- Department of Veterinary Microbiology and Preventive Medicine, Iowa State University, Ames, IA, United States
Supplementation of cattle diets with Saccharomyces cerevisiae fermentation products (SCFP) has been shown to improve health and performance of calves and cows in both the dairy and feedlot. Numerous studies have shown SCFP supplementation is beneficial in the context of production- and infection-related stressors, promoting resilience, accelerated resolution of inflammation or oxidative stress, and enabling the cow or calf to maintain homeostasis. SCFPs, derived from yeast fermentation, encompass a rich array of bioactive compounds, including vitamins, minerals, amino acids, and metabolites, which likely influence the host through both distinct and overlapping processes. Understanding the mechanisms by which SCFPs exert their beneficial effects is crucial for optimizing their utilization in cattle production systems. In this review, we focused not only on the beneficial effects of SCFPs on health and performance but also on their influence on host microbiota, epithelial barrier integrity, and the host immune system, providing mechanistic insights. Previous studies have suggested that SCFPs impact host metabolism, modulate rumen and hindgut microbial populations, exert antioxidant and immunomodulatory effects, and stimulate the expression of genes involved in maintaining tissue barrier integrity. However, there are still gaps in understanding certain mechanistic pathways, particularly those involving the nervous system, as well as the paradoxical effects of SCFPs in enhancing immune responses while simultaneously mitigating excessive inflammation. This review summarizes several recent reports describing the health benefits of SCFP supplementation in cattle and considers the available evidence on the mode of action.
1 Introduction
Saccharomyces cerevisiae fermentation products (SCFP) are a dry feed supplement produced by anaerobic fermentation with S. cerevisiae. Based on consensus definition, SCFP products are considered a postbiotic, a “preparation of inanimate microorganisms and/or their components that confers a health benefit on the host” (Salminen et al., 2021). Among the more than 2,000 species of yeast, most have been reported to have no significant impact on the health of animals or humans. However, specific yeast species such as Saccharomyces cerevisiae, Kluyveromyces marxianus, Candida utilis, and Saccharomyces boulardii have been shown to positively affect animal health (Pang et al., 2022). Notably, Saccharomyces cerevisiae has been the most extensively used yeast in animal production and nutrition, owing to its safety, stability, extensive research evidence, and its applicability to a wide range of livestock species (Elghandour et al., 2020; Parapouli et al., 2020; Fernández-Pacheco et al., 2021; Pang et al., 2022). Postbiotics are comprised of microbial remnants including cell walls and other cellular contents, the culture matrix and metabolites or secreted products from the fermentation process (Salminen et al., 2021). Thus, they differ from probiotics, which are live microorganisms, and prebiotics, which are substrates that are utilized by host microorganisms to support host health. The composition of SCFP specifically is proprietary but is known to include amino acids, antioxidants, polyphenols, and B vitamins, and to a lesser extent, β-glucan and other yeast cell wall components. Supplementation with SCFP has been shown to benefit health and production parameters in cows and other species, including swine (Yan et al., 2024), poultry (Gao et al., 2009), horses (Lucassen et al., 2021), dogs (Lin et al., 2019) and humans (Moyad et al., 2008, 2009, 2010). In the dairy, supplementation with SCFP improved dry matter intake (DMI) in early lactation while decreasing DMI in mid to late lactation (Poppy et al., 2012), increased milk production (Zaworski et al., 2014) and improved the outcome of several health challenges, including subacute ruminal acidosis (SARA) (Guo et al., 2022), heat stress (Al-Qaisi et al., 2020), respiratory disease (Mahmoud et al., 2020; McDonald et al., 2021), mastitis (Vailati-Riboni et al., 2021) and digital dermatitis (Anklam et al., 2022). In beef cattle, supplementing with SCFP positively impacted feed efficiency and improved total tract digestibility (Deters and Hansen, 2019), while exerting positive effects on health challenges such as liver abscesses and SARA (Shen et al., 2019). Because postbiotics such as SCFP products are a mixture of bioactive substances, they likely benefit the host through many different mechanisms. The objective of this review is to summarize recent literature regarding the benefits of SCFP supplementation in cattle during both healthy and disease conditions, and to consider possible mechanisms of action which contribute to the observed effects. The scope of this article is recent literature describing health-related effects of SCFP supplementation on cattle.
2 Beneficial effects of SCFP supplementation on health and performance in dairy and beef cattle
Supplementation with SCFP has shown benefits in multiple bovine models of stress or infection (Table 1; Figure 1). In adult dairy cows receiving SCFP, clinical disease signs are reduced in the context of both digital dermatitis (DD) and mastitis (Ferguson et al., 2018; Vailati-Riboni et al., 2021; Anklam et al., 2022). DD is an infectious disease that causes ulcerative and necrotizing foot lesions. The condition is extremely painful and a major welfare concern that leads to additional complications such as reduced milk production, reduced reproductive rates and premature culling (Evans et al., 2016). Anklam et al. conducted a study at a commercial dairy farm using more than 900 cows, with half receiving SCFP supplementation (Anklam et al., 2022). Cows receiving SCFP had almost 2 times lower odds ratio of developing infectious, active digital dermatitis lesions (M2M2P lesions) compared to control cows. Of those cows that did develop active lesions, control cows progressed 2.2 times faster than SCFP fed cows (Anklam et al., 2022). Additional approaches to addressing the development of DD and limiting pathogen spread within a herd can have profound effects due to the increasing risk of antibiotic resistance of pathogens and multi-pathogen nature of the disease (Wilson-Welder et al., 2015).
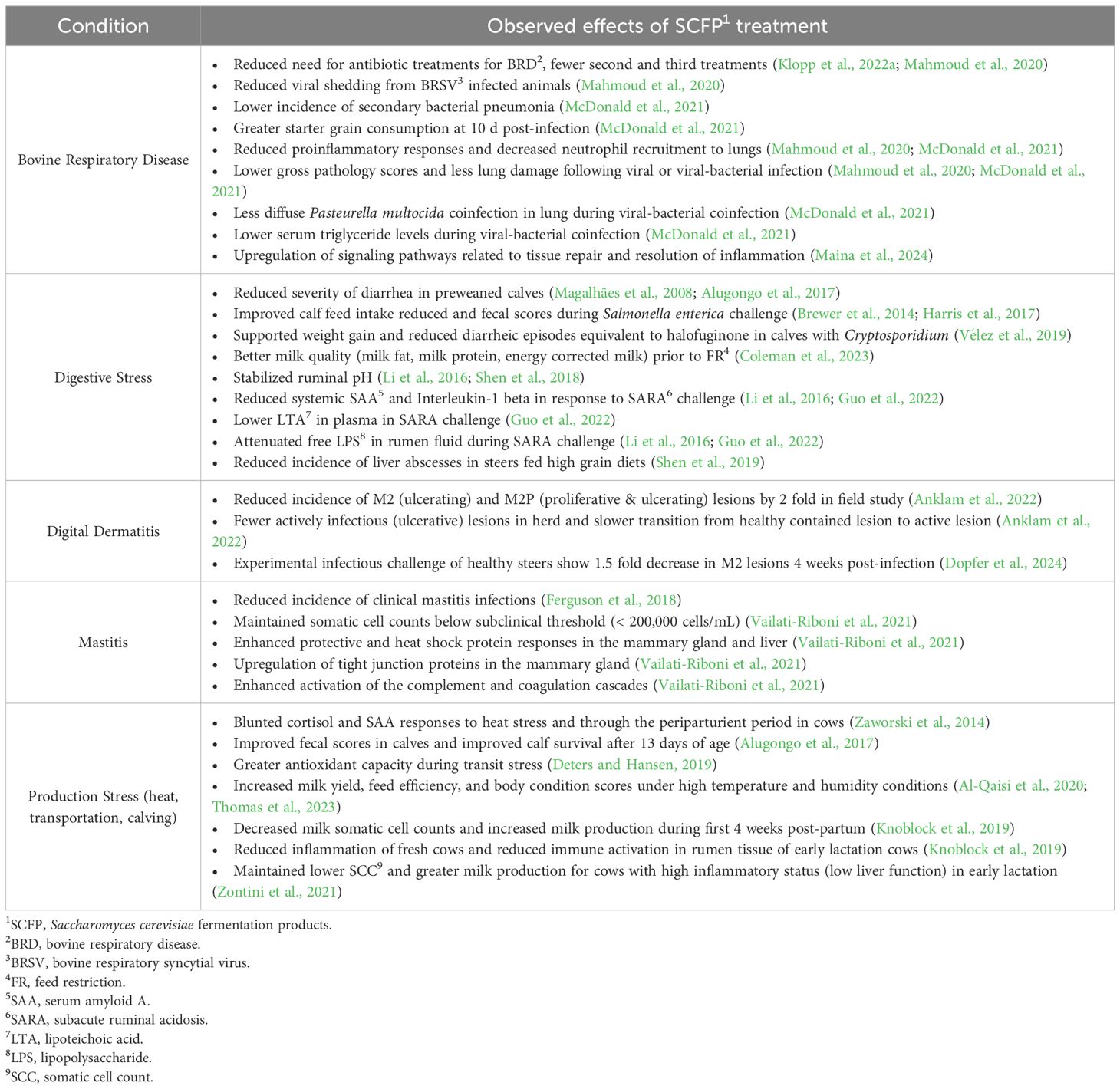
Table 1. Summary of the benefits of SCFP supplementation in cows and calves responding to various disease and production-related stressors.
