- 1Department of Evolution, Ecology, and Organismal Biology, University of California, Riverside, Riverside, CA, United States
- 2School of BioSciences, The University of Melbourne, Melbourne, VIC, Australia
Along with higher average temperatures, global climate change is expected to lead to more frequent and intense extreme heat events, and these different types of warming are likely to differ in their effects on bees. Although solitary bees comprise >75% of bee species, and despite their ecological and economic value as pollinators, a literature search revealed that only 8% of studies on bee responses to warming involve solitary bees. Here we review studies that have addressed how solitary bees are affected by three main types of warming that vary in magnitude and duration: heat shocks, heat waves, and sustained warming. We focus on direct physiological and behavioral effects of warming on solitary bees, rather than the underlying mechanisms. We find that heat shocks have received little attention in solitary bees both in terms of number of studies and relative to social bees, and all of those studies examine the effects of heat shocks on a single genus, Megachile. This work has shown that heat-shocked eggs, larvae, and pupae tend to upregulate heat shock protein genes, while heat shock at the adult stage can increase mortality in male bees, potentially altering population sex ratios. We find that solitary bee responses to heat waves have received even less study, but the few studies suggest that these events can increase larval mortality and slow development time, and that bees may not be able to physiologically acclimate to heat wave conditions by increasing their critical thermal maxima. Finally, sustained warming, which has been relatively well-studied in solitary bees, can speed development rate, reduce body mass, increase mortality, and alter foraging behavior. Our review reveals knowledge gaps in the effects of heat shocks and heat waves on solitary bees and, more broadly, in the responses of unmanaged solitary bees to warming. To improve our ability to anticipate the consequences of climate change for these critical pollinators, we encourage research on solitary bee thermal responses that examines short-term, extreme warming and incorporates greater ecological realism and complexity.
1 Introduction
Climate change represents a major threat to global biodiversity, with effects spanning levels of biological organization from genes to biomes (Scheffers et al., 2016). In particular, rapidly increasing temperatures will challenge the limits of acclimatization and adaptation to novel environmental conditions (Sears and Angilletta, 2011). With regions experiencing simultaneous increases in mean average temperatures and in the magnitude of temperature fluctuations (Lewis and King, 2017; Chen et al., 2018; Arnell et al., 2019), it is crucial to understand how both sustained warming and shorter-term episodes of extreme warming will affect species, their interactions, and the ecosystem services and functions they provide.
Among the ecological systems threatened by warming, plant-pollinator interaction networks are critical for crop production (Klein et al., 2007; Eilers et al., 2011) and the maintenance of biodiversity in natural communities (Wei et al., 2021). Globally, 85% of crops are pollinated by animals, and cultivation of pollinator-dependent crops is increasing (Klein et al., 2007). Additionally, almost 90% of flowering plants rely on animals for pollination, and these plants provide resources for many other species (Ollerton et al., 2011). Thus, it is important to understand how warming, spanning various magnitudes and durations, will affect pollinators and thus pollination services.
Bees are ecologically valuable pollinators (Pardee et al., 2022) and are facing various environmental stressors thought to be responsible for widespread population declines, including warming associated with climate change (Goulson et al., 2015). Given bees are largely ectothermic, and many aspects of their life histories are known to be sensitive to temperature, climate warming is likely to have strong direct effects on their physiology, phenology, development, and behavior (Hegland et al., 2009; Scaven and Rafferty, 2013). Indeed, recent studies have documented multifaceted effects of warming on bees (Hayes and López-Martínez, 2021; Zaragoza-Trello et al., 2021; Melone et al., 2024). However, variation in the magnitude and duration of warming under study make it challenging to synthesize these effects. Furthermore, as we demonstrate herein, most research has focused on social bee responses to warming, leaving much to be understood about the effects of warming on the solitary bees that comprise more than 75% of all bee species (Danforth et al., 2019).
Here, we review the direct physiological and behavioral effects of climate warming on solitary bees, focusing on three main types of warming: heat shocks, heat waves, and sustained warming. Because exact, quantitative definitions of these different types of warming are not well-resolved (e.g., IPCC, 2018; Barriopedro et al., 2023), we use these categorical terms to identify and group studies on warming and solitary bees. In general terms, heat shocks refer to extreme, short-term spikes in temperature, whereas heat waves are mid-magnitude and medium-duration events. Finally, sustained warming reflects lower-magnitude, longer-term increases in global mean temperatures associated with climate change.
By organizing empirical studies according to these three different magnitudes and durations of warming, we can discern whether they have differing effects on solitary bees. Whereas heat shock may cause acute stress and immediate mortality if temperatures exceed critical thermal maxima, heat waves may cause chronic physiological stress as individuals attempt to acclimate (e.g., Hayes and López-Martínez, 2021; Gonzalez et al., 2024). The effects of sustained warming may be more subtle and cumulative, expressed in terms of altered sex ratio, size, phenology, behavior, and lifespan (e.g., Slominski and Burkle, 2019; de Manincor et al., 2023). Although small-scale spatial heterogeneity in temperature is likely to shape bee responses to warming (Sears et al., 2011), we focus on temporal aspects of warming while acknowledging that bees can encounter many different microclimates as the sun rises and heats up a nesting site, during flight and as they forage, and inside flowers they visit (Herrera, 1995).
