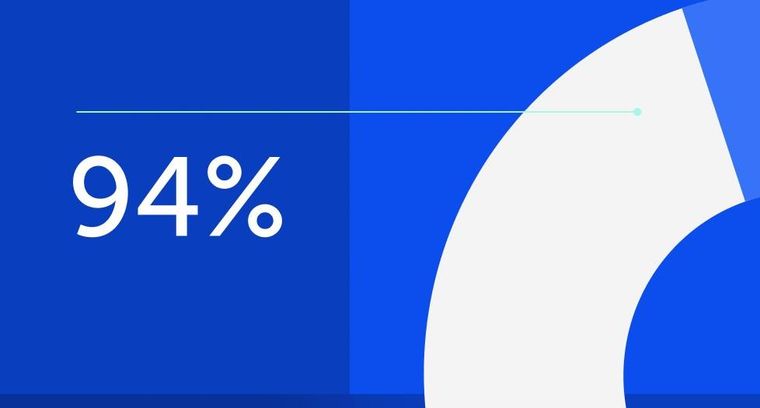
94% of researchers rate our articles as excellent or good
Learn more about the work of our research integrity team to safeguard the quality of each article we publish.
Find out more
ORIGINAL RESEARCH article
Front. Anim. Sci., 17 March 2023
Sec. Precision Livestock Farming
Volume 4 - 2023 | https://doi.org/10.3389/fanim.2023.1093857
Technologies for automating animal management and monitoring tasks can improve efficiency and productivity of livestock production. We developed the e-Synch system for automated control and monitoring the estrous cycle of cattle through intravaginal hormone delivery and sensing. Thus, our objective was to evaluate luteinizing hormone (LH) concentrations after intravaginal instillation of the Gonadotropin-releasing hormone (GnRH) analogue Gonadorelin with the e-Synch system. This system consists of an intravaginal electronically controlled automated hormone delivery and sensing device integrated with an IoT platform. Lactating Holstein cows with their estrous cycle synchronized were used in two experiments (Exp). In Exp 1, at 48 h after induction of luteolysis, cows (n=5-6 per group) were randomized to receive 100 µg of Gonadorelin through intramuscular (i.m.) injection, 100 µg of Gonadorelin in a 2 mL solution delivered with e-Synch, and an empty e-Synch device. In Exp 2, at 48 h after induction of luteolysis cows (n=6-7 per group) were randomized to receive 100 µg of Gonadorelin i.m., or an intravaginal treatment with e-Synch consisting of 100 or 1,000 µg of Gonadorelin in 2 or 10 mL of solution containing 10% citric acid as absorption enhancer. Circulating concentrations of LH were analyzed with linear mixed models with or without repeated measurements. In Exp 1, cows in the i.m. Gonadorelin treatment had a surge of LH whereas cows in the other two treatments did not have a surge of LH for up to 8 h after treatment. In Exp 2, the 1,000 µg dose of Gonadorelin elicited more LH release than the 100 µg dose, regardless of solution quantity. The overall LH response as determined by area under the curve, mean, and maximum LH concentrations was similar between cows receiving 1,000 µg of Gonadorelin delivered with e-Synch and 100 μg of Gonadorelin i.m. Increasing volume of solution for delivering the same dose of Gonadorelin partially increased LH release only for the 100 µg dose. We conclude that the e-Synch system could be used to automatically release Gonadorelin in a dose and volume that induces a surge of LH of similar magnitude than after i.m. injection of 100 μg of Gonadorelin. Also, the dose of Gonadorelin delivered by e-Synch is more critical than the volume of solution used.
Technologies for automating animal management and monitoring tasks can improve efficiency, productivity, and sustainability of livestock production systems (Berckmans, 2017; Aquilani et al., 2022). Moreover, automated devices improve animal well-being by reducing disruptions to daily routines and the number of interventions received by animals for maintaining productivity, remaining healthy, and achieving reproductive success (Berckmans, 2017; Halachmi et al., 2019; Rial et al., 2022). To this end, we developed the e-Synch system for enabling automated control and monitoring the estrous cycle of cattle through intravaginal (IVG) hormone delivery and sensing. The e-Synch was developed to address issues associated with giving dairy and beef cows multiple hormonal injections over days or weeks for synchronization of ovulation for timed artificial insemination (TAI) or embryo transfer. The full system, including an electronically controlled hormone delivery and sensing device integrated with an Internet of Things (IoT) platform, is thoroughly described in a companion manuscript (Ren et al., 2023).