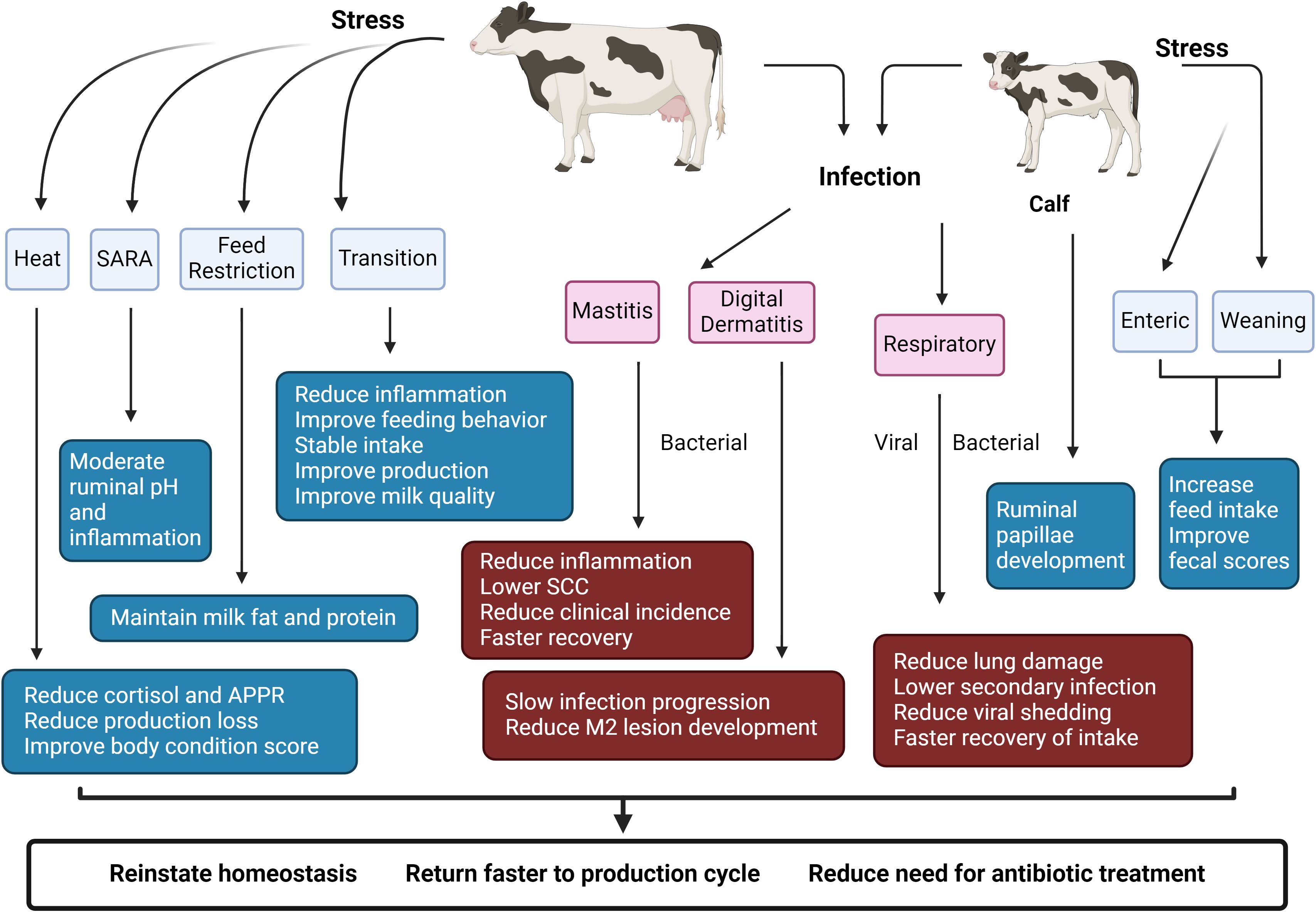
Figure 1. Beneficial effects of SCFP supplementation on cow and calf health. SCFP supplementation promotes faster recovery and increase resiliency against infectious and production-related stressors in adult cattle and young calves. Benefits of SCFP supplementation have been observed in calves experiencing diarrhea and respiratory infections, and in cows with mastitis and digital dermatitis. Supplementation with SCFP has also improved animal performance and health in the context of heat stress and feed restriction, as well as during subacute ruminal acidosis (SARA). SCC: somatic cell count. APR: acute phase response. Created with BioRender.com.
Mastitis is one of the most important diseases in the dairy industry, adversely impacting milk quality and milk yield, and resulting in losses due to premature culling, treatment and prevention costs and discarded milk. Yearly prevention costs to control mastitis have been estimated as high as $100 per cow in 2016 (van Soest et al., 2016; Aghamohammadi et al., 2018), while one recent estimate calculated the cost of a clinical mastitis case to be $581 per cow (Rodriguez et al., 2024). In a subclinical Streptococcus uberis mastitis challenge, Vailati-Riboni et al. demonstrated that SCFP supplemented cows had lower somatic cell scores, and lower temperatures in the infected quarter compared to control cows (Vailati-Riboni et al., 2021). Notably, by 30 hours post-challenge, cows fed with SCFP had somatic cell counts in their milk that were below the subclinical mastitis threshold (179,415 cells/mL), whereas the control group had much higher counts (1,076,592 cells/mL), indicating active mastitis (Vailati-Riboni et al., 2021). In a large-scale trial of SCFP products in 25 commercial herds, which included cows at all stages of lactation, supplementation with SCFP reduced the incidence of mastitis and reduced linear scores (Ferguson et al., 2018).
Beneficial effects of SCFP supplementation have also been observed in the context of respiratory disease. Bovine respiratory disease (BRD) negatively impacts both the feedlot and dairy industries. In one recent estimate, the cost of raising replacement dairy heifers was increased by $282 per BRD incident occurring in the first 120 days of life (Overton, 2020). In the feedlot, a mortality case of BRD cost producers a net average of $1072 USD per case, while animals requiring multiple BRD treatments returned an average of $250 less than animals not requiring treatment. Calves that were supplemented with SCFP beginning at 1-2 days of age and then infected at 3 weeks of age with bovine respiratory syncytial virus (BRSV) developed fewer gross lung lesions and a lower incidence of secondary bacterial infections compared to untreated controls (Mahmoud et al., 2020). Calves also shed less virus compared to control calves, resulting in a reduced risk of transmitting the virus to pen mates (Mahmoud et al., 2020). In a follow-up study, experimental coinfection with BRSV and Pasteurella multocida again resulted in less lung involvement in SCFP fed calves compared to control calves (McDonald et al., 2021). The effects of SCFP supplementation to reduce lung pathology, and thus maintain better lung capacity, may have both short and long-term implications on performance (Buczinski et al., 2021). In a recent study evaluating a group of 60 Holstein bull calves through 4 months of age, Klopp et al. observed that SCFP fed calves tended to have improved average daily gain post weaning, had increased feed efficiency and required fewer treatments for BRD compared to untreated controls (Klopp et al., 2022a). Thus, SCFP treatment may improve BRD outcomes in both preweaning and postweaning stages.
Supplementation with SCFP also shows benefits in the context of gut health, resulting in faster recovery from experimental or production stressors. The addition of SCFP prior to a feed restriction (FR) period resulted in improvements in yield of milk fat, milk protein, and energy corrected milk with improved feed efficiency and protected against fluctuations during the FR challenge (Coleman et al., 2023). SARA is a costly disease in high-producing dairy and beef cows. The disease results in increased translocation of bacterial components such as lipopolysaccharide (LPS) and lipotechoic acid (LTA) from the rumen into circulation, leading to systemic inflammation and increased risk for production diseases such as laminitis, liver abscesses and overall reduced productivity (Plaizier et al., 2012). SCFP supplementation during grain-based SARA challenges has been shown to reduce the variation in ruminal pH caused by the high-grain challenge, and to reduce the systemic inflammatory and acute phase responses that result from SARA episodes (Li et al., 2016; Guo et al., 2022). Additionally, during the SARA challenge, SCFP supplementation not only demonstrated superior pH regulation effects compared to the antibiotic-treated group (monensin and tylosin) but also elicited enhanced intestinal immune responses (Shen et al., 2018) and showed a level of efficacy in preventing liver abscesses similar to antibiotics, without affecting antibiotic resistance (Shen et al., 2019).
Heat stress is associated with reduced feed intake and systemic inflammatory responses. In cows experiencing experimentally induced heat stress with heat blanket, SCFP supplementation did not impact measures of intake or physiologic effects (rectal temperature, skin temperature or respiration rate), but blunted the acute phase response and fully negated the production of cortisol (Al-Qaisi et al., 2020). In a commercial environment under high temperature and high humidity conditions, SCFP fed multiparous cows produced more milk and all SCFP fed cows exhibited greater feed efficiency and had improved body condition scores compared to untreated controls (Thomas et al., 2023).
In calves raised in a commercial setting, feeding SCFP improves fecal scores both pre and postweaning (Alugongo et al., 2017). Magalhães et al. evaluated the effects of SCFP in more than 500 head of hutch-raised calves and observed improved fecal scores and reduced days with diarrhea compared to untreated controls, as well as an overall improvement in calf survival after 13 days of age (Magalhães et al., 2008). However, not all studies are consistent, and some have observed limited or no differences in calf health or diarrhea incidence with SCFP supplementation (Lesmeister et al., 2004; Pisoni and Relling, 2020). Differences in study outcomes have been attributed to the type of SCFP product used, dose administered, animal ages, sample size or population effects, but it is not clear what differentiates non-responders from responders. Brewer et al. challenged 40 SCFP-fed dairy calves with Salmonella and observed lower rectal temperatures, reduced fecal scores and fewer days of diarrhea (Brewer et al., 2014), as well as less Salmonella shedding. In a later study, Harris et al. also conducted an experimental Salmonella enterica challenge in preweaned calves and observed improved feed intake and a tendency for reduced fecal scores in SCFP fed calves, with a trend of reducing the number of days in fecal shedding of Salmonella (Harris et al., 2017).
In sum, although the effects tend to be somewhat variable depending on study population, challenge type, etc., supplementation with SCFP has shown an array of benefits, improving both cow and calf resilience in the face of stressors and health challenges.
3 Mechanisms of action
Understanding the mechanism of action of postbiotic formulations is critical for determining current product efficacy and for future approaches to improve or modify the activity of postbiotics to benefit animal health. However, because postbiotic composition is complex, there can be multiple mechanisms of action, and these mechanisms may act synergistically or function independently. Thus, discerning a single or isolated mechanism, particularly in the context of in vivo animal trials, can pose challenges. Through a review of the literature encompassing bacterial and yeast based postbiotic efficacy, the International Scientific Association of Probiotics and Prebiotics (ISAPP) has identified five distinct mechanisms of action by which postbiotics contribute to host health (Salminen et al., 2021): 1) modulation of host metabolic responses; 2) impacts on the host microbiota; 3) effects on epithelial barrier integrity; 4) immunomodulation; and 5) impacts on the nervous system. Currently, there is no available evidence of SCFP effects on nervous system signaling, but experimental evidence supports that SCFP can exert health benefits through the other 4 mechanisms. We will consider each of these potential mechanisms in the following sections. Figure 2 also summarizes the observed effects of SCFP supplementation within these four categories.
