survive in an ammonia-rich environment and perform well in ammonia removal (Supplementary Table S1). For example, in a synthetic wastewater containing about 206 mg/L ammonia, Spirulina platensis removed 80%–84% nitrogen. In water environment with low ammonia concentration, cyanobacteria could also efficiently fix ammonia (Wuang et al., 2016; Álvarez and Otero, 2020). Therefore, it is a practically-feasible method to use cyanobacteria for ammonia recovery.
Through glutamine synthetase-glutamine oxoglutarate aminotransferase (GS-GOGAT) pathway, absorbed ammonia can be converted to amino acids and protein by cyanobacteria (Lu et al., 2018a). Protein content in Spirulina sp., a typical species of cyanobacteria, could reach 59%–69% (Lu et al., 2019). If the nitrogen-to-protein conversion factor is set as 6.25, 1 kg cyanobacterial biomass (590–690 g protein) contains 94.4–110.4 g nitrogen, which is equal to 114.6–134.1 g ammonia. Accordingly, the cultivation of cyanobacteria can be regarded as an important process of the bio-conversion of ammonia to protein.
3.3 Utilization of cyanobacterial biomass as organic fertilizer
Protein-rich cyanobacterial biomass can be utilized as organic fertilizer, which can slowly release nutrients, including nitrogen, phosphorus, and organic carbon, to improve soil fertility (Malam Issa et al., 2006; Kumar et al., 2013). Compared to the direct use of ammonia solution as fertilizer, the use of cyanobacterial biomass as fertilizer has some advantages. Firstly, cyanobacteria-based fertilizer can release ammonia slowly with the gradual decomposition of cyanobacterial protein. Compared to chemical fertilizer, cyanobacteria-based fertilizer could release ammonia via a slow-release process (Lu and Xiao, 2022). The slow-releasing process of ammonia has not detrimental effects on crops but can continuously support the crop growth (Singh et al., 2019). Secondly, ammonia, which is slowly and continuously released from cyanobacterial biomass, can be assimilated by crops immediately. Under this situation, the loss of nitrogen resource caused by ammonia evaporation in fertilization process can be attenuated (Jhala et al., 2017; Lu et al., 2022).
As shown in Table 1, in the agricultural practice, with the addition of cyanobacteria in soil, soil fertility can be improved and the growth of plants can be enhanced (Abinandan et al., 2019). Firstly, due to the high content of protein in cyanobacteria, nitrogen content in soil can be improved after cyanobacteria inoculation (Acea, 2003). Besides, some cyanobacteria are able to fix atmospheric nitrogen during the fertilization process, further improving the nitrogen content in soil (Harding et al., 2018). The fixation of atmospheric nitrogen by cyanobacteria is another factor that contributes to the increase of nitrogen content in soil (Lu et al., 2022). Secondly, after cyanobacteria inoculation, microbial biomass carbon in soil can increase, contributing to the improvement of soil fertility (Karthikeyan et al., 2007; Song X. et al., 2021). Thirdly, cyanobacteria with filamentous cell structure and water retention ability can increase soil aggregate stability (Malam Issa et al., 2006). Previous studies have demonstrated the positive effects of cyanobacteria inoculation in soil on the growth of crops and vegetables (Karthikeyan et al., 2007; Kumar et al., 2013; Dash et al., 2016).
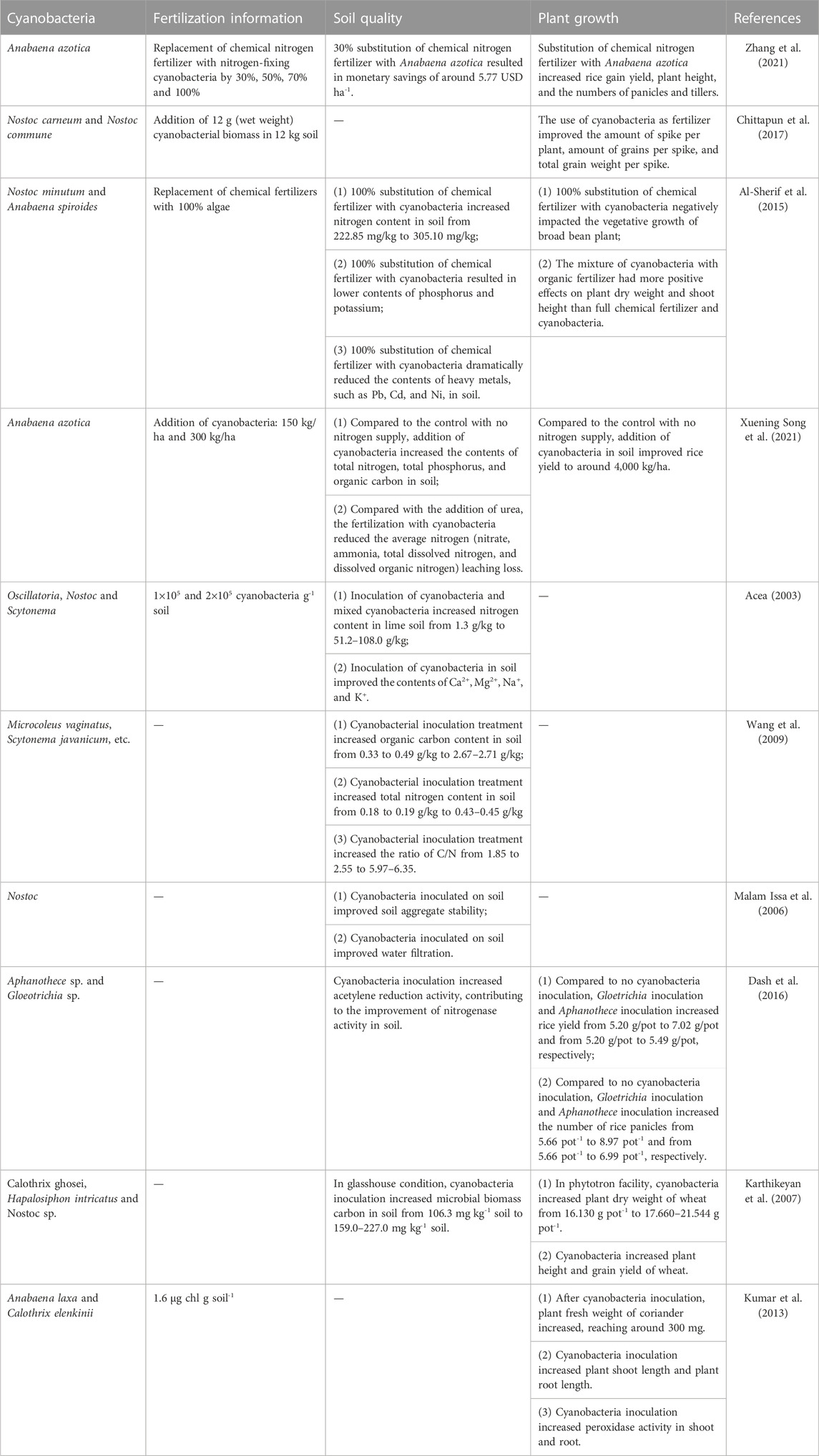
TABLE 1. Utilization of cyanobacteria as fertilizer to improve soil fertility and enhance plant growth.
