- 1International Livestock Research Institute, Nairobi, Kenya
- 2Faculty of Agriculture and Veterinary Sciences, University of Melbourne, Melbourne, VIC, Australia
- 3Integrative Agroecology Group, Research Division Agroecology and Environment, Agroscope, Zurich, Switzerland
A study was conducted with Red Maasai and Dorper lambs to evaluate the effects of infection with the gastrointestinal nematode (GIN) Haemonchus contortus on feed intake, liveweight gain (LWG), feed energy and nitrogen partitioning, and enteric methane (CH4) emissions. Six- to seven-month-old Red Maasai (n=12) and Dorper (n=12) lambs were randomly allocated to three treatments (n=8, four lambs per breed) in a 2×3 factorial cross-over study over two periods (P1 and P2) of 36 days each. The treatments consisted of three combinations of GIN infection and feeding level: Infected + ad libitum feeding (I-adlib), uninfected + ad libitum feeding (Un-adlib), and uninfected + restricted feeding (Un-restd), across the two breeds. Lambs in the I-adlib group were trickle-infected daily with 1,000 L3 stage larvae of H. contortus for four consecutive days (Days 1–4), whereas lambs in the other experimental treatments were kept GIN free. The feed intake was measured daily. Liveweight (LW), faecal egg counts (FEC), and packed cell volume (PCV) were measured on Day 1 and weekly thereafter. On Days 29–33 total faecal and urine outputs were determined in metabolic crates. The lambs were then housed in respiration chambers for three consecutive days (Days 34–36). There was a washout period of 21 days before P2 started. Uninfected lambs (Un-adlib and Un-restd) had undetectable FEC throughout the study. On Day 36, FEC did not differ between the breeds (P>0.05). Infected lambs (I-adlib) had lower PCV than uninfected (Un-adlib and Un-restd) lambs on day 36. Neither breed nor infection influenced feed and nutrient intake, but as expected, restricted-fed lambs had a lower intake (P<0.05). The LWG of Un-adlib lambs was significantly higher than that of I-adlib and Un-restd lambs (P<0.05), whereas there was no breed difference (P>0.05). Neither breed nor infection affected feed digestibility, nitrogen retention or energy metabolisability (P>0.05). However, feed restriction decreased feed intake, LWG and N retention, whereas feed digestibility and energy metabolisability were unaffected. Neither daily CH4 emissions nor yield (per unit of feed intake) were affected by experimental infection, but Un-restd lambs had lower CH4 emissions per day. Red Maasai lambs had consistently lower daily CH4 emissions and yields than Dorper (P<0.01). This study confirmed the relative resistance of indigenous sheep (Red Maasai) to H. contortus infection, but the increased CH4 emission and yield due to GIN observed in other studies was not confirmed. Further investigations are needed to test whether in environments with multiple stress factors, local or indigenous breeds or their crossbreeds with exotic breeds may be better equipped to sustain production and simultaneously have a reduced carbon footprint than purebred exotic breeds.
1 Introduction
Globally, the livestock sector contributes 14.5% of global greenhouse gas (GHG) emissions (Gerber et al., 2013). Approximately 56% of the global domestic ruminant population are small ruminants (Marino et al., 2016). The majority of GHG emissions from livestock production is in the form of methane (CH4), which is produced largely through enteric fermentation and to a lesser extent from manure decomposition (Gerber et al., 2013). Enteric CH4 emissions also represent loss of the dietary energy consumed by the animal (Moraes et al., 2014). Depending on various factors such as feed intake and forage quality, mature ewes emit about 27.5–35.5 g of CH4/hd/day representing an energy loss of approximately 1.5–2.0 MJ/hd/day (Eckard et al., 2010). Hence, ruminant methane production not only constitutes a powerful GHG, but also represents an inefficiency of feed energy utilization by the animal.
Haemonchus contortus (H. contortus) is a parasitic nematode that affects the abomasum (fourths stomach) of sheep. It is considered the most important gastrointestinal nematode (GIN) found in sheep, causing reduction in body condition and loss in productivity, hence leading to longer periods on farm before reaching market weight and often leads to death (Besier et al., 2016). H. contortus is endemic in most parts of Kenya and it has been shown that the indigenous Red Maasai sheep breed is more resistant to H. contortus infection than the Dorper breed (introduced from South Africa) (Mugambi et al., 1996; Mugambi et al., 1997; Wanyangu et al., 1997; Baker et al., 2003; Baker et al., 2004).
Infection with gastrointestinal tract (GIT) worms affects the factors that determine CH4 production such as feed intake, rumen retention time and rumen function (Houdijk et al., 2017; Fox et al., 2018). Various studies on CH4 emission from GIT infected small ruminants have reported increased methane emissions due to infection. For example, Fox et al. (2018) reported a 33% increase in CH4 yield (emissions per kg feed dry matter intake, DMI)) from lambs infected with 7,000 Teladorsagia circumcincta larvae compared to those uninfected. Furthermore, a study by Corrêa et al. (2021) reported an increase in CH4 yield of 38% and 50%, respectively, when lambs were separately infected with 5,000 H. contortus L3 and 10,000 Trichostrongylus (T.) colubriformes L3 respectively. Likewise, Fernandes et al. (2022) reported a 37% increase in CH4 yield from lambs with a mixed infection of 10,000 H. contortus L3 and 30,000 T. colubriformis L3, whereas for similar mixed infection, Lima et al. (2019) reported up to 61% increase in CH4 yield in lambs infected with 5,000 H. contortus and 7,500 T. colubriformis L3 stage larvae. However, a study conducted by Houdijk et al. (2017) with periparturient ewes infected with the abomasal worm Teladorsagia circumcincta, showed a 10% decrease in daily enteric CH4 emission (g/day), with no effect on CH4 yield (g/kg DMI). The above-named studies, almost all, not only provide evidence for increased enteric CH4 yields due to GIT worm infection, but also for the resulting increased emission intensity compounded by the compromised animal productivity resulting from infection. Similar studies are lacking in the sub-Saharan Africa (SSA) region, and there is a lack of studies comparing different breeds that differ in susceptibility to GIT infection.
This study was conducted to evaluate the effects of GIT worm infection on methane emissions across two sheep breeds that are either more resistant or more susceptible to H. contortus. The study hypothesized that sheep infected with H. contortus would have impaired feed intake, reduced nutrient utilization and liveweight (LW) gain (LWG), and increased methane yield. It was also hypothesized that due to its higher resistance to H. contortus, the expected negative impacts on the above-mentioned parameters would be less severe in the Red Maasai sheep than the Dorper sheep breed.
2 Materials and methods
The study was conducted at the Mazingira Centre, International Livestock Research Institute (ILRI), Nairobi, Kenya. The experimental protocol was reviewed and approved by the ILRI Institutional Animal Care and Use Committee (IACUC, Reference number 2020-20).
2.1 Experimental design
The experiment was a 2×3 factorial cross-over design, involving two sheep breeds (Red Maasai and Dorper) by three combinations of GIN infection and diet (hereafter named ‘experimental treatments’): Infected + ad libitum feeding (I-adlib), uninfected + ad libitum feeding (Un-adlib), and uninfected + restricted feeding (Un-restd). Experiment was conducted over two sequential time periods (P1 and P2), all combinations of breed by experimental treatment measured in each time period. Each experimental treatment had 4 lambs of each breed (balanced for sex) making a total of 24 lambs (twelve lambs of each breed). Rhodes grass (Chloris gayana) hay of poor quality was used as feed to mimic the prevailing quality of diets for sheep in small-holder farming systems in SSA.
Each period lasted 36 days (Day 1 to 36). Lambs within each breed were randomly allocated to the three experimental treatments in P1, balanced by entry LW and sex within each breed. The first experimental period (P1) was followed by a 21-day wash out period where all the lambs were dewormed, and faecal examination was done weekly to ensure the lambs remained worm free. Afterwards, lambs were re-allocated to their new experimental treatments for P2.
In order to conduct the CH4 measurements for each animal on the same days post infection (Days 34–36) and given the availability of only three animal respiration chambers, the lambs were divided into batches of three (one lamb from each treatment), the batches were also separated by breed. Therefore, each batch had either 3 Dorper or 3 Red Maasai. The batches entered the experiments in a staggered manner, every three days.
Acclimatization to the feed, feeding and housing was conducted over the quarantine period (defined below).
2.2 Experimental animals and housing
The lambs were selected from a flock of lambs born in 2020 at Kapiti Research Station 60 km South-East of Nairobi. The lambs were six to seven months old and their LW were 25.3 ± 2.9 and 27.6 ± 3.6 kg, for Red Maasai and Dorper, respectively. On arrival at the Mazingira Centre the lambs were treated with anthelmintics (10% Albendazole), treated with a topical acaricide, ear tagged and placed under quarantine for 21 days. At the end of the quarantine period, and prior to starting P1, the lambs were randomly allocated to the experimental treatment groups and housed in two separated in-door housing units, one for the infected animals, while the other unit housed the two uninfected treatment groups. The housing provided individual pens with a feed trough and automatic drinker. Rubber mats were placed in each pen to cover the concrete floor. Feeding in pens was conducted twice daily, in two equal portions.
The lambs were housed in individual pens over Day 1 to 26 of each experimental period. On Day 27 they were transferred into metabolic crates for a seven-day period, during which faeces and urine were collected over the last five days (nitrogen and energy balance period). At the end of the collection period, the animals were transferred to the respiration chambers for the last three days of the study (Days 34–36). The lambs were then dewormed and transferred back to Kapiti Research Station.
2.3 Experimental parasite larvae preparation and infection
The lambs were sourced from semi-arid grasslands and therefore had prior exposure to Haemonchus contortus. For deworming the lambs were orally dosed with 10% Albendazole (Albafas) at a dosage of 8 mg/kg LW on the first day of quarantine. Faecal samples taken after seven days of dosing revealed that the lambs still had parasite eggs. The lambs were then drenched with Levamisole at a dose of 7.5 mg/kg LW. Seven days after drenching with Levamisole and at the start of the trial the lambs were confirmed to be worm-free.
On their respective Day 1 of the experiment, the lambs in the infected experimental treatment group (I-adlib) were dosed orally with 1,000 L3 stage H. contortus larvae suspended in distilled water. Dosing was carried out over four consecutive days to achieve a total larval dose of 4,000 larvae. At the same time, the respective lambs in the two uninfected treatments were dosed with 10 ml of clean water, as a placebo control.
The larvae used for infection were prepared a month prior to the experiment by infecting five adult Red Maasai ewes as donors managed in a pen as a single group. These donor ewes were first dewormed by orally dosing them with 10% Albendazole at a dosage of 8 mg/kg LW. Once confirmed to be worm-free through faecal examination, one ewe was dosed with 4,000 H. contortus L3 stage larvae suspended in distilled water, while two ewes each were dosed with 6,000 and 8,000 H. contortus L3 stage larvae, respectively. After a 21-day prepatent period, a faecal bag was put on the donor ewes for total faeces collection. The collected faeces were mixed with vermiculite and water to achieve a dough like consistency, the mixture was put in 1,000 ml glass jars to fill approximately a quarter of the jar height from the bottom. The jars were loosely closed to allow air to flow in and incubated at 27°C in a thermostatically controlled cabinet (Lovibond, Germany) for larvae production. Once the larvae hatched, they crawled up the side of the jar and were visible as white lines. Larvae were washed off the side of the jar with distilled water into 160 ml plastic cell culture flasks. Each flask was topped up with distilled water to a maximum of 60 ml and stored in a regular refrigerator at 4°C until use. The viability of the larvae was confirmed under a dissection microscope (Zeiss, Model Axiocam ERc 5s, Germany) every morning before dosing the lambs in the I-adlib treatment group.
The L3 larvae doses were calculated by transferring 12 aliquots (0.1 ml each) of the distilled water containing the L3 larvae onto a petri dish and counting the number of viable L3 larvae in each aliquot and recorded. Then, the lowest and highest count aliquots were discarded, and the total of the remaining 10 aliquots calculated to get the number of L3 larvae per 1 ml. Using this figure, the amount of larvae filled distilled water for dosing the required 1000 L3 per animal was calculated. The donor ewes were not used for the experiment.
2.4 Feed and feeding
The experimental animals were fed solely on chaffed Rhodes grass (Chloris gayana) hay. The hay was sourced from a single plot on a commercial farm. The dry matter (DM) content of the hay was 92.1 ± 0.59%; whereas on a DM basis the hay contained 90.1 ± 0.74 9.9 ± 0.72% ash, 7.2 ± 0.56% crude protein (CP), 68.8 ± 1.74% neutral detergent fibre (NDF), 34.9 ± 1.29% acid detergent fibre (ADF), and 17.4 ± 0.22 MJ gross energy (GE) per kg DM (number of samples=12).