We first present the results of a literature survey to quantify how many studies have considered social vs. solitary bee responses to warming. We use this survey to draw attention to the paucity of work on the responses of solitary bees to heat shocks and heat waves, in particular. We conclude by suggesting directions for future research to improve our understanding of the impacts of warming on solitary bees.
2 Literature survey
We conducted literature searches to determine how many studies on bee responses to warming have involved solitary bees. We also used these searches to identify and synthesize studies on solitary bee responses to heat shocks, heat waves, and sustained warming. All topic searches were conducted in the Web of Science database, included “article,” “early access,” and “review article” document types, and spanned all years. Aside from the terms of interest, the parameters for each search were identical.
We first conducted a search for studies on all three types of warming and social bees, using a topic search for (warming OR warmed OR heating OR heated OR “heat shock*” OR heatshock* OR “heat wave*” OR heatwave*) AND (“social bee*” OR honeybee* OR “honey bee*” OR bumblebee* OR “bumble bee*” OR “stingless bee*”). This search yielded 1,013 results. We then repeated this search but replaced the social bee search terms with solitary bee search terms, using the topic search string (warming OR warmed OR heating OR heated OR “heat shock*” OR heatshock* OR “heat wave*” OR heatwave*) AND (“solitary bee*” OR “nonsocial bee*” OR “non-social bee*”), which returned 86 results.
We next constructed search strings that focused on either heat shocks or heat waves and either social or solitary bees. First, focusing on heat shocks, a topic search for (“heat shock*” OR heatshock*) AND (“social bee*” OR honeybee* OR “honey bee*” OR bumblebee* OR “bumble bee*” OR “stingless bee*”) gave 139 results that dealt with social bees. In contrast, the same topic search on solitary bees using the string (“heat shock*” OR heatshock*) AND (“solitary bee*” OR “nonsocial bee*” OR “non-social bee*”) produced 10 results. Second, focusing on heat waves, a topic search for (“heat wave*” OR heatwave*) AND (“social bee*” OR honeybee* OR “honey bee*” OR bumblebee* OR “bumble bee*” OR “stingless bee*”) yielded 37 studies on social bees. Repeating the same search for solitary bees with the string (“heat wave*” OR heatwave*) AND (“solitary bee*” OR “nonsocial bee*” OR “non-social bee*”) returned 2 results.
In summary, of the 1,099 studies that were detected in our literature search on warming and bees, only 7.8% (86) considered solitary bees in their titles, abstracts, or keywords (Figure 1). Narrowing to heat shocks and heat waves, we found that solitary bees were similarly underrepresented. Only 6.7% of the studies on heat shocks and 5.1% of the studies on heat waves considered solitary bees, amounting to 10 and 2 studies, respectively (Figure 1). Because many terms are used to describe lower-magnitude, longer-term warming, we were not able to isolate studies on bee responses to sustained warming specifically, but of the 1,099 studies identified in our first two broad searches on all types of warming, 911 did not consider heat shocks or heat waves, meaning that 82.9% of studies dealt with less-extreme warming. Thus, the vast majority of studies on bee responses to warming do not address heat shocks or heat waves.
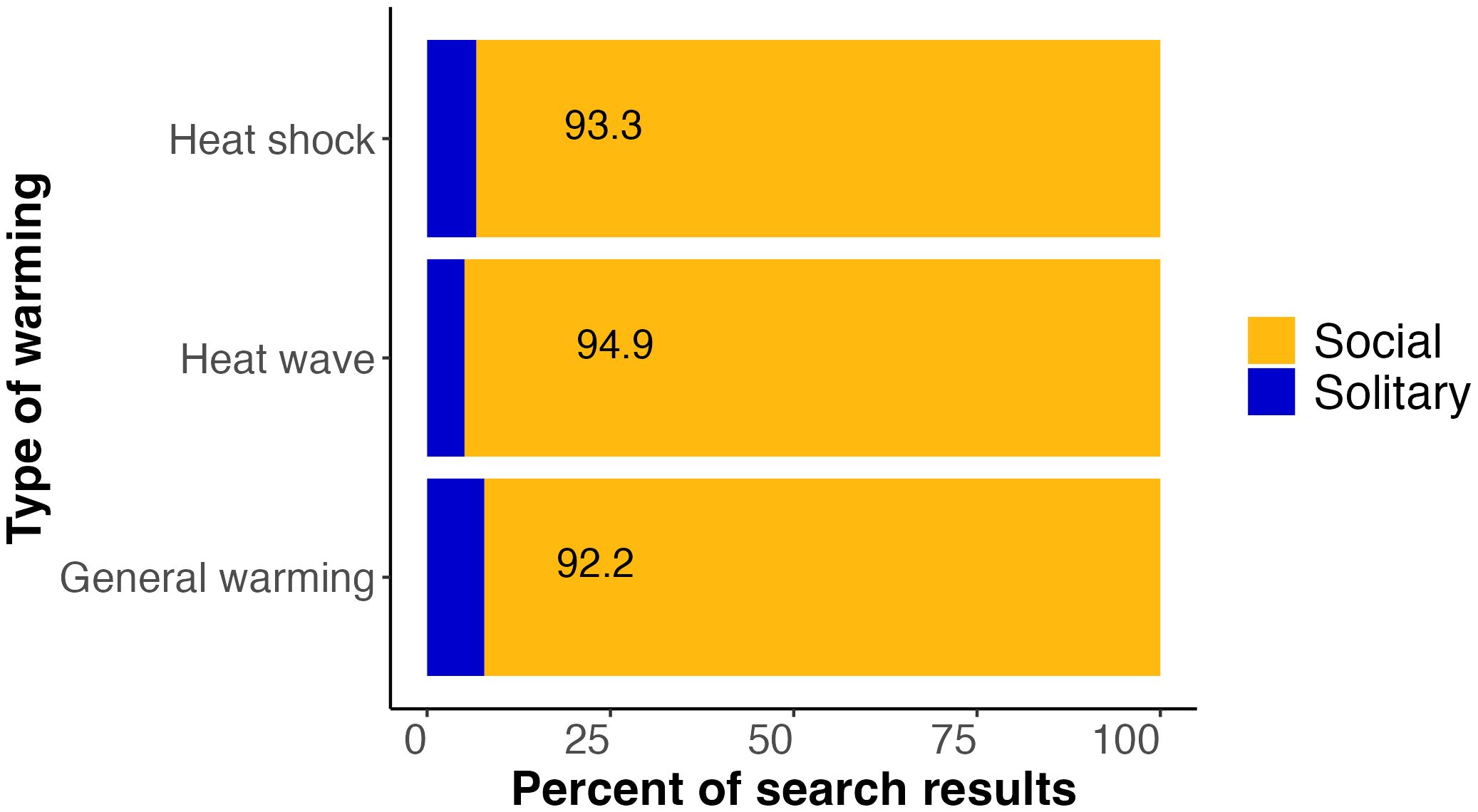
Figure 1 Bar plot showing the percent of literature search results for three different categories of warming (heat shock, heat wave, and general warming) that included terms related to social (orange) or solitary (blue) bees in the titles, abstracts, or author keywords. Across the three types of warming, 5.1–7.8% of the studies identified by these searches involved solitary bees. Detailed information on search terms is provided in the text.
In the sections that follow, we summarize the main findings of the studies identified in our literature search that pertain to solitary bee responses to heat shock, heat waves, and sustained warming. As this is a mini-review rather than a systematic review, our goal is to highlight key findings across different types of warming, rather than to exhaustively review all studies.