4 Application of microorganisms based fermentation to pretreat crop straw
4.1 Decomposition process of crop straw
Zhang et al. (2014) analyzed 784 crop straw samples, including cotton stalk samples, wheat straw, rape stalk, rice straw, and corn stover, collected from different regions of China, discovering that crop straw contains some essential elements, including carbon, nitrogen, sulfur, phosphorus, and potassium (Zhang et al., 2014; Wang et al., 2020). It was observed that in nature, crop straw can be decomposed gradually by bacteria, fungi, and other microbes with the assistance of hemicellulase, cellulase and ligninase (Henriksen and Breland, 2002; Jin et al., 2022). For example, Ji et al. (2018) reported that Methanosarcina and Methanothrix as well as hydrogentrophic Methanocella could be regarded as the important archaeal taxa involved in rice straw degradation (Ji et al., 2018).
Decomposition of crop straw by microorganisms-based fermentation can be divided into two major steps (Guo et al., 2018; Gao et al., 2016). Firstly, microorganisms secret extracellular enzymes, particularly cellulase and ligninase, which can break the chemical structure of crop straw, converting high-molecular-weight organics to low-molecular-weight organics (Xiong et al., 2014; Harindintwali et al., 2020). Secondly, after the enzymes-driven crop straw decomposition, nutrients of crop straw could be assimilated by microorganisms (Zhao et al., 2019). In this step, some microbial strains may secret organic acids with low-molecular-weight. Both two steps could play a key role in the improvement of soil fertility. On one hand, extracellular enzymes secreted by microorganisms could promote the decomposition of crop straw and accelerate crop straw return-to-field. On the other hand, organic acids secreted by some microbial strains could increase the organic carbon content in soil (Zhang Y. et al., 2022).
4.2 Microorganisms-based fermentation for crop straw pretreatment
Baige Zhang et al. (2022) cultivated Trichoderma reesei to pretreat corn straw before return-to-field, discovering that the humic acid carbon content in fungi-treated corn straw was much higher than that in untreated corn straw (Zhang Y. et al., 2022). Fermented corn straw treated with T. reesei is more conducive to increasing the content of easily oxidizable organic carbon in soil than direct application of corn straw. In addition, some conditions, such as temperature, O2 concentration, and air humidity, which can determine the fermentation process, could be optimized to enhance the performance of crop straw pretreatment (Yu et al., 2019; Mengqi et al., 2021). Last but not the least, some studies focused on the emission of greenhouse gases (GHG) during the decomposition of crop straw in soil (Devêvre and Horwáth, 2000). In the real-world application, it is also important to take measures to reduce the GHG emission during the microorganisms-based crop straw pretreatment.
At present, although the research directly related to bacteria- or fungi-based crop straw pretreatment is very limited, there are some studies focusing on microorganisms screening and major components of crop straw. Firstly, in the past decades, various microbial strains, including Bacillus subtilis, Neocallimastix frontalis, N. frontalis, Pleurotus sp., T. reesei, and so on, with the ability of secreting extracellular cellulase and ligninase were discovered and isolated (Wood et al., 1986; Singh et al., 2013; de Paula et al., 2019; Rathnan and John, 2021). In the practical application, to enhance the performance of crop straw pretreatment, a mixture of microbial strains with different extracellular enzymes could be applied. Secondly, major components of crop straw have been intensively studied, providing guidelines for the screening of microorganisms (Tamaki and Mazza, 2010). For example, to promote the decomposition of crop straw with high contents of cellulose, microbial strains secreting extracellular cellulase should be selected for the pretreatment.
5 Reduction of the carbon footprint of straw return-to-field by microalgae
5.1 Carbon footprint of straw return-to-field
Due to the high content of lignin, cellulose, and hemicellulose in crop straw, the decomposition of straw in soil could release a large amount of carbon (Supplementary Table S2). Gan et al. (2011) reported that straw and root decomposition could emit 120 kg CO2-eq ha−1, suggesting that straw in soil can be a source of carbon emission (Gan et al., 2011). Wu et al. (2022) reported that carbon footprint of wheat cultivation supplied with straw and chemical fertilizer reached 1978.72 kg CO2-eq ha−1 and carbon efficiency was only 11.87% (Wu et al., 2022). In addition to carbon footprint of single crop production, carbon footprint of crops rotation could be improved by the utilization of straw-based fertilizer. Shi-hao Li et al. (2020) found that in rice-wheat rotation, annual CH4 emission increased by 5.4%–72.2% with the straw return-to-field and carbon emission (4,275–4,989 kg CO2-eq ha−1) also increased with increasing straw returning amounts (Li S. H. et al., 2020). Similar result confirming the positive correlation between straw and soil carbon footprint was also discovered by Xuening Song et al. (2021) that assessed the effects of straw retention and straw removal on carbon footprint of continuous corn cropping and corn-soybean rotation (Song Q. et al., 2021). Hence, straw return-to-field usually gives rise to GHG emissions from the soil and increase carbon footprint of agricultural activity (Dhaliwal et al., 2019).