Lambs in the ad libitum feeding groups (I-adlib and Un-adlib) were offered 110% of voluntary feed intake (DM basis) using average feed intake per animal as measured during the acclimatization period, whereas the lambs within the restricted feeding level treatment (Un-restd) were offered 80% of their ad libitum feed on offer as measured during the same period. To avoid feed wastage through spillage, the lambs were fed in two equal portions, in the morning (08:30) and afternoon (16:30). Water was supplied ad libitum and a mineral block provided free choice.
2.5 Measurements and sample collection
2.5.1 Parasitological examination and packed cell volume
On Day 1 of the experiment, and thereafter on a weekly basis, before the morning feeding, a faecal sample was collected directly from the rectum from each experimental animal. Samples were collected into plastic collection bottles. Quantitative examination of faecal egg counts (FEC) was done using a modified McMaster technique (Whitlock, 1948). Parallel to faecal sampling, a blood sample was collected by jugular venepuncture in EDTA tubes for packed cell volume (PCV) determination using a microhematocrit centrifuge (Heraeus Sepatech, model 184587, Germany) at 14,489 g for five minutes.
2.5.2 Feed intake
Animals were fed individually according to their level of feeding (ad libitum or restricted). A sample of the feed on offer was collected daily at the time of weighing of the meals, stored at -20°C, with daily samples pooled weekly and stored at -20°C to await DM determination on a weekly-pooled sample. Refusals were collected daily per animal before the morning feeding.
Daily feed intakes by individual animals were calculated (on a DM basis) as the difference between feed on offer and refusals. Feed intakes were calculated separately for Days 1–26 when lambs were kept in individual pens, Days 27–33 corresponding to the balance period (total urine and faeces collection), and Days 34–36 when lambs were kept in respiration chambers.
2.5.3 Liveweight
Liveweight (LW) measurements were taken before the morning feedings on Day 1 and then weekly thereafter until the end of the experimental period (Day 36). A digital weighing scale was used for this purpose (YH-T3 Tscale Electronics MFG, Model KW; Kunshan, China).
2.5.4 Total faeces and urine outputs (balance period)
Total collection of daily outputs of faeces and urine were conducted while animals were in metabolic crates over Days 27–33. The first two days were used to acclimatize the sheep to the crates, while the final five days were used for collection. The crates allowed the separate collection of faeces and urine. The faeces fell through the crate floor and retained on a mesh placed underneath, while urine was allowed to pass the faeces mesh and then channeled through a Perspex tray to a collection bottle containing 70 ml 10% HCl to enable urine pH to drop to <3 at the end of the 24-h collection periods. During collection, the urine tray was sprayed with clean water five times a day (during daylight) to avoid urine drops losing N. Approximately 0.5 L of water was used for this purpose. Water addition was not measured as total volume of urine+water was measured as well as N and GE concentration in the mixture.
Daily faeces and urine outputs were collected immediately before the morning feeding. Faecal outputs from each individual animal were weighed, a 50 g subsample collected in a polythene zip lock bag and stored at -20°C. Later, the samples were pooled within animal over the 5-day period and stored back (-20°C) awaiting DM determination and analyses of chemical composition and GE contents. At collection, daily urine outputs were sieved and then measured for total volume. A 40 ml subsample of the daily urine output (+ sprayed water) was taken and stored in an airtight bottle at -20°C awaiting analyses of nitrogen and GE contents in within-animal pooled samples.
2.5.5 Enteric methane emission
Methane emissions from each individual animal were measured over three consecutive days (Days 34–36). Three open-circuit respiration chambers were used for the purpose. During measurement, each individual animal rotated through the three respiration chambers (one day per chamber). On each day, the measurements were carried out for approximately 23.5 h, with a 30-minute period used for feeding and watering (morning and afternoon) and cleaning (before morning feeding). Feeding and watering in respiration chambers were carried out as in the metabolism crates.
The respiration chamber system used in this study and the internal crates used to contain the animals inside the chambers are similar to those described by Pinares-Patiño et al. (2013), except that the chambers used in the present experiment share a common air conditioning system and have a volume of 2.5 m3. In the chambers, the sheep were held in metabolic crates that have provision for holding a feed bin as well a drinking water container. The respiration chambers are placed side by side so that the sheep can see each other through the Perspex walls. During measurement, the internal environment of the chambers was set at 22°C and relative humidity of 47%. Ventilation rate of the chambers was set to 8 l/s. A cavity ringdown laser absorption spectrometer (Picarro G2508 analyzer, Santa Clara, USA) was used to measure the CH4 concentrations (ppm) at intervals of approximately five minutes (including the incoming background gas sample).
A whole-system recovery of known volumes of pure CH4 into the system was carried out before the start and after of the methane measuring period using a gas phase titration unit (Environics 4020, Environics Inc., Tolland, USA). This test yielded a recovery rate of 98–106%.
2.5.6 Rumen sampling and pH measurement
Following the last day in the respiration chambers, rumen samples were collected from each sheep using an orogastric tube at 09:00 am in the morning before feeding. With the animal properly restrained, and the neck extended forward, a speculum made from a PVC tube was placed in the mouth. The flexible orogastric tube was gently passed through the speculum to the base of the tongue and while the animals were allowed to swallow it, the tube was slowly pushed through the esophagus and into the rumen. Once in the rumen, sampling was done using a pump connected to the tube. The first 10–20 ml of rumen sample collected were discarded, and a second sample (~40 ml) was taken for which the pH was measured immediately using a pH and conductivity meter (Jenway 3540 model).
2.6 Laboratory analyses
Samples of feed on offer (pooled on a weekly basis) and within-animal pooled samples of feed refusals and faeces were oven-dried (Genlab oven, Model SDO/425/DIG, UK) at 50°C for 72 h. The dried samples were ground through a five mm sieve using a clean dry Wiley mill (Model MF 10B, IKA Werke, Willmington, N.C., USA) and then passed through a hammer mill (MF 10 basic, IKA, Werke GmbH & CO. KG, Staufen, Germany) fitted with a 1 mm sieve. True DM was determined by drying the samples at 105°C in an oven (Genlab oven, model SDO/425/TDIG, UK) for 24 h while ash was determined by combustion in a muffle furnace (Nabertherm GmbH, Germany) at 550°C for six hours according to the methods of the Association of Official Analytical Chemists (AOAC, 1990 methods no. 924.05). Feed, refusals, and faecal samples were analyzed for NDF and ADF by the methods of Van Soest et al. (1991) using an Ankom 200 fibre analyzer, model A2001, USA. Total N content in urine samples was determined by micro-Kjeldhal procedure (AOAC 1990, method no. 988.05). Total N content in feed, refusals, and fecal samples was determined with an organic elemental analyzer (Vario Max, model vario SOLID Sampler, Germany)
Gross energy (GE) contents of feed on offer, feed refusal and faeces were determined according to the methods by Harris (1970) using a bomb calorimeter (Parr 6200, model A1290DDEE, UK). Gross energy contents of urine samples were determined by a method described by Zhao et al. (2016) where the urine was soaked in a filter paper whose GE was predetermined, the soaked filter paper was dried, weighed and its GE determined in a bomb calorimeter. To get the urine GE, the GE value of the filter paper was subtracted from the GE value of the urine-soaked filter paper.
2.7 Calculations
Feed intakes were calculated for the periods when animals were in individual pens, metabolic crates, and respiration chambers. Daily feed intakes were expressed on daily basis (g/d) as well as a g/kg metabolic LW (g/kg LW0.75)
Apparent total tract digestibility (%) of feed DM and its constituents (OM, N, NDF, ADF, GE; DM basis) were calculated from the ingested and faecal excretions of each item during the feed digestibility and balance period (Days 29–33). For the same period, the nitrogen balance (retained N) was calculated by subtracting the amounts excreted in faeces and urine from the amount ingested. Nitrogen balance was expressed as g N/d as well as % of the N ingested.
Daily metabolizable energy (ME) intake was calculated by subtracting the energy outputs in faeces, and urine (determined for the balance period) and the energy lost in CH4 calculated using the energy conversion factor (Ym, MJ CH4/MJ GEI) determined during the chamber period.
Average daily liveweight gain (LWG, g/d) was calculated by subtracting the LW measured on Day 1 from that on Day 27 (morning) and dividing the value by 26 to represent the days in individual pen, while the whole period LWG was calculated by subtracting the LW measured on Day 1 from that on Day 37 (morning) and dividing the value by 36.
Measurements of daily CH4 emissions were conducted over approximately 23.5 h, and mathematically extrapolated to 24 h. Given that in each period each animal was measured thrice (one day per each chamber), the average daily emissions from each animal were calculated averaging the emissions over the three days. Calculations of the daily emissions in each chamber took into account the ventilation rate and net concentrations at each data point, the gas conditions, and the gas recovery rate. Daily emission of CH4 was expressed on an absolute basis (g/d), CH4 yield (g/kg dry matter intake, DMI; g/kg organic matter intake, OMI; g/kg digestible DMI, DDMI and g/kg digestible OMI, DOMI), as well as Ym (CH4 energy/GEI).
2.8 Data analysis
Data were analysed using a linear mixed model in R 4.1.2 (R Development Core Team, USA). The dependent variables measured were first tested for normality by plotting normal quantile-quantile plots in R and visual inspection of the plots for normal distribution of data conducted. The model for each variable was fitted using “lme4” package in R with Breed (B), Experimental treatment (T), Period (P), and interactions of B×T, P×B, P×T and P×B×T as fixed effects and animal identity and batch number as random effect. ANOVA type 3 analysis was used to test for significance of the fixed factors. Least square means were calculated using package “lsmeans” package and Tukey’s built in “Multicompview” package in R was used to separate the means per treatment. The significance level was determined at (P<0.05).
Statistical analysis had two limitations caused by an imbalance in the crossover design. First, from Period 1 of the study (P1), lambs in treatment groups were not equally re-distributed to the alternate treatments for Period 2 (P2); i.e., four lambs in I-adlib group during P1 would ideally have two lambs moved to Un-adlib, and two to Un-restd for P2, but this was not the case except for Dorper Un-adlib treatment in P1. Secondly, one Dorper lamb was mistakenly allocated to the Un-restd treatment group in both periods. These two limitations mean that statistical estimation of treatment × period effect (carry-over effect) could not be efficiently measured. Where the carry-over effect was significant in the models the paper reports in the results and discusses why it should not alter the outcomes and conclusions of the experiment.
3 Results
There were no significant Breed×Experimental treatment effects for any of the measured variables (P>0.05).
3.1 Packed cell volume and faecal egg counts
At the start of the study (Day 1), the packed cell volume (PCV Day 1) did not differ between experimental treatments (P>0.05). At this time, Dorper lambs had lower PCV than their Red Maasai counterparts, the difference approaching statistical significance (P=0.06) (Table 1). There was a significant period effect (P<0.05) on PCV Day 1, with lambs at start of P2 having higher PCV than at start of P1 (31.2 vs 27.5%). At the end of the study (Day 36) the PCV of infected lambs (I-adlib) was significantly lower (P<0.01) than those of uninfected lambs (Un-adlib and Un-restd) (Table 1) and Dorper lambs continued having lower PCV than Red Maasai (P<0.05). There was a significant Experimental treatment×Period interaction (P<0.05) on PCV Day 36, where the uninfected lambs had higher PCV in P2 than P1 (Un-adlib 29.8 vs 25.7%; Un-restd 30.7 vs 25.9%), whereas the infected (I-adlib) lambs had similar values of PCV Day 36 (P>0.05) in both P2 and P1 (22.5 vs 21.0%).
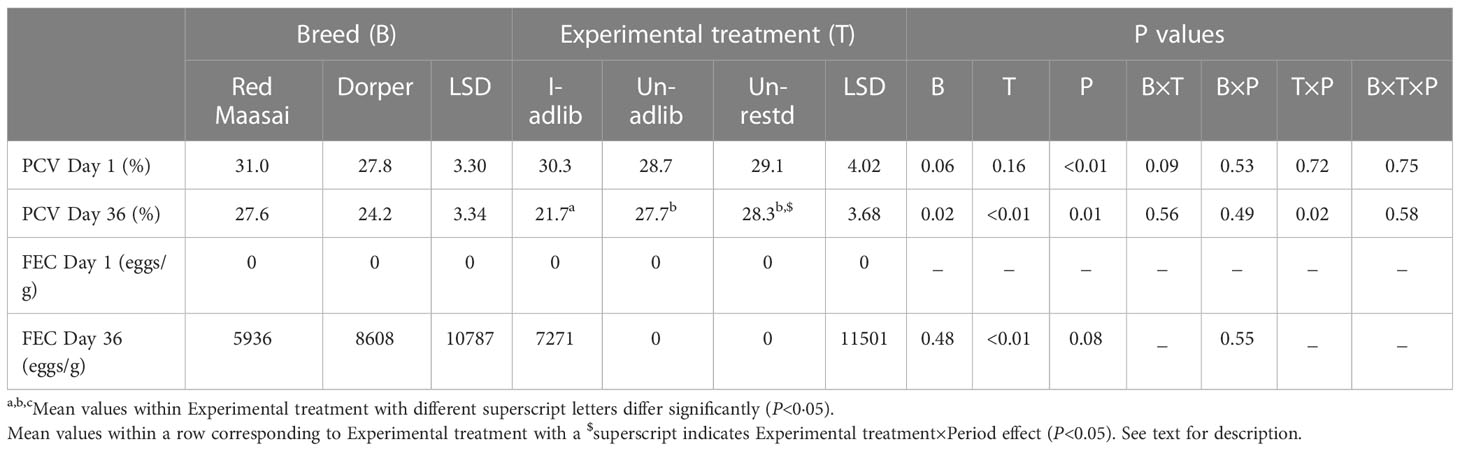
Table 1 Mean ( ± least significant difference, LSD) packed cell volume (PCV, %) and faecal egg counts (FEC, eggs/g) of H. contortus on Day 1 and Day 36 post infection for Red Maasai and Dorper lambs infected with H. contortus and fed ad libitum (I-adlib), and uninfected and fed ad libitum (Un-adlib) or restricted (Un-restd).
