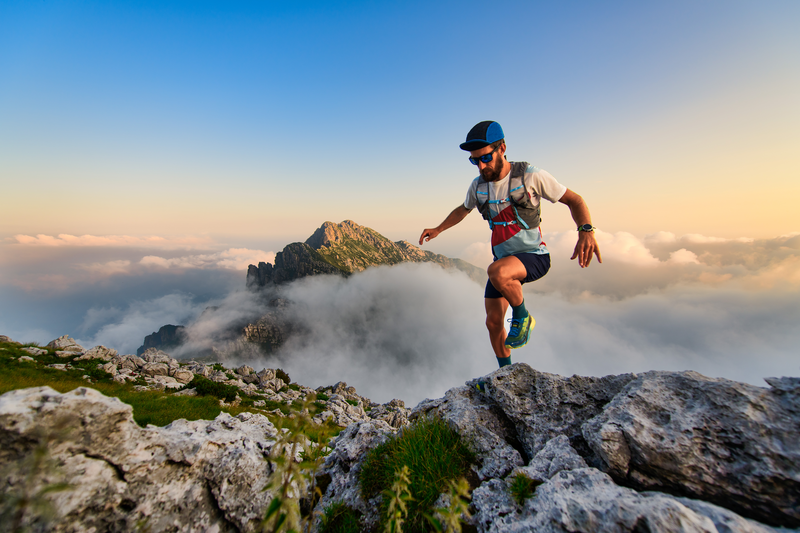
95% of researchers rate our articles as excellent or good
Learn more about the work of our research integrity team to safeguard the quality of each article we publish.
Find out more
REVIEW article
Front. Bioeng. Biotechnol. , 07 October 2022
Sec. Biomaterials
Volume 10 - 2022 | https://doi.org/10.3389/fbioe.2022.999358
This article is part of the Research Topic Molecular and Nanoscale Engineering of Nucleic Acid Theranostics and Vaccines View all 8 articles
As the outbreak of Coronavirus disease 2019 (COVID-19) caused by severe acute respiratory disease coronavirus 2 (SARS-COV-2), fast, accurate, and economic detection of viral infection has become crucial for stopping the spread. Polymerase chain reaction (PCR) of viral nucleic acids has been the gold standard method for SARS-COV-2 detection, which, however, generally requires sophisticated facilities and laboratory space, and is time consuming. This review presents recent advances in PCR-free nucleic acid detection methods for SARS-CoV-2, including emerging methods of isothermal amplification, nucleic acid enzymes, electrochemistry and CRISPR.
The COVID-19 outbreak, which was caused by SARS-COV-2, started in Wuhan, China in 2019 and has lasted for more than 2 years (Wu et al., 2020a). According to WHO statistics, SARS-COV-2 has infected more than five billion people and caused 6 million deaths by the end of May 2022 (Who Health Organization, 2020). Meanwhile, studies showed that SARS-CoV-2 reinfection increased the hospitalization and mortality compared to the first infection (Alotaiby et al., 2022; Comba et al., 2022), which means that it is important to maintain long-term monitoring of this virus.
Due to the high transmissibility characteristic of SARS-COV-2, rapid and accurate diagnostics methods are needed to prevent the virus from spreading. PCR is a reliable and widely used gold standard method in clinical and research laboratories around the world (Lan et al., 2020). PCR exhibits excellent specificity and sensitivity in virus detection. However, the technique needs to be performed in a laboratory with expensive equipment, qualified clinical laboratory personnel, and a clean environment to avoid contamination. Therefore, the technology cannot operated at the point of care and is less cost-effective (Yadav et al., 2021). Moreover, the standard real-time-polymerase chain reaction (RT-PCR) protocol requires 2–3 h to complete. Limited by the time of sample collection, transportation, and manipulation, the final diagnosis result cannot be obtained within 4–6 h. Other detection methods also have some limitations (Table 1), such as antigen tests are less accurate when used in people with no symptoms (Khandker et al., 2021). Therefore, there is an urgent need to develop fast, accurate, and cost-effective methods for SARS-COV-2 diagnosis. In this review, we summarized the recent reports on PCR-free detection methods for SARS-COV-2.
Reverse Transcription Loop‐Mediated Isothermal Amplification (RT-LAMP) systems are the most commonly used isothermal amplified nucleic acid detection methods and have the characteristics of speediness, high sensitivity and high specificity (Notomi et al., 2000; Chaouch, 2021). As regards SARS-CoV-2 detection, RT-LAMP is considered as an ideal alternative to RT-PCR. Lots of LAMP-based systems have been developed and some of them have been used to prevent further spread of the SARS-COV-2 pandemic (Andryukov et al., 2021).
The RT-LAMP detection systems are based on strand displacement DNA polymerase and 4-6 primers (Parida et al., 2004; Wong et al., 2018). Technically, two inner primers and two outer primers are designed to recognize the particular regions in the target sequence, while two extra loop primers are used to accelerate amplification and maintain stability (Chaouch, 2021). The limit of detection (LoD) of one step RT-LAMP is 10 copies of RNA fragments within 40 min at 65°C and the test results can be visualized by agarose gel electrophoresis, turbidity, fluorescence or colorimetry (Yuan et al., 2020). Researchers from different countries have done a number of clinical tests to study the detection performance of RT-LAMP. Results showed that it had excellent specificity, while the sensitivity fluctuated between 87% and 98% (Lee et al., 2020a; Broughton et al., 2020; Ganguli et al., 2020; Jiang et al., 2020; Anahtar et al., 2021). To further increase the sensitivity and reduce the contamination, several studies focused on the improvement of one step RT-LAMP. For example, replacing the universal transport medium with saline and adding an upfront RNase inactivation step can raise the sensitivity (Anahtar et al., 2021). Designing special primers for fluorogenic oligonucleotide strand exchange (OSD) probes can allow multiple genomic targets of SARS-CoV-2 included in one assay (Lamb et al., 2020). Based on the optimization of RT-LAMP, the Id NOW™ COVID-19 assay (Abbott Laboratories) has been approved by FDA with Emergency Use Authorization (EUA) (Yuan et al., 2020). This method targets the SARS-CoV-2 RdRp gene and can show positive results in high concentration samples within 5 min. However, one step RT-LAMP systems are still mostly used as lab-centric diagnostic techniques (Song et al., 2017; Pang et al., 2020). In order to meet the actual application requirements, many researchers chose to combine RT-LAMP with other innovative techniques. Researchers from China devised a diagnostic method based on RT-LAMP and nanoparticle-based lateral flow biosensor (LFB) (Zhu et al., 2020). In this reaction system, primers are labeled by FITC (fluorescein)-digoxin and biotin, so the results can be seen by naked eyes through immunoreactions and biotin/streptavidin interaction. The LoD is 12 copies per reaction and the sensitivity and specificity were both 100% (Zhu et al., 2020). Some experts believe this technology can be a useful diagnostic tool for resource-poor regions (Chaouch, 2021).