5.2 Microalgae-based carbon assimilation in soil
Microalgae, which can efficiently assimilate atmospheric CO2 and organic carbon, are widely spread in the river, desert, lake, and ocean. It was reported that carbon content in Chlorella pyrenoidosa fell in a range of 47.00%–56.02% (Huang et al., 2015). If CO2 is the sole carbon source of microalgae, the accumulation of 1 kg microalgae biomass means that 1.477–1.761 kg CO2 has been assimilated. According to the study of Lu et al. (2018a), the maximum O2 productivity of 8 g microalgae on biofilm (200 cm2) could reach 249.44 mg h−1 (Lu et al., 2018b). In other words, 1 kg microalgae could assimilate CO2 at a maximum rate of 42.87 g h−1. Hence, microalgae culture can be regarded as an efficient way for carbon capture. Since microalgae perform well in carbon fixation and can be easily obtained, it may be a practically-feasible way to use microalgae to reduce carbon footprint in agricultural activity.
The reduction of carbon footprint of soil by living microalgae might be attributed to two mechanisms (Figure 1). Firstly, living microalgae on the surface of soil can capture atmospheric CO2 through photosynthesis. If the environmental factors, such as temperature, sunlight, and moisture, are suitable to the algal photosynthesis, microalgae on the surface of soil can be regarded as a carbon sink, which continuously convert atmospheric CO2 to biomass (Abinandan et al., 2019). In this case, microalgae could reduce the total carbon emission of soil by creating a carbon-negative micro-environment on the surface of soil. Secondly, microalgae could establish synergistic relations with soil bacteria and fungi for carbon utilization. Soil microorganisms, including microalgae, bacteria, and fungi, can form consortia, in which microbial cells not only interact physically, but also exchange nutrients (Perera et al., 2018). For example, CO2 released by soil bacteria and fungi can be immediately captured by microalgae in the consortia. In this way, carbon emission from the heterotrophic metabolisms of soil bacteria and fungi can be reduced to some extent.
In the practical application, some important issues merit the researchers’ attentions. Firstly, although the effects of alien microalgal species on soil environment have not been fully studied yet, potential threats of biological invasion caused by the use of alien microalgal species should be avoided (Korneva, 2014). Hence, native microalgal species, instead of alien microalgal species, should be isolated and cultured for carbon footprint reduction. Secondly, to improve the survival ratio of microalgae added in soil, soil properties, such as salinity, temperature fluctuation, moisture and so on, must be taken into consideration. For example, to reduce the carbon footprint of saline-alkali land, microalgal species with high tolerance to salt should be screened and then inoculated in soil (Qiao et al., 2015). Thirdly, to support microalgae growth in soil and promote carbon fixation, microalgae growth-promoting bacteria can be co-inoculated with microalgae in soil. It was discovered that some bacteria could promote the growth of microalgae by producing and secreting indole acetic acid (Dao et al., 2018). With the faster reproduction of microalgae in soil, a larger carbon sink can be created for carbon reduction.
6 Challenges and prospects
In practice, some challenges to these strategies are discussed and potential solutions are provided as well.
Firstly, it is not easy to improve ammonia assimilation ratio during cyanobacteria cultivation. Theoretically, cyanobacteria could efficiently assimilate dissolved ammonia in media. However, cyanobacteria growth could continuously increase the pH of media, disturbing the equilibrium of H+ and OH−. The pH of media with Spirulina cultivation could reach over 11, creating an alkaline environment (Pagels et al., 2019; Li H. et al., 2020). As a result, a high portion of ionized ammonium will be converted to unionized ammonia, causing ammonia emission. In the view of the present authors, to improve the ammonia assimilation ratio during cyanobacteria growth, pH of culture media should be strictly controlled to prevent the continuous increase of pH. For example, buffer can be added in culture media to attenuate the conversion of ionized ammonium to unionized ammonia during cyanobacteria growth. In addition, crop straw ammoniation and cyanobacteria cultivation can be performed simultaneously to ensure that ammonia released from ammoniation process can be immediately assimilated by cyanobacteria.
Secondly, in agricultural practice, due to the harsh environment, such as temperature fluctuation between day and night, microorganisms-based fermentation may be hindered. In addition, if microbial strains could not be preserved properly, their bio-activity may be negatively impacted. Therefore, measures must be taken to enhance the microorganisms-based crop straw pretreatment. Firstly, microbial strains should be preserved properly to prevent the decrease of microbial bio-activity and the degradation of microorganisms. Secondly, the optimal environment should be identified according to the biological characteristics of microorganisms. Then, the pretreatment of crop straw should be conducted under the optimal conditions to ensure that a higher ratio of nutrients in crop straw can be converted to low-molecular-weight nutrients. Thirdly, physical pretreatment or chemical pretreatment can be integrated with microorganisms-based crop straw pretreatment. For example, physical crushing could increase the total surface area of crop straw and enhance the interaction between microorganisms and crop straw.
Thirdly, production cost of microalgae must be further reduced to lower the total cost of the reduction of carbon footprint of crop straw return-to-field. Due to the high cost of culture media, artificial illumination, and biomass harvesting, the utilization of microalgae is seriously hindered in many sectors of agriculture and industry. Acien et al. (2012) reported that the unit production cost of microalgae biomass (dry weight) could reach 96 €/kg and the simplification of technology could reduce the unit production cost of microalgae biomass to 12.6 €/kg (Acien et al., 2012). In our view, some measures can be taken to lower the production cost of microalgae. Firstly, wastewater enriched with nutrients can be employed to cultivate microalgae (Xu et al., 2020; Zhong et al., 2021). Compared to artificial culture media, wastewater can be obtained at much lower cost, reducing the production cost of microalgae. Secondly, advanced technologies or equipment can be adopted to simplify the microalgae harvesting process. For example, compared to centrifugation, immobilized microalgae attached on biofilm can be harvested by using scrappers with much lower energy consumption and cost (Hu et al., 2021). Thirdly, photovoltaic technology can be employed to reduce the energy consumption caused by illumination. With the adoption of photovoltaic technology, solar energy can be stored in the form of electrical energy to provide illumination for microalgae growth at night (Zahedi, 2011). Thus, the energy consumption and cost of microalgae production can be reduced.