3 Heat shocks
Of the studies identified by our literature survey, all those that directly applied heat shock focused on species of Megachile (Megachilidae), examining the effects of heat shock at various developmental stages. In M. rotundata, work has involved heat shock at the prepupal, pupal, and adult stages, with documented consequences for gene expression and life history traits. In M. apicalis, the effects of heat shock were similarly documented at the genetic level and in terms of survival across developmental stages. We first summarize the findings for M. rotundata before turning to those for M. apicalis.
A study focused on M. rotundata pupae examined the expression of heat shock protein genes following a 1-hour heat shock at 40°C in the lab (Yocum et al., 2005). Transcript expression screens indicated that certain heat shock proteins were strongly upregulated in heat-shocked pupae post-diapause (Yocum et al., 2005). Relatedly, possible differential upregulation of heat shock protein genes was hypothesized to underlie differences in adult M. rotundata survival following a 1-hour heat shock at 50°C in the lab (Hayes and López-Martínez, 2021). Timing of the heat shock was an important predictor of starvation survival, with bees shocked in the morning surviving longer than bees shocked in the afternoon, possibly because preparatory stress responses follow a circadian rhythm. Life stage was also an important predictor of response to heat shock. Overall, prepupae and pupae tolerated heat shock, whereas adults had reduced activity, shorter lifespans, and elevated risk of mortality. Indeed, for adult male bees the mortality rate was 80% during the 3 weeks following the heat shock treatment, whereas none of the control bees died in that time. In contrast, adult female bees that were heat-shocked showed no difference in mortality rates compared to control bees. The reasons underlying this difference in male and female mortality rates are not well-understood in M. rotundata, but the greater sensitivity of males to heat shock could translate into altered sex ratios. Similarly, a slightly less severe 1-hour heat shock at 45°C delayed adult male emergence but did not delay female emergence. Together, these findings point to life stage-specific and sex-specific heat shock effects in M. rotundata, with adult males exposed to afternoon heat likely being the most vulnerable (Hayes and López-Martínez, 2021).
In M. apicalis, nest site preferences, stress responses, and offspring survival rates were studied in the context of heat shock in a field setting (Hranitz et al., 2009). Artificial nesting cavities were placed in the field and oriented such that they were either exposed to direct sunlight (south-facing) or shaded (north-facing), with maximum temperatures within nests approaching 50°C during the hottest 2–3 hours of the day in the exposed treatment. Females preferentially nested in the shaded cavities, where the rate of nest failure was lower (8.9% vs. 23.2%). Indeed, the exposed cavities induced stress responses in the form of elevated heat shock protein concentrations across offspring developmental stages, especially eggs. Although offspring survival did not differ between exposed and shaded nests, a greater proportion of offspring died for unexplained reasons in the exposed cavities, while a greater proportion of larvae died due to parasitoids in the shaded cavities. Thus, heat shock can induce physiological stress responses in offspring within semi-natural nesting cavities and can likely result in similar levels of mortality as those imposed by parasitoids in M. apicalis (Hranitz et al., 2009).
4 Heat waves
To the best of our knowledge, the effects of heat waves have been studied in only two taxa of solitary bees, Osmia (Megachilidae) and Xylocopa (Apidae). In Osmia, work has primarily examined how heat waves affect development of larvae and critical thermal maxima of adults, whereas in Xylocopa, work has assessed shifts in critical thermal maxima of adults in response to heat waves. We summarize the findings for Osmia first, then Xylocopa.