Although most of these have not yet been optimized for SARS-CoV-2, they have potential applications in the future. Digital RT-LAMP can realize absolute quantification of target sequences and offers a flexible test way for common laboratories (Lin et al., 2019). Rolling circle amplification (RCA) is a linear signal amplifying mechanism. RCA-LAMP can significantly improve the detection sensitivity and the reaction efficiency (Tian et al., 2019). Using naphthoquinone-imidazole (NQIM) probes to couple with LAMP can realize DNA amplification in 10 min (Chen et al., 2019). In summary, RT-LAMP-based technology has a great advantage in specificity and detection speed. In terms of sensitivity, it is reported to be one to two times less than RT‐PCR (Jiang et al., 2020; Wang et al., 2022a). The complexity of primer design is an inevitable drawback of RT-LAMP (Figure 1), but it is still a promising assay due to its compatibility for many other technologies (Qian et al., 2020; Chen et al., 2020).
FIGURE 1. Schematic of Isothermal Amplification methods for SARS-COV-2. (A) eRPA assay for detection of SARS-COV-2. Viral RNA is first copied to cDNA by RT, then degraded by RNase H. The cDNA product is amplified by RPA using a forward and a FAM-labeled reverse pair of primers specific to the target sequence. The amplified material is then denatured and hybridized to a biotinylated probe. Dual FAM-labeled and biotin-labeled products are detected on lateral flow strips. Source data are available in the Source Data file. Reprint from (Qian et al., 2020), copyright (2020), with permission from Springer Nature. (B) NISDA assay for rapid detection of SARS-CoV-2 RNA. The reaction mixture contains three key components, including a DNA duplex (the Initiator), and two DNA molecular beacon structures (probe M1 and probe M2). In presence of viral RNA/DNA, toehold-mediated template displacement and cascade signal amplification occur sequentially, following by fluorescence detection after 30 min at 42 °C. INA is intercalating nucleic acid with enhanced binding affinity. F and Q denote for 6-Fam fluorophore and bhq-1 quencher, respectively. Letter labels denote for domains. The domains labeled with primes are complementary sequences. Reprint from (Mohammadniaei et al., 2021), copyright (2020), with permission from Springer Nature.
Owing to its low operation temperature (25–42°C) and freeze-dried reagents, recombinase polymerase amplification (RPA) is undoubtedly a promising isothermal molecular technique for pathogen detection (Daher et al., 2016; Esbin et al., 2020). RPA entails two primers with simple design requirements compared to LAMP. It uses the Escherichia coli RecA recombinase and single-strand DNA binding protein (SSB) to substitute the heat denaturation step (Piepenburg et al., 2006). Meanwhile, DNA polymerase, usually from Staphylococcus aureus, is used to extend the chain. Polyethylene glycol or Carbowax20M initiates the reaction and creatine kinase generates ATP for the system (Piepenburg et al., 2006; Li and Macdonald, 2015). As for RNA targets, reverse transcriptase can realize one-step RT-RPA operation in 20 min (Hill-Cawthorne et al., 2014). RPA can tolerate common nucleic acid amplification inhibitors and can operate normally with various sample types, such as serum (Teoh et al., 2015), stool (Amer et al., 2013), nasal (Boyle et al., 2014) and even milk (Santiago-Felipe et al., 2015). Because of the advantages mentioned above, RPA has been used for the detection of a wide range of pathogens and cancers and has led to a number of post-detection methods (Amer et al., 2013; Loo et al., 2013). For example, hybridization assays can be realized when combined with ELISA (Santiago-Felipe et al., 2014). When combined with LAMP, it can be 10 times more sensitive than qPCR (Yuan et al., 2020). The limit of detection and turnaround time varies between amplicon size, assay and primers.
RPA-based detection technology of SARS-CoV-2 ensures accuracy while being faster and more portable than other current technologies (Wu et al., 2020b). Because of using simple primers, RPA makes it easier to achieve simultaneous detection of multiple pathogens or multiple targets, which further improves detection accuracy (Yuan et al., 2020). Ahmed et al. developed three RT-RPA assays targeting the RNA-dependent RNA polymerase (RdRP), envelope protein (E), and nucleocapsid protein (N) genes of SARS-CoV-2 (El Wahed et al., 2021). This method can detect three targets simultaneously at 42°C for 15 min and the entire reaction system is integrated into a mobile suitcase. The LoD of this assay is two RNA molecules for the RdRP gene and 15 RNA molecules for E and N genes (El Wahed et al., 2021). As for clinical specimens, the sensitivity and specificity in comparison to RT-PCR are 94% and 100% for RdRP gene, 65% and 77% for E gene; and 83% and 94% for N gene (El Wahed et al., 2021). This RPA-based technology can contribute to assisting the detection of SARS-CoV-2 in low-resource areas such as Africa. Another RPA-based assay is combined with CRISPR-Cas12a. Ding et al. (2020) described a new reaction in which all components were incubated in a single system without separate preamplification steps. This assay can detect SARS-CoV-2 and HIV-1 simultaneously at 37°C for 40 min. As an innovative attempt, it is able to detect as low as 4.6 copies RNA targets and 1.2 copies DNA targets (Ding et al., 2020). Some studies have shown that HIV infection can alter T cell functions and increase the chance of severe disease in patients with COVID-19 (Riou et al., 2021). So, this technique has potential value for screening critical patients.
In summary, RPA-based assay has the shortest reaction time of all current nucleic acid amplification technology (Amer et al., 2013). Its reaction temperature is around 37°C and can even react at room temperature under certain conditions (Loo et al., 2013). In addition, RPA allows for co-detection of multiple targets, which brings it a wider range of clinical application settings (Ding et al., 2020; El Wahed et al., 2021).