Author contributions
QL: Revision; YX: Draft writing; PW: Revision.
Conflict of interest
The authors declare that the research was conducted in the absence of any commercial or financial relationships that could be construed as a potential conflict of interest.
Publisher’s note
All claims expressed in this article are solely those of the authors and do not necessarily represent those of their affiliated organizations, or those of the publisher, the editors and the reviewers. Any product that may be evaluated in this article, or claim that may be made by its manufacturer, is not guaranteed or endorsed by the publisher.
Supplementary material
The Supplementary Material for this article can be found online at: https://www.frontiersin.org/articles/10.3389/fbioe.2023.1152778/full#supplementary-material
References
Abinandan, S., Subashchandrabose, S. R, Venkateswarlu, K., and Megharaj, M. (2019). Soil microalgae and cyanobacteria: The biotechnological potential in the maintenance of soil fertility and health. Crit. Rev. Biotechnol. 39, 981–998. doi:10.1080/07388551.2019.1654972
Acea, M. (2003). Cyanobacterial inoculation of heated soils: Effect on microorganisms of C and N cycles and on chemical composition in soil surface. Soil Biol. Biochem. 35, 513–524. doi:10.1016/s0038-0717(03)00005-1
Acien, F. G., Fernandez, J. M., Magan, J. J., and Molina, E. (2012). Production cost of a real microalgae production plant and strategies to reduce it. Biotechnol. Adv. 30, 1344–1353. doi:10.1016/j.biotechadv.2012.02.005
Al-Sherif, E. A., Abd El-Hameed, M. S., Mahmoud, M. A., and Ahmed, H. S. (2015). Use of cyanobacteria and organic fertilizer mixture as soil bioremediation. American-Eurasian J. Agric. Environ. Sci. 15, 794–799.
Álvarez, X., and Otero, A. (2020). Nutrient removal from the centrate of anaerobic digestion of high ammonium industrial wastewater by a semi-continuous culture of Arthrospira sp. and Nostoc sp. PCC 7413. J. Appl. Phycol. 32, 2785–2794. doi:10.1007/s10811-020-02175-4
Borhami, B., Sundstøl, F., and Garmo, T. (1982). Studies on ammonia-treated straw. II. Fixation of ammonia in treated straw by spraying with acids. Animal Feed Sci. Technol. 7, 53–59. doi:10.1016/0377-8401(82)90036-0
Cheah, W. Y., Show, P. L., Chang, J.-S., Ling, T. C., and Juan, J. C. (2015). Biosequestration of atmospheric CO2 and flue gas-containing CO2 by microalgae. Bioresour. Technol. 184, 190–201. doi:10.1016/j.biortech.2014.11.026
Chittapun, S., Limbipichai, S., Amnuaysin, N., Boonkerd, R., and Charoensook, M. (2017). Effects of using cyanobacteria and fertilizer on growth and yield of rice, pathum thani I: A pot experiment. J. Appl. Phycol. 30, 79–85. doi:10.1007/s10811-017-1138-y
Cloete, S., and Kritzinger, N. (1984). A laboratory assessment of various treatment conditions affecting the ammoniation of wheat straw by urea. 1. The effect of temperature, moisture level and treatment period. South Afr. J. Animal Sci. 14, 55–58.
Cui, Y.-f., Meng, J., Wang, Q.-x., Zhang, W.-m., Cheng, X.-y., and Chen, W.-f. (2017). Effects of straw and biochar addition on soil nitrogen, carbon, and super rice yield in cold waterlogged paddy soils of North China. J. Integr. Agric. 16, 1064–1074. doi:10.1016/s2095-3119(16)61578-2
Dao, G.-H., Wu, G.-X., Wang, X.-X., Zhang, T.-Y., Zhan, X.-M., and Hu, H.-Y. (2018). Enhanced microalgae growth through stimulated secretion of indole acetic acid by symbiotic bacteria. Algal Res. 33, 345–351. doi:10.1016/j.algal.2018.06.006
Dash, N., Kumar, A., Kaushik, M. S., and Singh, P. K. (2016). Cyanobacterial (unicellular and heterocystous) biofertilization to wetland rice influenced by nitrogenous agrochemical. J. Appl. Phycol. 28, 3343–3351. doi:10.1007/s10811-016-0871-y
Datsomor, O., Yan, Q., Wang, K., Mohamed, S., Opoku-Mensah, L., Zhao, G., et al. (2022). Effect of ammoniated and/or basidiomycete white-rot fungi treatment on rice straw proximate composition, cell wall component, and in vitro rumen fermentation characteristics. Fermentation 8, 228. doi:10.3390/fermentation8050228
de Paula, R. G., Antonieto, A. C. C., Nogueira, K. M. V., Ribeiro, L. F. C., Rocha, M. C., Malavazi, I., et al. (2019). Extracellular vesicles carry cellulases in the industrial fungus Trichoderma reesei. Biotechnol. Biofuels 12, 146. doi:10.1186/s13068-019-1487-7
Devêvre, O. C., and Horwáth, W. R. (2000). Decomposition of rice straw and microbial carbon use efficiency under different soil temperatures and moistures. Soil Biol. Biochem. 32, 1773–1785. doi:10.1016/s0038-0717(00)00096-1
Dhaliwal, S. S., Naresh, R. K., Gupta, R. K., Panwar, A. S., Mahajan, N. C., Singh, R., et al. (2019). Effect of tillage and straw return on carbon footprints, soil organic carbon fractions and soil microbial community in different textured soils under rice–wheat rotation: A review. Rev. Environ. Sci. Bio/Technology 19, 103–115. doi:10.1007/s11157-019-09520-1
Doğan, V., and Hayırlı, A. (2022). Efficacy of ammonization to eliminate common mycotoxins. Turkish J. Agriculture-Food Sci. Technol. 10, 1982–1986. doi:10.24925/turjaf.v10i10.1982-1986.5371