Although several reproductive hormones can be used to control the estrous cycle of cattle, the combination of Gonadotropin releasing hormone (GnRH) and Prostaglandin F2α (PGF) analogues in Ovsynch and Ovsynch-like protocols is the most widely used in many parts of the world (De Rensis and Peters, 1999; Wiltbank and Pursley, 2014). Therefore, an important step towards automated synchronization of ovulation with e-Synch is demonstrating efficacy of GnRH and PGF analogues to exert their biological responses after IVG administration. In a previous experiment, we provided proof-of-concept that delivering PGF with e-Synch caused luteal regression and a similar progesterone (P4) concentration profile than after i.m. injection (Masello et al., 2020). Conversely, the biological response of cows to vaginal administration of GnRH analogues with e-Synch has not been evaluated. Furthermore, the dose and volume of GnRH analogue solution needed for IVG administration to elicit the same biological response as i.m. injection of GnRH analogues are unknown.
After i.m. injection of a pharmacological dose, GnRH analogues induce ovulation through the release of large amounts of luteinizing hormone (LH) from the pituitary gland in a surge-like pattern. Maximum (i.e., peak) circulating concentrations of LH are observed within 1 to 3 h after GnRH analogue administration with a return to baseline within 6 to 8 h (Colazo et al., 2009; Giordano et al., 2012a; Armengol-Gelonch et al., 2017). Previously, we evaluated the feasibility of inducing a surge of LH in dairy cattle after IVG administration of GnRH analogues in the cranial portion of the vagina through a catheter (Wijma et al., 2017). We demonstrated that 1,000 μg of the GnRH analogue Gonadorelin delivered in 10 mL of solution was needed to cause an LH surge of similar magnitude than after a 100 μg i.m. injection. Moreover, GnRH analogues had to be mixed with citric acid (CA) as an absorption enhancer to elicit an LH response. The need to administer 1,000 μg rather than 100 μg of Gonadorelin, which is the approved dose for use in cattle in the US, would make synchronization of ovulation protocols more expensive. Moreover, using 10 mL of solution for each treatment would limit the number of doses that could be delivered by e-Synch in a round of synchronization of ovulation. Thus, it is necessary to identify the minimal dose and volume of GnRH analogues solution delivered with e-Synch that causes a surge of LH of sufficient magnitude to induce ovulation in cattle.
To this end, two experiments were conducted to evaluate circulating concentrations of LH after IVG instillation of solutions containing Gonadorelin and CA as an absorption enhancer via e-Synch. For experiment 1 (Exp 1), we hypothesized that the same LH response would be observed in cows that received 100 μg of Gonadorelin with e-Synch or i.m. injection. Therefore, the objective of Exp 1 was to compare circulating concentrations of LH after administration of 100 μg of Gonadorelin through instillation via e-Synch and i.m. injection. A negative control treatment including a placebo e-Synch device was also included to evaluate if the presence of e-Synch in the vaginal cavity could cause LH release. For experiment 2 (Exp 2), we hypothesized that a 1,000 μg dose of Gonadorelin would result in more LH released than a 100 μg dose, and regardless of dose, larger volume of administration would also result in more LH release. Ultimately, we expected a similar response after the larger dose of Gonadorelin in the larger volume of solution compared with the 100 μg i.m. injection. Therefore, the objective of Exp 2 was to compare circulating concentrations of LH in cows that received different doses of Gonadorelin in different volumes of solution via e-Synch, and in cows that received Gonadorelin by i.m. injection.
All procedures performed with cows were approved by the Animal Care and Use Committee of Cornell University (Ithaca, NY, USA) under protocols 2016-0093 and 2021-0010.
Non-pregnant lactating multiparous Holstein cows from the Cornell University Ruminant Center (Harford, NY, USA) were enrolled in two experiments. Experiment 1 (Exp 1) was conducted from June to July of 2020 and experiment 2 (Exp 2) from January to March of 2022. Cows were housed in freestall barns until the day before treatment. The freestall barn was equipped with deep-bedded sand stalls, sprinklers and headlocks in the feedline and fans above stalls and feedline. Cows were moved to a tie-stall barn from the day before to the day after application of treatments. The tie-stall barn was tunnel ventilated with individual stalls covered with rubber mattresses and sawdust. Each stall had an individual feed bin and waterer which provided ad libitum access to water and a total mixed ration diet formulated to meet or exceed requirements for milk production and stage of lactation. All cows were milked three times a day at approximately 8 h intervals.