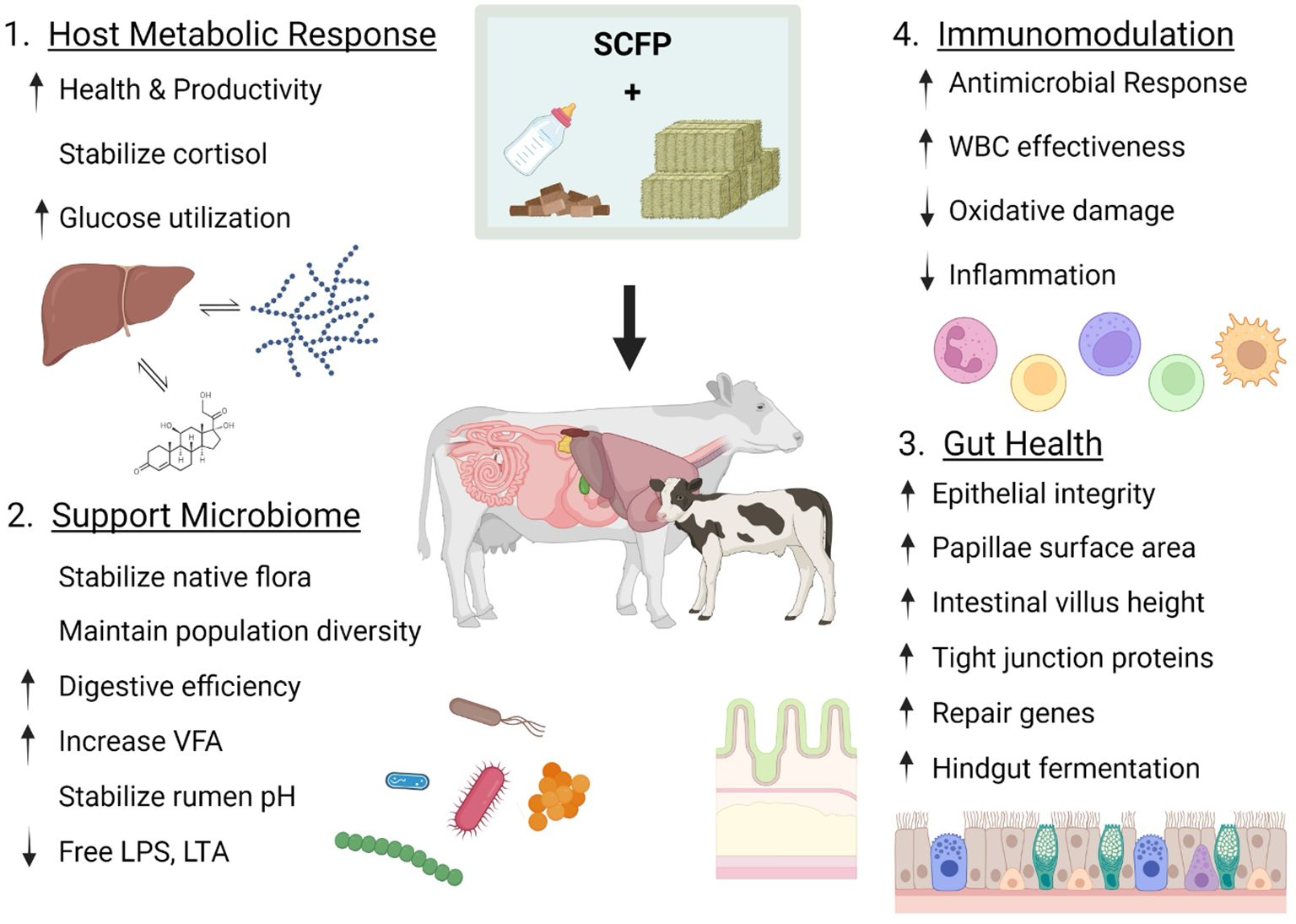
Figure 2. Proposed mechanisms of action of SCFP supplementation in cattle. Supplementation with postbiotic SCFP is proposed to benefit the animal through four mechanisms of action: 1) equilibration of host metabolic responses to stressors, 2) provision of supportive nutrients and cellular material to promote a healthy host microbiota, 3) maintenance of the epithelial integrity and efficient repair of epithelial tissues in barrier sites such as the lung, gut and mammary glands, and 4) modulation of the host immune system to promote efficient antimicrobial protection, reduce inflammation-associated damage and expedite tissue repair and recovery. Created with BioRender.com.
4 Beneficial effects of SCFP supplementation on the modulation of host metabolism
Several studies have evaluated the metabolic effects of SCFP feeding in cattle at various stages, but most research in healthy animals did not demonstrate significant metabolic changes (Irvine et al., 2011; Olagaray et al., 2019). Yuan et al. reported an increase in plasma β-hydroxybutyric acid (BHB) following a quadratic dose effect when SCFP was administered from three weeks before to 42 days after calving (Yuan et al., 2015). Zaworski et al. found that high doses of SCFP (112 g/d) resulted in significantly higher urea nitrogen levels 28 days post-calving compared to lower doses of SCFP (56 g /d) or the control group (Zaworski et al., 2014). Urea is classified as a non-protein nitrogen (NPN) source and contains a much higher concentration of nitrogen compared to proteins. Cattle can efficiently convert urea nitrogen into microbial protein in the rumen, which can be a more efficient process than using dietary protein directly, thus this is beneficial to the cow. Shi et al. also showed that feeding 19 g/d of SCFP from four weeks pre-calving to 4-5 weeks post-calving resulted in higher plasma glucose and lower plasma BHB and free fatty acids concentrations during the post-fresh period (24 d to 44 d), and significant dietary starch interactions during the fresh diet period (Shi et al., 2019). While metabolic changes in healthy cows are rarely reported, various studies have shown that supplementation can mitigate negative alterations in hosts with different disease states or help recover from disease conditions. In the S. uberis mastitis challenge in mid-lactation dairy cows, all blood parameters related to metabolism (glucose, non-esterified fatty acids (NEFA), BHB, cholesterol, urea, and creatinine) exhibited significant circadian concentration fluctuations within the first 36 hours and trends of increasing or decreasing post-biopsy (Vailati-Riboni et al., 2021). However, in animals supplemented with SCFP, an increasing trend in blood urea concentrations was observed, and a significant interaction between SCFP feeding and time was noted in creatinine levels (Vailati-Riboni et al., 2021). Additionally, Zhu et al. (2016) demonstrated that SCFP supplementation in heat-stressed dairy cows led to an increased net energy balance, calculated based on DMI and feed efficiency (milk yield/DMI), even though the supplementation did not alter DMI itself (Zhu et al., 2016).
Several metabolic disturbances in the digestive system, including SARA and FR, have been studied, and it has been observed that they are alleviated by supplementation with SCFP. In a study with differing starch contents, the reduction in rumen pH in the high-starch diet group was mitigated by SCFP supplementation (Shi et al., 2019). Similar findings were observed in another study with dose dependent supplementation of SCFP during a SARA challenge (Khalouei et al., 2020). Cows administered with higher concentration of SCFP (38 g/d) showed lower propionate concentrations, higher acetate to propionate ratios, decreased volatile fatty acid (VFA) levels in the rumen, increased rumen pH, and decreased fecal pH compared to cows receiving lower concentrations of SCFP (19 g/d) and control group. These results suggest the location of fermentation shifts from the rumen to the hindgut with SCFP intake to mitigate the risk of rumen acidosis (Khalouei et al., 2020). Allen and Ying (2012) used ruminally and duodenally cannulated cows to investigate the impacts of SCFP supplementation on starch digestibility. Cows with lower DMI had an increased rate of ruminal starch digestion when supplemented with SCFP, while cows with high DMI had decreased rates of ruminal starch digestion due to SCFP. Thus SCFP supplementation helps stabilize ruminal environment when large amount of starch are consumed to support high performing cows (Allen and Ying, 2012). In another report, during a FR challenge, there were notable reductions in plasma glucose and increases in plasma BHB concentrations, with greater effects seen in those supplemented with SCFP, leading the authors to suggest that these outcomes stemmed from SCFP supplementation enhancing glucose utilization to support immune function (Marins et al., 2023). However, no effects of SCFP treatment on plasma biomarkers in energy metabolism, liver function and inflammation were observed in a different FR trial (Coleman et al., 2023). Given the inconsistent results observed, further research is needed to elucidate the impact of SCFP supplementation on host metabolism. Further, due to their interdependency, it can be very difficult to distinguish between modulation of host metabolism and a change in substrates available to the animal due to upstream effects on the microbiota. Unraveling these individual mechanisms of action will be an important area of future study.
Zontini et al. (2021) conducted a study evaluating the effects of SCFP supplementation not only in specific disease conditions, but also in a general inflammatory state (Zontini et al., 2021). Using a liver functionality index (LFI), determined by profiles of specific blood inflammatory markers in the first month of lactation, the efficacy of SCFP was compared across host inflammation status. While supplementation with SCFP (19 g/day) from 60 days pre-calving to 42 days post-calving did not yield significant effects in the high LFI group (low inflammatory status), in the low LFI group (high inflammatory status), where some inflammation might be present, cows supplemented with SCFP showed a faster recovery of rumination time postpartum, a greater milk production and lower SCC compared to the control group. Additionally, NEFA levels, which were significantly elevated in the control group at 7 days postpartum, resembled those from the high LFI group in the low LFI with SCFP supplementation group (Zontini et al., 2021).
5 Beneficial effects of SCFP supplementation on the host microbiota
Given that cattle rely on symbiotic microbial communities within the gastrointestinal system to utilize dietary nutrients, the microbiota of the gastrointestinal tract is recognized as crucial for cattle health (Plaizier et al., 2018). The microbiota of cattle comprises rumen bacteria, methanogenic archaea, ciliate protozoa, amoebas, fungi, and bacteriophages, with rumen bacteria being the most abundant (Matthews et al., 2019). Due to the amount of forage component of dairy cattle diet, cellulolytic bacteria capable of breaking down cellulose and hemicellulose are crucial (Koike and Kobayashi, 2009). The fermentation by these bacteria and other rumen microbes leads to the production of VFA, including acetate, butyrate, and propionate, which can provide up to 80% of the cattle's total energy requirement (Liu et al., 2021). Additionally, there are pectinolytic bacteria that break down pectin to produce acetate, the most highly produced VFA during bacterial fermentation (Dušková and Marounek, 2001). Furthermore, there are bacteria that either utilize or produce lactate (Liu et al., 2021) that are important in development of ruminal acidosis.