Gan, Y., Liang, C., Huang, G., Malhi, S. S., Brandt, S. A., and Katepa-Mupondwa, F. (2011). Carbon footprint of canola and mustard is a function of the rate of N fertilizer. Int. J. Life Cycle Assess. 17, 58–68. doi:10.1007/s11367-011-0337-z
Gao, H., Chen, X., Wei, J., Zhang, Y., Zhang, L., Chang, J., et al. (2016). Decomposition dynamics and changes in chemical composition of wheat straw residue under anaerobic and aerobic conditions. PloS one 11, e0158172. doi:10.1371/journal.pone.0158172
Gordon, A., and Chesson, A. (1983). The effect of prolonged storage on the digestibility and nitrogen content of ammonia-treated barley straw. Animal Feed Sci. Technol. 8, 147–153. doi:10.1016/0377-8401(83)90096-2
Guo, T., Zhang, Q., Ai, C., Liang, G., He, P., and Zhou, W. (2018). Nitrogen enrichment regulates straw decomposition and its associated microbial community in a double-rice cropping system. Sci. Rep. 8, 1847–1912. doi:10.1038/s41598-018-20293-5
Hans, M., Kumar, S., Chandel, A. K., and Polikarpov, I. (2019). A review on bioprocessing of paddy straw to ethanol using simultaneous saccharification and fermentation. Process Biochem. 85, 125–134. doi:10.1016/j.procbio.2019.06.019
Harding, K., Turk-Kubo, K. A., Sipler, R. E., Mills, M. M., Bronk, D. A., and Zehr, J. P. (2018). Symbiotic unicellular cyanobacteria fix nitrogen in the Arctic Ocean. Proc. Natl. Acad. Sci. 115, 13371–13375. doi:10.1073/pnas.1813658115
Harindintwali, J. D., Zhou, J., and Yu, X. (2020). Lignocellulosic crop residue composting by cellulolytic nitrogen-fixing bacteria: A novel tool for environmental sustainability. Sci. Total Environ. 715, 136912. doi:10.1016/j.scitotenv.2020.136912
He, Z., Yang, X., Xiang, J., Wu, Z., Shi, X., Gui, Y., et al. (2022). Does straw returning amended with straw decomposing microorganism inoculants increase the soil major nutrients in China’s farmlands? Agronomy 12, 890. doi:10.3390/agronomy12040890
Henriksen, T. M., and Breland, T. A. (2002). Carbon mineralization, fungal and bacterial growth, and enzyme activities as affected by contact between crop residues and soil. Biol. Fertil. Soils 35, 41–48. doi:10.1007/s00374-001-0438-0
Hong, J., Ren, L., Hong, J., and Xu, C. (2016). Environmental impact assessment of corn straw utilization in China. J. Clean. Prod. 112, 1700–1708. doi:10.1016/j.jclepro.2015.02.081
Hu, Y., Xiao, Y., Liao, K., Leng, Y., and Lu, Q. (2021). Development of microalgal biofilm for wastewater remediation: From mechanism to practical application. J. Chem. Technol. Biotechnol. 96, 2993–3008. doi:10.1002/jctb.6850
Huang, Y., Cheng, J., Lu, H., Huang, R., Zhou, J., and Cen, K. (2015). Simultaneous enhancement of microalgae biomass growth and lipid accumulation under continuous aeration with 15% CO2. RSC Adv. 5, 50851–50858. doi:10.1039/c5ra08401f
Islam, M. U., Guo, Z., Jiang, F., and Peng, X. (2022). Does straw return increase crop yield in the wheat-maize cropping system in China? A meta-analysis. Field Crops Res. 279, 108447. doi:10.1016/j.fcr.2022.108447
Jhala, Y. K., Panpatte, D. G., and Vyas, R. V. (2017). “Cyanobacteria: Source of organic fertilizers for plant growth,” in Microorganisms for green revolution: Volume 1: Microbes for sustainable crop production. Editors D. G. Panpatte, Y. K. Jhala, R. V. Vyas, and H. N. Shelat, 253–264.
Ji, Y., Liu, P., and Conrad, R. (2018). Response of fermenting bacterial and methanogenic archaeal communities in paddy soil to progressing rice straw degradation. Soil Biol. Biochem. 124, 70–80. doi:10.1016/j.soilbio.2018.05.029
Jin, M., Guan, H., Zhang, W., Tian, D., Wei, J., Kalkhajeh, Y. K., et al. (2022). High level of iron inhibited maize straw decomposition by suppressing microbial communities and enzyme activities. Agronomy 12, 1286. doi:10.3390/agronomy12061286
Karthikeyan, N., Prasanna, R., Nain, L., and Kaushik, B. D. (2007). Evaluating the potential of plant growth promoting cyanobacteria as inoculants for wheat. Eur. J. Soil Biol. 43, 23–30. doi:10.1016/j.ejsobi.2006.11.001
Korneva, L. (2014). Invasions of alien species of planktonic microalgae into the fresh waters of Holarctic (Review). Russ. J. Biol. invasions 5, 65–81. doi:10.1134/s2075111714020052
Kumar, M., Prasanna, R., Bidyarani, N., Babu, S., Mishra, B. K., Kumar, A., et al. (2013). Evaluating the plant growth promoting ability of thermotolerant bacteria and cyanobacteria and their interactions with seed spice crops. Sci. Hortic. 164, 94–101. doi:10.1016/j.scienta.2013.09.014
Li, H., Chen, S., Liao, K., Lu, Q., and Zhou, W. (2020). Microalgae biotechnology as a promising pathway to ecofriendly aquaculture: A state-of-the-art review. J. Chem. Technol. Biotechnol. 96, 837–852. doi:10.1002/jctb.6624
Li, H., Dai, M., Dai, S., and Dong, X. (2018). Current status and environment impact of direct straw return in China's cropland - a review. Ecotoxicol. Environ. Saf. 159, 293–300. doi:10.1016/j.ecoenv.2018.05.014
Li, J., Zhang, J., Huang, W., Kong, F., Li, Y., Xi, M., et al. (2016). Comparative bioavailability of ammonium, nitrate, nitrite and urea to typically harmful cyanobacterium Microcystis aeruginosa. Mar. Pollut. Bull. 110, 93–98. doi:10.1016/j.marpolbul.2016.06.077