Using Osmia lignaria, a lab study subjected eggs and larvae to four different heat wave treatments, with maximum temperatures of either 31 or 37°C, lasting 4 or 7 days (Melone et al., 2024). Although heat wave duration did not affect mortality, eggs and larvae exposed to heat waves of 37°C had higher mortality than those exposed to heat waves of 31°C, which in turn did not differ in mortality rates compared to larvae maintained at the control temperature (25°C). Similarly, larval development time was slowed by 37°C heat waves in comparison to the control treatment, whereas 31°C heat waves did not affect development time. Finally, for bees that completed larval development and spun cocoons, heat wave treatment did not translate into any effects on adult emergence probability. Thus, magnitude rather than duration of warming was key to explaining the larval responses of this cavity-nesting species to heat waves, indicating that even shorter-term episodes of temperatures that exceed the optimum temperature range by as little as 6°C can cause mortality of immature life history stages (Melone et al., 2024).
Although not identified in our literature search, likely because the term “extreme heating event” is used in place of heat wave, another lab study examined heat wave responses in adult females of both O. lignaria and O. cornifrons (Porras et al., 2023). Specifically, critical thermal maxima were measured after bees were warmed to approximately 30°C and held at that temperature for approximately 1 day. In both species, the critical thermal maxima of female bees previously subjected to the heat wave treatment were reduced by several degrees on average, suggesting that exposure to heat waves can erode thermal tolerances in these species, exacerbating their vulnerability to near-future warming events (Porras et al., 2023).
A larger lab study that exposed six bee species to heat waves included two species of solitary bees, Xylocopa violacea and X. olivieri (Gonzalez et al., 2024). Although both Xylocopa species had higher critical thermal maxima than the other, smaller species in the study, neither maxima increased when adult bees were exposed to 32°C for 2 days. Thus, bees did not acclimate by this important metric when warmed to a moderate temperature that, although more than 10°C below the critical thermal maxima of either species, represents a 5°C increase above mean summer temperatures for these wild-caught bees. These findings indicate critical thermal maxima do not respond plastically to heat wave conditions in these solitary species, suggesting that similar warming events will force bees to rely on behavioral mechanisms to avoid overheating (Gonzalez et al., 2024).
5 Sustained warming
Experimental sustained warming of solitary bees has been implemented in several studies, especially those focusing on agriculturally important bee genera, such as Osmia and Megachile. Because these studies encompass a wide array of lower-magnitude, longer-term warming treatments, rather than detailing the methods of each study, we outline some of the key findings, largely organized by species.
In a field experiment focused on O. ribifloris, nest boxes were painted black or white to promote passive warming or cooling, respectively (CaraDonna et al., 2018). Bees that developed within the warmed nests emerged with lower body mass and fat content, likely due to elevated metabolism, and experienced 30–73% mortality, whereas bees that developed in the cooler nests experienced <4% mortality. Warmed bees also emerged later, which could reduce synchrony with floral resources. Ultimately, such high mortality rates could lead to extirpation of populations from the warmest parts of the distribution (CaraDonna et al., 2018).
In O. bicornis (synonym O. rufa), offspring survival decreased by about 30% in experimentally warmed nests in the field primarily due to egg mortality, and females preferentially selected nest sites with more moderate temperatures. For adults that successfully completed development within warmed nests, sustained warming led to weight loss in both sexes (Ostap-Chec et al., 2021). Another study that imposed sustained warming on O. bicornis nests in the field found warming (in combination with low flower abundance) was associated with reduced female body size but increased male body size, as well as a male-biased sex ratio that likely reflects greater female investment in the offspring sex that requires fewer resources. Although the reasons for larger male size with warming are not understood, larger males may have reduced mating success, and possible consequences of smaller female size include reduced reproductive output (Zaragoza-Trello et al., 2021). In a lab setting, the duration of almost all developmental phases (except prepupal) decreased with elevated temperatures in O. bicornis, as did adult survival rates (Giejdasz and Fliszkiewicz, 2016). Additionally, rearing O. bicornis at constant high temperatures vs. naturally fluctuating temperatures in the lab resulted in high mortality of adults (up to 100%), likely because sustained warming elevated their metabolic rates and exhausted their energy reserves (Radmacher and Strohm, 2011).
A lab study that exposed O. lignaria to elevated temperatures throughout development (from egg to adult) found that development time was shortened by 50% under the warmest conditions (Bosch and Kemp, 2000). In a follow-up study that imposed constant warmer temperatures only after cocoon completion, development time was similarly generally reduced, and surprisingly longevity of adult females increased slightly under the warmest treatment (Kemp and Bosch, 2005). A lab study focused on experimentally elevated overwintering temperatures for O. lignaria documented early emergence, increased mortality, greater fat body depletion, and decreased longevity in response to warming (Bosch and Kemp, 2003). Finally, in a greenhouse study sustained warming of adult O. lignaria during the nesting season resulted in bees spending less time foraging, making fewer, shorter visits to flowers, and being more generalized in their foraging behavior (de Manincor et al., 2023).