None of the experimental animals had parasite eggs in faeces on Day 1 of the experiment (FEC Day 1). As expected, experimental treatments differed in FEC at Day 36 (P<0.01). Uninfected lambs (Un-adlib and Un-restd) had no parasite eggs in their faeces. The faecal egg count (FEC) of infected lambs (I-adlib) did not differ with breed (P>0.05) at any point of the measurement period. On Day 36, infected Dorper lambs had only a numerically higher (P>0.05) FEC than their Red Maasai counterparts (Table 1).
The presence of H. contortus eggs in faeces and drop in PCV in the infected lambs (I-adlib) indicate that parasitic infection was achieved, while lack of eggs in the faeces of uninfected lambs indicates that they were worm-free.
3.2 Feed dry matter intake, liveweight and liveweight gain
Table 2 shows feed dry matter intakes (DMI) during the individual pen period (Days 1−26). Infection did not affect DMI (P>0.05). As expected, daily feed intakes (expressed either as g/d or per kg metabolic LW) of lambs fed ad libitum (I-adlib and Un-adlib) were higher than those of restricted-fed lambs (Un-rest) (P<0.01). There was no breed effect on feed DMI (P>0.05). However, there was a Breed×Period interaction effect on daily DMI (g/d), with Dorper lambs having higher intakes in P1 than in P2 of the study (744 vs 672 g/d; P<0.05), whereas DMI by Red Maasai were maintained across the two periods. The Breed×Period effect on feed DMI per kg of metabolic LW had similar trend as for when expressed as g/d and approached statistical significance (P=0.06).
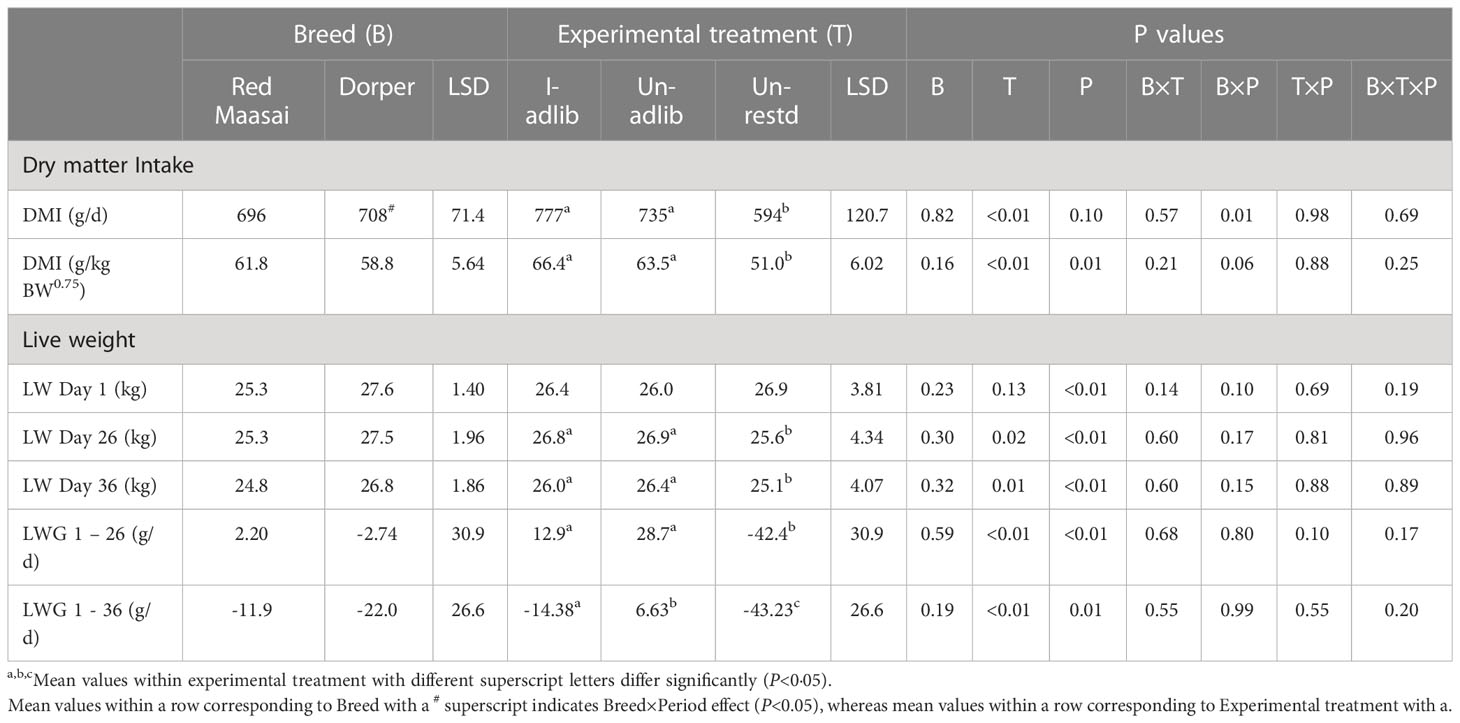
Table 2 Mean ( ± least significant difference, LSD) feed dry matter intake (DMI, daily and per unit metabolic LW (LW0.75)) over the individual pen period (Days 1−26) and liveweight (LW) and LW gain (LWG) over the individual pen period (Days 1−26) and the whole experiment (Days 1−36) for Red Maasai and Dorper lambs infected with H. contortus and fed ad libitum (I-adlib), and uninfected and fed ad libitum (Un-adlib) or restricted (Un-restd).
The liveweight of lambs at the start of the study (LW Day 1) and the subsequent LWs (LW Day 26 and LW Day 36) did not differ between breeds (P>0.05) (Table 2). Experimental treatments did not differ in LW measured at Day 1 (P>0.05), however, there was a significant treatment difference on Day 26 (LW Day 26) and on Day 36 (LW Day 36), with ad libitum fed lambs (I-adlib and Un-adlib) being significantly heavier (P<0.05) than lambs restricted-fed (Un-restd) (Table 2).
The experimental treatments differed (P<0.01) in LWG by lambs over the first 26 days of study (LWG 1−26) as well as over the entire period of study (LWG 1−36) (Table 2). Over Days 1−26 (LWG 1−26), restricted-fed lambs (Un-rest) had negative LWG, whereas ad libitum fed lambs (I-adlib and Un-adlib) had positive and similar LWG (P>0.05). Over Days 1−36, the LWG of lambs in all the experimental treatments differed from each other (P<0.05). The restricted-fed lambs continued losing LW. Over this same period infected lambs had negative LWG, whereas Un-adlib lambs had a smaller positive LWG.
here were significant period effects (P<0.01) on LW and LWG at all the measuring times (Table 2). Liveweight of lambs were higher in P2 than in P1 (26.9 vs 25.9 kg, 27.3 vs 25.6 kg, and 26.7 vs 25.0 kg, for LW Day 1, LW Day 26 and LW Day 36, respectively). Similarly, LWG in P2 were higher (P<0.01) than in P1 (12.1 vs -12.7 g/d and -6.88 vs -27.11 g/d for LWG 1−26 and LWG 1−36, respectively) (Table 2).
3.3 Nutrient intakes in feed, apparent feed digestibility, and nitrogen and energy partitioning (balance period)
Feed dry matter intake (DMI, g/day and g/kg LW0.75) during the balance period (Days 29−33) (Table 3) followed a similar pattern observed during the previous period (Days 1−26) (Table 2); i.e., experimental treatments differed significantly in DMI (P<0.01), whereas breeds did not (P>0.05). The daily intakes of feed chemical constituents (OM, CP, NDF and ADF) followed the pattern of DMI (P<0.01). As expected, the restricted-fed lambs (Un-restd) had lower intakes of DMI and chemical constituents (g/d) than their ad libitum fed counterparts (I-adlib and Un-adlib) (P<0.01). Overall, infection did not influence feed intake during the balance period (P>0.05) (Table 3). Breeds did not differ (P>0.05) in daily intakes of chemical constituents of feed (Table 3). However, there was a significant period effect on DMI per kg of metabolic liveweight (g/kg LW0.75), with lambs in P1 having larger DMI intakes than in P2 (60.4 vs 55.2 g/kg LW0.75). The effect of period on DMI (g/d) approached statistical significance (P=0.06). There was a significant effect of period (P<0.01) on the intake of crude protein (CPI), being this larger in P1 than in P2 (53.9 vs 49.5 g/d). There were significant Breed×Period effects (P<0.05) on intakes (g/d) of NDF (NDFI) and ADF (ADFI) (Table 3), with Dorper lambs having larger NDF (483 vs 444 g/d) and ADF (236 vs 227 g/d) intakes in P1 than in P2, whereas Red Maasai maintained similar intakes in both periods.
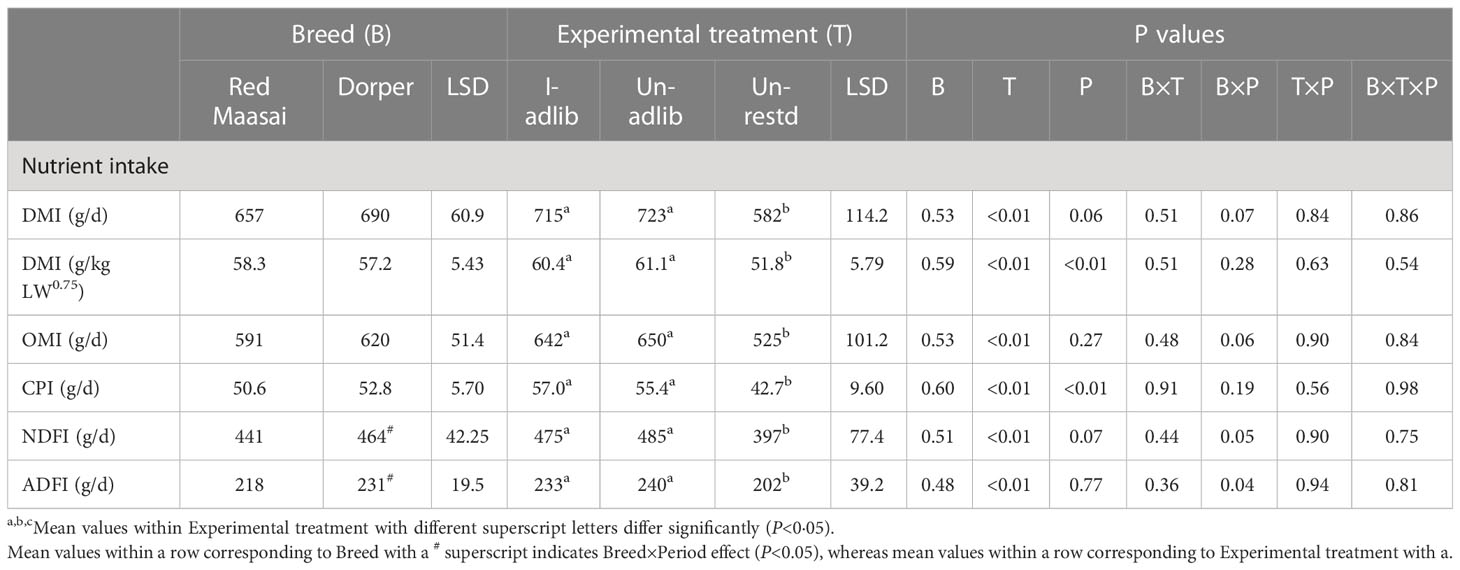
Table 3 Mean ( ± least significant difference, LSD) feed dry matter intake (DMI) and intakes of organic matter (OMI), crude protein (CPI), neutral detergent fibre (NDFI) and acid detergent fibre (ADFI) by Red Maasai and Dorper lambs infected with H. contortus and fed ad libitum (I-adlib), and uninfected and fed ad libitum (Un-adlib) or restricted (Un-restd) during the balance period (Days 29−33).