Rolling circle amplification (RCA) is another rapid and effective isothermal nucleic acid amplification method. Circular DNA template, DNA or RNA polymerase, short primer and deoxy-nucleotide triphosphates (dNTP) or nucleotide triphosphates (NTP) are the main component of the RCA reaction system (Kang et al., 2022). For RNA sequence amplification, Escherichia Coli RNA polymerase is essential for the successful running of the reaction (Lizardi et al., 1998). RCA also has the ability to integrate with other emerging technologies like nanobiotechnology and CRISPR (Kang et al., 2022), which allows it to monitor the background level and reduce the false-positive rate (Tian et al., 2020). As for SARS-CoV-2 detection, RCA-based techniques also contribute to the control of the pandemic. Liu et al. (2021) presented a completely new demonstration of the RCA-CRISPR system, which consists of a padlock probe-based RCA step and a subsequent CRISPR-Cas12a-based signal amplification step. The whole reaction takes 3 h at 30°C, with a LOD of 30.3 fM and a good specificity. Detection results of clinical samples (n = 48) by this method showed 100% concordance with RT-PCR (Liu et al., 2021). In summary, RCA is an easy and efficient isothermal enzymatic method using unique DNA and RNA polymerases with high specificity.
The reaction system of Nicking Enzyme Amplification Reaction (NEAR) includes three enzymes: reverse transcriptase, isothermal amplification enzyme (Bst), and nicking enzyme. The upstream and downstream primers perform strand displacement amplification on the target sequence under the action of isothermal amplification enzyme, and the nickase could recognize the specific sequence and cut the 8–16 base single strand to form a gap. The gap allows for the continued synthesis of short sequences using isothermally amplified DNA polymerases. The synthesized short-sequence are then combined with fluorescent primers for quantitative analysis. The ID NOW COVID-19 assay is a rapid and easy SARS-COV-2 method via NEAR. The ID NOW COVID-19 assay yielded a sensitivity, specificity, positive prediction value (PPV) and negative prediction value (NPV) of 98.0%, 97.5%, 96.2%, and 98.7% (NguyenVan et al., 2021).
Since most of the existing nucleic acid amplification techniques rely on biological enzymes, Mohammadniaei et al. (2021) developed a non-enzymatic whole genome detection method named non-enzymatic isothermal strand displacement and amplification (NISDA). This technique adds the displacement and amplification step on the basis of toehold-mediated strand displacement (TMSD) (Yurke et al., 2000; Lee et al., 2020b; Do et al., 2021). One DNA duplex and two DNA molecular beacon structures are the main components of the reaction system. The LoD of this method reaches 10 copies μL−1 under the condition of 42°C for 30 min (Mohammadniaei et al., 2021). As for clinical verification, NISDA assay represents 100% specificity and 96.77% sensitivity. This efficient method has generous storage conditions so it is thought to be a very fine complement to RT-PCR (Mohammadniaei et al., 2021).
The isothermal amplification methods could greatly reduce the dependence of detection on equipment. However, this method still has the problems such as the contamination of amplification products and high cost of multiple enzymes. We summarize some isothermal amplification methods in the Table 2; Figure 1.
Electrochemical biosensors have attracted much attention since they were developed and have been widely used in many fields, mainly focusing on the detection of pollutants in water and pathogenic microorganisms. Based on the specificity of DNA sequences and the principle of complementary base pairing of Watson-Crick, electrochemical nucleic acid biosensors have become an important branch of sensors and play an important role in biomedicine. Such devices work by converting DNA base pair recognition events into useful electrical signals (Wang, 2002). The materials of the sensor components are various according to different experimental designs, but the core is the design of the capture sequence and complementary sequence and the conversion of the signal.
Electrochemical sensor is very promising, which brings us a rapid and sensitive POCT detection for SARS-COV-2 detection solution. However, the guaranteed profit in electrochemical sensors in commercial applications depends on the selection of suitable nanomaterials as useful biosensors. In addition, the risk of errors such as false positives in virus detection should be considered when using Reusable and portability nanomaterials. We summarize some electrochemistry methods in the Table 3 and Figure 2.
FIGURE 2. Schematic of Electrochemical detect for SARS-COV-2. (A) Direct accounting assay is realized by the design of Y-shaped DNA double probe (Y-dual probe) which is modified on a graphene field effect transistor (gFET) to simultaneously detect ORF1ab and N gene of SARS-CoV-2 nucleic acid. Reprint from (Kong et al., 2021), copyright (2020), with permission from Springer Nature. (B) Low-cost and real-time detection of 2019-nCoV by immobilizing an aptamer against the SARS-CoV-2 spike protein on a gold-coated polyester substrate. Reprint from (Lasserre et al., 2022), copyright (2022), with permission from ACS Publications.
CRISPR (the clustered regularly interspaced short palindromic repeats) and CRISPR–Cas (clustered regularly interspaced short palindromic repeats–CRISPR-associated proteins) are adaptive immune systems in archaea and bacteria, bringing great changes to the development of gene editing technology (Barrangou et al., 2007; Feng et al., 2021). CRISPR–Cas systems use Cas protein as endonuclease to recognize and cleave specific nucleic acid sequences under the guidance of single-guide RNA (Abudayyeh et al., 2016). Based on the high specificity and high sensitivity of the CRISPR-Cas system, the detection technology of CRISPR-Cas has been rapidly developed and applied to the next-generation nucleic acid detection technology. CRISPR-Cas-based diagnostic technologies mainly use Cas12 and Cas13 enzymes, which can target DNA or RNA and possess collateral DNase or RNase activities (Freije and Sabeti, 2021). Besides, this system also has great potentials in biosensing devices that can be used for SARS-CoV-2 detection (Morales-Narvaez and Dincer, 2020). Long Ma et al. reported a CRISPR-Cas12a powered visual biosensor for ultrasensitive detection of SARS-CoV-2 (Ma et al., 2022). Fozouni et al. reported the development of an amplification-free CRISPR/Cas13a assay for detecting SARS-CoV-2 directly from nasal swab RNA that can be read with a mobile phone microscope (Fozouni et al., 2021). Kai Zhang et al. constructed an exonuclease III cleavage reaction-based isothermal amplification of nucleic acids with CRISPR/Cas12a-mediated pH-induced regenerative Electrochemiluminescence (ECL) biosensor for detection of SARS-CoV-2 nucleic acids (Zhang et al., 2022). In general, the CRISPR-Cas system has good specificity and sensitivity, and has good application prospects in the detection of viruses such as SARS-COV-2. However, the pretreatment of some CRISPR detection systems still relies on amplification, which may lead to problems such as product contamination and high cost of detection. Most of these methods are visualized, fast and sensitive, as listed in Table 4; Figure 3.