Several species of Osmia and Megachile were subjected to temperatures projected under continued climate change in a lab study exploring the effects of changes in fall duration and spring onset in overwintering solitary bees (Slominski and Burkle, 2019). Pre-emergence weight loss was associated with temperature treatment in bees of both genera but tended to increase with warming in Osmia while decreasing with warming in Megachile. Post-emergence lifespan was reduced by 1–2 days in male and by 1.5 days in female Osmia due to sustained warming, but this effect depended on the timing of spring onset and the duration of cooler temperatures in the fall. In contrast, Megachile post-emergence lifespan was longer in the warm temperature treatments. The disparate findings for these two genera may relate to a key difference in their life histories; whereas Megachile overwinter as prepupae, Osmia overwinter as adults, and therefore may be likely to exhaust their energy reserves before emerging (Slominski and Burkle, 2019).
Altogether, these findings suggest sustained warming will have varied, largely negative effects on these solitary bee taxa. At the same time, study- and species-specific responses point to the need for meta-analytic and trait-based syntheses of solitary bee responses to increases in global mean temperatures.
6 Discussion
In grouping studies by the magnitude and duration of warming, our review indicates that a warmer climate with more frequent and intense intervals of heat will likely negatively affect solitary bees physiologically and behaviorally (Figure 2). First, we found that the effects of heat shocks on solitary bees have been studied solely in Megachilid bees and cause upregulation of heat shock protein genes in immature stages and increased mortality in adult male bees (Yocum et al., 2005; Hranitz et al., 2009; Hayes and López-Martínez, 2021). Second, we found few studies on solitary bee responses to heat waves, but the limited evidence available indicates these events cause higher mortality and slower development in larvae (Melone et al., 2024). Further, not only may solitary bees fail to acclimate by increasing their critical thermal maxima but also their thermal tolerances may be reduced by heat waves (Gonzalez et al., 2024; Porras et al., 2023). Third, our survey revealed that of the three types of warming we considered, sustained warming has been relatively well-studied in solitary bees across developmental stages. Multiple studies found that sustained warming can accelerate development and result in lower body mass, likely as fat bodies are depleted, ultimately elevating mortality (Bosch and Kemp, 2003; Radmacher and Strohm, 2011; CaraDonna et al., 2018). Changes to solitary bee foraging behavior are also likely, with direct consequences for pollination success (de Manincor et al., 2023). Altogether, the available empirical data on these different types of warming indicate negative fitness consequences that span life history stages.
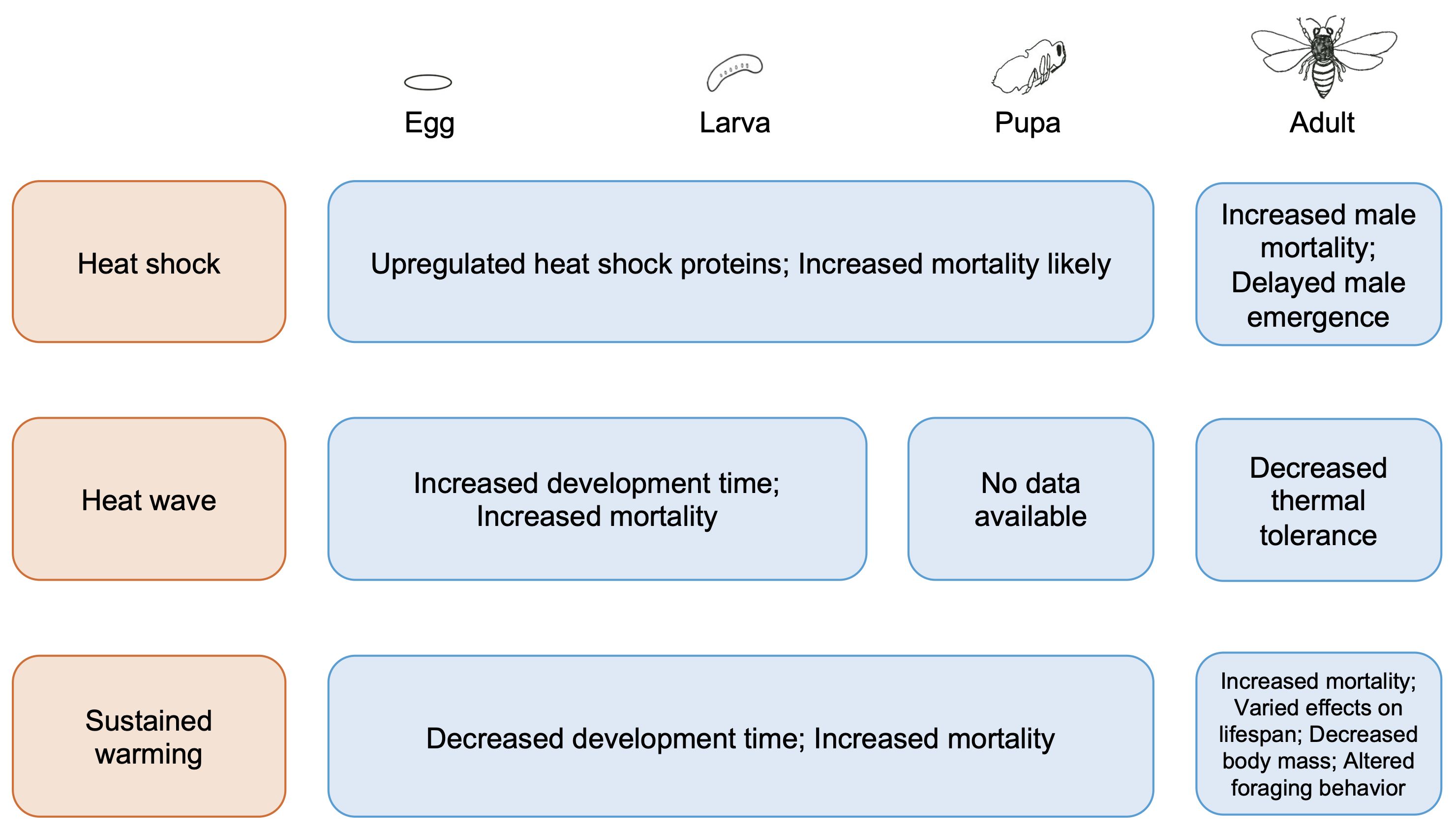
Figure 2 Summary of the direct effects of heat shocks, heat waves, and sustained warming on solitary bees at various life stages (egg, larva, pupa, and adult).