Neither experimental treatments nor breeds differed in the digestibilities of feed dry matter (DM) or its chemical constituents (OM, CP, NDF, ADF and GE) (P>0.05) (Table 4). However, there were significant period effects (P<0.01) on digestibilities of DM (DMD), CP (CPD) and ADF (ADFD) (Table 4), these values being higher in P2 than in P1 (68.7 vs 63.5%, 56.2 vs 51.4% and 67.2 vs 63.5%, for DMD, CPD and NDFD, respectively). Nevertheless, for the CPD there was Experimental treatment×Period interaction effect (P=0.01), with lambs in the ad libitum feeding treatments having higher CPD in P1 than in P2 (59.6 vs 50.2% and 57.3 vs 49.3%, for I-adlib and Un-adlib, respectively) (P<0.05), whereas the corresponding CPD for lambs within the Un-rest treatments were only numerically different between periods (51.7 vs 54.4%, respectively). Experimental treatment×Period interaction effect on gross energy digestibility (GED, %) approached statistical significance (P=0.06), showing a trend similar to that of CPD (Table 4).
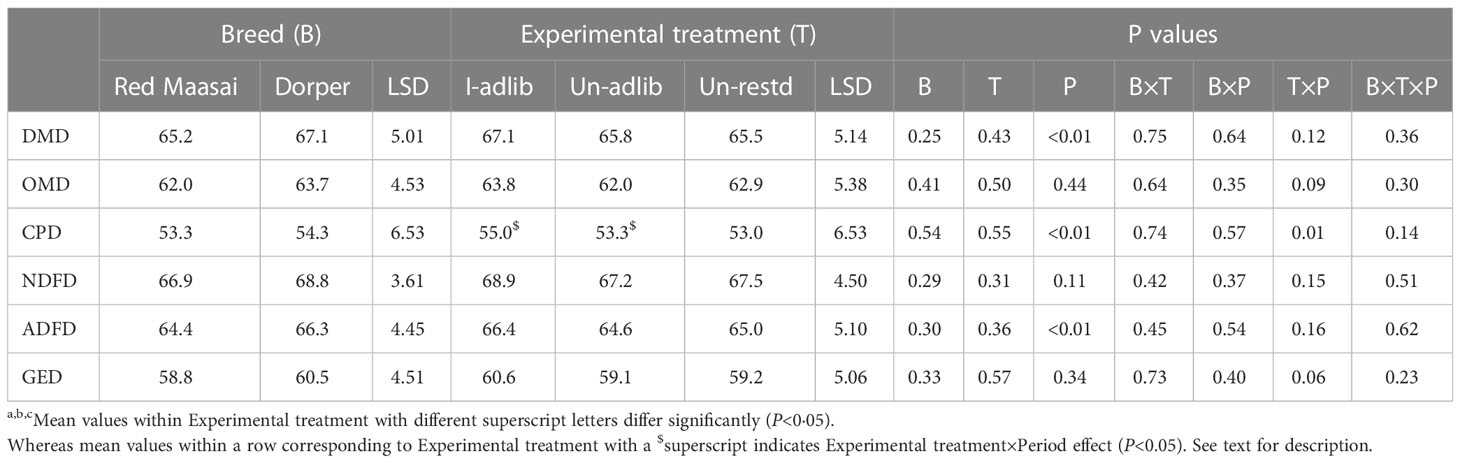
Table 4 Mean ( ± least significant difference, LSD) apparent nutrient digestibilities (%) of dry matter (DMD), organic matter (OMD), crude protein (CPD), neutral; detergent fibre (NDFD), acid detergent fiber (ADFD) and gross energy (GED) by Red Maasai and Dorper lambs infected with H. contortus and fed ad libitum (I-adlib), and uninfected and fed ad libitum (Un-adlib) or restricted (Un-restd) during the balance period (Days 29−33).
During the balance period, the daily intakes of nitrogen (NI) by lambs fed ad libitum (I-adlib and Un-adlib) were larger than those of restricted-fed (Un-Restd) lambs (P<0.05), but no effect of infection was observed (P>0.05) on NI; i.e., there were no differences between lambs in I-adlib and Un-adlib treatments (Table 5). Breeds did not differ in NI or N partitioning during the balance period (P>0.05) (Table 5).
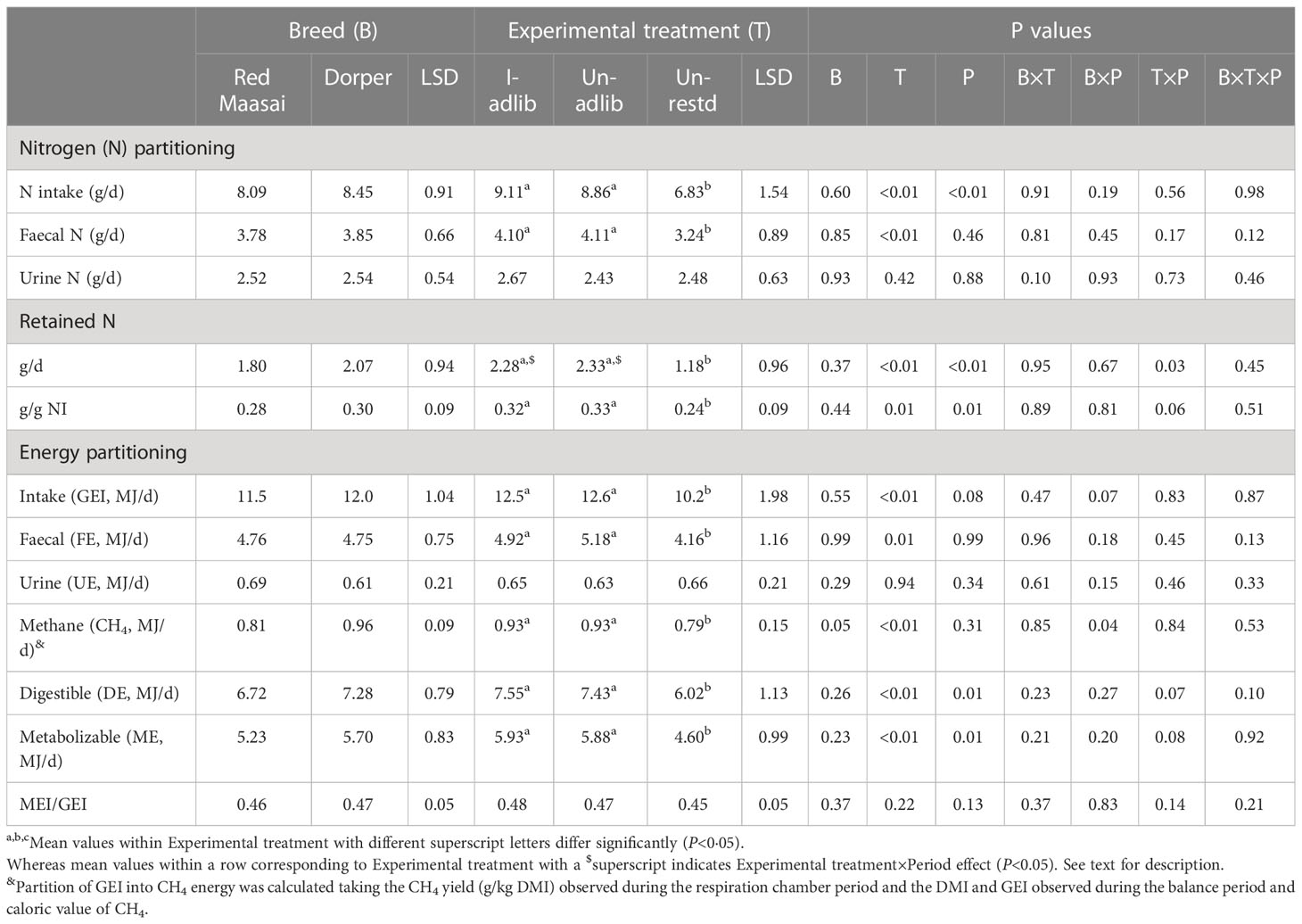
Table 5 Mean ( ± least significant difference, LSD) intakes of nitrogen (NI) and gross energy (GEI) and their partitioning by Red Maasai and Dorper lambs infected with H. contortus and fed ad libitum (I-adlib), and uninfected and fed ad libitum (Un-adlib) or restricted (Un-restd) during the balance period (Days 29−33).
Experimental treatments differed in faecal N output (P<0.05) but not in urinary N (P>0.05) (Table 5), with ad libitum fed lambs having larger faecal N outputs than restricted fed lambs (P<0.05); whereas faecal N output was not affected by infection status (P>0.05). The effect of experimental treatments on retained N (g/d and g/g NI) followed the same pattern as for NI and faecal N output, with no effect of infection status (P>0.05) (Table 5). There was a significant period effect (P<0.01) on NI, with intakes being higher in P1 than in P2 (8.62 vs 7.92 g/d). Similarly, retained N in P1 also was higher (P<0.01) in P1 than in P2 (2.34 vs 1.53 g/d and 0.33 vs 0.26 g/g NI). Most importantly, there was Experimental treatment×Period interaction effect (P<0.05) on retained N (g/d), with lambs within the I-adlib treatment having higher retained N in P1 than in P2 (3.01 vs 1.60 g/d). This pattern in I-adlib lambs also approached statistical significance (P=0.06) when retained N was expressed as g/g NI (0.32 vs 0.19 g/g NI for P1 and P2, respectively). Lambs in the Un-adlib treatment also had similar pattern of difference between periods in retained N as those observed for I-adlib lambs (P<0.05) (2.88 vs 1.77 g/d and 0.30 vs 0.21 g/g NI), whereas retention of N by lambs fed at restricted level remained unchanged.
The daily intakes of gross energy (GEI, MJ/d) by lambs fed ad libitum (I-adlib and Un-adlib) were larger than those of restricted-fed (Un-Restd) lambs (P<0.05), but again no effect of infection was observed (P>0.05) on GEI (Table 5). Breeds did not differ (P>0.05) in GEI or energy partitioning, digestibility or metabolisability, except that the calculated CH4 energy output was higher (P=0.05) in Dorper than in Red Maasai (Table 5). Experimental treatments differed (P<0.01) in partitioning (MJ/d) of GE into faecal energy (FE), calculated CH4 energy, digestible energy (DE) and metabolizable energy (ME), but not in urinary energy (UE) or metabolisability of energy (MEI/GEI) (Table 5). Overall, restricted fed lambs (Un-restd) had lower partitioning (P<0.05) than ad libitum fed lambs (I-adlib and Un-adlib), with no effect (P>0.05) of infection (Table 5).
There was a significant period effect (P<0.05) on DE and ME partition (Table 5), with values in P1 being higher than in P2 (7.23 vs 6.77 MJ/d and 5.71 vs 522 MJ/d for DE and ME, respectively). There was as a significant Breed×Period interaction effect (P<0.05) on the calculated CH4 energy output (Table 5, with Dorper lambs having higher outputs in P1 than in P2 (0.99 vs 0.93 MJ/d).
3.4 Enteric methane emission
There was effect of experimental treatments (P<0.01) on daily CH4 emissions (g/d), with emission from ad libitum fed lambs (I-adlib and Un-adlib) being higher than those from restricted fed lambs (Un-restd), but there was no effect (P>0.05) of infection on daily CH4 emission (g/d) (Table 6). On the other hand, treatments did not differ (P>0.05) in CH4 yield (emissions per kg of intake of dry matter (DMI), organic matter (OMI), digestible DMI (DDMI), digestible organic matter intake (DOMI)) or CH4 conversion factor (Ym, MJ CH4/MJ GEI) (Table 6). Breeds differed (P<0.05) in daily CH4 emissions (g/d) and CH4 yields per kg DMI and OMI, and Ym, with Dorper lambs having higher emissions of CH4 than their Red Maasai counterparts (Table 6). Dorper lambs also tended to have higher CH4 yields (P<0.08), when emissions were expressed per kg of digestible DMI (DDMI) and digestible OMI (DOMI) (Table 6).