At 32 to 41 days in milk (DIM), cows without apparent clinical health disorders or abnormalities of the reproductive tract (n = 20) were enrolled in a Double-Ovsynch (DO) protocol (Souza et al., 2008; Wiltbank et al., 2015) to receive up to the last PGF treatment of the protocol (Pre-Ovsynch: GnRH, 7 d later PGF, 3 d later GnRH, 7 d later Breeding-Ovsynch: GnRH, 7 d later PGF, 12 h later PGF; Figure 1). The GnRH analogue treatments of the protocol consisted of 100 µg i.m. of Gonadorelin diacetate tetrahydrate (Cystorelin, Merial Ltd., Duluth, GA, USA) and all PGF analogue treatments were 25 mg i.m. of Dinoprost tromethamine (Lutalyse HighCon, Zoetis, Parsippany, NJ, USA). The estrous cycle was synchronized to replicate the hormonal environment observed at the time of induction of ovulation with GnRH and before TAI, which is characterized by low circulating concentrations of P4 and elevated circulating concentrations of estradiol (Giordano et al., 2012a; Motta et al., 2020). Before treatment day (d 0 of the experiment), three cows were removed from the experiment because of absence of a CL and follicle >10 mm at induction of luteolysis (n = 1) or the researchers considered that the tail was not sound to undergo the intensive sampling period (n = 2) after treatment. At 48 h after induction of luteolysis with the first PGF treatment of the Breeding-Ovsynch portion of the protocol, cows were randomly assigned to a positive control group (GnRH-IM; n = 6), in which cows received 100 µg of GnRH through i.m. injection, a negative control group (Placebo-eS; n = 5), in which cows received an empty e-Synch device as a placebo, and an e-Synch GnRH group (GnRH-eS; n = 6), in which cows received an e-Synch device loaded with 2 mL of solution with 100 µg of Gonadorelin (GnRH) and 10% CA.
Figure 1 Graphical depiction of experimental procedures in experiment 1 and 2. In both experiments lactating Holstein cows were enrolled in a Double-Ovsynch protocol (Pre-Ovsynch: GnRH, 7 (experiment 1) or 8 (experiment 2) d later PGF2α, 3 d later GnRH, 7 d later Breeding-Ovsynch: GnRH, 7 d later PGF2α, 12 h later PGF2α) to receive up to the last PGF treatment of the protocol. At 48 h after induction of luteolysis with the first PGF treatment of the Breeding-Ovsynch portion of the protocol, cows received the experimental treatments (described in the text). In both experiments, blood was collected and transrectal ultrasonography of the ovaries conducted on d -9, -2, 0, and 7, relative to treatment administration, for estimation of circulating concentrations of progesterone. In experiment 2, ovaries were also evaluated by ultrasonography at 48 h after treatments. On the day of treatment, blood was collected at 0, 1, 2, 2.5, 3, 4, 6 and 8 h (i.e., 0 to 8 h) after intramuscular (i.m.) injections or e-Synch device release start to estimate circulating concentrations of luteinizing hormone. At 2 d before, on the day of treatment but after e-Synch device removal, and 7 d after treatment a vaginal integrity and mucus score were recorded through vaginoscopy utilizing a speculum and a source of light. BC, blood collection; TUS, transrectal ultrasonography; VAG, vaginal integrity and mucus score. †TUS was conducted at 48 h after treatment in experiment 2 only.
At 35 to 50 DIM, cows without apparent clinical health disorders or abnormalities of the reproductive tract (n = 37) were enrolled in the same synchronization protocol used in Exp 1, except that for logistical reasons, cows received the first PGF of the DO protocol 8 d instead of 7 d after the initial GnRH treatment. Three cows were removed from the experiment before application of treatments due to sickness (n = 2) and sale (n = 1). On d 0 of the experiment, which was at 48 h after induction of luteolysis with the first PGF treatment of the Breeding-Ovsynch portion of the protocol, cows were randomly assigned to a positive control group (GnRH-IM; n = 7), in which cows received 100 µg of GnRH through i.m. injection, or one of four treatments administered intravaginally via an e-Synch device. The treatments were 100 µg of Gonadorelin diluted in 2 mL of solution (LoD-LoV; n = 6), 100 µg of Gonadorelin in 10 mL of solution (LoD-HiV; n = 7), 1,000 µg of Gonadorelin in 2 mL of solution (HiD-LoV; n = 7), and 1,000 µg of Gonadorelin in 10 mL of solution (HiD-HiV; n = 7). All solutions for IVG instillation contained 10% CA.