Our review points to several gaps in our understanding of how continued climate warming will affect solitary bees. First, we identified a need to broaden the assessment of heat shock to other solitary taxa beyond the two species of Megachile that, to the best of our knowledge, represent the only studies to date. A second area where more research is needed is in understanding the effects of heat waves on solitary bees, particularly in a field setting. Heat waves have increased in frequency and intensity in most land areas over the past several decades and are projected to continue to intensify with greater levels of global warming (IPCC, 2023). The few lab-based studies we have identified here suggest that heat waves may have disastrous effects on solitary bees, potentially causing high levels of larval mortality (Melone et al., 2024).
Our review also suggests a pattern of studying the effects of heat shocks, heat waves, and warming in general on social, colony-forming bees, which are unlikely to reflect the responses of solitary bees. For example, although the nests of some social bees such as Bombus species (Apidae) may be more vulnerable to warming than previously realized (Kevan et al., 2024), many social bees (e.g., Apis species [Apidae]) rely on workers to behaviorally moderate nest temperatures (Jones et al., 2004). However, behavioral thermoregulation of nests does not occur in solitary bees, potentially leaving their offspring especially defenseless to extreme warming.
Lastly, many of the studies we reviewed involved bees used in commercial pollination, likely due to the relative ease of rearing and experimentally manipulating managed bees. Thus, although we acknowledge the value of using agriculturally important pollinators for climate change research, we have much to discover about the effects of warming on truly wild solitary bees.
Taking a longer-term view from observational field studies that encompass multiple generations, there is evidence that traits such as body size and nesting habit will interact with climate warming to influence bee population dynamics and community composition. For example, trait-based studies indicate that small-bodied bee species may increase in abundance under warmer temperatures (Pardee et al., 2022; Maihoff et al., 2023). In contrast, large-bodied, comb-building cavity-nesting bees are expected to decline in relative abundance under long-term climate warming (Pardee et al., 2022). Finally, a trait-based analysis conducted in the context of urban environments that can act as heat islands suggests that both solitary and cavity-nesting bees are likely to decline under future warming (Hamblin et al., 2017). These longer-term projections, together with the effects of the types of warming we reviewed, point to major perturbations to wild bee ecology and suggest key functional roles may be lost from bee communities with climate change.
In conclusion, there is a pressing need to improve our understanding of how solitary bees, particularly unmanaged solitary bees, will be affected by warming of various magnitudes and durations. An important step will be to incorporate greater complexity and realism in our study of bee thermal ecology, a goal that can help to reveal the emergent effects of warming on bees as parts of ecological communities. As we approach a future in which bees are faced with heat shocks, heat waves, and sustained warming, concurrently with drought and other environmental stressors, this basic information is critical to informing conservation of wild solitary bees and the valuable pollination services they provide.
Author contributions
KV-R: Conceptualization, Investigation, Writing – original draft, Writing – review & editing. NR: Conceptualization, Investigation, Supervision, Writing – original draft, Writing – review & editing.
Funding
The author(s) declare financial support was received for the research, authorship, and/or publication of this article. This work was supported by USDA NIFA AFRI Postdoctoral Fellowship Award #2022-67012-36737 to KV-R.
Acknowledgments
We thank Margaret Mayfield for the invitation to contribute to this article collection. We also thank two reviewers for comments that greatly improved this manuscript.
Conflict of interest
The authors declare that the research was conducted in the absence of any commercial or financial relationships that could be construed as a potential conflict of interest.
Publisher’s note
All claims expressed in this article are solely those of the authors and do not necessarily represent those of their affiliated organizations, or those of the publisher, the editors and the reviewers. Any product that may be evaluated in this article, or claim that may be made by its manufacturer, is not guaranteed or endorsed by the publisher.