FIGURE 3. Schematic of CRISPR-Cas methods for SARS-COV-2. (A) Optimization workflow for RNA recovery and CRISPR-FDS reaction efficiency. Reprint from (Ma et al., 2022), copyright (2021), with permission from AAAS. (B) Workflow of MORIARTY detection of SARS-CoV-2 through a multiplex one-pot reaction strategy and schematic SARS-CoV-2 genome harboring Orf1ab, S, E, M, and N genes. Reprint from (Sridhara et al., 2021), copyright (2021), with permission from Springer Nature.
The development of in vitro selection methods, such as Systematic Evolution of Ligands by Exponential Enrichment (SELEX), to screen DNA or RNA from random sequence libraries has led to the development of further study of synthetic nucleic acids with special properties (Tuerk and Gold, 1990). These new DNA or RNA sequence offer more stable, and less expensive choice for detection platforms (McConnell et al., 2020). DNAzymes are synthetic ssDNA oligonucleotides with specific catalytic abilities (Silverman, 2010), designed for the detection of SARS-CoV-2 RNA. This design was highly sensitive (103 copies of viral RNA) for the N gene of SARS-CoV-2, which is not present in other viruses (Anantharaj et al., 2020). Yang reported a novel platform a detection limit of ≤20 a.m. for SARS-COV-2 in 1 h with a XNAzyme 10–23 (Figure 4), (Yang and Chaput, 2021). Compared with CRISPR system, nucleic acid enzyme has the advantages of no PAM motif, no protein expression and low cost.
FIGURE 4. Schematic of XNAzyme methods for SARS-COV-2. Reprint from (Yang and Chaput, 2021) copyright (2021), with permission from ACS.
The ongoing epidemic of SARS-COV-2 has severely impacted the global economy, travel, work and living habits. Recovery from the social and economic impact of SARS-CoV-2 has been prolonged due to differences in virus control measures in different countries (Kevadiya et al., 2021). Therefore, there is still a continuing demand for the diagnosis of SARS-CoV-2. The development of various new technologies brings us potential methods for better and faster viral nucleic acid detection (Li et al., 2021; Song et al., 2022). Most importantly, PCR-free virus detection techniques facilitate the development of sensitive, simple, scalable, rapid, and cost-effective detection methods for SARS-CoV-2, which could be translated to the development of rapid detection systems for the early response to various other infectious diseases, including newly found viruses, waste water-based epidemiology etc. However, few studies take full advantage of PCR-free methods in clinical translation. Many new methods always have some problems, such as instrument-dependent, expensive, and poor product reproducibility. Only assays that are sensitive, simple, rapid, reproducible, and cost-effective could be used in clinical translation.
In this review, we summarized methods of isothermal amplification, electrochemistry, CRISPR, and SERS methods developed for SARS-CoV-2 detection including the underlying scientific principles. We hope this review article would help the readers have a basic understanding of the current state of non-PCR methods for SARS-CoV-2 detection.
All authors listed have made a substantial, direct, and intellectual contribution to the work and approved it for publication.
The authors declare that the research was conducted in the absence of any commercial or financial relationships that could be construed as a potential conflict of interest.
The reviewer XZ declared a shared affiliation with the authors at the time of review.
All claims expressed in this article are solely those of the authors and do not necessarily represent those of their affiliated organizations, or those of the publisher, the editors and the reviewers. Any product that may be evaluated in this article, or claim that may be made by its manufacturer, is not guaranteed or endorsed by the publisher.
Abudayyeh, O. O., Gootenberg, J. S., Konermann, S., Joung, J., Slaymaker, I. M., Cox, D. B., et al. (2016). C2c2 is a single-component programmable RNA-guided RNA-targeting CRISPR effector. Science 353 (6299), aaf5573. doi:10.1126/science.aaf5573
Alafeef, M., Dighe, K., Moitra, P., and Pan, D. (2020). Rapid, ultrasensitive, and quantitative detection of SARS-CoV-2 using antisense oligonucleotides directed electrochemical biosensor chip. ACS Nano 14 (12), 17028–17045. doi:10.1021/acsnano.0c06392
Alotaiby, M., Krissaane, I., Seraihi, A. A., Alshenaifi, J., Qahtani, M. H., Aljeri, T., et al. (2022). SARS-CoV-2 reinfection rate and outcomes in Saudi arabia: A national retrospective study. Int. J. Infect. Dis. 122, 758–766. doi:10.1016/j.ijid.2022.07.025
Amer, H. M., Abd El Wahed, A., Shalaby, M. A., Almajhdi, F. N., Hufert, F. T., and Weidmann, M. (2013). A new approach for diagnosis of bovine coronavirus using a reverse transcription recombinase polymerase amplification assay. J. Virol. Methods 193 (2), 337–340. doi:10.1016/j.jviromet.2013.06.027
Anahtar, M. N., McGrath, G. E. G., Rabe, B. A., Tanner, N. A., White, B. A., Lennerz, J. K. M., et al. (2021). Clinical assessment and validation of a rapid and sensitive SARS-CoV-2 test using reverse transcription loop-mediated isothermal amplification without the need for RNA extraction. Open Forum Infect. Dis. 8 (2), ofaa631. doi:10.1093/ofid/ofaa631
Anantharaj, A., Das, S. J., Sharanabasava, P., Lodha, R., Kabra, S. K., Sharma, T. K., et al. (2020). Visual detection of SARS-CoV-2 RNA by conventional PCR-induced generation of DNAzyme sensor. Front. Mol. Biosci. 7, 586254. doi:10.3389/fmolb.2020.586254
Andryukov, B. G., Besednova, N. N., Kuznetsova, T. A., and Fedyanina, L. N. (2021). Laboratory-based resources for COVID-19 diagnostics: Traditional tools and novel technologies. A perspective of personalized medicine. J. Pers. Med. 11 (1), 42. doi:10.3390/jpm11010042
Barrangou, R., Fremaux, C., Deveau, H., Richards, M., Boyaval, P., Moineau, S., et al. (2007). CRISPR provides acquired resistance against viruses in prokaryotes. Science 315 (5819), 1709–1712. doi:10.1126/science.1138140
Boyle, D. S., McNerney, R., Teng Low, H., Leader, B. T., Perez-Osorio, A. C., Meyer, J. C., et al. (2014). Rapid detection of Mycobacterium tuberculosis by recombinase polymerase amplification. PLoS One 9 (8), e103091. doi:10.1371/journal.pone.0103091
Broughton, J. P., Deng, X., Yu, G., Fasching, C. L., Singh, J., Streithorst, J., et al. Rapid detection of 2019 novel coronavirus SARS-CoV-2 using a CRISPR-based DETECTR lateral flow assay. medRxiv. 2020.