The microbiota necessary for cattle digestion varies with changes in the rumen environment, structure, and the physiological changes of the host, making it crucial to maintain a balance through the interaction between the host and its microbiota. The supplementation of SCFP has been reported to have beneficial effects related to these ruminal environments. SCFP supplementation in dairy calves has been linked to structural growth in digestive organs, evidenced by increased rumen papillae dimensions and improved villus to crypt ratios in the small intestine, potentially enhancing nutrient absorption and intestinal microbial composition (Kaldmäe et al., 2008; Xiao et al., 2016). Also, SCFP supplementation is known to regulate pH by stimulating the growth of lactic acid-utilizing bacteria (Callaway and Martin, 1997) and protozoa that engulf starch granules, thus reducing starch degradation by amylolytic bacteria, which might reduce ruminal pH (Williams and Coleman, 1997). Consequently, changes within the host's digestive system have been reported. Hučko et al. observed an increase in the acetate:propionate ratio and a significant rise in microbial cellulolytic activity in calves (Hučko et al., 2009). Additionally, in high-starch-fed dairy cows during the transition period, SCFP supplementation moderates rumen pH fluctuations, maintains higher nadir pH levels, and shortens periods of low pH (Shi et al., 2019). Furthermore, free bacterial endotoxin (LPS) from gram-negative bacteria and in rumen fluid, which might come from death of bacteria that cannot stand suboptimal ruminal pH (Khafipour et al., 2016), showed a tendency to be reduced after SCFP treatment during moderate SARA challenge (Guo et al., 2022).
Supplementing dairy calves with SCFP has been shown to significantly alter the rumen fluid's microbial composition, notably decreasing Prevotella and increasing Butyrivibrio abundance in 28-day-old dairy calves and enhancing colonization by fibrolytic bacteria (Lachnospiraceae and Ruminococcaceae) in both the rumen and large intestine of 56-day-old dairy calves (Xiao et al., 2016, 2018). Even though these changes did not extend to improvements in calf body weight or average daily gain, the observed changes at 28 days may increase butyrate production. This, in turn, can lead to the development of the forestomach, abomasum, and small intestine, which may ultimately result in enhanced performance (Xiao et al., 2016; Górka et al., 2018; Xiao et al., 2018). In a study by Zhu et al. (2017), SCFP supplementation in lactating cows receiving low-quality forage resulted in improved nitrogen conversion and an increase in total ruminal VFA. Populations of rumen fungi and cellulolytic bacteria (R. flavefaciens and Fibrobacter succinogenes) increased linearly with increasing quantities of SCFP, while lactate-utilizing bacteria (Selenomonas ruminantium and Megaspheara elsdenii) and lactate-producing bacteria (Streptococcus bovis) decreased. These findings suggest that SCFP supplementation positively influenced rumen functionality and increased rumen fermentation efficiency (Zhu et al., 2017).
The capability of different components of SCFP to prevent alterations in the microbiota under stressful production conditions has also been reported. In the study of dairy cows fed a high-grain diet, which leads to decreased pH levels, there was a decrease in the richness and diversity of the rumen microbiota, with alterations in the Firmicutes to Bacteroidetes ratio and an increase in populations of several amylolytic bacteria (Tun et al., 2020). However, the supplementation of SCFP (14 g/d) led to an increase in the populations of major fibrolytic and amylolytic bacteria, ciliate protozoa, and Bifidobacterium spp., mitigating the SARA-related reductions in the richness and diversity of the rumen microbiota, indicating the effects of SCFP supplementation were evident in attenuating the outcomes of SARA challenges (Tun et al., 2020). Additionally, supplementation of SCFP (19 g/d) in intestinal barrier challenge by 40% DMI FR showed the greater abundance of R. flavefaciens and F. succinogenes, major cellulolytic bacteria in rumen, with metabolomics changes (upregulation of the pentose phosphate pathway and photorespiration pathway) in rumen (Jiang et al., 2024). The same research team, under the same conditions also reported that supplementation with SCFP resulted in a higher relative abundance of Lactobacillales and an increase in enzymes such as gluconokinase, oligosaccharide reducing-end xylanase, and 3-hydroxy acid dehydrogenase. Additionally, a decrease in metabolic pathways (adenosylcobalamin biosynthesis I and the de novo biosynthesis III of pyrimidine deoxyribonucleotides) was observed, suggesting that SCFP supplementation could attenuate the dysfunction of ileal microbiome by FR (Jiang et al., 2023).
6 Beneficial effects of SCFP supplementation on epithelial barrier integrity
Loss of gut barrier integrity, so called ‘leaky gut’, has adverse effects on intestinal architecture, with reductions in villus height and mucosal surface area. In addition to adversely impacting nutrient absorption, compromised barrier integrity in the gut leads to increased translocation of gut microbes, pathogens and endotoxin into circulation and leading to systemic inflammation and immune activation (Lian et al., 2020). In an early study, SCFP supplementation did not impact fecal LPS, but was shown to reduce plasma LPS concentrations in a group of early to mid-lactation cows (Zhang et al., 2013). Subsequently, FR models have proven to be an effective method for inducing intestinal changes in cattle including increased intestinal permeability, alterations in intestinal morphology such as decreased ileal villus height and elevated concentrations of proinflammatory markers such as LPS binding protein and serum amyloid A (SAA) (Kvidera et al., 2017). Jiang et al. supplemented SCFP for 9 weeks, then subjected cows to a 5-day FR challenge (Jiang et al., 2023, 2024). Analysis of the ileal transcriptome revealed that control cows experiencing FR upregulated pathways such as “Mucin type O-glycan biosynthesis”, “ECM-receptor interaction”, “Cell adhesion molecules”, and “Tight junction”, indicative of compromised barrier function in the gut and attempts by the host to restore barrier integrity. In contrast, cows fed SCFP downregulated these pathways and overall expressed lower levels of genes associated with mucin synthesis and extracellular matrix remodeling (Jiang et al., 2023, 2024). This suggests SCFP supplementation was able to maintain and promote proper homeostasis of the mucosal barrier despite the FR challenge.
As mentioned above, feeding SCFP in calf starter resulted in improved jejunal and ileal villus-to-crypt ratio in calves, as well as increased papilla length in the rumen (Xiao et al., 2016). This increase may be due to microbiota changes such as the increase in butyrate-producing Butyrivibrio in the rumen (Xiao et al., 2016). Similar beneficial effects on intestinal morphology have been observed in lab animal models as well. In rats, exposure to heat stress results in decreased villi height, reduced mucosal thickness and increased translocation of LPS into the bloodstream (Giblot Ducray et al., 2016). Supplementation with SCFP prior to the heat stress challenge mitigated these pathological events in the intestine, maintaining villus height and mucosal integrity and thus preventing the increase in plasma LPS concentrations (Giblot Ducray et al., 2016).
In a SARA challenge in lactating dairy cows, supplementation with SCFP reduced concentrations of LTA and LPS in the plasma, as well as attenuated serum proinflammatory markers SAA and IL-1beta (Guo et al., 2022). The authors speculate that the reduction in plasma LPS and LTA may be due to improved epithelial integrity in the gut or improved immune function that enabled more efficient clearance of LPS and LTA from circulation (Guo et al., 2022). In beef heifers fed high grain rations, supplementation with SCFP via top-dress improved ruminal pH status, thus reduced the risk of SARA (Shen et al., 2019). However, there were no differences in systemic inflammatory markers between control and SCFP fed heifers. This may be because the SARA challenge was relatively mild compared to the repeated SARA challenge done by Guo et al. (2022), or rumen epithelial integrity was not impacted in this study.
While the most direct effects of postbiotic consumption are expected on barrier integrity in the gastrointestinal tract, recent reports have shown that the benefits may extend beyond the GI tract. Transcriptome analysis of mammary biopsies isolated from cows challenged with S. uberis revealed that cows fed SCFP had higher expression of tight-junction pathways and higher expression of genes related to protection of the mammary epithelial tissue (Vailati-Riboni et al., 2021). Thus, SCFP supplementation helped maintain barrier integrity in the mammary gland, protecting from S. uberis invasion. In our own work with a viral-bacterial challenge in preweaned calves, supplementation with SCFP induced greater expression of tissue-repair genes (Maina et al., 2024). Upregulation of several serine protease inhibitors and genes in the plasminogen activating system in SCFP treated calves was indicative of more active and effective wound repair responses in the lungs, compared to control calves which had increased and sustained inflammatory responses (Maina et al., 2024). Thus, SCFP supplementation helped resolve and repair the barrier in the lung. A similar response was observed in the context of DD. While the authors did not investigate the mechanisms contributing to DD protection, expression of tight-junction related proteins and maintenance of tissue integrity are essential for resistance to DD (Wilson-Welder et al., 2015; Evans et al., 2016), suggesting a beneficial role for postbiotic SCFP supplementation on epithelial barriers, even in the skin.
7 Beneficial effects of SCFP supplementation on the host immune system
Postbiotics have the potential to impact the host immune system both locally and systemically (Salminen et al., 2021). Probiotic components may interact directly with pattern recognition receptors on immune and epithelial cells lining of the gut such as toll like receptors (TLR) or nucleotide oligomerization domain (NOD)-like receptors. Beta-glucans from S. cerevisiae cell walls are known to interact with TLR2 and lectin receptors (Zhong et al., 2023), while yeast nucleic acids can interact with TLR3 and TLR9, as well as stimulator of interferon genes (STING) receptors in the cell cytosol (Biondo et al., 2011). Microbial components and pathogen-associated molecular patterns are also known to reach beyond the gut to impact other organ systems such as the lung (Bulanda and Wypych, 2022). However, microbial fermentation metabolites from postbiotics likely play a larger part in systemic immunomodulatory effects than the cell constituents through their effects on the gut microbiota, thus indirectly impacting to the host, or in some cases directly signaling to host cells (Salminen et al., 2021; Bulanda and Wypych, 2022). Metabolites (both host and microbial) then act as the messengers both locally and at distal sites to impact immunity (Bulanda and Wypych, 2022). The gut-lung axis is one of the most well described examples of this systemic communication, however, gut-skin and gut-mammary interactions have also been described (De Pessemier et al., 2021; Hu et al., 2024).
In vitro, SCFP treatment directly impacts immune cell function. Treatment with SCFP induces potent natural killer (NK) cell activation and enhances tumor cell killing by human NK cells (Jensen et al., 2008). Treatment with SCFP also enhances B cell activation in vitro, inducing upregulation of activation markers (Jensen et al., 2007). In contrast, SCFP treatment inhibits in vitro T cell activation and reduces mitogen-induced production of IL-2 and IFN-gamma, and downregulates expression of several proinflammatory chemokine receptors (Jensen et al., 2007). Thus, SCFP treatment is generally anti-inflammatory under in vitro culture conditions, but can promote enhanced immune function under some circumstances.