Gonadotropin releasing hormone solutions delivered via e-Sych were made by adding 10% CA (Sigma C0759, Sigma-Aldrich, St. Louis, MO, USA) to a commercially available product containing 50 μg of Gonadorelin diacetate tetrahydrate per mL (Cystorelin, Merial Ltd., Duluth, GA, USA) and adjusting the pH to 4.0 by adding NaOH solution. Cystorelin also contains 9 mg of benzyl alcohol and 7.47 mg of sodium chloride per mL. To adjust for the dilution of Gonadorelin by addition of CA and NaOH solutions, devices were filled with an amount of solution equivalent to 100 µg of Gonadorelin (i.e., 2.1 to 2.3 mL).
All treatment solutions, including the GnRH-IM treatment were made utilizing the same Gonadorelin acetate (Cayman Chemical Company, Ann Arbor, MI, USA). The solution for the GnRH-IM treatment was made using 10 mL of 0.9% sterile saline solution (Vet One saline solution, Vet One, Boise, ID, USA) as the base. From this amount of saline, 86 µg were replaced by 86 µg of benzyl alcohol to approximately replicate the concentration of benzyl alcohol in the commercially available product used for i.m. injection (i.e., Cystorelin). Thereafter, 300 µg of Gonadorelin acetate were mixed with 6 mL of the saline and benzyl alcohol solution to reach a concentration of 50 µg/mL of Gonadorelin in solution. The pH was adjusted to 6.7 by adding 2N NaOH if needed.
Solutions for IVG delivery with e-Synch were prepared by adding Gonadorelin acetate powder to sterile saline solution containing 10% CA to reach a concentration of 500 µg/mL. This stock solution contained the concentration of Gonadorelin required for delivery of 1,000 µg of Gonadorelin in 2 mL (i.e., HiD-LoV treatment) and therefore was used to make the less concentrated solutions for the other treatments. For the latter, stock solution was diluted with saline solution containing 10% CA until the desired amount of gonadorelin acetate and volume was achieved.
In both experiments, e-Synch devices, as described in the companion manuscript (Ren et al., 2023) and originally described in Masello et al. (2020), were used for IVG instillation of Gonadorelin solution at the time of treatment. In Exp 1, a total of 4 functional and 4 non-functional devices (Dev) were assembled and used to complete the experiment (number of times used for functional and non-functional devices: Dev1 = 2, Dev2 = 1, Dev3 = 1, Dev4 = 1). For Exp 2, six fully functional devices were assembled and used to complete the experiment. All devices were used more than once (number of times used: Dev1 = 4, Dev2 = 4, Dev3 = 5, Dev4 = 6, Dev5 = 5, Dev6 = 3).
The fluid reservoirs were filled manually using syringe and needle. In Exp 1, only one reservoir was partially filled with 2 mL of solution, whereas in Exp 2, either one reservoir was partially filled with 2 mL of solution for the low volume treatments (LoD-LoV and HiD-LoV) or two reservoirs were filled completely (i.e., 5 mL each) for the (LoD-HiV and HiD-HiV) treatments. To avoid delivery of less than the target amount of solution due to filling tubbing and peristaltic pumps, each reservoir was filled with an extra 0.2 mL of solution. Each device was programmed independently as described in the companion manuscript (Ren et al., 2023), to run for 5 s to determine proper functionality. This was evaluated by hearing the noise made by pumps when activated and observation of fluid in the device external opening. Prior to IVG insertion, devices were programmed to have individual pumps run for 1,000 s, based on the known release rate of fluid (Masello et al., 2020). For treatments which required delivery of solution from two reservoirs, pumps were programmed to run separately and in sequence. Thus, e-Synch devices for the high-volume treatments required 2,000 s (33.3 min) to release the contents of both reservoirs. Pumps were programmed to run for 60 s longer than the time required to release the solutions to ensure delivery of all content.