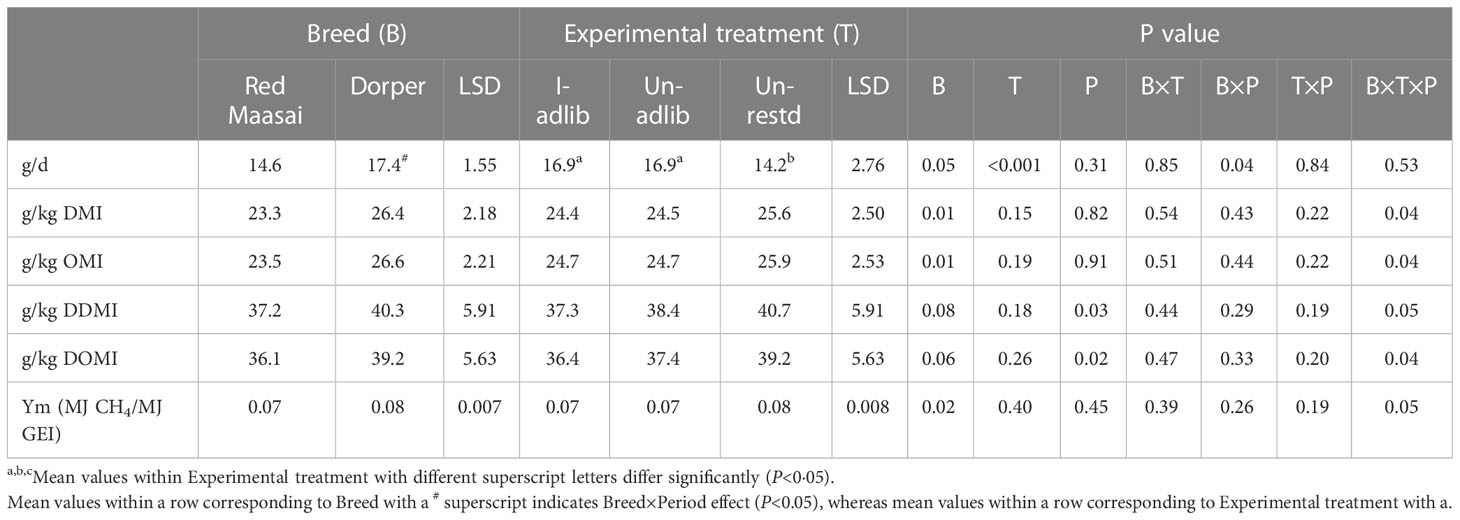
Table 6 Mean ( ± least significant difference, LSD) daily methane emission (g/d), methane yield (expressed per kg of intake of dry matter (DMI), organic matter (OMI), digestible DMI (DDMI), digestible organic matter intake (DOMI)) and methane conversion factor (Ym, MJ CH4/MJ GEI) by Red Maasai and Dorper lambs infected with H. contortus and fed ad libitum (I-adlib), and uninfected and fed ad libitum (Un-adlib) or restricted (Un-restd) during the balance period (Days 29−33).
There was a significant Breed×Period effect on daily CH4 emissions (g/d) (P<0.05), with Dorper lambs having higher emissions in P1 than in P2 (18.0 vs 16.8 g/d), while emissions from Red Maasai were similar between periods (14.4 vs 14.8 g/d). There were period effects on CH4 yields expressed per kg of DDMI and DOMI. Emissions being higher in P1 than in P2 (40.5 vs 37.0 g/kg DDMI and 39.4 vs 35.8 g/kg DOMI).
There was significant Experimental treatment×Breed×Period interaction effects (P<0.05) on CH4 yields (emissions per kg DMI, OMI, DDMI, DOMI) and CH4 conversion factor (Ym). Daily CH4 yields per unit of DMI and OMI by infected (I-adlib) Red Maasai lambs in P1 (20.7 g/kg DMI and 20.8 g/kg OMI) were significantly lower than those of Infected (I-adlib) Dorper lambs in P2, Un-adlib Dorper lambs in P2 and Un-restd Dorper lambs in both P1 and P2 (27.0 and 27.3, 26.6 and 26.9, 27.1 and 27.4, and 26.5 and 26.5 g/kg DMI and OMI, respectively). In the same line, Daily CH4 yields per unit of DDMI and DOMI by infected (I-adlib) Red Maasai lambs in P1 (29.2 g/kg DDMI and 28.5 g/kg DOMI) were significantly lower than those of Un-restd Red Maasai lambs in P1, Un-adlib Dorper lambs in P1 and Un-restd Dorper lambs in P1 (43.8 and 42.0, 41.5 and 40.7, and 44.0 and 42.6 g/kg DDMI and g/kg DOMI, respectively). The CH4 conversion factor (Ym) by I-adlib Red Maasai lambs in P1 (0.063) was also significantly higher than the Ym of I-adlib Dorper lambs in P2 and Un-adlib Dorper lambs in P2 (0.083 and 0.081, respectively).
3.5 Rumen pH
The pH of rumen fluid was found in a narrow range (6.8-6.9) with no difference between treatments and breeds.
4 Discussion
4.1 Effects of GIN infection and breed on feed intake, LWG, feed digestibility, nitrogen retention and diet metabolisability
The PCV and FEC are preferred indicators of gastrointestinal nematode (GIN) infection (Xiang et al., 2021). In this study, the lower PCV and higher FEC observed in infected lambs (I-adlib) compared to those of uninfected counterparts (Un-adlib and Un-restd) indicates clearly that parasite infection was achieved. However, at the beginning of the experiment infected Dorper lambs exhibited lower PCV levels compared to their Red Maasai counterparts, and this pattern was maintained throughout the study. In addition, following the two weeks post-infection, FEC increased at a faster rates in infected Dorper lambs than in their Red Maasai counterparts, resulting in 45% higher FEC in Dorper lambs at the end of the study (Day 36). However, this difference was not statistically significant. The above is in line with findings from previous studies (Mugambi et al., 1996; Mugambi et al., 1997; Wanyangu et al., 1997), which reported Red Maasai sustaining higher PCV and lower FEC than Dorper. Nevertheless, breeds did not differ in feed intake, LWG feed digestibility, N retention or metabolisability of feed energy.
According to Alba-Hurtado and Muñoz-Guzmán (2013), sheep can be susceptible, resistant or resilient to H. contortus infection and animals can be found anywhere in the combination of resilience (low–high) × resistance (low–high) quadrants (Blackburn et al., 2013). Resistant animals, based on their innate and acquired immunological capabilities, have lower parasitic load than susceptible counterparts; whereas resilient animals are able to perform in the face of parasite infection, compensating for the negative consequences of infection (Alba-Hurtado and Muñoz-Guzmán, 2013; Blackburn et al., 2013). Improved feed efficiency and protein synthesis are the mechanisms by which susceptible animals display resilience (Doyle et al., 2011). There is general agreement (Alba-Hurtado and Muñoz-Guzmán, 2013; Albuquerque et al., 2019) that native breeds are resistant due to their indirect selection in the prevailing GIN endemic environment, with no treatments against helminths. This is supported by Mugambi et al. (1997) who found that Red Maasai sheep are resistant to H. contortus infection, whereas Dorper sheep were susceptible.
The damage caused by haemonchosis is associated with the parasites blood-feeding activity, a destruction of the abomasal mucosa and increased mucus production (Ramos-Bruno et al., 2021). These events affect feed digestibility and the absorption of nutrients, hence reducing feed conversion efficiency (Hoste et al., 2016). Gastrointestinal parasitism, such as haemonchosis, is also associated with anorexia (Kyriazakis, 2014), which degree of presentation can be affected by the level of infection, species of parasite, site of infection, and animal breed, age and resistance (Sahoo and Khan, 2016). Blood loss, depressed feed intake, digestion and absorption, all affect animal performance. However, further reductions in performance due to H. contortus infection are associated to the nutritional cost of infection; i.e., the use of macro- and micro-nutrients required for the repair of damaged tissues and the setting of the immune response (Sahoo and Khan, 2016; Méndez-Ortíz et al., 2019; Ramos-Bruno et al., 2021). A recent study conducted by Xiang et al. (2021) concluded that lambs infected by H. contortus had decreased feed intake and digestibilities (especially of protein and lipids), reduced LWG, disturbed protein absorption, altered plasma amino acid profiles, and changes in the gastrointestinal microbial community composition. In the present study, however, no effect of parasite infection on feed intake, feed digestibility, nitrogen retention or diet metabolisability was found; neither was the rumen pH altered (6.8–6.9).
Unlike other GIN infections, such as Teladorsagia (T.) circumcincta (Fox et al., 2018), that induce a persistent reduction in voluntary feed intake, the effect of H. contortus on feed intake seems much less pronounced and transient (Sykes and Coop, 1977; Coop et al., 1982; Kyriazakis et al., 1996; Hoste et al., 2016). Furthermore, contradicting results on the effect of H. contortus infection have been reported elsewhere. For instance, Doyle et al. (2014) reported that Merino lambs trickle infected with approximately 9,000 H. contortus L3 in an 8-week experimental period did not show depression in feed intake. Similarly, studies with creole kids infected with 10,000 H. contortus L3 in two 7-week phases (a primary and a secondary infection) (Bambou et al., 2009; Bambou et al., 2013) revealed that a transient feed intake depression was only observed during the 3-week period post the primary infection. In line with the above, a meta-analyses study conducted by Méndez-Ortíz et al. (2019) on the effects of gastrointestinal nematodes on feed intake and LWG by lambs revealed that 41% and 27% of the studies did not observe negative effect of GIN on feed intake and LWG, respectively.
Parasite-induced anorexia is viewed as the result of the interaction of the unavoidable consequence of infection and the coping mechanism of the animal to deal with infection (Kyriazakis, 2014). In the present study, daily feed intake was measured over the entire experimental period. Considering that the housing change of the animals from individual pens to metabolic crates and then to respiration chambers may have had implied some stress response. Feed intake and LWG was calculated for the individual pen period (Days 1–26) as well as for the entire experimental period (Days 1–36). These calculations did show effects of feed restriction on feed intake and LWG (restricted-fed lambs having lower feed intakes and LWG than ad libitum fed lambs). On Day 1-26 the uninfected and infected ad libitum fed lambs had similar LWG. However, when LWG was calculated for the entire experimental period (Days 1-36), infected ad libitum fed lambs lost weight while their uninfected counterparts had a marginal LWG, this is in line with other studies where infected sheep had an increased weight depression in the later stages of the experiment (three to five weeks post infection) compared to the first three weeks post infection (Albers et al., 1989). Pen studies allow for full control of the GIT worm infection, however, this also obscures the normal effect of infection on sheep production as opposed to field conditions where the effects of anemia and the resultant lethargy would exert more pronounced negative effects on feed intake as the sheep search for sufficient feed and water (Albers et al., 1989). This could explain why infected lambs in the present study did not have a significant drop in feed intake and LWG in earlier stages of infection, but the difference became more evident by a drop in LWG in the later weeks of infection.
Various studies (Retama-Flores et al., 2012; Méndez-Ortíz et al., 2019; Ramos-Bruno et al., 2021) have tried to assess the nutritional cost of GIN infection. The most used approach is assessing the N retention, metabolisability of feed energy and LWG of infected and non-infected animals. Ramos-Bruno et al. (2021) have suggested that the nutritional cost of infection would depend on the animal’s ability to withstand the parasitic impact (resilience) or the ability to mount an immune response (resistance). In line with the latter, Blackburn et al. (2013) have suggested that mounting the immune response can be of a higher cost than displaying resilience, and host immune response rather than parasite damage seems the major cause of loss in productivity. Sahoo and Khan (2016) have stated that resistance and animal performance compete each other for nutrients, especially protein.
Infection with H. contortus in the present study not only did not affect apparent nutrient digestibility, but also there was no evidence of nutritional cost judged from the nitrogen retention and metabolisability of the diet by infected and uninfected lambs. However, regarding energy cost, it should be pointed out that the best indicator of it would be ratio of heat production to metabolizable energy; i.e., the higher the ratio, the higher the energy cost of infection. However, heat production was not measured in this study.
The nutritional status of the host animal is an important factor influencing the host-parasite relationship and the pathogenesis of infection (Sahoo and Khan, 2016). Positive energy balance and improved body condition score are important in setting up the immune response (Lord, 2002; Valderrabano et al., 2006). In the same line, improved protein nutrition is important for the reduction in the establishment of infection by improving the immune response as well as improving the resistance and resilience to infection (Houdijk et al., 2006; Louvandini et al., 2006), as well as the repair of the damage in the gastrointestinal tract (Khan et al., 2011; Ramanan et al., 2016) caused by GIT worms. In this study, the diet was poor both in crude protein and metabolizable energy contents and the animals fairly maintained their body condition score around 3 (scale 1 to 5), but the negative impact of infection on animal performance was mild.
Reasons for the lack of effect of parasite infection on feed intake, N retention and diet metabolisability can only be hypothesized to be due to: (1) the level of infection achieved was not severe enough to produce feed intake depression and hence LW loss during the pen period. Further, at Day 36 (when infection peaked) the impact of parasitism on LWG was still marginal (2) both sheep breeds have high resistance to parasite infection (especially Red Maasai), as discussed earlier; i.e., Red Maasai being indigenous to the environment and Dorper having an indigenous genetic component (Blackhead Persian). (3) In the present experiment, the lambs were sourced from a production system based on the utilization of rangelands and therefore had prior exposure to H. contortus. It is therefore plausible that due to the prior exposure, the lambs had acquired a degree of resistance that could explain the lack of pronounced effects of infection on feed intake and hence LWG. (4) both sheep breeds have adaptation to the low feeding value of the diets, allowing them to respond to infection and sustain the immune response against parasite infection (Ramos-Bruno et al., 2021). The mechanisms by which nutritional status of the animal enhances resilience and resistance to parasites are still unknown (Sahoo and Khan, 2016). However, the low N and ME contents of the diet used in the study makes it difficult to sustain the latter hypothesis.