Chaibun, T., Puenpa, J., Ngamdee, T., Boonapatcharoen, N., Athamanolap, P., O'Mullane, A. P., et al. (2021). Rapid electrochemical detection of coronavirus SARS-CoV-2. Nat. Commun. 12 (1), 802. doi:10.1038/s41467-021-21121-7
Chaouch, M. (2021). Loop-mediated isothermal amplification (LAMP): An effective molecular point-of-care technique for the rapid diagnosis of coronavirus SARS-CoV-2. Rev. Med. Virol. 31 (6), e2215. doi:10.1002/rmv.2215
Chen, B. J., Mani, V., Huang, S. T., Hu, Y. C., and Shan, H. P. (2019). Bisintercalating DNA redox reporters for real-time electrochemical qLAMP. Biosens. Bioelectron. X. 129, 277–283. doi:10.1016/j.bios.2018.09.056
Chen, Y., Shi, Y., Chen, Y., Yang, Z., Wu, H., Zhou, Z., et al. (2020). Contamination-free visual detection of SARS-CoV-2 with CRISPR/cas12a: A promising method in the point-of-care detection. Biosens. Bioelectron. X. 169, 112642. doi:10.1016/j.bios.2020.112642
Comba, I. Y., Riestra Guiance, I., Corsini Campioli, C., Challener, D., Sampathkumar, P., Orenstein, R., et al. (2022). Clinical characteristics and outcomes of patients with SARS-CoV-2 reinfection. Mayo Clin. Proc. Innovations Qual. Outcomes 6 (4), 361–372. doi:10.1016/j.mayocpiqo.2022.05.004
Crevillen, A. G., Mayorga‐Martinez, C. C., Vaghasiya, J. V., and Pumera, M. (2022). 3D‐Printed SARS‐CoV‐2 RNA genosensing microfluidic system. Adv. Mat. Technol. 7, 2101121. doi:10.1002/admt.202101121
Daher, R. K., Stewart, G., Boissinot, M., and Bergeron, M. G. (2016). Recombinase polymerase amplification for diagnostic applications. Clin. Chem. 62 (7), 947–958. doi:10.1373/clinchem.2015.245829
Deng, Y., Peng, Y., Wang, L., Wang, M., Zhou, T., Xiang, L., et al. (2022). Target-triggered cascade signal amplification for sensitive electrochemical detection of SARS-CoV-2 with clinical application. Anal. Chim. acta 1208, 339846. doi:10.1016/j.aca.2022.339846
Ding, X., Yin, K., Li, Z., and Liu, C. All-in-One dual CRISPR-cas12a (AIOD-CRISPR) assay: A case for rapid, ultrasensitive and visual detection of novel coronavirus SARS-CoV-2 and HIV virus. bioRxiv. 2020.
Do, J. Y., Jeong, J. Y., and Hong, C. A. (2021). Catalytic hairpin DNA assembly-based chemiluminescent assay for the detection of short SARS-CoV-2 target cDNA. Talanta 233, 122505. doi:10.1016/j.talanta.2021.122505
El Wahed, A. A., Patel, P., Maier, M., Pietsch, C., Ruster, D., Bohlken-Fascher, S., et al. (2021). Suitcase lab for rapid detection of SARS-CoV-2 based on recombinase polymerase amplification assay. Anal. Chem. 93 (4), 2627–2634. doi:10.1021/acs.analchem.0c04779
Esbin, M. N., Whitney, O. N., Chong, S., Maurer, A., Darzacq, X., and Tjian, R. (2020). Overcoming the bottleneck to widespread testing: a rapid review of nucleic acid testing approaches for COVID-19 detection. RNA 26 (7), 771–783. doi:10.1261/rna.076232.120
Farzin, L., Sadjadi, S., Sheini, A., and Mohagheghpour, E. (2021). A nanoscale genosensor for early detection of COVID-19 by voltammetric determination of RNA-dependent RNA polymerase (RdRP) sequence of SARS-CoV-2 virus. Microchim. Acta 188 (4), 121–212. doi:10.1007/s00604-021-04773-6
Feng, W., Newbigging, A. M., Tao, J., Cao, Y., Peng, H., Le, C., et al. (2021). CRISPR technology incorporating amplification strategies: molecular assays for nucleic acids, proteins, and small molecules. Chem. Sci. 12 (13), 4683–4698. doi:10.1039/d0sc06973f
Fozouni, P., Son, S., Diaz de Leon Derby, M., Knott, G. J., Gray, C. N., D'Ambrosio, M. V., et al. (2021). Amplification-free detection of SARS-CoV-2 with CRISPR-Cas13a and mobile phone microscopy. Cell 184 (2), 323–333. doi:10.1016/j.cell.2020.12.001
Freije, C. A., and Sabeti, P. C. (2021). Detect and destroy: CRISPR-based technologies for the response against viruses. Cell Host Microbe 29 (5), 689–703. doi:10.1016/j.chom.2021.04.003
Ganguli, A., Mostafa, A., Berger, J., Aydin, M., Sun, F., Valera, E., et al. Rapid isothermal amplification and portable detection system for SARS-CoV-2. bioRxiv. 2020.