Li, S.-H., Guo, L.-J., Cao, C.-G., and Li, C.-F. (2020). Effects of straw returning levels on carbon footprint and net ecosystem economic benefits from rice-wheat rotation in central China. Environ. Sci. Pollut. Res. 28, 5742–5754. doi:10.1007/s11356-020-10914-w
Li, Y., Bi, M., Li, B., and Gao, W. (2018). Explosion behaviors of ammonia–air mixtures. Combust. Sci. Technol. 190, 1804–1816. doi:10.1080/00102202.2018.1473859
Li, Y., Feng, H., Xia, L., Li, J., Li, C., Zang, H., et al. (2022). Ammoniated straw incorporation increases wheat yield, yield stability, soil organic carbon and soil total nitrogen content. Field Crops Res. 284, 108558. doi:10.1016/j.fcr.2022.108558
Liu, B., Wu, Q., Wang, F., and Zhang, B. (2019). Is straw return-to-field always beneficial? Evidence from an integrated cost-benefit analysis. Energy 171, 393–402. doi:10.1016/j.energy.2019.01.031
Lu, Q., Chen, P., Addy, M., Zhang, R., Deng, X., Ma, Y., et al. (2018a). Carbon-dependent alleviation of ammonia toxicity for algae cultivation and associated mechanisms exploration. Bioresour. Technol. 249, 99–107. doi:10.1016/j.biortech.2017.09.175
Lu, Q., Han, P., Chen, F., Liu, T., Li, J., Leng, L., et al. (2019). A novel approach of using zeolite for ammonium toxicity mitigation and value-added Spirulina cultivation in wastewater. Bioresour. Technol. 280, 127–135. doi:10.1016/j.biortech.2019.02.042
Lu, Q., Ji, C., Yan, Y., Xiao, Y., Li, J., Leng, L., et al. (2018b). Application of a novel microalgae-film based air purifier to improve air quality through oxygen production and fine particulates removal. J. Chem. Technol. Biotechnol. 94, 1057–1063. doi:10.1002/jctb.5852
Lu, Q., Liu, H., Liu, W., Zhong, Y., Ming, C., Qian, W., et al. (2017). Pretreatment of brewery effluent to cultivate Spirulina sp. for nutrients removal and biomass production. Water Sci. Technol. 76, 1852–1866. doi:10.2166/wst.2017.363
Lu, Q., and Xiao, Y. (2022). From manure to high-value fertilizer: The employment of microalgae as a nutrient carrier for sustainable agriculture. Algal Res. 67, 102855. doi:10.1016/j.algal.2022.102855
Lu, Q., Xiao, Y., and Lu, Y. (2022). Employment of algae-based biological soil crust to control desertification for the sustainable development: A mini-review. Algal Res. 65, 102747. doi:10.1016/j.algal.2022.102747
Lu, Q., Zhou, W., Min, M., Ma, X., Chandra, C., Doan, Y. T., et al. (2015). Growing Chlorella sp. on meat processing wastewater for nutrient removal and biomass production. Bioresour. Technol. 198, 189–197. doi:10.1016/j.biortech.2015.08.133
Ma, Y., Chen, X., Zahoor Khan, M., Xiao, J., Liu, S., Wang, J., et al. (2020b). The impact of ammoniation treatment on the chemical composition and in vitro digestibility of rice straw in Chinese holsteins. Animals (Basel) 10, 1854. doi:10.3390/ani10101854
Ma, Y., Chen, X., Zahoor Khan, M., Xiao, J., Liu, S., Wang, J., et al. (2020). The impact of ammoniation treatment on the chemical composition and in vitro digestibility of rice straw in Chinese holsteins. Animals 10, 1854. doi:10.3390/ani10101854
Ma, Y., Liu, L., Schwenke, G., and Yang, B. (2019). The global warming potential of straw-return can be reduced by application of straw-decomposing microbial inoculants and biochar in rice-wheat production systems. Environ. Pollut. 252, 835–845. doi:10.1016/j.envpol.2019.06.006
Ma, Y., Shen, Y., and Liu, Y. (2020a). State of the art of straw treatment technology: Challenges and solutions forward. Bioresour. Technol. 313, 123656. doi:10.1016/j.biortech.2020.123656
Malam Issa, O., Défarge, C., Le Bissonnais, Y., Marin, B., Duval, O., Bruand, A., et al. (2006). Effects of the inoculation of cyanobacteria on the microstructure and the structural stability of a tropical soil. Plant Soil 290, 209–219. doi:10.1007/s11104-006-9153-9
Mengqi, Z., Shi, A., Ajmal, M., Ye, L., and Awais, M. (2021). Comprehensive review on agricultural waste utilization and high-temperature fermentation and composting. Biomass Convers. Biorefinery. doi:10.1007/s13399-021-01438-5
Pagels, F., Guedes, A. C., Amaro, H. M., Kijjoa, A., and Vasconcelos, V. (2019). Phycobiliproteins from cyanobacteria: Chemistry and biotechnological applications. Biotechnol. Adv. 37, 422–443. doi:10.1016/j.biotechadv.2019.02.010
Perera, I., Subashchandrabose, S. R., Venkateswarlu, K., Naidu, R., and Megharaj, M. (2018). Consortia of cyanobacteria/microalgae and bacteria in desert soils: An underexplored microbiota. Appl. Microbiol. Biotechnol. 102, 7351–7363. doi:10.1007/s00253-018-9192-1
Petrova, E., Kukarskikh, G., Krendeleva, T., and Antal, T. (2020). The mechanisms and role of photosynthetic hydrogen production by green microalgae. Microbiology 89, 251–265. doi:10.1134/s0026261720030169
Qiao, K., Takano, T., and Liu, S. (2015). Discovery of two novel highly tolerant NaHCO3 Trebouxiophytes: Identification and characterization of microalgae from extreme saline–alkali soil. Algal Res. 9, 245–253. doi:10.1016/j.algal.2015.03.023
Rathnan, R. K., and John, D. (2021). Isolation, screening, identification and optimized production of extracellular cellulase from Bacillus subtilis using cellulosic waste as carbon source. J. Microbiol. Biotechnol. Food Sci. 2021, 2383–2386.