In cattle, treatment with SCFP often promotes anti-inflammatory or regulatory responses. Heat stress in cows promotes an increase in systemic inflammation and acute phase responses. Using an electric heat blanket to model heat stress, Al-Qaisi et al. demonstrated that control cows had increased plasma cortisol concentrations and a rise in serum amyloid A and LPS binding protein, but that SCFP supplementation for 21 days prior to the heat stress event resulted in lower levels of SAA, LPS binding protein and cortisol, thus mitigating the inflammatory effects of heat stress (Al-Qaisi et al., 2020). Further, SCFP supplementation positively impacted leukocyte counts, with increased concentrations of circulating total white blood cells and neutrophils, suggesting cows were better positioned to withstand stress or an infection challenge (Al-Qaisi et al., 2020). Importantly, the controlled inflammatory response in this model is likely driven by a combination of multiple mechanisms, including improved barrier integrity and gut health, in addition to direct immunomodulatory effects of SCFP treatment.
In calves fed SCFP for the first 21 days of life, Mahmoud et al. observed that immune cells isolated from the peripheral blood of calves receiving SCFP had an increased capacity for proinflammatory cytokine secretion when stimulated with TLR agonists such as LPS, PAM3CSK4 or Poly(I:C) compared to cells from control calves (Mahmoud et al., 2020). This suggests that SCFP supplementation positions the systemic immune system to mount a more rapid and robust response against invading pathogens. Interestingly, this effect was opposite when cells from the lung were stimulated with the same microbial components. Cells isolated from the airways of SCFP supplemented calves produced less proinflammatory cytokines in response to TLR stimulation than cells from the control group (Mahmoud et al., 2020). Thus, SCFP treatment may promote a quieter response in the lung mucosa, protecting the lung from excessive inflammation and tissue damage. In a follow-up study, RNAseq analysis was performed on airway cells isolated before and after a viral-bacterial coinfection, and on lung tissues isolated on day 10 after co-infection from SCFP fed calves and controls (Maina et al., 2024). Analysis of cells isolated from the airways (bronchoalveolar lavage samples) of SCFP fed calves prior to infection revealed an upregulation of biological pathways corresponding to immune processes such as ‘lymphocyte activation’, ‘innate immune activation’ and ‘cytokine-cytokine receptor interactions’ (Maina et al., 2024). This analysis suggests that SCFP treatment may prime the lung immune system, so it is more prepared to fight an infectious insult. In support of this hypothesis, analysis of the airways and lung tissues after viral-bacterial infection revealed that SCFP fed calves had higher expression of genes related to antiviral immunity such as OAS and several interferon stimulated genes (ISG), but lower expression of inflammation related genes such as CCL8, CXCL5 and CXCL8 which are chemoattractants for neutrophils and monocytes. Calves supplemented with SCFP did indeed accumulate fewer numbers of neutrophils in the airways following both viral (Mahmoud et al., 2020) and viral-bacterial coinfection (McDonald et al., 2021), supporting the results of the transcriptome analysis. Neutrophil-mediated immunopathology is a major contributor to lung damage and poor disease outcomes during BRD (McGill and Sacco, 2020), thus, limiting these effects may be one mechanism by which SCFP treatment benefits the host.
Vailati-Riboni et al. performed a transcriptional analysis of mammary tissue from SCFP fed cows following S. uberis challenge (Vailati-Riboni et al., 2021). This analysis revealed an increase in pathways related to antibacterial immune responses and genes such as NOS and CATHL4, as well as an upregulation of regulatory and tissue-repair related genes such as ATF3, encoding a transcription factor regulating anti-inflammatory cascades, IER3, a gene promoting apoptosis and resolution of inflammation, and several heat shock proteins which play a role in resolving inflammation and restoring homeostasis (Vailati-Riboni et al., 2021). Consistent with the regulatory gene signatures observed in the lung during respiratory infection (Maina et al., 2024), and the mammary gland during mastitis (Vailati-Riboni et al., 2021), SCFP supplementation also seems to modulate aspects of the immune response in feed restricted cows (Jiang et al., 2023). Along with promoting tissue barrier integrity (discussed above), cows receiving SCFP during a FR challenge expressed lower levels of proinflammatory genes in the ileum compared to control cows such as CXCL12, CCL14 and CXCL14 (Jiang et al., 2023). Thus, maintaining tissue homeostasis and regulating damaging inflammation in mucosal sites seems to be a consistent effect of SCFP treatment.
The effects of SCFP on immune function and inflammation are not always consistent across trials in other models of immune function. In a trial in light-weight crossbred beef steers, Burdick-Sanchez et al. fed 12 g/h/d SCFP for 21 days, then challenged calves with intravenous LPS (Burdick Sanchez et al., 2020). Calves receiving SCFP had higher rectal temperatures in the 24 h following LPS challenge, although control calves had higher sickness behavior scores. SCFP fed calves had lower concentrations of serum TNF-alpha and IL-6, but did not differ in acute phase protein concentrations (Burdick Sanchez et al., 2020). Interestingly, when Klopp et al. performed a similar LPS challenge in weaned Holstein bull calves (Klopp et al., 2022b), SCFP fed calves had a more pronounced reaction to LPS, with increased serum IL-6 and TNF-alpha compared to control calves. However, in a parallel study, Klopp et al. observed that SCFP fed calves had overall lower incidences of respiratory disease with fewer required treatments and fewer second and third treatments compared to controls (Klopp et al., 2022a). Thus, Klopp et al. suggested that SCFP treatment may increase basal levels of innate immune activation, an observation which is consistent with the transcriptomics results observed by Maina et al. in the airways (Maina et al., 2024), and Vailati-Riboni et al. in the mammary gland (Vailati-Riboni et al., 2021), although there seems to be a balancing effect of SCFP treatment, as many animals have simultaneously demonstrated more controlled inflammatory responses. The reason for these differences in proinflammatory cytokine production across trials are not immediately clear, although one aspect may be the age of the animals. Burdick Sanchez et al. used weaned, crossbred steer calves weighing 274 kg (>6 months of age), while Klopp et al. used 50-day-old calves. Klopp et al. also noted in their discussion that differences across studies might be attributed to factors such as dosage, health status, and the age of the animals. In a trial with piglets and a similar intravenous LPS challenge, animals receiving SCFP developed higher concentrations of serum TNF-α and IL-6 compared to the control group (Burdick Sanchez et al., 2018). Piglets in this trial were 19-21 days of age, and these findings align with Klopp et al. in young calves (Burdick Sanchez et al., 2018). Thus, we speculate that SCFPs might play different roles in disease resilience in younger versus older animals.
The impact of SCFP supplementation seems to be primarily restricted to the innate immune system, as studies measuring adaptive immunity have shown no or minimal effects. Zaworski et al. fed two different concentrations of SCFP to transition cows from 28 days prior to calving through 28 d postpartum (Zaworski et al., 2014). No differences were observed in serum IgA, IgG or IgM concentrations, however antigen-specific responses were not evaluated. Therefore, Sivinski et al. measured the immune response to the model antigen ovalbumin (OVA) in transition cows that received SCFP or not for 29 days prior to calving through 42 d post calving (Sivinski et al., 2022). No differences were observed in OVA-specific serum antibody responses. Likewise, Knoblock et al. saw no changes in serum IgG responses in transition cows fed increasing starch diets by immunizing with OVA on d 7 and 21 post calving (Knoblock et al., 2019). In calves, Magalhães et al. immunized with OVA at 3, 21 and 42 days of age, but observed no differences in the serum antibody response between SCFP and control calves (Magalhães et al., 2008). Mahmoud et al. evaluated adaptive immune responses to BRSV infection in preweaned calves on day 7 after infection (Mahmoud et al., 2020). Although the brief time following infection may not have enabled optimal development of the T and B cell response, there were no differences in cellular or humoral responses to the BRSV infection (Mahmoud et al., 2020).
In other species, supplementation with SCFP has shown some effects on adaptive immunity. Horses receiving SCFP showed differences in circulating CD4 T cell populations following booster immunization against equine influenza, and SCFP fed horses had elevated antibody titers against some influenza strains in the vaccine, although this was not evident against all of the strains in the booster (Lucassen et al., 2021). In broilers, supplementation with SCFP resulted in an accelerated response to infectious bursal disease vaccination (Soren et al., 2024), with higher titers on d 28 after immunization compared to control birds, although all birds reached similar antibody titers by day 35 after vaccination. Thus, some aspects of SCFP effects may differ in cows compared to other species, or SCFP effects may be dependent on the antigen tested, or host status, such as history of prior stress or health challenges, or vaccination status.
SCFP supplementation has clear effects on the host immune system, as evidence by the beneficial outcomes in multiple types of health challenges. However, the effects of SCFP supplementation seem somewhat paradoxical both in vitro and in vivo, in some cases promoting robust antimicrobial and proinflammatory responses, while in other instances promoting regulatory responses or reducing inflammation. Further, the immunomodulatory effects of SCFP are difficult to unravel from the antioxidant activity. Oxidative stress occurs when oxidative free radicals exceed antioxidant capacity in the cell, which can lead to damage of lipids, nucleic acids and proteins. Oxygen free radicals are a potent tool used by the immune system to control infection, and cellular oxidants are also generated by natural physiologic processes. Oxidative stress is known to trigger or perpetuate downstream inflammatory responses (Biswas, 2016). In vitro, SCFP has potent antioxidant effects and protects cells from oxidative damage (Jensen et al., 2008). In vitro treatment with SCFP also reduces oxidative burst activity in neutrophils (Jensen et al., 2008), which may be due to a combination of its immunomodulatory and antioxidant effects. Supplementation with SCFP also supports antioxidant capacity in vivo as has been observed in finishing beef cattle (Rients et al., 2021), beef cattle experiencing transport stress (Deters and Hansen, 2019) and in humans (Jensen et al., 2011, 2015). Thus, some immune-related effects of SCFP may also be linked to this antioxidant activity.