Before device insertion, the vulva and perineal area was cleaned and disinfected using 2% Chlorhexidine solution (Nolvasan, Fort Dodge Animal Health, Fort Dodge, IA, USA) diluted in water. After scrubbing, the perineal area was dried off with paper towels. Individual devices were turned on and functionality evaluated as described above. Devices were mounted in the front portion of the custom-built applicator, which was rubbed with a thin film of sterile lubricant (Priority Care, First Priority Inc, Elgin, IL, USA). The applicator containing the device was inserted into the vagina until it reached the vaginal fornix. At this point, the applicator was pulled backwards 5 to 10 cm to enable release of the e-Synch device through pressure on the applicator rod. Once the device was released, the applicator was removed. In both experiments, devices remained in the cows for up to 8 h after insertion. During insertion, the gateway was placed within the barn approximately 10 m away from the cows to enable communication with devices for data collection, as described in the companion manuscript (Ren et al., 2023).
Devices were removed from the vagina through transrectal application of pressure on the front end of the device until it was reachable through the vaginal opening. After removal from the vagina, devices were disassembled to confirm hormone release from reservoirs. All except for one device in Exp 2 released the hormone solution. In all cases, reservoirs from all devices that functioned properly were empty.
In both experiments, blood was collected at the same timepoints. Samples were collected on d -9, -2, 0, and 7 relative to treatment day for estimation of circulating concentrations of progesterone (P4). Data for circulating concentrations of P4 was used to assess response to synchronization, confirm luteal regression before application of treatments, and to aid with confirmation of ovulation. On the day of treatment (d 0), blood was collected at 0, 1, 2, 2.5, 3, 4, 6, and 8 h after i.m. injection or e-Synch device start of Gonadorelin solution release to estimate circulating concentrations of LH. All samples were collected from coccygeal blood vessels using evacuated tubes containing sodium heparin (Vacutainer, BD, Franklin Lakes, NJ, USA). After collection, blood tubes were immediately placed in crushed ice until centrifugation for 20 minutes at 2,000 x g in a refrigerated centrifuge set at -4°C. After centrifugation, plasma was harvested and stored in duplicates in 5 mL Eppendorf tubes at -20°C until assayed.
In both experiments, transrectal ultrasonography (TUS) of the ovaries and reproductive tract was conducted at the time of the first GnRH and PGF treatments of the Breeding-Ovsynch portion of the DO protocol, immediately before application of treatments, and 7 d after treatment to evaluate response to the synchronization protocol and ovulation after treatments. In Exp 2, an additional TUS session was conducted 48 h after treatment. At each TUS session, the location and size of all follicles > 4 mm and all corpora lutea present were recorded on ovarian maps. Ovulation was determined 7 d after treatment based on the presence of a corpus luteum (CL) at the same location where the largest follicle was present at the time of treatment. In Exp 2, ovulation was also confirmed based on follicle disappearance within 48 h of treatment. All TUS sessions were conducted using a portable ultrasound machine with an attached 7.5 MHz linear array transducer (Ibex Pro; E.I. Medical Imaging, Loveland, CO, USA).
In both experiments, body condition score (BCS) on a scale from 1 to 5 (Edmonson et al., 1989) and body weight (BW) in kg were recorded during the synchronization protocol. Body condition was recorded at the time of the first GnRH of the Breeding-Ovsynch portion and BW was collected using an electronic walk-over scale one day before treatments.
The effect of e-Synch insertion on integrity of the vaginal mucosa and cow behavior were evaluated in both experiments as described in detail in the companion manuscript Ren et al. (2023). Data for vaginal integrity, mucus score, and cow behavior are presented in the companion manuscript.
Samples collected for determination of circulating concentrations of P4 in both experiments were analyzed in duplicate using a commercial solid-phase, no-extraction radioimmunoassay (ImmuChem Coated Tube; MP Biomedicals, Solon, OH, USA). The detection limit of the assay was 0.1 ng/mL. Samples from Exp 1 were run in a single assay including samples with known concentrations of P4 (4.5 and 0.9 ng/mL) at the beginning, middle, and end of the assay to assess reliability. The average intra-assay coefficient of variation (CV) was 2.6 and 6.6% for the high and low P4 concentration sample, respectively. Samples from Exp 2, were run in two separate assays. For the first assay, the intra-assay CV, based on the same quality control samples, was the same as the samples from Exp 1. For the second assay the intra-assay CV was 1.9 and 3.0%, for P4 samples with high (3.4 ng/mL) and low (0.5 ng/mL) concentrations, respectively, included at the beginning, middle, and end of the assay. The inter-assay CV could not be estimated due to the use of different quality control samples.