4.2 Effects of GIN infection and breed on daily methane emission and yield
Daily enteric methane emissions from ruminants are closely related to the amount of feed consumed (e.g., Buddle et al., 2011; Hammond et al., 2013; Charmley et al., 2015) and the results of the present study confirm the above relationship, with daily methane emission (g/d) from restricted-fed lambs being lower than those from their ad libitum fed counterparts.
Comparison of emissions across experiments is more relevant when emissions are expressed in terms of CH4 yield (e.g., g/kg DMI, g/kg DOMI, and on energy basis, MJ CH4/MJ GEI). The CH4 yield found in this study (23.3–26.4 g/kg DMI) is within the range found by other studies in tropical environments with tropical low-quality forages (Hunter, 2007; Archimède et al., 2018; Costa et al., 2020; Gere et al., 2022). For example, Archimède et al. (2018), reported CH4 yields in the range of 15–29 g/kg DMI from sheep fed low quality C4 grasses; whereas Hunter (2007) and Gere et al. (2022) reported even a higher CH4 yield (31 g/kg DMI each) from cattle fed on Angleton grass and sheep fed on Rhodes grass hay (a forage similar to that used in the present study), respectively. These values are higher than those reported from studies in temperate regions with temperate forages. For example, numerous studies conducted in New Zealand (Muetzel and Clark, 2015; Swainson et al., 2016) with temperate pasture forages reported a mean CH4 yield of 23.5 g/kg DMI, with no difference between cattle and sheep. Ruminants fed tropical forages usually produce more enteric CH4 (yield) than ruminants fed temperate forages and this fact is related to the more fibrous nature of C4 grasses compared to C3, adaptation of animals to low quality C4 grasses (e.g., longer retention time of lower quality feed) and rumen environment more favorable to acetogenic fermentation and methanogenesis (Beauchemin et al., 2009; Archimède et al., 2011; Kennedy and Charmley, 2012). For example, Rira et al. (2016) reported more abundant total ruminal bacteria and methanogens in animals kept in tropical environments than in temperate environments. Nevertheless, much lower CH4 yields were reported from other studies with sheep (Amaral et al., 2016; Lima et al., 2019; Corrêa et al., 2021; Fernandes et al., 2022). For example, the study of Amaral et al. (2016) reported CH4 yield of 13 g/kg DMI from sheep fed on high quality (N fertilized) C4 annual grasses. In the present study, the lambs were fed on a low-quality tropical grass with high fibre but low crude protein contents. A study using Boran steers fed poor quality tropical forages providing sub-optimal maintenance energy requirements showed that although the methane production was reduced due to reduced feed intake, both CH4 yield and CH4 conversion factor increased as intake fell (Goopy et al., 2020).
One important finding from the present study regarding enteric CH4 emissions was that H. contortus infection did not affect the absolute daily enteric CH4 emissions (g/d) or CH4 yield (g/kg DMI). This is in contrast with findings from other studies (Fox et al., 2018; Lima et al., 2019; Corrêa et al., 2021; Fernandes et al., 2022), which showed not only increased CH4 yields from GIN infected sheep, but also depressed feed intake and LWG. The research by Lima et al. (2019); Corrêa et al. (2021) and Fernandes et al. (2022) were all conducted with parasite naive lambs of a tropical sheep breed (Santa Ines), fed either on Cynodon dactylon hay as a sole diet (Corrêa et al., 2021) or a mixed diet containing 70% (Lima et al., 2019) or 60% (Fernandes et al., 2022) of this forage plus concentrates. Corrêa et al. (2021) reported increase in CH4 yield of 62 and 99%, respectively when the lambs were separately infected with H.contortus and T. colubriformes. In turn, Fernandes et al. (2022) reported a 59% increase in CH4 yield from lambs infected with both H. contortus and T. colubriformes, whereas for a similar mixed infection, Lima et al. (2019) reported an up to 250% increase in CH4 yield. In a similar way, from a research work with parasite naïve lambs infected with T. circumcincta and fed on grass pellets (DM digestibility of 55.4–58.4%), Fox et al. (2018) reported 33% increase in CH4 yield. In the present study, the lack of effect of parasite infection on CH4 yield is in line with the lack of effect on feed intake and LWG.
Infection by GIN in small ruminants alters substantially the gastrointestinal tract and understanding of the effects of infection on microbiome diversity and function, and ultimately on rate of methanogenesis is still in early development (Li et al., 2016; El-Ashram et al., 2017; Correa et al., 2020; Corrêa et al., 2021; Xiang et al., 2021). These studies showed alterations in the ruminal and abomasal microbial functional structure and composition as consequence of GIN infection. Corrêa et al. (2021) hypothesized that the increased CH4 yield from lambs infected with GIN might be related to the increase in methanogenic archaea population in the rumen, as well as to the higher abundance of genes related to methane metabolism and key enzymes involved in methanogenesis (e.g., methyl-coenzyme M and methanopterin). In the present study no measurements of rumen function and microbiome were carried out, except for rumen pH, which was observed not to be affected by infection.
To our knowledge, this is the first study that compared CH4 emissions from the two most common breeds in Sub-Saharan Africa, whereas fed on a low-quality tropical forage, that mimicked the predominant diet quality found in production systems. Under these conditions, Dorper had higher daily methane emission and methane yields than their Red Maasai counterparts. It is difficult to ascertain about the animal, dietary and environmental (e.g., health stress) factors that are likely involved in the observed difference in CH4 emission between these breeds. The only other observed measured difference between these two breeds was the health status (i.e., PCV and FEC) resulting from infection with H. contortus. It is unknown whether rumen microbial population structure and function (e.g., Corrêa et al., 2021) and digesta retention time in rumen (Pinares-Patiño et al., 2003) were altered in Dorper breed in response to infection and hence leading to increased CH4 emission (absolute and CH4 yield). Although, in this study, feed digestibility was not affected by breed or infection, the effect of breed on emissions per unit of digestible feed intake (g/kg DDMI, g/kg DOMI) approached significance, with Dorper breed having higher CH4 yields than Red Maasai. Pinares-Patiño et al. (2013) from extensive measurements of CH4 yield from sheep fed on temperate forages in respiration chambers reported that CH4 yield is heritable and a major factor for differences between low and high CH4 yield animals is the rumen retention time of the particulate fraction of digesta. In the same line, a study by Goopy et al. (2014) comparing high and low methane yield producing sheep reported that sheep that had a low methane yield also had lower mean rumen retention time and lower rumen volume and fill compared to high methane yield sheep despite having similar DMI and DM digestibility. These results could provide a plausible explanation for the differences observed between the Red Maasai and Dorper sheep in the present study, and further investigation would be beneficial in understanding these results. In this respect, a study conducted by Archimède et al. (2018) where a tropical indigenous (Blackbelly) and a highly selected temperate (Texel) sheep breeds were compared at tropical and temperate sites while fed on good (N fertilized) and poor quality of both tropical (C4) and temperate (C3) forages, revealed that CH4 yield (g/kg DMI) was not affected by experimental site, whereas there was a breed × site interaction on CH4 yield. In the tropical site, the CH4 yield was consistently (all forage types and qualities) lower for the tropical breed than for the temperate breed, whereas the opposite was observed in the temperate site. The latter suggests that CH4 emission is the result of complex interactions of factors such as diet, animal genetics and general environment.
4.3 Period effects on the measured variables
In this study PCV at Day 1 (PCV Day 1) and PCV at Day 36 (PCV Day 36) differed with experimental periods (P), being higher in P1 than P2. It is difficult to ascertain the reasons for these observations. The depression in PCV from P1 to P2 may be an artifact of a continuous feeding on a low-quality diet rather than a more severe infection in P2 than in P1. The higher PCV Day 36 observed in uninfected lambs in P2 than in P1 and no change in PCV Day 36 of infected lambs support the above hypothesis.
There were period (P) effects on LW, LWG, digestibilities of dry matter (DMD), crude protein (CPD) and of acid detergent fibre (ADFD), being these values higher in P2 than in P1. The above may indicate that the improved LW and LWG in P2 was probably due to improved digestibilities rather than to improved feed intake. In fact, DMI during the first 26 days (pen period) and over the balance period (Days 29–33) were higher in P1 than in P2. In the same way, intakes of crude protein (CPI), N intake and retention, and intakes of digestible energy (DE) and metabolizable energy (ME) were higher in P1 than in P2. So, determining the causal reasons for improved LWG observed in P2 is not straight forward.
There were Breed×Period interaction effects on intakes of DM (DMI), NDF (NDFI) and ADF (ADFI), being these higher for Dorper in P1 than in P2. Accordingly, Dorper had higher daily CH4 emissions (g/d) in P1 than in P2. However, there were complex Experimental treatment×Breed×Period interaction effects on CH4 yields and Ym, with emissions being higher for Dorper, especially in P2; whereas emissions were the lowest for infected (I-adlib) Red Maasai in P2. Therefore, it can be postulated that the higher CH4 (g/d) emissions observed in Dorper compared to Red Maasai was derived mostly from P1 and this was due to higher DMI and NDFI observed in this period. On the other hand, the higher CH4 yields observed in Dorper, compared to Red Maasai arose principally from higher yields in P2 observed in Dorper. The feed used in this study was sourced from a single crop plot and was relatively uniform in quality.
The above-described interactions cannot be attributed to GIN infection. Firstly, as stated earlier, effects of parasite infection on feed intake, LWG, feed digestibility, nitrogen retention, diet metabolisability or methane emission were not evident. Secondly, the lambs in the infected group (I-adlib) in Period 1 had recovered from anemia and their PCV returned to normal after anthelmintic treatment and washout period. Regarding H. contortus infection, Albers et al. (1989) concluded that sheep recover rapidly from anemia after treatment, but effects of anemia on growth may continue for a few days post treatment, while compensatory growth and refilling of the gastrointestinal will also take place. Ascertaining the reasons for the above-described interactions are beyond the scope of this manuscript and it can only be hypothesized that it was a result from an artefact related to the low quality of feed as well as the contrasting adaptabilities of the sheep breeds.
The feed used in the present study was of a very poor quality (7.2% crude protein and 8.0 MJ ME/kg DM), whereas the lambs were in a physiological stage with high demand for nutrients. The feed was chosen to represent poor nutritional qualities of the feed commonly found in farming systems in SSA. Due to long-term natural selection under environmental stressors at specific environments, indigenous breeds of ruminants can cope with periods of nutritional stress (e.g., shortage of feed and quality deterioration) (De Tarso et al., 2016; Zhou et al., 2019). The latter may explain the inability of Dorper (introduced breed) to sustain consistent intakes of the low-quality diet over the extended experimental period (114 days including the quarantine period), as indicated by the drop in DMI from Period 1 to Period 2, whereas Red Maasai (indigenous) was able to cope.
It is generally accepted that a decrease in feed intake results in an increase in diet digestibility, due to a longer retention time of feed particles in the rumen, leading to a more efficient reduction in feed particles by comminution, hence favoring microbial digestion (Galyean and Owens, 1991). However, the above mechanism seems less marked with forage diets than with concentrate-rich diets (Grimaud and Doreau, 1995). Nevertheless, longer retention times are conducive to enhanced methanogenesis (Pinares-Patiño et al., 2003). The latter seems to be in line with the observed higher CH4 emissions by Dorper lambs than by Red Maasai, but similar feed digestibilities between breeds.
Microbial protein is the main protein source for ruminants fed on diets low in nitrogen, with energy and N availability in the rumen being the most important limiting factors for microbial growth and hence rumen fermentation (Firkins, 1996). It is difficult to ascertain whether the drop in DMI and NDFI from Period 1 to Period 2 observed in Dorper lambs had an influential effect on the observed reduction in CPD and retained N from Period 1 to Period 2 in the ad libitum fed lambs. The rates of endogenous N loss and hindgut microbial protein synthesis could also have been altered by nutritional status (Giráldez et al., 1997), hence leading to decreased apparent CPD and retention of N from Period 1 to 2
Decreased nitrogen excretion by the kidney and recycling of urea to the rumen during undernutrition seems to be the mechanism by which some breeds and species of ruminants maintain digestion of poor-quality forages low in N content (Reynolds and Kristensen, 2008). Whether Red Maasai were better equipped than Dorper to maintain rumen fermentation from Period 1 to Period 2 is unknown.