Hill-Cawthorne, G. A., Hudson, L. O., El Ghany, M. F., Piepenburg, O., Nair, M., Dodgson, A., et al. (2014). Recombinations in staphylococcal cassette chromosome mec elements compromise the molecular detection of methicillin resistance in Staphylococcus aureus. PLoS One 9 (6), e101419. doi:10.1371/journal.pone.0101419
Jiang, M., Pan, W., Arasthfer, A., Fang, W., Ling, L., Fang, H., et al. (2020). Development and validation of a rapid, single-step reverse transcriptase loop-mediated isothermal amplification (RT-LAMP) system potentially to Be used for reliable and high-throughput screening of COVID-19. Front. Cell. Infect. Microbiol. 10, 331. doi:10.3389/fcimb.2020.00331
Kang, T., Lu, J., Yu, T., Long, Y., and Liu, G. (2022). Advances in nucleic acid amplification techniques (NAATs): COVID-19 point-of-care diagnostics as an example. Biosens. Bioelectron. X. 206, 114109. doi:10.1016/j.bios.2022.114109
Kashefi-Kheyrabadi, L., Nguyen, H. V., Go, A., Baek, C., Jang, N., Lee, J. M., et al. (2022). Rapid, multiplexed, and nucleic acid amplification-free detection of SARS-CoV-2 RNA using an electrochemical biosensor. Biosens. Bioelectron. 195, 113649. doi:10.1016/j.bios.2021.113649
Kevadiya, B. D., Machhi, J., Herskovitz, J., Oleynikov, M. D., Blomberg, W. R., Bajwa, N., et al. (2021). Diagnostics for SARS-CoV-2 infections. Nat. Mat. 20 (5), 593–605. doi:10.1038/s41563-020-00906-z
Khandker, S. S., Nik Hashim, N. H. H., Deris, Z. Z., Shueb, R. H., and Islam, M. A. (2021). Diagnostic accuracy of rapid antigen test kits for detecting SARS-CoV-2: A systematic review and meta-analysis of 17, 171 suspected COVID-19 patients. J. Clin. Med. 10 (16), 3493. doi:10.3390/jcm10163493
Kong, D., Wang, X., Gu, C., Guo, M., Wang, Y., Ai, Z., et al. (2021). Direct SARS-CoV-2 nucleic acid detection by Y-shaped DNA dual-probe transistor assay. J. Am. Chem. Soc. 143 (41), 17004–17014. doi:10.1021/jacs.1c06325
Kumar, M., Nandeshwar, R., Lad, S. B., Megha, K., Mangat, M., Butterworth, A., et al. (2021). Electrochemical sensing of SARS-CoV-2 amplicons with PCB electrodes. Sensors Actuators B Chem. 343, 130169. doi:10.1016/j.snb.2021.130169
Lamb, L. E., Bartolone, S. N., Ward, E., and Chancellor, M. B. (2020). Rapid detection of novel coronavirus/Severe Acute Respiratory Syndrome Coronavirus 2 (SARS-CoV-2) by reverse transcription-loop-mediated isothermal amplification. PLoS One 15 (6), e0234682. doi:10.1371/journal.pone.0234682
Lan, L., Xu, D., Ye, G., Xia, C., Wang, S., Li, Y., et al. (2020). Positive RT-PCR test results in patients recovered from COVID-19. JAMA 323 (15), 1502–1503. doi:10.1001/jama.2020.2783
Lasserre, P., Balansethupathy, B., Vezza, V. J., Butterworth, A., Macdonald, A., Blair, E. O., et al. (2022). SARS-CoV-2 aptasensors based on electrochemical impedance spectroscopy and low-cost gold electrode substrates. Anal. Chem. 94 (4), 2126–2133. doi:10.1021/acs.analchem.1c04456
Lee, J. Y. H., Best, N., McAuley, J., Porter, J. L., Seemann, T., Schultz, M. B., et al. (2020). Validation of a single-step, single-tube reverse transcription loop-mediated isothermal amplification assay for rapid detection of SARS-CoV-2 RNA. J. Med. Microbiol. 69 (9), 1169–1178. doi:10.1099/jmm.0.001238
Lee, T., Mohammadniaei, M., Zhang, H., Yoon, J., Choi, H. K., Guo, S., et al. (2020). Single functionalized pRNA/gold nanoparticle for ultrasensitive MicroRNA detection using electrochemical surface-enhanced Raman spectroscopy. Adv. Sci. (Weinh). 7 (3), 1902477. doi:10.1002/advs.201902477
Li, J., and Macdonald, J. (2015). Advances in isothermal amplification: novel strategies inspired by biological processes. Biosens. Bioelectron. X. 64, 196–211. doi:10.1016/j.bios.2014.08.069
Li, M., Yin, F., Song, L., Mao, X., Li, F., Fan, C., et al. (2021). Nucleic acid tests for clinical translation. Chem. Rev. 121 (17), 10469–10558. doi:10.1021/acs.chemrev.1c00241
Lin, X., Huang, X., Urmann, K., Xie, X., and Hoffmann, M. R. (2019). Digital loop-mediated isothermal amplification on a commercial membrane. ACS Sens. 4 (1), 242–249. doi:10.1021/acssensors.8b01419
Liu, R., Hu, Y., He, Y., Lan, T., and Zhang, J. (2021). Translating daily COVID-19 screening into a simple glucose test: a proof of concept study. Chem. Sci. 12 (26), 9022–9030. doi:10.1039/d1sc00512j
Lizardi, P. M., Huang, X., Zhu, Z., Bray-Ward, P., Thomas, D. C., and Ward, D. C. (1998). Mutation detection and single-molecule counting using isothermal rolling-circle amplification. Nat. Genet. 19 (3), 225–232. doi:10.1038/898
Loo, J. F., Lau, P. M., Ho, H. P., and Kong, S. K. (2013). An aptamer-based bio-barcode assay with isothermal recombinase polymerase amplification for cytochrome-c detection and anti-cancer drug screening. Talanta 115, 159–165. doi:10.1016/j.talanta.2013.04.051
Lu, R., Wu, X., Wan, Z., Li, Y., Jin, X., and Zhang, C. (2020). A novel reverse transcription loop-mediated isothermal amplification method for rapid detection of SARS-CoV-2. Int. J. Mol. Sci. 21 (8), 2826. doi:10.3390/ijms21082826
Lu, S., Tong, X., Han, Y., Zhang, K., Zhang, Y., Chen, Q., et al. (2022). Fast and sensitive detection of SARS-CoV-2 RNA using suboptimal protospacer adjacent motifs for Cas12a. Nat. Biomed. Eng. 6 (3), 286–297. doi:10.1038/s41551-022-00861-x
Ma, L., Yin, L., Li, X., Chen, S., Peng, L., Liu, G., et al. (2022). A smartphone-based visual biosensor for CRISPR-Cas powered SARS-CoV-2 diagnostics. Biosens. Bioelectron. X. 195, 113646. doi:10.1016/j.bios.2021.113646
McConnell, E. M., Cozma, I., Morrison, D., and Li, Y. (2020). Biosensors made of synthetic functional nucleic acids toward better human health. Anal. Chem. 92 (1), 327–344. doi:10.1021/acs.analchem.9b04868