Samples collected from 0 to 8 h after treatments in both experiments were analyzed for determination of circulating concentrations of LH at the Animal Reproduction and Biotechnology Laboratory at Colorado State University, USA. Plasma samples were run in duplicates in a radioimmunoassay validated for bovine and described in detail in Niswender et al. (1969). The standard curve for the assay ranged from 1 to 28 pg/mL of LH. Samples with concentrations expected to fall above the limit of the standard curve were run in different volumes (20, 50, or 200 μL of sample) to have at least one sample fall within the most reliable range of the standard curve (20 to 90% binding). Samples from Exp 1 were run in a single assay with a sensitivity of 0.13 ng/mL and an intra-assay CV of 10.0% based on three quality control samples with concentrations of 0.32, 4.13, and 10.93 ng/mL. Samples from Exp 2 were run in two assays with sensitivity of 0.24 and 0.23 ng/mL, respectively. The intra-assay CV was 4.2% for assay 1 and 12.5% for assay 2, based on the same quality control samples used for the assay for samples in Exp 1. The average inter-assay CV for all quality control samples was 11.7%.
Unless otherwise stated, the same methodology was used to analyze data from Exp 1 and 2. To be included in data analyses, cows had to respond to the synchronization of ovulation protocol, which was defined as the presence of a functional CL (P4 > 1 ng/mL) at the time of the first PGF treatment of the Breeding-Ovsynch portion of the DO protocol, followed by complete CL regression (P4 < 1 ng/mL).
A surge of LH after treatment was defined for individual cows in both experiments. A surge was defined as an increase in mean circulating concentrations of LH from basal levels (0 h) that was equal or greater to the mean increase from basal to maximum concentrations minus two standard deviations for the GnRH-IM treatment in the same experiment. The cutoff concentration value to confirm a surge of LH was 12.7 ng/mL and 1.6 ng/mL for Exp 1 and Exp 2, respectively. Area under the curve (AUC) for LH concentrations from 0 to 8 h after treatment was approximated through the trapezoidal method, as described in Giordano et al. (2012a).
Continuous data (concentrations of P4 at the time of treatment, concentrations of LH overtime, AUC for concentrations of LH, maximum LH concentration, and time to maximum LH concentration) were analyzed with linear mixed models fitting a normal distribution with or without repeated measurements using the MIXED procedure of SAS (version 9.4, SAS Institute Inc., Cary, NC, USA). Normality of the raw data was evaluated using the Shapiro-Wilk statistic generated with the UNIVARIATE procedure of SAS. In addition, normality of residuals and homoscedasticity of variance were verified using graphical methods (Q-Q plot and conditional studentized residual plot) generated with the residual option of the MIXED procedure of SAS. Except for concentrations of P4 7 d after treatment, all other data required transformations because assumptions of normality and homosedasticity of variance were violated. For Exp 1, data for LH mean concentrations overtime were log transformed, whereas data for LH AUC and maximum LH concentrations were square root transformed. No transformation corrected the lack of normality of P4 concentration at d 0 and therefore, the non-parametric Kruskal-Wallis test generated using the NPAR1WAY procedure of SAS was used. For Exp 2, data for P4 concentrations at d 0, mean LH concentrations overtime, LH AUC, and maximum LH concentrations were log transformed. Data for time to maximum LH concentration underwent an inverse transformation for positively skewed data.
All models for non-repeated measures data included treatment as fixed effect. Luteinizing hormone concentration was analyzed using models for repeated measures, with a spatial power covariance structure to adjust for varying intervals in blood sample collection, and included treatment, time, and the treatment by time interaction as fixed effects. For all models, parity group (primiparous vs. multiparous), BCS, and BW as continuous values were offered as confounders. Final models were obtained by backwards stepwise selection. All confounders were removed from all models since P > 0.10. Cow nested within treatment was included as a random effect for all models. When appropriate, the Least Significant Difference post-hoc mean separation test was used to determine differences between Least Square Means.
Even though the experiment was not designed to test hypotheses nor to validate effects of treatments on binomial outcome data, these outcomes were recorded and analyzed. Binomial outcomes (proportion of cows with a surge of LH and cows with confirmed ovulation after treatment) were analyzed using Fisher’s exact test with the FREQ procedure of SAS because some treatment groups had either 0% or 100% of the cows with a positive outcome, and several groups had less than 5 observations for the event of interest. Mean separation analysis was not conducted for binomial outcomes because of the small number of observations did not allow for meaningful comparisons.