5 Conclusion and recommendations
This was the first study of its kind in the Sub-Saharan Africa (SSA) addressing the health, feed intake, feed digestibility, liveweight gain and methane emissions impact of Haemonchus (H.) contortus infection, in sheep differing in genetic background (indigenous and exotic). H. contortus infection is a prevailing health issue in SSA, and under the predominant system of production dependent on the utilization of poor-quality rangelands forage it was expected that infection would provide clear negative effects on animal health, feed intake and digestibility, animal performance and hence emission intensity of methane (emissions per unit of product). Despite significant effects of GIN infection on animal health response (PCV and FEC), the expected impacts on feed intake, nutrient metabolism and methane emissions were not evident. Although GIN infection did not show an impact on methane emissions, Dorper sheep had higher emissions (and yields) of methane than their Red Maasai counterparts. Whether this is related to adaptative mechanisms involved in feed conversion efficiency and rumen digestion process needs further studies preferable under free-ranging situations.
In this study animals were infected over a short period (4 days) and the 36-day experimental period was rather short. However, in field conditions sheep would ingest larvae at multiple continuous times. This study also involved a single GIN, which do not represent real life situations. It would therefore be advisable to conduct studies under field conditions and across seasons with natural process of infection over a longer period. This would provide a more reasonable assessment of the effect of worm burden and animal genetics background on animal welfare (resistance and resilience to GIN infection) and productivity.
The two breeds showed differences in animal health response to infection, with the Dorper breed having higher negative health impact than their indigenous counterparts, hence confirming that Red Maasai are more resistant to GIN infection and well adapted to the harsh environmental conditions of SSA.
The GIN burden in small ruminants in SSA remains largely unattended and mainly addressed through anthelmintic drugs. Development of anthelmintic resistance by worms is a growing concern in sheep production systems. Therefore, exploiting the natural resistance and resilience of animals to GIN would be a cost-effective GIN control strategy, especially when the seasonal low-quality of diets is supplemented with nutrients such as nitrogen.
Data availability statement
The raw data supporting the conclusions of this article will be made available by the authors, without undue reservation.
Ethics statement
The animal study was reviewed and approved by ILRI Institutional Animal Care and Use Committee (IACUC, Reference number 2020-20).
Author contributions
PM: Contributed to planning and conducting the study, data acquisition, and statistical analysis, and drafted the manuscript. RE, CP-P, SM contributed to the conception and design of the study, manuscript revision, and interpretation of data. LM contributed to funding acquisition and manuscript revision. IG contributed to planning the study and manuscript revision. JK and DM contributed to the planning and conducting the study. All authors contributed to the article and approved the submitted version.
Funding
The study was funded by the International Development Research Centre (IDRC) - Canada. Project 109211 - Livestock Keeping in a Changing Climate.
Acknowledgments
A special appreciation goes to the Tick Unit, Animal and Human health, ILRI for facilitating faecal egg counts and packed cell volume examination in their facility. We thank CLINGLOBAL South Africa for providing the H. contortus L3 used for infection and training the experimental team on infection and larvae propagation techniques. The authors are Thankful to Jane Poole and Nicholas Ndiwa for their guidance on experimental design and statistical analyses. Erick Kiprotich and Nelson Saya are thanked for assisting with animal handling and care, sample collection and preparation, and respiration chamber management. We also appreciate Dr. Alice Onyango, Rodgers Rogito, Francis Njenga, and Collins Oduor for carrying out chemical analysis of the samples. Daniel Korir is thanked for his support.
Conflict of interest
The authors declare that the research was conducted in the absence of any commercial or financial relationships that could be construed as a potential conflict of interest.
Publisher’s note
All claims expressed in this article are solely those of the authors and do not necessarily represent those of their affiliated organizations, or those of the publisher, the editors and the reviewers. Any product that may be evaluated in this article, or claim that may be made by its manufacturer, is not guaranteed or endorsed by the publisher.
References
Alba-Hurtado F., Muñoz-Guzmán M. A. (2013). Immune responses associated with resistance to haemonchosis in sheep. BioMed. Res. Int. 2013, 162158. doi: 10.1155/2013/162158
Albers G. A. A., Gray G. D., Le Jambre L. F., Piper L. R., Barger I. A., Barker J. S. F. (1989). The effect of Haemonchus contortus on liveweight gain and wool growth in young merino sheep. Aust. J. Agric. Res. 40 (2), 419–432. doi: 10.1071/AR9890419
Albuquerque A. C. A., Bassetto C. C., Almeida F. A., Hildersley K. A., McNeilly T. N., Britton C., et al. (2019). Differences in immune responses to Haemonchus contortus infection in the susceptible Ile de France and the resistant Santa Ines sheep under different anthelmintic treatments regimens. Veterinary Res. 50 (1), 1–12. doi: 10.1186/s13567-019-0722-3
Amaral G. A., David D. B., Gere J. I., Savian J. V., Kohmann M. M., Nadin L. B., et al. (2016). Methane emissions from sheep grazing pearl millet (Penissetum americanum (L.) Leeke) swards fertilized with increasing nitrogen levels. Small Ruminant Res. 141, 118–123. doi: 10.1016/j.smallrumres.2016.07.011
Archimède H., Eugène M., Magdeleine C. M., Boval M., Martin C., Morgavi D. P., et al. (2011). Comparison of methane production between C3 and C4 grasses and legumes. Anim. Feed Sci. Technol. 166, 59–64. doi: 10.1016/j.anifeedsci.2011.04.003
Archimède H., Rira M., Eugène M., Fleury J., Lastel M.-L., Periacarpin F., et al. (2018). Intake, total-tract digestibility and methane emissions of Texel and Blackbelly sheep fed C4 and C3 grasses tested simultaneously in a temperate and a tropical area. J. Cleaner Production 185, 455–463. doi: 10.1016/j.jclepro.2018.03.059
Baker R. L., Mugambi J. M., Audho J. O., Carles A. B., Thorpe W. (2004). Genotype by environment interactions for productivity and resistance to gastro-intestinal nematode parasites in Red Maasai and Dorper sheep. Anim. Sci. 79 (3), 343–353. doi: 10.1017/S1357729800090214
Baker R. L., Nagda S., Rodriguez-Zas S. L., Southey B. R., Audho J. O., Aduda E. O., et al. (2003). Resistance and resilience to gastro-intestinal nematode parasites and relationships with productivity of Red Maasai, Dorper and Red Maasai✕ Dorper crossbred lambs in the sub-humid tropics. Anim. Sci. 76 (1), 119–136. doi: 10.1017/S1357729800053388
Bambou J.-C., Arquet R., Archimède H., Alexandre G., Mandonnet N., González-García E. (2009). Intake and digestibility of naive kids differing in genetic resistance and experimentally parasitized (indoors) with Haemonchus contortus in two successive challenges. J. Anim. Sci. 87 (7), 2367–2375. doi: 10.2527/jas.2008-1702
Bambou J.-C., Cei W., Camous S., Archimède H., Decherf A., Philibert L., et al. (2013). Effects of single or trickle Haemonchus contortus experimental infection on digestibility and host responses of naïve Creole kids reared indoor. Veterinary Parasitol. 191 (3–4), 284–292. doi: 10.1016/j.vetpar.2012.09.026
Beauchemin K. A., McAllister T. A., McGinn S. M. (2009). Dietary mitigation of enteric methane from cattle. CABI Rev. 4, 1–18. doi: 10.1079/PAVSNNR20094035
Besier R. B., Kahn L. P., Sargison N. D., Van Wyk J. A. (2016). Diagnosis, treatment and management of Haemonchus contortus in small ruminants. Adv. Parasitol. 93, 181–238. doi: 10.1016/bs.apar.2016.02.024
Blackburn P. J., Carmichael I. H., Walkden-Brown S. W., Greenslade S. (2013). “Cost of immune response to Trichostrongylus vitrinus infection in meat sheep,” in Proceedings of the Australian Sheep Veterinarians 2013 Conference, Albany. (Sydney, Australia: Sheep Veterinary Association) 41–46.
Buddle B. M., Denis M., Attwood G. T., Altermann E., Janssen P. H., Ronimus R. S., et al. (2011). Strategies to reduce methane emissions from farmed ruminants grazing on pasture. Veterinary J. 188 (1), 11–17. doi: 10.1016/j.tvjl.2010.02.019
Charmley E., Williams S. R. O., Moate P. J., Hegarty R. S., Herd R. M., Oddy V. H., et al. (2015). A universal equation to predict methane production of forage-fed cattle in Australia. Anim. Production Sci. 56 (3), 169–180. doi: 10.1071/AN15365
Coop R. L., Sykes A. R., Angus K. W. (1982). The effect of three levels of intake of Ostertagia circumcincta larvae on growth rate, food intake and body composition of growing lambs. J. Agric. Sci. 98 (2), 247–255. doi: 10.1017/S0021859600041782
Correa P. S., Mendes L. W., Lemos L. N., Crouzoulon P., Niderkorn V., Hoste H., et al. (2020). Tannin supplementation modulates the composition and function of ruminal microbiome in lambs infected with gastrointestinal nematodes. FEMS Microbiol. Ecol. 96 (3), fiaa024. doi: 10.1093/femsec/fiaa024
Corrêa P. S., Mendes L. W., Lemos L. N., Sampaio A. C. K., Issakowicz J., McManus C. M., et al. (2021). The effect of Haemonchus contortus and Trichostrongylus colubriforms infection on the ruminal microbiome of lambs. Exp. Parasitol. 231, 108175. doi: 10.1016/j.exppara.2021.108175
Costa H., Saliba E., Bomfim M., Lana Â.M., Borges A. L., Landim A., et al. (2020). Sheep methane emission on semiarid native pasture—Potential impacts of either zinc sulfate or propylene glycol as mitigation strategies. Animals 10 (3), 395. doi: 10.3390/ani10030395
De Tarso S., Oliveira D., Afonso J. A. B. (2016). Ruminants as part of the global food system: how evolutionary adaptations and diversity of the digestive system brought them to the future. J. Dairy Vet. Anim. Res. 3 (5), 94. doi: 10.15406/jdvar.2016.03.00094
Doyle E. K., Kahn L. P., McClure S. J. (2014). Nutrient partitioning of Merino sheep divergently selected for genetic difference in resistance to Haemonchus contortus. Veterinary Parasitol. 205 (1–2), 175–185. doi: 10.1016/j.vetpar.2014.06.028
Doyle E. K., Kahn L. P., McClure S. J., Lea J. M. (2011). Voluntary feed intake and diet selection of Merino sheep divergently selected for genetic difference in resistance to Haemonchus contortus. Veterinary Parasitol. 177 (3–4), 316–323. doi: 10.1016/j.vetpar.2011.01.043
Eckard R. J., Grainger C., De Klein C. A. M. (2010). Options for the abatement of methane and nitrous oxide from ruminant production: A review. Livestock Sci. 130 (1–3), 47–56. doi: 10.1016/j.livsci.2010.02.010
El-Ashram S., Al Nasr I., Abouhajer F., El-Kemary M., Huang G., Dinçel G., et al. (2017). Microbial community and ovine host response varies with early and late stages of Haemonchus contortus infection. Veterinary Res. Commun. 41 (4), 263–277. doi: 10.1007/s11259-017-9698-5
Fernandes M. A., Lima P., de M. T., do Amarante A. F. T., Abdalla A. L., Louvandini H. (2022). Hematological, biochemical alterations and methane production in sheep submitted to mixed infection of Haemonchus contortus and Trichostrongylus colubriformis. Small Ruminant Res. 216, 106798. doi: 10.1016/j.smallrumres.2022.106798
Firkins J. L. (1996). Maximizing microbial protein synthesis in the rumen. J. Nutr. 126 (suppl_4), 1347S–1354S. doi: 10.1093/jn/126.suppl_4.1347S
Fox N. J., Smith L. A., Houdijk J. G. M., Athanasiadou S., Hutchings M. R. (2018). Ubiquitous parasites drive a 33% increase in methane yield from livestock. Int. J. Parasitol. 48 (13), 1017–1021. doi: 10.1016/j.ijpara.2018.06.001
Galyean M. L., Owens F. N. (1991). “Effects of diet composition and level of feed intake on site and extent of digestion in ruminants,” in Physiological aspects of digestion and metabolism in ruminants (Elsevier), 483–514.
Gerber P. J., Steinfeld H., Henderson B., Mottet A., Opio C., Dijkman J., et al. (2013). Tackling climate change through livestock: a global assessment of emissions and mitigation opportunities (Food and Agriculture Organization of the United Nations (FAO).