References
Arnell N. W., Lowe J. A., Challinor A. J., Osborn T. J. (2019). Global and regional impacts of climate change at different levels of global temperature increase. Clim. Change 155, 377–391. doi: 10.1007/s10584-019-02464-z
Barriopedro D., García-Herrera R., Ordóñez C., Miralles D. G., Salcedo-Sanz S. (2023). Heat waves: Physical understanding and scientific challenges. Rev. Geophys. 61, e2022RG000780. doi: 10.1029/2022RG000780
Bosch J., Kemp W. P. (2000). Development and emergence of the orchard pollinator Osmia lignaria (Hymenoptera: Megachilidae). Environ. Èntomol. 29, 8–13. doi: 10.1603/0046-225X-29.1.8
Bosch J., Kemp W. P. (2003). Effect of wintering duration and temperature on survival and emergence time in males of the orchard pollinator Osmia lignaria (Hymenoptera: Megachilidae). Environ. Entomol. 32, 711–716. doi: 10.1603/0046-225X-32.4.711
CaraDonna P. J., Cunningham J. L., Iler A. M. (2018). Experimental warming in the field delays phenology and reduces body mass, fat content and survival: Implications for the persistence of a pollinator under climate change. Funct. Ecol. 16, 1206–1212. doi: 10.1111/1365-2435.13151
Chen Y., Moufouma-Okia W., Masson-Delmotte V., Zhai P., Pirani A. (2018). Recent progress and emerging topics on weather and climate extremes since the Fifth Assessment Report of the Intergovernmental Panel on Climate Change. Annu. Rev. Environ. Resour. 43, 35–59. doi: 10.1146/annurev-environ-102017-030052
Danforth B. N., Minckley R. L., Neff J. L. (2019). The Solitary Bees: Biology, Evolution, Conservation (Princeton, New Jersey, USA: Princeton University Press). doi: 10.1515/9780691189321
de Manincor N., Fisogni A., Rafferty N. E. (2023). Warming of experimental plant–pollinator communities advances phenologies, alters traits, reduces interactions and depresses reproduction. Ecol. Lett. 26, 323–334. doi: 10.1111/ele.14158
Eilers E. J., Kremen C., Greenleaf S. S., Garber A. K., Klein A.-M. (2011). Contribution of pollinator-mediated crops to nutrients in the human food supply. PloS One 6, e21363. doi: 10.1371/journal.pone.0021363
Giejdasz K., Fliszkiewicz M. (2016). Effect of temperature treatment during development of Osmia rufa L., on mortality, emergence and longevity of adults. J. Apic. Sci. 60, 221–232. doi: 10.1515/jas-2016-0029
Gonzalez V. H., Herbison N., Perez G. R., Panganiban T., Haefner L., Tscheulin T., et al. (2024). Bees display limited acclimation capacity for heat tolerance. Biol. Open 13, bio060179. doi: 10.1242/bio.060179
Goulson D., Nicholls E., Botías C., Rotheray E. L. (2015). Bee declines driven by combined stress from parasites, pesticides, and lack of flowers. Science 347, 1255957. doi: 10.1126/science.1255957
Hamblin A. L., Youngsteadt E., López-Uribe M. M., Frank S. D. (2017). Physiological thermal limits predict differential responses of bees to urban heat-island effects. Biol. Lett. 13, 20170125. doi: 10.1098/rsbl.2017.0125
Hayes T., López-Martínez G. (2021). Resistance and survival to extreme heat shows circadian and sex-specific patterns in A cavity nesting bee. Curr. Res. Insect Sci. 1, 100020. doi: 10.1016/j.cris.2021.100020
Hegland S. J., Nielsen A., Lázaro A., Bjerknes A.-L., Totland Ø. (2009). How does climate warming affect plant-pollinator interactions? Ecol. Lett. 12, 184–195. doi: 10.1111/j.1461-0248.2008.01269.x
Herrera C. M. (1995). Floral biology, microclimate, and pollination by ectothermic bees in an early-blooming herb. Ecology 76, 218–228. doi: 10.2307/1940644
Hranitz J. M., Barthell J. F., Thorp R. W., Overall L. M., Griffith J. L. (2009). Nest site selection influences mortality and stress responses in developmental stages of MegaChile apicalis Spinola (Hymenoptera: Megachilidae). Environ. Èntomol. 38, 484–492. doi: 10.1603/022.038.0223
IPCC (2018). Annex I: Glossary [Matthews, J.B.R. (ed.)]. In: Global Warming of 1.5°C. An IPCC Special Report on the impacts of global warming of 1.5°C above pre-industrial levels and related global greenhouse gas emission pathways, in the context of strengthening the global response to the threat of climate change, sustainable development, and efforts to eradicate poverty. Eds. Masson-Delmotte V., Zhai P., Pörtner H.-O., Roberts D., Skea J., Shukla P. R., Pirani A., Moufouma-Okia W., Péan C., Pidcock R., Connors S., Matthews J. B. R., Chen Y., Zhou X., Gomis M. I., Lonnoy E., Maycock T., Tignor M., Waterfield T. (Cambridge, UKand New York, NY, USA: Cambridge University Press), 541–562. doi: 10.1017/9781009157940.008
IPCC (2023). Climate Change 2023: Synthesis Report. Contribution of Working Groups I, II and III to the Sixth Assessment Report of the Intergovernmental Panel on Climate Change. Eds. Team C. W., Lee H., Romero J. (Geneva, Switzerland: IPCC), 35–115. doi: 10.59327/IPCC/AR6–9789291691647
Jones J. C., Myerscough M. R., Graham S., Oldroyd B. P. (2004). Honey bee nest thermoregulation: Diversity promotes stability. Science 305, 402–404. doi: 10.1126/science.1096340
Kemp W. P., Bosch J. (2005). Effect of temperature on Osmia lignaria (Hymenoptera: Megachilidae) prepupa–adult development, survival, and emergence. J. Econ. Entomol. 98, 1917–1923. doi: 10.1093/jee/98.6.1917