All values for continuous variables are presented as arithmetic means and SE generated with the MEANS procedure of SAS. All proportions were generated using the FREQ procedure of SAS. All explanatory variables included as fixed effects in models were considered significant if P < 0.05, whereas 0.05 ≤ P ≤ 0.10 were considered a tendency.
Two cows (GnRH-IM n = 1; GnRH-eS n = 1) were removed prior to data analysis because circulating concentrations of P4 at the time of induction of luteolysis with PGF in the Breeding-Ovsynch portion of the DO protocol (i.e., 48 h before treatment) were <1 ng/mL. This P4 concentration was indicative of the absence of a functional CL that could respond to the PGF treatment. In addition, both cows had P4 concentrations >1 ng/mL (7.4 and 4.0 ng/mL) at the time of the first GnRH of the Breeding-Ovsynch portion of the DO protocol, which confirmed spontaneous CL regression from the time of the GnRH to the PGF treatment of the protocol. Another cow from the GnRH-IM treatment was removed because circulating concentrations of LH were more than 10-fold greater than for the rest of the cows in the GnRH-IM treatment. After removal of all cows that did not meet the criteria to be included in data analysis, four cows were left for the GnRH-IM treatment and five cows each for the Placebo-eS and GnRH-eS groups.
Mean plasma concentrations of P4 at the time of treatment (d 0) were not different between treatments (P = 0.52; Table 1). All treatments had mean P4 concentrations of 0.1 ± 0.1 ng/mL, which is below the 1 ng/mL cutoff used to confirm complete luteal regression. Similarly, at the individual cow level, all cows had P4 concentrations <1 ng/mL. The maximum individual P4 concentration was 0.17 ng/mL.
Table 1 Circulating concentrations of progesterone (P4) at 0 h relative to treatment administration, luteinizing hormone (LH) secretion dynamics, and ovulation after treatment in Experiment 1.
Mean plasma concentrations of LH after treatment were affected by treatment (P < 0.001), time (P < 0.001), and the treatment by time interaction (P < 0.001). Cows in the GnRH-IM group had greater LH concentrations from 1 to 4 h after treatment than cows in the Placebo-eS and GnRH-eS treatments (Figure 2). At 6 h after treatment, cows in the GnRH-IM treatment only had greater LH concentrations than cows in the GnRH-eS treatment. At 8 h, all treatments had similar LH concentrations.
Figure 2 Circulating concentrations of luteinizing hormone (LH) from 0 to 8 h after treatment for cows in experiment 1. At 48 h after induction of luteolysis, lactating Holstein cows were randomly assigned to receive 100 µg of Gonadorelin in 2 mL by intramuscular injection (GnRH-IM; n = 4), an empty e-Synch device (Placebo-eS; n = 5), or 100 µg of Gonadorelin in 2 mL of solution containing citric acid delivered intravaginally with the e-Synch device (GnRH-eS; n = 5). Mean circulating concentrations of LH after treatment were affected by treatment (P < 0.001), time (P < 0.001), and the treatment by time interaction (P < 0.001). *Cows in the GnRH-IM group had greater (P < 0.05) LH concentrations than cows in the Placebo-eS and GnRH-eS treatments based on the LSD post-hoc mean separation test. Other differences described in the text. Trt, treatment.
Cows in the GnRH-IM treatment had greater AUC (P < 0.001) and maximum LH concentration (P < 0.001) than cows in the Placebo-eS and GnRH-eS treatment (Table 1). The AUC for LH was 8.7 and 6.9 times greater for the GnRH-IM than the Placebo-eS and GnRH-eS treatments, respectively. Similarly, the maximum LH concentration was 16 and 10 times greater for the GnRH-IM than the Placebo-eS and GnRH-eS treatments, respectively. An LH surge was only detected for the cows on the GnRH-IM treatment (rise in LH from baseline of > 12.7 ng/mL); therefore, time to maximum LH concentration and the proportion of cows with a surge of LH (Table 1) could only be estimated for the GnRH-IM treatment.
All cows from all treatments had a CL detected by TUS 7 d after treatment. Circulating concentrations of P4 7 d after treatment were greater (P = 0.02) for the GnRH-IM than for the Placebo-eS and GnRH-eS treatments (Table 1).