Ren, J., Yu, P., and Xu, X. (2019). Straw utilization in China—status and recommendations. Sustainability 11, 1762. doi:10.3390/su11061762
Singh, G., Gupta, M. K., Chaurasiya, S., Sharma, V. S., and Pimenov, D. Y. (2021). Rice straw burning: A review on its global prevalence and the sustainable alternatives for its effective mitigation. Environ. Sci. Pollut. Res. 28, 32125–32155. doi:10.1007/s11356-021-14163-3
Singh, J. S., Kumar, A., and Singh, M. (2019). Cyanobacteria: A sustainable and commercial bio-resource in production of bio-fertilizer and bio-fuel from waste waters. Environ. Sustain. Indic. 3, 100008. doi:10.1016/j.indic.2019.100008
Singh, M. P., Vishwakarma, S. K., and Srivastava, A. K. (2013). Bioremediation of direct blue 14 and extracellular ligninolytic enzyme production by white rot fungi: Pleurotus spp. Pleurotus spp, Biomed. Res. Int. 2013, 180156. doi:10.1155/2013/180156
Singh, S. K., Sundaram, S., Sinha, S., Rahman, M. A., and Kapur, S. (2016). Recent advances in CO2 uptake and fixation mechanism of cyanobacteria and microalgae. Crit. Rev. Environ. Sci. Technol. 46, 1297–1323. doi:10.1080/10643389.2016.1217911
Song, Q., Zhu, J., Gong, Z., Feng, Y., Wang, Q., Sun, Y., et al. (2021). Effect of straw retention on carbon footprint under different cropping sequences in Northeast China. Environ. Sci. Pollut. Res. Int. 28, 54792–54801. doi:10.1007/s11356-021-14316-4
Song, X., Zhang, J., Peng, C., and Li, D. (2021). Replacing nitrogen fertilizer with nitrogen-fixing cyanobacteria reduced nitrogen leaching in red soil paddy fields. Agric. Ecosyst. Environ. 312, 107320. doi:10.1016/j.agee.2021.107320
Srimongkol, P., Thongchul, N., Phunpruch, S., and Karnchanatat, A. (2019). Ability of marine cyanobacterium Synechococcus sp. VDW to remove ammonium from brackish aquaculture wastewater. Agric. Water Manag. 212, 155–161. doi:10.1016/j.agwat.2018.09.006
Su, P., Brookes, P. C., He, Y., Wu, J., and Xu, J. (2016). An evaluation of a microbial inoculum in promoting organic C decomposition in a paddy soil following straw incorporation. J. Soils Sediments 16, 1776–1786. doi:10.1007/s11368-015-1340-y
Su, Y., Lv, J. L., Yu, M., Ma, Z. H., Xi, H., Kou, C. L., et al. (2020). Long-term decomposed straw return positively affects the soil microbial community. J. Appl. Microbiol. 128, 138–150. doi:10.1111/jam.14435
Sun, J., Lian, F., Liu, Z., Zhu, L., and Song, Z. (2014). Biochars derived from various crop straws: Characterization and Cd (II) removal potential. Ecotoxicol. Environ. Saf. 106, 226–231. doi:10.1016/j.ecoenv.2014.04.042
Tamaki, Y., and Mazza, G. (2010). Measurement of structural carbohydrates, lignins, and micro-components of straw and shives: Effects of extractives, particle size and crop species. Industrial Crops Prod. 31, 534–541. doi:10.1016/j.indcrop.2010.02.004
Wang, D., Zhou, L., Huang, S., Li, C., and Cao, C. (2013). Short-term effects of tillage practices and wheat-straw returned to the field on topsoil labile organic carbon fractions and yields in central China. J. Agro-Environment Sci. 32, 735–740.
Wang, J., Wang, X., Xu, M., Feng, G., Zhang, W., and Lu, C. a. (2015). Crop yield and soil organic matter after long-term straw return to soil in China. Nutrient Cycl. Agroecosyst. 102, 371–381. doi:10.1007/s10705-015-9710-9
Wang, N., Yu, J.-G., Zhao, Y.-H., Chang, Z.-Z., Shi, X.-X., Ma, L. Q., et al. (2018). Straw enhanced CO2 and CH4 but decreased N2O emissions from flooded paddy soils: Changes in microbial community compositions. Atmos. Environ. 174, 171–179. doi:10.1016/j.atmosenv.2017.11.054
Wang, W., Liu, Y., Li, D., Hu, C., and Rao, B. (2009). Feasibility of cyanobacterial inoculation for biological soil crusts formation in desert area. Soil Biol. Biochem. 41, 926–929. doi:10.1016/j.soilbio.2008.07.001
Wang, X., Yang, Z., Liu, X., Huang, G., Xiao, W., and Han, L. (2020). The composition characteristics of different crop straw types and their multivariate analysis and comparison. Waste Manag. 110, 87–97. doi:10.1016/j.wasman.2020.05.018
Wang, Y., Wu, P., Mei, F., Ling, Y., Qiao, Y., Liu, C., et al. (2021). Does continuous straw returning keep China farmland soil organic carbon continued increase? A meta-analysis. J. Environ. Manag. 288, 112391. doi:10.1016/j.jenvman.2021.112391
Wang, Z., Wang, W., and Lu, Y. (2022). Biodegradation of insecticides by gut bacteria isolated from stored grain beetles and its implication in host insecticide resistance. J. Stored Prod. Res. 96, 101943. doi:10.1016/j.jspr.2022.101943
Wood, T. M., Wilson, C. A., McCrae, S. I., and Joblin, K. N. (1986). A highly active extracellular cellulase from the anaerobic rumen fungus Neocallimastix frontalis. FEMS Microbiol. Lett. 34, 37–40. doi:10.1111/j.1574-6968.1986.tb01344.x
Wu, L., Zhang, X., Chen, H., Wang, D., Nawaz, M. M., Danso, F., et al. (2022). Nitrogen fertilization and straw management economically improve wheat yield and energy use efficiency, reduce carbon footprint. Agronomy 12, 848. doi:10.3390/agronomy12040848
Wuang, S. C., Khin, M. C., Chua, P. Q. D., and Luo, Y. D. (2016). Use of Spirulina biomass produced from treatment of aquaculture wastewater as agricultural fertilizers. Algal Res. 15, 59–64. doi:10.1016/j.algal.2016.02.009