8 Conclusions
SCFP supplementation induces changes in the habitat and composition of microbiome necessary for digestion by cattle, resulting in alterations in the gastrointestinal tract function. This, in turn, enhances digestion efficiency, promotes energy utilization, and triggers metabolic changes. Additionally, through alterations in both local and systemic immunological mechanisms, SCFP supplementation exhibits immunomodulatory effects, ultimately enhancing resistance to various stresses and infections in the cow. While several studies have reported clearly positive impacts of SCFP on health, the outcomes of supplementation are not always consistent and can vary across different conditions. At this time, it is not clear if some animals are ‘responders’ or ‘non-responders’, or if SCFP supplementation is more beneficial in the context of certain diseases or stressors. These discrepancies highlight the importance of understanding the underlying mechanisms of action. Here, we have identified four distinct mechanisms likely contributing to the efficacy of SCFP postbiotics. However, questions remain regarding the interactions or synergisms between these compartments. Improved understanding of the interactions between the host microbiota and immunomodulatory modes of action would result in more intentional approaches for modifying formulations that target or enhance these interactions. Research on the physical and physiological changes in the gastrointestinal tract, respiratory tract, and mammary glands of cattle due to SCFP supplementation, and the consequent alterations in the microbiome and metabolome, is ongoing. In depth studies in the context of respiratory disease, feed restriction and mastitis have so far been provided insights into plausible mechanisms of action of SCFP. Further transcriptional or metabolic analyses in other disease or stress conditions will further improve our understanding of mode of action. As the need for efficacious alternatives to antibiotics becomes more pressing, SCFP supplementation represents a promising and economical alternative for improving cattle performance and resilience.
Author contributions
JBC: Conceptualization, Writing – original draft, Writing – review & editing. ADS: Visualization, Writing – original draft, Writing – review & editing. JLM: Supervision, Writing – original draft, Writing – review & editing.
Funding
The author(s) declare that no financial support was received for the research, authorship, and/or publication of this article.
Acknowledgments
The authors would like to acknowledge Dr. Ilkyu Yoon for useful discussions and input during the writing process.
Conflict of interest
The authors declare that the research was conducted in the absence of any commercial or financial relationships that could be construed as a potential conflict of interest.
The authors have received previous funding support and worked collaboratively with Diamond V on research projects.
Publisher’s note
All claims expressed in this article are solely those of the authors and do not necessarily represent those of their affiliated organizations, or those of the publisher, the editors and the reviewers. Any product that may be evaluated in this article, or claim that may be made by its manufacturer, is not guaranteed or endorsed by the publisher.
References
Aghamohammadi M., Haine D., Kelton D. F., Barkema H. W., Hogeveen H., Keefe G. P., et al. (2018). Herd-level mastitis-associated costs on Canadian dairy farms. Front. Vet. Sci. 5. doi: 10.3389/fvets.2018.00100
Allen M. S., Ying Y. (2012). Effects of Saccharomyces cerevisiae fermentation product on ruminal starch digestion are dependent upon dry matter intake for lactating cows. J. Dairy Sci. 95, 6591–6605. doi: 10.3168/jds.2012-5377
Al-Qaisi M., Horst E. A., Mayorga E. J., Goetz B. M., Abeyta M. A., Yoon I., et al. (2020). Effects of a Saccharomyces cerevisiae fermentation product on heat-stressed dairy cows. J. Dairy Sci. 103, 9634–9645. doi: 10.3168/jds.2020-18721
Alugongo G. M., Xiao J. X., Chung Y. H., Dong S. Z., Li S. L., Yoon I., et al. (2017). Effects of Saccharomyces cerevisiae fermentation products on dairy calves: Performance and health. J. Dairy Sci. 100, 1189–1199. doi: 10.3168/jds.2016-11399
Anklam K., Cernek P., Yoon I., Wheeler J., Birkle T., Döpfer D. (2022). Effects of supplementing a Saccharomyces cerevisiae fermentation product on the prevention and control of digital dermatitis in lactating dairy cows. Appl. Anim. Sci. 38, 98–109. doi: 10.15232/aas.2021-02229
Biondo C., Signorino G., Costa A., Midiri A., Gerace E., Galbo R., et al. (2011). Recognition of yeast nucleic acids triggers a host-protective type I interferon response. Eur. J. Immunol. 41, 1969–1979. doi: 10.1002/eji.201141490
Biswas S. K. (2016). Does the interdependence between oxidative stress and inflammation explain the antioxidant paradox? Oxid. Med. Cell. Longevity 2016, 5698931. doi: 10.1155/2016/5698931
Brewer M. T., Anderson K. L., Yoon I., Scott M. F., Carlson S. A. (2014). Amelioration of salmonellosis in pre-weaned dairy calves fed Saccharomyces cerevisiae fermentation products in feed and milk replacer. Vet. Microbiol. 172, 248–255. doi: 10.1016/j.vetmic.2014.05.026
Buczinski S., Achard D., Timsit E. (2021). Effects of calfhood respiratory disease on health and performance of dairy cattle: A systematic review and meta-analysis. J. Dairy Sci. 104, 8214–8227. doi: 10.3168/jds.2020-19941
Bulanda E., Wypych T. P. (2022). Bypassing the gut–lung axis via microbial metabolites: implications for chronic respiratory diseases. Front. Microbiol. 13. doi: 10.3389/fmicb.2022.857418
Burdick Sanchez N. C., Carroll J. A., Broadway P. R., Bass B. E., Frank J. W. (2018). Modulation of the acute phase response following a lipopolysaccharide challenge in pigs supplemented with an all-natural Saccharomyces cerevisiae fermentation product. Livestock Sci. 208, 1–4. doi: 10.1016/j.livsci.2017.11.022
Burdick Sanchez N. C., Carroll J. A., Broadway P. R., Edrington T. S., Yoon I., Belknap C. R. (2020). Some aspects of the acute phase immune response to a lipopolysaccharide (LPS) challenge are mitigated by supplementation with a Saccharomyces cerevisiae fermentation product in weaned beef calves. Trans. Anim. Sci. 4, txaa156. doi: 10.1093/tas/txaa156
Callaway E., Martin S. (1997). Effects of a Saccharomyces cerevisiae culture on ruminal bacteria that utilize lactate and digest cellulose. J. dairy Sci. 80, 2035–2044. doi: 10.3168/jds.S0022-0302(97)76148-4
Coleman D. N., Jiang Q., Lopes M. G., Ritt L., Liang Y., Aboragah A., et al. (2023). Feeding a Saccharomyces cerevisiae fermentation product before and during a feed restriction challenge on milk production, plasma biomarkers, and immune function in Holstein cows. J. Anim. Sci. 101, 1–12. doi: 10.1093/jas/skad019
De Pessemier B., Grine L., Debaere M., Maes A., Paetzold B., Callewaert C. (2021). Gut–skin axis: current knowledge of the interrelationship between microbial dysbiosis and skin conditions. Microorganisms 9, 353. doi: 10.3390/microorganisms9020353
Deters E. L., Hansen S. L. (2019). Effect of supplementing a Saccharomyces cerevisiae fermentation product during a preconditioning period prior to transit on receiving period performance, nutrient digestibility, and antioxidant defense by beef steers. Trans. Anim. Sci. 3, 1227–1237. doi: 10.1093/tas/txz140
Dopfer D., Ordaz Puga S., Aviles M., Henschel S., Buetttner J., Henige M., et al. (2024). 2024. Effect of postbiotic Saccharomyces cerevisiae fermentation product on experimentally induced digital dermatitis infections in Holstein Friesian steers. Am. Dairy Sci. Assoc. Annu. Meet. J. Dairy Sci. 107 (Suppl. 1), abstract 2059.
Dušková D., Marounek M. (2001). Fermentation of pectin and glucose, and activity of pectin-degrading enzymes in the rumen bacterium Lachnospira multiparus. Lett. Appl. Microbiol. 33, 159–163. doi: 10.1046/j.1472-765x.2001.00970.x
Elghandour M. M. Y., Tan Z. L., Abu Hafsa S. H., Adegbeye M. J., Greiner R., Ugbogu E. A., et al. (2020). Saccharomyces cerevisiae as a probiotic feed additive to non and pseudo-ruminant feeding: a review. J. Appl. Microbiol. 128, 658–674. doi: 10.1111/jam.14416"10.1111/jam.14416
Evans N. J., Murray R. D., Carter S. D. (2016). Bovine digital dermatitis: Current concepts from laboratory to farm. Vet. J. 211, 3–13. doi: 10.1016/j.tvjl.2015.10.028
Ferguson J. D., Sattler M. A., Hanson D. L., Davis C. P., Edrington T. S., Yoon I. (2018). “Feeding NutriTek reduces linear scores and clinical mastitis cases,” in 2018 American Dairy Science Association Annual Meeting (Knoxville, Tennessee: Journal of Dairy Science).