Kevan P. G., Rasmont P., Martinet B. (2024). Thermodynamics, thermal performance and climate change: temperature regimes for bumblebee (Bombus spp.) colonies as examples of superorganisms. Front. Bee Sci. 2. doi: 10.3389/frbee.2024.1351616
Klein A. M., Vaissiere B. E., Cane J. H., Steffan-Dewenter I., Cunningham S. A., Kremen C., et al. (2007). Importance of pollinators in changing landscapes for world crops. Proc. R. Soc. B Biol. Sci. 274, 303–313. doi: 10.1098/rspb.2006.3721
Lewis S. C., King A. D. (2017). Evolution of mean, variance and extremes in 21st century temperatures. Weather Clim. Extremes 15, 1–10. doi: 10.1016/j.wace.2016.11.002
Maihoff F., Friess N., Hoiss B., Schmid-Egger C., Kerner J., Neumayer J., et al. (2023). Smaller, more diverse and on the way to the top: Rapid community shifts of montane wild bees within an extraordinary hot decade. Divers. Distrib. 29, 272–288. doi: 10.1111/ddi.13658
Melone G. G., Stuligross C., Williams N. M. (2024). Heatwaves increase larval mortality and delay development of a solitary bee. Ecol. Èntomol. 49, 433–444. doi: 10.1111/een.13317
Ollerton J., Winfree R., Tarrant S. (2011). How many flowering plants are pollinated by animals? Oikos 120, 321–326. doi: 10.1111/j.1600-0706.2010.18644.x
Ostap-Chec M., Kierat J., Kuszewska K., Woyciechowski M. (2021). Red mason bee (Osmia bicornis) thermal preferences for nest sites and their effects on offspring survival. Apidologie 52, 707–719. doi: 10.1007/s13592-021-00858-6
Pardee G. L., Griffin S. R., Stemkovski M., Harrison T., Portman Z. M., Kazenel M. R., et al. (2022). Life-history traits predict responses of wild bees to climate variation. Proc. R. Soc B 289, 20212697. doi: 10.1098/rspb.2021.2697
Porras M. F., Navas C. A., Agudelo-Cantero G. A., Santiago-Martínez M. G., Loeschcke V., Sørensen J. G., et al. (2023). Extreme heat alters the performance of hosts and pathogen. Front. Ecol. Evol. 11. doi: 10.3389/fevo.2023.1186452
Radmacher S., Strohm E. (2011). Effects of constant and fluctuating temperatures on the development of the solitary bee Osmia bicornis (Hymenoptera: Megachilidae). Apidologie 42, 711–720. doi: 10.1007/s13592-011-0078-9
Scaven V. L., Rafferty N. E. (2013). Physiological effects of climate warming on flowering plants and insect pollinators and potential consequences for their interactions. Curr. Zool. 59, 418–426. doi: 10.1093/czoolo/59.3.418
Scheffers B. R., Meester L. D., Bridge T. C. L., Hoffmann A. A., Pandolfi J. M., Corlett R. T., et al. (2016). The broad footprint of climate change from genes to biomes to people. Science 354, aaf7671–aaf7671. doi: 10.1126/science.aaf7671
Sears M. W., Angilletta M. J. (2011). Introduction to the symposium: Responses of organisms to climate change: A synthetic approach to the role of thermal adaptation. Integr. Comp. Biol. 51, 662–665. doi: 10.1093/icb/icr113
Sears M. W., Raskin E., Angilletta M. J. (2011). The world is not flat: Defining relevant thermal landscapes in the context of climate change. Integr. Comp. Biol. 51, 666–675. doi: 10.1093/icb/icr111
Slominski A. H., Burkle L. A. (2019). Solitary bee life history traits and sex mediate responses to manipulated seasonal temperatures and season Length. Front. Ecol. Evol. 7. doi: 10.3389/fevo.2019.00314
Wei N., Kaczorowski R. L., Arceo-Gómez G., O’Neill E. M., Hayes R. A., Ashman T.-L. (2021). Pollinators contribute to the maintenance of flowering plant diversity. Nature 597, 688–692. doi: 10.1038/s41586-021-03890-9
Yocum G. D., Kemp W. P., Bosch J., Knoblett J. N. (2005). Temporal variation in overwintering gene expression and respiration in the solitary bee MegaChile rotundata. J. Insect Physiol. 51, 621–629. doi: 10.1016/j.jinsphys.2004.11.008
Keywords: climate change, foraging, nesting, pollination, pollinators, thermal ecology
Citation: Vilchez-Russell KA and Rafferty NE (2024) Effects of heat shocks, heat waves, and sustained warming on solitary bees. Front. Bee Sci. 2:1392848. doi: 10.3389/frbee.2024.1392848
Received: 28 February 2024; Accepted: 20 June 2024;
Published: 03 July 2024.
Edited by:
Peter Kevan, University of Guelph, CanadaReviewed by:
Victor Gonzalez, University of Kansas, United StatesMelissa Oddie, Norwegian Beekeepers Association, Norway
Copyright © 2024 Vilchez-Russell and Rafferty. This is an open-access article distributed under the terms of the Creative Commons Attribution License (CC BY). The use, distribution or reproduction in other forums is permitted, provided the original author(s) and the copyright owner(s) are credited and that the original publication in this journal is cited, in accordance with accepted academic practice. No use, distribution or reproduction is permitted which does not comply with these terms.
*Correspondence: Kaleigh A. Vilchez-Russell, a3ZpbGNoZXotcnVzc2VsbEBjb2xsZWdlb2Z0aGVkZXNlcnQuZWR1; Nicole E. Rafferty, bmljb2xlLnJhZmZlcnR5QHVuaW1lbGIuZWR1LmF1
†Present address: Kaleigh A. Vilchez-Russell, Department of Biology, College of the Desert, Palm Desert, CA, United States