Mohammadniaei, M., Zhang, M., Ashley, J., Christensen, U. B., Friis-Hansen, L. J., Gregersen, R., et al. (2021). A non-enzymatic, isothermal strand displacement and amplification assay for rapid detection of SARS-CoV-2 RNA. Nat. Commun. 12 (1), 5089. doi:10.1038/s41467-021-25387-9
Morales-Narvaez, E., and Dincer, C. (2020). The impact of biosensing in a pandemic outbreak: COVID-19. Biosens. Bioelectron. X. 163, 112274. doi:10.1016/j.bios.2020.112274
NguyenVan, J. C., Gerlier, C., Pilmis, B., Mizrahi, A., Pean de Ponfilly, G., Khaterchi, A., et al. (2021). Prospective evaluation of ID NOW COVID-19 assay used as point-of-care test in an emergency department. J. Clin. Virol. 145, 105021. doi:10.1016/j.jcv.2021.105021
Ning, B., Yu, T., Zhang, S., Huang, Z., Tian, D., Lin, Z., et al. (2021). A smartphone-read ultrasensitive and quantitative saliva test for COVID-19. Sci. Adv. 7 (2), eabe3703. doi:10.1126/sciadv.abe3703
Notomi, T., Okayama, H., Masubuchi, H., Yonekawa, T., Watanabe, K., Amino, N., et al. (2000). Loop-mediated isothermal amplification of DNA. Nucleic Acids Res. 28 (12), e63. doi:10.1093/nar/28.12.e63
Pang, B., Xu, J., Liu, Y., Peng, H., Feng, W., Cao, Y., et al. (2020). Isothermal amplification and ambient visualization in a single tube for the detection of SARS-CoV-2 using loop-mediated amplification and CRISPR technology. Anal. Chem. 92 (24), 16204–16212. doi:10.1021/acs.analchem.0c04047
Parida, M., Posadas, G., Inoue, S., Hasebe, F., and Morita, K. (2004). Real-time reverse transcription loop-mediated isothermal amplification for rapid detection of west nile virus. J. Clin. Microbiol. 42 (1), 257–263. doi:10.1128/jcm.42.1.257-263.2004
Peng, Y., Pan, Y., Sun, Z., Li, J., Yi, Y., Yang, J., et al. (2021). An electrochemical biosensor for sensitive analysis of the SARS-CoV-2 RNA. Biosens. Bioelectron. 186, 113309. doi:10.1016/j.bios.2021.113309
Piepenburg, O., Williams, C. H., Stemple, D. L., and Armes, N. A. (2006). DNA detection using recombination proteins. PLoS Biol. 4 (7), e204. doi:10.1371/journal.pbio.0040204
Qian, J., Boswell, S. A., Chidley, C., Lu, Z. X., Pettit, M. E., Gaudio, B. L., et al. (2020). An enhanced isothermal amplification assay for viral detection. Nat. Commun. 11 (1), 5920. doi:10.1038/s41467-020-19258-y
Riou, C., du Bruyn, E., Stek, C., Daroowala, R., Goliath, R. T., Abrahams, F., et al. (2021). Relationship of SARS-CoV-2-specific CD4 response to COVID-19 severity and impact of HIV-1 and tuberculosis coinfection. J. Clin. Invest. 131 (12), 149125. doi:10.1172/jci149125
Santiago-Felipe, S., Tortajada-Genaro, L. A., Puchades, R., and Maquieira, A. (2014). Recombinase polymerase and enzyme-linked immunosorbent assay as a DNA amplification-detection strategy for food analysis. Anal. Chim. Acta X. 811, 81–87. doi:10.1016/j.aca.2013.12.017
Santiago-Felipe, S., Tortajada-Genaro, L. A., Morais, S., Puchades, R., and Maquieira, A. (2015). Isothermal DNA amplification strategies for duplex microorganism detection. Food Chem. x. 174, 509–515. doi:10.1016/j.foodchem.2014.11.080
Silverman, S. K. (2010). DNA as a versatile chemical component for catalysis, encoding, and stereocontrol. Angew. Chem. Int. Ed. 49 (40), 7180–7201. doi:10.1002/anie.200906345
Song, J., Liu, C., Mauk, M. G., Rankin, S. C., Lok, J. B., Greenberg, R. M., et al. (2017). Two-stage isothermal enzymatic amplification for concurrent multiplex molecular detection. Clin. Chem. 63 (3), 714–722. doi:10.1373/clinchem.2016.263665
Song, L., Zhuge, Y., Zuo, X., Li, M., and Wang, F. (2022). DNA walkers for biosensing development. Adv. Sci. (Weinh). 9 (18), e2200327. doi:10.1002/advs.202200327
Sridhara, S., Goswami, H. N., Whyms, C., Dennis, J. H., and Li, H. (2021). Virus detection via programmable Type III-A CRISPR-Cas systems. Nat. Commun. 12 (1), 5653. doi:10.1038/s41467-021-25977-7
Teoh, B. T., Sam, S. S., Tan, K. K., Danlami, M. B., Shu, M. H., Johari, J., et al. (2015). Early detection of dengue virus by use of reverse transcription-recombinase polymerase amplification. J. Clin. Microbiol. 53 (3), 830–837. doi:10.1128/jcm.02648-14
Tian, W., Li, P., He, W., Liu, C., and Li, Z. (2019). Rolling circle extension-actuated loop-mediated isothermal amplification (RCA-LAMP) for ultrasensitive detection of microRNAs. Biosens. Bioelectron. X. 128, 17–22. doi:10.1016/j.bios.2018.12.041
Tian, B., Minero, G. A. S., Fock, J., Dufva, M., and Hansen, M. F. (2020). CRISPR-Cas12a based internal negative control for nonspecific products of exponential rolling circle amplification. Nucleic Acids Res. 48 (5), e30. doi:10.1093/nar/gkaa017
Tuerk, C., and Gold, L. (1990). Systematic evolution of ligands by exponential enrichment: RNA ligands to bacteriophage T4 DNA polymerase. Science 249 (4968), 505–510. doi:10.1126/science.2200121
Wang, H. J., Xiang, Y. H., Hu, R., Ji, R., and Wang, Y. P. (2022). Research progress in laboratory detection of SARS-CoV-2. Ir. J. Med. Sci. 191 (2), 509–517. doi:10.1007/s11845-021-02604-4
Wang, Y., Xue, T., Wang, M., Ledesma-Amaro, R., Lu, Y., Hu, X., et al. (2022). CRISPR-Cas13a cascade-based viral RNA assay for detecting SARS-CoV-2 and its mutations in clinical samples. Sensors Actuators B Chem. 362, 131765. doi:10.1016/j.snb.2022.131765
Wang, J. (2002). Electrochemical nucleic acid biosensors. Anal. Chim. acta 469 (1), 63–71. doi:10.1016/s0003-2670(01)01399-x
Who Health Organization (2020). WHO coronavirus (COVID-19) dashboard. Available from: https://covid19.who.int.