Fernández-Pacheco P., Ramos Monge I. M., Fernández-González M., Poveda Colado J. M., Arévalo-Villena M. (2021). Safety evaluation of yeasts with probiotic potential. Front. Nutr. 8, 659328. doi: 10.3389/fnut.2021.659328
Gao J., Zhang H. J., Wu S. G., Yu S. H., Yoon I., Moore D., et al. (2009). Effect of Saccharomyces cerevisiae fermentation product on immune functions of broilers challenged with Eimeria tenella. Poult Sci. 88, 2141–2151. doi: 10.3382/ps.2009-00151
Giblot Ducray H. A., Globa L., Pustovyy O., Reeves S., Robinson L., Vodyanoy V., et al. (2016). Mitigation of heat stress-related complications by a yeast fermentate product. J. Thermal Biol. 60, 26–32. doi: 10.1016/j.jtherbio.2016.06.002
Górka P., Kowalski Z. M., Zabielski R., Guilloteau P. (2018). Invited review: Use of butyrate to promote gastrointestinal tract development in calves. J. dairy Sci. 101, 4785–4800. doi: 10.3168/jds.2017-14086
Guo J., Xu L., Khalouei H., Fehr K., Senaratne V., Ghia J. E., et al. (2022). Saccharomyces cerevisiae fermentation products reduce bacterial endotoxin concentrations and inflammation during grain-based subacute ruminal acidosis in lactating dairy cows. J. Dairy Sci. 105, 2354–2368. doi: 10.3168/jds.2021-20572
Harris T. L., Liang Y., Sharon K. P., Sellers M. D., Yoon I., Scott M. F., et al. (2017). Influence of Saccharomyces cerevisiae fermentation products, SmartCare in milk replacer and Original XPC in calf starter, on the performance and health of preweaned Holstein calves challenged with Salmonella enterica serotype Typhimurium. J. Dairy Sci. 100, 7154–7164. doi: 10.3168/jds.2016-12509
Hu X., He Z., Zhao C., He Y., Qiu M., Xiang K., et al. (2024). Gut/rumen-mammary gland axis in mastitis: Gut/rumen microbiota–mediated “gastroenterogenic mastitis. J. Adv. Res. 55, 159–171. doi: 10.1016/j.jare.2023.02.009
Hučko B., Bampidis V. A., Kodeš A., Christodoulou V., Mudřik Z., Poláková K., et al. (2009). Rumen fermentation characteristics in pre-weaning calves receiving yeast culture supplements. Czech J. Anim. Sci. 54, 435–442. doi: 10.17221/1674-CJAS
Irvine L. D., Freeman M. J., Donaghy D. J., Yoon I., Lee G., Roche J. R. (2011). Short communication: Responses to supplemental Saccharomyces cerevisiae fermentation product and triticale grain in dairy cows grazing high-quality pasture in early lactation. J. Dairy Sci. 94, 3119–3123. doi: 10.3168/jds.2010-3894
Jensen G. S., Carter S. G., Reeves S. G., Robinson L. E., Benson K. F. (2015). Anti-inflammatory properties of a dried fermentate in vitro and in vivo. J. med. Food 18, 378–384. doi: 10.1089/jmf.2013.0158
Jensen G. S., Hart A. N., Schauss A. G. (2007). An antiinflammatory immunogen from yeast culture induces activation and alters chemokine receptor expression on human natural killer cells and B lymphocytes in vitro. Nutr. Res. 27, 327–335. doi: 10.1016/j.nutres.2007.04.008
Jensen G. S., Patterson K. M., Yoon I. (2008). Yeast culture has anti-inflammatory effects and specifically activates NK cells. Comp. Immunol. Microbiol. Infect. Dis. 31, 487–500. doi: 10.1016/j.cimid.2007.08.005
Jensen G. S., Redman K. A., Benson K. F., Carter S. G., Mitzner M. A., Reeves S., et al. (2011). Antioxidant bioavailability and rapid immune-modulating effects after consumption of a single acute dose of a high-metabolite yeast immunogen: results of a placebo-controlled double-blinded crossover pilot study. J. med. Food 14, 1002–1010. doi: 10.1089/jmf.2010.0174
Jiang Q., Sherlock D. N., Elolimy A. A., Vailati-Riboni M., Yoon I., Loor J. J. (2023). Impact of a Saccharomyces cerevisiae fermentation product during an intestinal barrier challenge in lactating Holstein cows on ileal microbiota and markers of tissue structure and immunity. J. Anim. Sci. 101, skad309. doi: 10.1093/jas/skad309
Jiang Q., Sherlock D. N., Elolimy A. A., Yoon I., Loor J. J. (2024). Feeding a Saccharomyces cerevisiae fermentation product during a gut barrier challenge in lactating Holstein cows impacts the ruminal microbiota and metabolome. J. Dairy Sci. 107, 4476–4494. doi: 10.3168/jds.2023-24147
Kaldmäe H., Suurmets H., Järveots T., Suuroja T., Kärt O. (2008). Effects of supplemental yeast (Saccharomyces cerevisiae) culture on rumen development and growth in calves. Akadeemiline Pollumajanduse Selts 19, 19–23.
Khafipour E., Li S., Tun H., Derakhshani H., Moossavi S., Plaizier J. (2016). Effects of grain feeding on microbiota in the digestive tract of cattle. Anim. Front. 6, 13–19. doi: 10.2527/af.2016-0018
Khalouei H., Seranatne V., Fehr K., Guo J., Yoon I., Khafipour E., et al. (2020). Effects of Saccharomyces cerevisiae fermentation products and subacute ruminal acidosis on feed intake, fermentation, and nutrient digestibilities in lactating dairy cows. Can. J. Anim. Sci. 101, 143–157. doi: 10.1139/cjas-2020-0018
Klopp R. N., Centeno-Martinez R. E., Yoon I., Johnson T. A., Boerman J. P. (2022a). Effects of feeding Saccharomyces cerevisiae fermentation products on the health and growth performance of Holstein dairy calves. JDS Commun. 3, 174–179. doi: 10.3168/jdsc.2021-0172
Klopp R. N., Yoon I., Eicher S., Boerman J. P. (2022b). Effects of feeding Saccharomyces cerevisiae fermentation products on the health of Holstein dairy calves following a lipopolysaccharide challenge. J. Dairy Sci. 105, 1469–1479. doi: 10.3168/jds.2021-20341
Knoblock C. E., Shi W., Yoon I., Oba M. (2019). Effects of supplementing a Saccharomyces cerevisiae fermentation product during the periparturient period on the immune response of dairy cows fed fresh diets differing in starch content. J. Dairy Sci. 102, 6199–6209. doi: 10.3168/jds.2018-16224
Koike S., Kobayashi Y. (2009). Fibrolytic rumen bacteria: their ecology and functions. Asian-Australasian J. Anim. Sci. 22, 131–138. doi: 10.5713/ajas.2009.r.01
Kvidera S. K., Horst E. A., Sanz Fernandez M. V., Abuajamieh M., Ganesan S., Gorden P. J., et al. (2017). Characterizing effects of feed restriction and glucagon-like peptide 2 administration on biomarkers of inflammation and intestinal morphology. J. Dairy Sci. 100, 9402–9417. doi: 10.3168/jds.2017-13229
Lesmeister K. E., Heinrichs A. J., Gabler M. T. (2004). Effects of supplemental yeast (Saccharomyces cerevisiae) culture on rumen development, growth characteristics, and blood parameters in neonatal dairy calves. J. Dairy Sci. 87, 1832–1839. doi: 10.3168/jds.S0022-0302(04)73340-8
Li S., Yoon I., Scott M., Khafipour E., Plaizier J. C. (2016). Impact of Saccharomyces cerevisiae fermentation product and subacute ruminal acidosis on production, inflammation, and fermentation in the rumen and hindgut of dairy cows. Anim. Feed Sci. Technol. 211, 50–60. doi: 10.1016/j.anifeedsci.2015.10.010
Lian P., Braber S., Garssen J., Wichers H. J., Folkerts G., Fink-Gremmels J., et al. (2020). Beyond heat stress: intestinal integrity disruption and mechanism-based intervention strategies. Nutrients 12, 734. doi: 10.3390/nu12030734
Lin C.-Y., Alexander C., Steelman A. J., Warzecha C. M., de Godoy M. R. C., Swanson K. S. (2019). Effects of a Saccharomyces cerevisiae fermentation product on fecal characteristics, nutrient digestibility, fecal fermentative end-products, fecal microbial populations, immune function, and diet palatability in adult dogs1. J. Anim. Sci. 97, 1586–1599. doi: 10.1093/jas/skz064
Liu K., Zhang Y., Yu Z., Xu Q., Zheng N., Zhao S., et al. (2021). Ruminal microbiota–host interaction and its effect on nutrient metabolism. Anim. Nutr. 7, 49–55. doi: 10.1016/j.aninu.2020.12.001
Lucassen A., Finkler-Schade C., Schuberth H. J. (2021). A Saccharomyces cerevisiae Fermentation Product (Olimond BB) Alters the Early Response after Influenza Vaccination in Racehorses. Animals 11, 2726. doi: 10.3390/ani11092726
Magalhães V. J., Susca F., Lima F. S., Branco A. F., Yoon I., Santos J. E. (2008). Effect of feeding yeast culture on performance, health, and immunocompetence of dairy calves. J. Dairy Sci. 91, 1497–1509. doi: 10.3168/jds.2007-0582
Mahmoud A. H. A., Slate J. R., Hong S., Yoon I., Mcgill J. L. (2020). Supplementing a Saccharomyces cerevisiae fermentation product modulates innate immune function and ameliorates bovine respiratory syncytial virus infection in neonatal calves. J. Anim. Sci. 98, 1–16. doi: 10.1093/jas/skaa252
Maina T., McDonald P., Samuel B., Sardi M., Yoon I., Rogers A., et al. (2024). Feeding Saccharomyces cerevisiae fermentation postbiotic products alters immune function and the lung transcriptome of preweaning calves with an experimental viral-bacterial coinfection. J. Dairy Sci. 107, 2253–2267. doi: 10.3168/jds.2023-23866
Marins T. N., Gutierrez Oviedo F. A., Costa M. L. G. F., Chen Y. C., Goodnight H., Garrick M., et al. (2023). Impacts of feeding a Saccharomyces cerevisiae fermentation product on productive performance, and metabolic and immunological responses during a feed-restriction challenge of mid-lactation dairy cows. J. Dairy Sci. 106, 202–218. doi: 10.3168/jds.2022-22522
Matthews C., Crispie F., Lewis E., Reid M., O’Toole P. W., Cotter P. D. (2019). The rumen microbiome: a crucial consideration when optimising milk and meat production and nitrogen utilisation efficiency. Gut Microbes 10, 115–132. doi: 10.1080/19490976.2018.1505176
McDonald P. O., Schill C., Maina T. W., Samuel B., Porter M., Yoon I., et al. (2021). Feeding Saccharomyces cerevisiae fermentation products lessens the severity of a viral-bacterial coinfection in preweaned calves. J. Anim. Sci. 99, 1–13. doi: 10.1093/jas/skab300
McGill J. L., Sacco R. E. (2020). The immunology of bovine respiratory disease: recent advancements. Vet. Clinics: Food Anim. Pract. 36, 333–348. doi: 10.1016/j.cvfa.2020.03.002
Moyad M. A., Robinson L. E., Zawada E. T., Kittelsrud J. M., Chen D. G., Reeves S. G., et al. (2008). Effects of a modified yeast supplement on cold/flu symptoms. Urol Nurs. 28, 50–55.