Gere J. I., Feksa Frasson M., Wawrzkiewicz M., Fernández Pepi M. G., Ramos M. L., Bualó R., et al. (2022). Enteric methane emission from Sheep fed with rhodes grass hay (Chloris gayana) alone or supplemented with dried distillers’ grains with solubles. Methane 1 (3), 210–217. doi: 10.3390/methane1030017
Giráldez F. J., Peláez R., Frutos P., Mantecón A. R. (1997). The influence of digestible organic matter and nitrogen intake on faecal and urinary nitrogen losses in sheep. Livestock Production Sci. 51 (1–3), 183–190. doi: 10.1016/S0301-6226(97)00066-3
Goopy J. P., Donaldson A., Hegarty R., Vercoe P. E., Haynes F., Barnett M., et al. (2014). Low-methane yield sheep have smaller rumens and shorter rumen retention time. Br. J. Nutr. 111 (4), 578–585. doi: 10.1017/S0007114513002936
Goopy J. P., Korir D., Pelster D., Ali A. I. M., Wassie S. E., Schlecht E., et al. (2020). Severe below-maintenance feed intake increases methane yield from enteric fermentation in cattle. Br. J. Nutr., 1239–1246. doi: 10.1017/S0007114519003350
Grimaud P., Doreau M. (1995). Effect of extended underfeeding on digestion and nitrogen balance in nonlactating cows. J. Anim. Sci. 73 (1), 211–219. doi: 10.2527/1995.731211x
Hammond K. J., Burke J. L., Koolaard J. P., Muetzel S., Pinares-Patiño C. S., Waghorn G. C. (2013). Effects of feed intake on enteric methane emissions from sheep fed fresh white clover (Trifolium repens) and perennial ryegrass (Lolium perenne) forages. Anim. Feed Sci. Technol. 179 (1–4), 121–132. doi: 10.1016/j.anifeedsci.2012.11.004
Hoste H., Torres-Acosta J. F. J., Quijada J., Chan-Perez I., Dakheel M. M., Kommuru D. S., et al. (2016). Interactions between nutrition and infections with Haemonchus contortus and related gastrointestinal nematodes in small ruminants. Adv. Parasitol. 93, 239–351. doi: 10.1016/bs.apar.2016.02.025
Houdijk J. G. M., Jackson F., Coop R. L., Kyriazakis I. (2006). Rapid improvement of immunity to Teladorsagia circumcincta is achieved through a reduction in the demand for protein in lactating ewes. Int. J. Parasitol. 36 (2), 219–227. doi: 10.1016/j.ijpara.2005.09.014
Houdijk J. G. M., Tolkamp B. J., Rooke J. A., Hutchings M. R. (2017). Animal health and greenhouse gas intensity: The paradox of periparturient parasitism. Int. J. Parasitol. 47 (10–11), 633–641. doi: 10.1016/j.ijpara.2017.03.006
Hunter R. A. (2007). Methane production by cattle in the tropics. Br. J. Nutr. 98 (3), 657. doi: 10.1017/S0007114507727460
Kennedy P. M., Charmley E. (2012). Methane yields from Brahman cattle fed tropical grasses and legumes. Anim. Production Sci. 52 (4), 225–239. doi: 10.1071/AN11103
Khan F. A., Sahoo A., Dhakad S., Pareek A. K., Karim S. A. (2011). Effect of trickle infection with Haemonchus contortus on pathophysiology and metabolic responses of growing lambs. Indian J. Anim. Sci. 81 (10), 1005.
Kyriazakis I. (2014). Pathogen-induced anorexia: a herbivore strategy or an unavoidable consequence of infection? Anim. Production Sci. 54 (9), 1190–1197. doi: 10.1071/AN14431
Kyriazakis I., Anderson D. H., Oldham J. D., Coop R. L., Jackson F. (1996). Long-term subclinical infection with Trichostrongylus colubriformis: effects on food intake, diet selection and performance of growing lambs. Veterinary Parasitol. 61 (3–4), 297–313. doi: 10.1016/0304-4017(95)00824-1
Li R. W., Li W., Sun J., Yu P., Baldwin R. L., Urban J. F. (2016). The effect of helminth infection on the microbial composition and structure of the caprine abomasal microbiome. Sci. Rep. 6 (1), 1–10. doi: 10.1038/srep20606
Lima P., de M. T., Crouzoulon P., Sanches T. P., Zabré G., Kabore A., et al. (2019). Effects of Acacia mearnsii supplementation on nutrition, parasitological, blood parameters and methane emissions in Santa Inês sheep infected with Trichostrongylus colubriformis and Haemonchus contortus. Exp. Parasitol. 207, 107777. doi: 10.1016/j.exppara.2019.107777
Lord G. (2002). Role of leptin in immunology. Nutr. Rev. 60 (suppl_10), S35–S38. doi: 10.1301/002966402320634913
Louvandini H., Veloso C. F. M., Paludo G. R., Dell’Porto A., Gennari S. M., McManus C. M. (2006). Influence of protein supplementation on the resistance and resilience on young hair sheep naturally infected with gastrointestinal nematodes during rainy and dry seasons. Veterinary Parasitol. 137 (1–2), 103–111. doi: 10.1016/j.vetpar.2006.01.004
Marino R., Atzori A. S., D’Andrea M., Iovane G., Trabalza-Marinucci M., Rinaldi L. (2016). Climate change: Production performance, health issues, greenhouse gas emissions and mitigation strategies in sheep and goat farming. Small Ruminant Res. 135, 50–59. doi: 10.1016/j.smallrumres.2015.12.012
Méndez-Ortíz F. A., Sandoval-Castro C. A., Vargas-Magaña J. J., Sarmiento-Franco L., Torres-Acosta J. F. J., Ventura-Cordero J. (2019). Impact of gastrointestinal parasitism on dry matter intake and live weight gain of lambs: A meta-analysis to estimate the metabolic cost of gastrointestinal nematodes. Veterinary Parasitol. 265, 1–6. doi: 10.1016/j.vetpar.2018.11.008
Moraes L. E., Strathe A. B., Fadel J. G., Casper D. P., Kebreab E. (2014). Prediction of enteric methane emissions from cattle. Global Change Biol. 20 (7), 2140–2148. doi: 10.1111/gcb.12471
Muetzel S., Clark H. (2015). Methane emissions from sheep fed fresh pasture. New Z. J. Agric. Res. 58 (4), 472–489. doi: 10.1080/00288233.2015.1090460
Mugambi J. M., Bain R. K., Wanyangu S. W., Ihiga M. A., Duncan J. L., Murray M., et al. (1997). Resistance of four sheep breeds to natural and subsequent artificial Haemonchus contortus infection. Veterinary Parasitol. 69 (3–4), 265–273. doi: 10.1016/S0304-4017(96)01128-4
Mugambi J. M., Wanyangu S. W., Bain R. K., Owango M. O., Duncan J. L., Stear M. J. (1996). Response of Dorper and Red Maasai lambs to trickle Haemonchus contortus infections. Res. Veterinary Sci. 61 (3), 218–221. doi: 10.1016/S0034-5288(96)90066-1
Pinares-Patiño C. S., Hickey S. M., Young E. A., Dodds K. G., MacLean S., Molano G., et al. (2013). Heritability estimates of methane emissions from sheep. Animal 7, 316–321. doi: 10.1017/S1751731113000864
Pinares-Patiño C. S., Ulyatt M. J., Lassey K. R., Barry T. N., Holmes C. W. (2003). Rumen function and digestion parameters associated with differences between sheep in methane emissions when fed chaffed lucerne hay. J. Agric. Sci. 140 (2), 205–214. doi: 10.1017/S0021859603003046
Ramanan D., Bowcutt R., Lee S. C., Tang M. S., Kurtz Z. D., Ding Y., et al. (2016). Helminth infection promotes colonization resistance via type 2 immunity. Science 352 (6285), 608–612. doi: 10.1126/science.aaf3229
Ramos-Bruno E., Sandoval-Castro C. A., Torres-Acosta J. F. J., Sarmiento-Franco L. A., Torres-Fajardo R., Chan-Pérez J. I., et al. (2021). Nitrogen retention in hair sheep lambs with a gradient of Haemonchus contortus infection. Veterinary Parasitol. 296, 109488. doi: 10.1016/j.vetpar.2021.109488
Retama-Flores C., Torres-Acosta J. F. J., Sandoval-Castro C. A., Aguilar-Caballero A. J., Cámara-Sarmiento R., Canul-Ku H. L. (2012). Maize supplementation of Pelibuey sheep in a silvopastoral system: fodder selection, nutrient intake and resilience against gastrointestinal nematodes. Animal 6 (1), 145–153. doi: 10.1017/S1751731111001339
Reynolds C. K., Kristensen N. B. (2008). Nitrogen recycling through the gut and the nitrogen economy of ruminants: an asynchronous symbiosis. J. Anim. Sci. 86 (suppl_14), E293–E305. doi: 10.2527/jas.2007-0475
Rira M., Morgavi D. P., Popova M., Marie-Magdeleine C., Silou-Etienne T., Archimède H., et al. (2016). Ruminal methanogens and bacteria populations in sheep are modified by a tropical environment. Anim. Feed Sci. Technol. 220, 226–236. doi: 10.1016/j.anifeedsci.2016.08.010
Sahoo A., Khan F. (2016). Nutritional and biological control synergism against gastrointestinal nematodes in small ruminants. J. Vet. Sci. Anim. Husb 4 (1), 104. doi: 10.15744/2348-9790.4.104
Swainson N., Muetzel S., Clark H. (2016). Updated predictions of enteric methane emissions from sheep suitable for use in the New Zealand national greenhouse gas inventory. Anim. Production Sci. 58 (6), 973–979. doi: 10.1071/AN15766
Sykes A. R., Coop R. L. (1977). Intake and utilization of food by growing sheep with abomasal damage caused by daily dosing with Ostertagia circumcincta larvae. J. Agric. Sci. 88 (3), 671–677. doi: 10.1017/S0021859600037369
Valderrabano J., Gomez-Rincon C., Uriarte J. (2006). Effect of nutritional status and fat reserves on the periparturient immune response to Haemonchus contortus infection in sheep. Veterinary Parasitol. 141 (1–2), 122–131. doi: 10.1016/j.vetpar.2006.04.029
Van Soest P. J., Robertson J. B., Lewis B. A. (1991). Methods for dietary fiber, neutral detergent fiber, and nonstarch polysaccharides in relation to animal nutrition. J. Dairy Sci. 74 (10), 3583–3597. doi: 10.3168/jds.S0022-0302(91)78551-2
Wanyangu S. W., Mugambi J. M., Bain R. K., Duncan J. L., Murray M., Stear M. J. (1997). Response to artificial and subsequent natural infection with Haemonchus contortus in Red Maasai and Dorper ewes. Veterinary Parasitol. 69 (3–4), 275–282. doi: 10.1016/S0304-4017(96)01129-6
Whitlock H. V. (1948). Haemonchus contortus infection alters gastrointestinal Some modifications of the McMaster helminth egg-counting technique and apparatus. J. Council Sci. Industr. Res. 21(3), 177–180.
Xiang H., Fang Y., Tan Z., Zhong R. (2021). Haemonchus contortus infection alters gastrointestinal microbial community composition, protein digestion and amino acid allocations in lambs. Front. Microbiol. 12. doi: 10.3389/fmicb.2021.797746
Zhao J., Ma X., Jin Y., Su R., Liu W., Ren Y., et al. (2016). Energy requirements for the maintenance and growth of Dorper-Jinzhong crossbred ram lambs. Ital. J. Anim. Sci. 15 (1), 94–102. doi: 10.1080/1828051X.2016.1147336
Keywords: gastrointestinal nematode, climate change mitigation, Rhodes grass, sheep, Haemonchus contortus, methane
Citation: Mwangi PM, Eckard R, Gluecks I, Merbold L, Mulat DG, Gakige J, Pinares-Patino CS and Marquardt S (2023) Impact of Haemonchus contortus infection on feed intake, digestion, liveweight gain, and enteric methane emission from Red Maasai and Dorper sheep. Front. Anim. Sci. 4:1212194. doi: 10.3389/fanim.2023.1212194
Received: 25 April 2023; Accepted: 06 July 2023;
Published: 27 July 2023.
Edited by:
Long Cheng, The University of Melbourne, AustraliaReviewed by:
Sreemol Nair, The University of Melbourne, AustraliaXuezhao Sun, AgResearch Ltd, New Zealand
Copyright © 2023 Mwangi, Eckard, Gluecks, Merbold, Mulat, Gakige, Pinares-Patino and Marquardt. This is an open-access article distributed under the terms of the Creative Commons Attribution License (CC BY). The use, distribution or reproduction in other forums is permitted, provided the original author(s) and the copyright owner(s) are credited and that the original publication in this journal is cited, in accordance with accepted academic practice. No use, distribution or reproduction is permitted which does not comply with these terms.
*Correspondence: Cesar S. Pinares-Patino, Yy5wYXRpbm9AY2dpYXIub3Jn
†These authors have contributed equally to this work and share last authorship