Wong, Y. P., Othman, S., Lau, Y. L., Radu, S., and Chee, H. Y. (2018). Loop-mediated isothermal amplification (LAMP): a versatile technique for detection of micro-organisms. J. Appl. Microbiol. 124 (3), 626–643. doi:10.1111/jam.13647
Wu, F., Zhao, S., Yu, B., Chen, Y. M., Wang, W., Song, Z. G., et al. (2020). A new coronavirus associated with human respiratory disease in China. Nature 579 (7798), 265–269. doi:10.1038/s41586-020-2008-3
Wu, H., Qian, C., Wu, C., Wang, Z., Wang, D., Ye, Z., et al. (2020). End-point dual specific detection of nucleic acids using CRISPR/Cas12a based portable biosensor. Biosens. Bioelectron. X. 157, 112153. doi:10.1016/j.bios.2020.112153
Yadav, H., Shah, D., Sayed, S., Horton, S., and Schroeder, L. F. (2021). Availability of essential diagnostics in ten low-income and middle-income countries: results from national health facility surveys. Lancet Glob. Health 9 (11), e1553–e1560. doi:10.1016/s2214-109x(21)00442-3
Yang, K., and Chaput, J. C. (2021). REVEALR: a multicomponent XNAzyme-based nucleic acid detection system for SARS-CoV-2. J. Am. Chem. Soc. 143 (24), 8957–8961. doi:10.1021/jacs.1c02664
Yuan, X., Yang, C., He, Q., Chen, J., Yu, D., Li, J., et al. (2020). Current and perspective diagnostic techniques for COVID-19. ACS Infect. Dis. 6 (8), 1998–2016. doi:10.1021/acsinfecdis.0c00365
Yurke, B., Turberfield, A. J., Mills, A. P., Simmel, F. C., and Neumann, J. L. (2000). A DNA-fuelled molecular machine made of DNA. Nature 406 (6796), 605–608. doi:10.1038/35020524
Zhang, K., Fan, Z., Ding, Y., and Xie, M. (2022). A pH-engineering regenerative DNA tetrahedron ECL biosensor for the assay of SARS-CoV-2 RdRp gene based on CRISPR/Cas12a trans-activity. Chem. Eng. J. 429, 132472. doi:10.1016/j.cej.2021.132472
Zhao, H., Liu, F., Xie, W., Zhou, T. C., OuYang, J., Jin, L., et al. (2021). Ultrasensitive supersandwich-type electrochemical sensor for SARS-CoV-2 from the infected COVID-19 patients using a smartphone. Sensors Actuators B Chem. 327, 128899. doi:10.1016/j.snb.2020.128899
Zhao, H., Zhang, Y., Chen, Y., Ho, N. R., Sundah, N. R., Natalia, A., et al. (2021). Accessible detection of SARS-CoV-2 through molecular nanostructures and automated microfluidics. Biosens. Bioelectron. 194, 113629. doi:10.1016/j.bios.2021.113629
Keywords: SARS-CoV-2, PCR-free detection, nucleic acids, CRISPR, isothermal amplification
Citation: Ma X, Xu J, Zhou F, Ye J, Yang D, Wang H, Wang P and Li M (2022) Recent advances in PCR-free nucleic acid detection for SARS-COV-2. Front. Bioeng. Biotechnol. 10:999358. doi: 10.3389/fbioe.2022.999358
Received: 21 July 2022; Accepted: 26 September 2022;
Published: 07 October 2022.
Edited by:
Guizhi Zhu, Virginia Commonwealth University, United StatesReviewed by:
Haipeng Liu, Wayne State University, United StatesCopyright © 2022 Ma, Xu, Zhou, Ye, Yang, Wang, Wang and Li. This is an open-access article distributed under the terms of the Creative Commons Attribution License (CC BY). The use, distribution or reproduction in other forums is permitted, provided the original author(s) and the copyright owner(s) are credited and that the original publication in this journal is cited, in accordance with accepted academic practice. No use, distribution or reproduction is permitted which does not comply with these terms.
*Correspondence: Hua Wang, ZmRlZHV3aEAxMjYuY29t; Pengfei Wang, cGVuZ2ZlaS53YW5nQHNqdHUuZWR1LmNu; Min Li, cmpsaW1pbkBzaHNtdS5lZHUuY24=
Disclaimer: All claims expressed in this article are solely those of the authors and do not necessarily represent those of their affiliated organizations, or those of the publisher, the editors and the reviewers. Any product that may be evaluated in this article or claim that may be made by its manufacturer is not guaranteed or endorsed by the publisher.
Research integrity at Frontiers
Learn more about the work of our research integrity team to safeguard the quality of each article we publish.