Moyad M. A., Robinson L. E., Kittelsrud J. M., Reeves S. G., Weaver S. E., Guzman A. I., et al. (2009). Immunogenic yeast-based fermentation product reduces allergic rhinitis-induced nasal congestion: a randomized, double-blind, placebo-controlled trial. Adv. Ther. 26, 795–804. doi: 10.1007/s12325-009-0057-y
Moyad M. A., Robinson L. E., Zawada E. T., Kittelsrud J., Chen D. G., Reeves S. G., et al. (2010). Immunogenic yeast-based fermentate for cold/flu-like symptoms in nonvaccinated individuals. J. Altern. Complement Med. 16, 213–218. doi: 10.1089/acm.2009.0310
Olagaray K. E., Sivinski S. E., Saylor B. A., Mamedova L. K., Sauls-Hiesterman J. A., Yoon I., et al. (2019). Effect of Saccharomyces cerevisiae fermentation product on feed intake parameters, lactation performance, and metabolism of transition dairy cattle. J. Dairy Sci. 102, 8092–8107. doi: 10.3168/jds.2019-16315
Overton M. W. (2020). Economics of respiratory disease in dairy replacement heifers. Anim. Health Res. Rev. 21, 143–148. doi: 10.1017/S1466252320000250
Pang Y., Zhang H., Wen H., Wan H., Wu H., Chen Y., et al. (2022). Yeast probiotic and yeast products in enhancing livestock feeds utilization and performance: An overview. J. Fungi 8, 1191. doi: 10.3390/jof8111191
Parapouli M., Vasileiadis A., Afendra A. S., Hatziloukas E. (2020). Saccharomyces cerevisiae and its industrial applications. AIMS Microbiol. 6, 1. doi: 10.3934/microbiol.2020001
Pisoni L., Relling A. E. (2020). The effects of supplementing yeast fermentation products on gut permeability, hormone concentration, and growth in newborn dairy calves1. Trans. Anim. Sci. 4, 809–821. doi: 10.1093/tas/txaa004
Plaizier J. C., Khafipour E., Li S., Gozho G. N., Krause D. O. (2012). Subacute ruminal acidosis (SARA), endotoxins and health consequences. Anim. Feed Sci. Technol. 172, 9–21. doi: 10.1016/j.anifeedsci.2011.12.004
Plaizier J., Mesgaran M. D., Derakhshani H., Golder H., Khafipour E., Kleen J., et al. (2018). Enhancing gastrointestinal health in dairy cows. Animal 12, s399–s418. doi: 10.1017/S1751731118001921
Poppy G. D., Rabiee A. R., Lean I. J., Sanchez W. K., Dorton K. L., Morley P. S. (2012). A meta-analysis of the effects of feeding yeast culture produced by anaerobic fermentation of Saccharomyces cerevisiae on milk production of lactating dairy cows. J. Dairy Sci. 95, 6027–6041. doi: 10.3168/jds.2012-5577
Rients E., Deters E., McGill J., Belknap C., Hansen S. (2021). Novel Saccharomyces cerevisiae fermentation product affects growth performance, immune system, and antioxidant capacity of finishing beef steers. Trans. Anim. Sci. 5, S111–S114. doi: 10.1093/tas/txab159
Rodriguez Z., Cabrera V. E., Hogeveen H., Ruegg P. L. (2024). Economic impact of treatment of subclinical mastitis in early lactation using intramammary nisin. J. Dairy Sci. 107, 4634–4645. doi: 10.3168/jds.2023-24311
Salminen S., Collado M. C., Endo A., Hill C., Lebeer S., Quigley E. M. M., et al. (2021). The International Scientific Association of Probiotics and Prebiotics (ISAPP) consensus statement on the definition and scope of postbiotics. Nat. Rev. Gastroenterol. Hepatol. 18, 649–667. doi: 10.1038/s41575-021-00440-6
Shen Y., Davedow T., Ran T., Saleem A. M., Yoon I., Narvaez C., et al. (2019). Ruminally protected and unprotected Saccharomyces cerevisiae fermentation products as alternatives to antibiotics in finishing beef steers1. J. Anim. Sci. 97, 4323–4333. doi: 10.1093/jas/skz270
Shen Y., Wang H., Ran T., Yoon I., Saleem A. M., Yang W. (2018). Influence of yeast culture and feed antibiotics on ruminal fermentation and site and extent of digestion in beef heifers fed high grain rations1. J. Anim. Sci. 96, 3916–3927. doi: 10.1093/jas/sky249
Shi W., Knoblock C., Yoon I., Oba M. (2019). Effects of supplementing a Saccharomyces cerevisiae fermentation product during the transition period on rumen fermentation of dairy cows fed fresh diets differing in starch content. J. dairy Sci. 102, 9943–9955. doi: 10.3168/jds.2019-16671
Sivinski S. E., Meier K. E., Mamedova L. K., Saylor B. A., Shaffer J. E., Sauls-Hiesterman J. A., et al. (2022). Effect of Saccharomyces cerevisiae fermentation product on oxidative status, inflammation, and immune response in transition dairy cattle. J. Dairy Sci. 105, 8850–8865. doi: 10.3168/jds.2022-21998
Soren S., Mandal G. P., Mondal S., Pradhan S., Mukherjee J., Banerjee D., et al. (2024). Efficacy of saccharomyces cerevisiae fermentation product and probiotic supplementation on growth performance, gut microflora and immunity of broiler chickens. Animals 14, 866. doi: 10.3390/ani14060866
Thomas M., Serrenho R. C., Puga S. O., Torres J. M., Puga S. O., Stangaferro M. (2023). Effect of feeding a Saccharomyces cerevisiae fermentation product to Holstein cows exposed to high temperature and humidity conditions on milk production performance and efficiency—A pen-level trial. J. Dairy Sci. 106, 4650–4665. doi: 10.3168/jds.2022-22516
Tun H. M., Li S., Yoon I., Meale S. J., Azevedo P. A., Khafipour E., et al. (2020). Saccharomyces cerevisiae fermentation products (SCFP) stabilize the ruminal microbiota of lactating dairy cows during periods of a depressed rumen pH. BMC Vet. Res. 16, 1–17. doi: 10.1186/s12917-020-02437-w
Vailati-Riboni M., Coleman D. N., Lopreiato V., Alharthi A., Bucktrout R. E., Abdel-Hamied E., et al. (2021). Feeding a Saccharomyces cerevisiae fermentation product improves udder health and immune response to a Streptococcus uberis mastitis challenge in mid-lactation dairy cows. J. Anim. Sci. Biotechnol. 12, 62. doi: 10.1186/s40104-021-00560-8
van Soest F. J. S., Santman-Berends I. M. G. A., Lam T. J. G. M., Hogeveen H. (2016). Failure and preventive costs of mastitis on Dutch dairy farms. J. Dairy Sci. 99, 8365–8374. doi: 10.3168/jds.2015-10561
Vélez J., Lange M. K., Zieger P., Yoon I., Failing K., Bauer C. (2019). Long-term use of yeast fermentation products in comparison to halofuginone for the control of cryptosporidiosis in neonatal calves. Vet. Parasitol. 269, 57–64. doi: 10.1016/j.vetpar.2019.04.008
Williams A. G., Coleman G. S. (1997). "The Rumen Protozoa", in The Rumen Microbial Ecosystem. 2nd Edition. Eds. Hobson P. N., Stewart C. S. (Dordrecht: Springer), 73–139. doi: 10.1007/978-94-009-1453-7_3
Wilson-Welder J., Alt D., Nally J. (2015). Digital dermatitis in cattle: current bacterial and immunological findings. Animals 5, 1114–1135. doi: 10.3390/ani5040400
Xiao J., Alugongo G., Chung R., Dong S., Li S., Yoon I., et al. (2016). Effects of Saccharomyces cerevisiae fermentation products on dairy calves: Ruminal fermentation, gastrointestinal morphology, and microbial community. J. Dairy Sci. 99, 5401–5412. doi: 10.3168/jds.2015-10563
Xiao J., Alugongo G. M., Ji S., Wu Z., Dong S., Li S., et al. (2018). Effects of Saccharomyces cerevisiae fermentation products on the microbial community throughout the gastrointestinal tract of calves. Animals 9, 4. doi: 10.3390/ani9010004
Yan H., Xing Q., Xiao X., Yu B., He J., Mao X., et al. (2024). Effect of saccharomyces cerevisiae postbiotics and essential oil on growth performance and intestinal health of weanling pigs during K88 ETEC infection. J. Anim. Sci. 102, skae007. doi: 10.1093/jas/skae007
Yuan K., Liang T., Muckey M. B., Mendonça L. G., Hulbert L. E., Elrod C. C., et al. (2015). Yeast product supplementation modulated feeding behavior and metabolism in transition dairy cows. J. Dairy Sci. 98, 532–540. doi: 10.3168/jds.2014-8468
Zaworski E. M., Shriver-Munsch C. M., Fadden N. A., Sanchez W. K., Yoon I., Bobe G. (2014). Effects of feeding various dosages of Saccharomyces cerevisiae fermentation product in transition dairy cows. J. Dairy Sci. 97, 3081–3098. doi: 10.3168/jds.2013-7692
Zhang R.-y., Yoon I., Zhu W.-y., Mao S.-y. (2013). Effect of saccharomyces cerevisiae fermentation product on lactation performance and lipopolysaccharide concentration of dairy cows. Asian-Australas J. Anim. Sci. 26, 1137–1143. doi: 10.5713/ajas.2013.13181
Zhong X., Wang G., Li F., Fang S., Zhou S., Ishiwata A., et al. (2023). Immunomodulatory effect and biological significance of β-glucans. Pharmaceutics 15, 1615. doi: 10.3390/pharmaceutics15061615
Zhu W., Wei Z., Xu N., Yang F., Yoon I., Chung Y., et al. (2017). Effects of Saccharomyces cerevisiae fermentation products on performance and rumen fermentation and microbiota in dairy cows fed a diet containing low quality forage. J. Anim. Sci. Biotechnol. 8, 1–9. doi: 10.1186/s40104-017-0167-3
Zhu W., Zhang B. X., Yao K. Y., Yoon I., Chung Y. H., Wang J. K., et al. (2016). Effects of Supplemental Levels of Saccharomyces cerevisiae Fermentation Product on Lactation Performance in Dairy Cows under Heat Stress. Asian-Australas J. Anim. Sci. 29, 801–806. doi: 10.5713/ajas.15.0440
Keywords: postbiotic, Saccharomyces cerevisiae fermentation product, immunomodulation, immune response, cattle
Citation: Chae J-B, Schoofs AD and McGill JL (2024) Beneficial effects of Saccharomyces cerevisiae fermentation postbiotic products on calf and cow health and plausible mechanisms of action. Front. Anim. Sci. 5:1491970. doi: 10.3389/fanim.2024.1491970
Received: 05 September 2024; Accepted: 31 October 2024;
Published: 20 November 2024.
Edited by:
Mohan Mondal, ICAR-National Dairy Research Institute, Eastern Regional Station, IndiaReviewed by:
Christi L. Swaggerty, Agricultural Research Service (USDA), United StatesPoulad Pourazad, Delacon Biotechnik, Austria
Copyright © 2024 Chae, Schoofs and McGill. This is an open-access article distributed under the terms of the Creative Commons Attribution License (CC BY). The use, distribution or reproduction in other forums is permitted, provided the original author(s) and the copyright owner(s) are credited and that the original publication in this journal is cited, in accordance with accepted academic practice. No use, distribution or reproduction is permitted which does not comply with these terms.
*Correspondence: Jodi L. McGill, amxtY2dpbGxAaWFzdGF0ZS5lZHU=