Xiong, X., Liao, H., Ma, J., Liu, X., Zhang, L., Shi, X., et al. (2014). Isolation of a rice endophytic bacterium, P antoea sp. S d-1, with ligninolytic activity and characterization of its rice straw degradation ability. Lett. Appl. Microbiol. 58, 123–129. doi:10.1111/lam.12163
Xu, M. Y., Zeng, Q. Q., Li, H. K., Zhong, Y. M., Tong, L. Y., Ruan, R. G., et al. (2020). Contribution of glycerol addition and algal-bacterial cooperation to nutrients recovery: A study on the mechanisms of microalgae-based wastewater remediation. J. Chem. Technol. Biotechnol. 95, 1717–1728. doi:10.1002/jctb.6369
Yan, C., Yan, S.-S., Jia, T.-Y., Dong, S.-K., Ma, C.-M., and Gong, Z.-P. (2019). Decomposition characteristics of rice straw returned to the soil in northeast China. Nutrient Cycl. Agroecosyst. 114, 211–224. doi:10.1007/s10705-019-09999-8
Yan, X., Yagi, K., Akiyama, H., and Akimoto, H. (2005). Statistical analysis of the major variables controlling methane emission from rice fields. Glob. Change Biol. 11, 1131–1141. doi:10.1111/j.1365-2486.2005.00976.x
Ye, X., Ma, Z., Zhang, J., Du, H., Chen, J., Chen, H., et al. (2011). Important role of ammonia on haze formation in Shanghai. Environ. Res. Lett. 6, 024019. doi:10.1088/1748-9326/6/2/024019
Yin, H., Zhao, W., Li, T., Cheng, X., and Liu, Q. (2018). Balancing straw returning and chemical fertilizers in China: Role of straw nutrient resources. Renew. Sustain. Energy Rev. 81, 2695–2702. doi:10.1016/j.rser.2017.06.076
Yu, K., Feng, H., Zhao, Y., and Dong, Q. (2015). Ammoniated straw incorporation promoting straw decomposition and improving winter wheat yield and water use efficiency. Trans. Chin. Soc. Agric. Eng. 31, 103–111.
Yu, Q., Liu, R., Li, K., and Ma, R. (2019). A review of crop straw pretreatment methods for biogas production by anaerobic digestion in China. Renew. Sustain. Energy Rev. 107, 51–58. doi:10.1016/j.rser.2019.02.020
Zahedi, A. (2011). Maximizing solar PV energy penetration using energy storage technology. Renew. Sustain. energy Rev. 15, 866–870. doi:10.1016/j.rser.2010.09.011
Zhang, B., Li, H., Chen, L., Fu, T., Tang, B., Hao, Y., et al. (2022). Recent advances in the bioconversion of waste straw biomass with steam explosion technique: A comprehensive review. Processes 10, 1959. doi:10.3390/pr10101959
Zhang, J., Song, X., Wei, H., Zhou, W., Peng, C., and Li, D. (2021). Effect of substituting nitrogen fertilizer with nitrogen-fixing cyanobacteria on yield in a double-rice cropping system in southern China. J. Appl. Phycol. 33, 2221–2232. doi:10.1007/s10811-021-02455-7
Zhang, M., Du, M.-y., Wang, G.-x., Wang, Z.-y., and Lu, Y.-j. (2020). Identification, mRNA expression, and functional analysis of chitin synthase 2 gene in the rusty grain beetle, Cryptolestes ferrugineus. J. Stored Prod. Res. 87, 101622. doi:10.1016/j.jspr.2020.101622
Zhang, S., Li, Z., Liu, J., Li, Q., and Yang, X. (2014). Long-Term effects of straw and manure on crop micronutrient nutrition under a wheat-maize cropping system. J. Plant Nutr. 38, 742–753. doi:10.1080/01904167.2014.957390
Zhang, Y., Dou, S., Ndzelu, B. S., Ma, R., Zhang, D., Zhang, X., et al. (2022). Effects of returning corn straw and fermented corn straw to fields on the soil organic carbon pools and humus composition. Soil 8, 605–619. doi:10.5194/soil-8-605-2022
Zhao, S., Qiu, S., Xu, X., Ciampitti, I. A., Zhang, S., and He, P. (2019). Change in straw decomposition rate and soil microbial community composition after straw addition in different long-term fertilization soils. Appl. Soil Ecol. 138, 123–133. doi:10.1016/j.apsoil.2019.02.018
Zhong, Y., Liu, H., Li, H., Lu, Q., and Sun, Y. (2021). Does exogenous carbon source always promote algal biomass and nutrients removal in algal-bacterial wastewater remediation? J. Clean. Prod. 281, 125371. doi:10.1016/j.jclepro.2020.125371
Zhu, L., Hu, N., Yang, M., Zhan, X., and Zhang, Z. (2014). Effects of different tillage and straw return on soil organic carbon in a rice-wheat rotation system. PLoS One 9, e88900. doi:10.1371/journal.pone.0088900
Keywords: crop straw, algae, microorganisms, environment, agricultural resource
Citation: Lu Q, Xiao Y and Wu P (2023) Emerging technologies of employing algae and microorganisms to promote the return-to-field of crop straws: A mini-review. Front. Bioeng. Biotechnol. 11:1152778. doi: 10.3389/fbioe.2023.1152778
Received: 28 January 2023; Accepted: 16 March 2023;
Published: 30 March 2023.
Edited by:
Hongli Zheng, Nanchang University, ChinaReviewed by:
Huankai Li, Hong Kong Baptist University, Hong Kong SAR, ChinaYuchuan Wang, University of Illinois at Urbana-Champaign, United States
Chunyang Ma, National University of Singapore, Singapore
Copyright © 2023 Lu, Xiao and Wu. This is an open-access article distributed under the terms of the Creative Commons Attribution License (CC BY). The use, distribution or reproduction in other forums is permitted, provided the original author(s) and the copyright owner(s) are credited and that the original publication in this journal is cited, in accordance with accepted academic practice. No use, distribution or reproduction is permitted which does not comply with these terms.
*Correspondence: Qian Lu, bHVxaWFuQGp1c3QuZWR1LmNu; Yu Xiao, eGlhb3l1QGlzc2FzLmFjLmNu