One cow from the HiD-HiV treatment was removed prior to data analysis because the device failed to release the Gonadorelin solution. Four cows (LoD-HiV n = 1; HiD-LoV n=1; HiD-HiV n = 2) were removed prior to data analysis because plasma P4 was < 1 ng/mL at the time of induction of luteolysis with PGF in the Breeding-Ovsynch portion of the DO protocol (i.e., 48 h before treatment). In addition, these cows had P4 concentrations >1 ng/mL (3.9, 5.6, 3.0, and 6.0 ng/mL) at the time of the first GnRH of the Breeding-Ovsynch, which confirmed spontaneous CL regression from the time of the GnRH to the PGF treatment of the protocol. After removal of cows not available for analysis, the number of cows per treatment for reporting of results were: 7 for GnRH-IM, 6 for LoD-LoV, 6 for LoD-HiV, 6 for HiD-LoV, and 4 for HiD-HiV.
Mean plasma concentrations of P4 at the time of treatment (i.e., d 0) were not different between treatments (P = 0.17; Table 2). All treatments had mean P4 concentrations below the 1.0 ng/mL cutoff used to confirm complete luteal regression. Similarly, at the individual cow level, all cows had P4 concentrations < 1 ng/mL. The cow with the greatest circulating P4 concentration had 0.9 ng/mL whereas all other cows had < 0.3 ng/mL of P4.
Table 2 Circulating concentrations of progesterone (P4) at 0 h relative to treatment administration, luteinizing hormone (LH) secretion dynamics, and ovulation after treatment in Experiment 2.
Mean circulating concentrations of LH after treatment (Figure 3) were affected by treatment (P < 0.001), time (P < 0.001), and the treatment by time interaction (P < 0.001). At 0 h, there were no differences in LH concentrations between treatments, except a 0.55 ng/mL difference (P < 0.05) between the HiD-HiV and the LoD-LoV treatments. From 1 to 6 h after treatment, cows in the GnRH-IM, HiD-LoV, and HiD-HiV treatments had greater LH concentrations than cows in the LoD-HiV and LoD-LoV treatments. During the same period concentrations of LH did not differ at any time point for the HiD-LoV and HiD-HiV treatments or among these two treatments and the GnRH-IM treatment. There were only two exceptions, whereby at 1 h the HiD-HiV treatment had smaller LH concentrations than the GnRH-IM treatment and at 4 h when the GnRH-IM had smaller LH concentrations than both the HiD-LoV and HiD-HiV treatments. Also, the LoD-HiV treatment had greater mean LH concentrations than the LoD-LoV treatment from 2.5 to 6 h. At the end of the sampling period at 8 h after treatment, there were no differences (P > 0.05) in mean LH concentrations among treatments except for greater (P < 0.03) LH concentrations observed for the HiD-HiV than the LoD-LoV treatment (0.9 ng/mL).
Figure 3 Circulating concentrations of luteinizing hormone (LH) from 0 to 8 h after treatment for cows in experiment 2. At 48 h after induction of luteolysis, lactating Holstein cows were randomly assigned to receive 100 µg of Gonadorelin in 2 mL of solution by intramuscular injection (GnRH-IM; n = 7), 100 µg of Gonadorelin in 2 mL (LoD-LoV; n = 6), 100 µg of Gonadorelin in 10 mL (LoD-HiV; n = 6), 1,000 µg of Gonadorelin in 2 mL (HiD-LoV; n, 6), or 1,000 µg of Gonadorelin in 10 mL (HiD-HiV; n = 4) with the e-Synch device. Blood was collected at 0, 1, 2, 2.5, 3, 4, 6 and 8 h after treatment. Mean circulating concentrations of LH were affected by treatment (P < 0.001), time (P < 0.001), and the treatment by time interaction (P < 0.001). *HiD-Lov, HiD-HiV, and GnRH-IM were greater (P < 0.05) than the LoD-LoV and LoD-HiV treatments, based on the LSD post-hoc mean separation test. †LoD-HiV was greater (P < 0.05) than the LoD-LoV treatment, based on the LSD post-hoc mean separation test. Other differences described in the text. Trt, treatment.
Cows on the GnRH-IM, HiD-LoV, and HiD-HiV treatments had greater AUC (P < 0.001) than cows in the other treatments, whereas cows on the LoD-HiV treatment had greater AUC than cows on the LoD-LoV treatment (Table 2). Despite a 1.5 fold greater AUC, there were no significant differences between cows on the HiD-LoV and HiD-HiV treatments and cows on the GnRH-IM treatment. Maximum LH concentration was also greater (P < 0.001) for cows on the GnRH-IM, HiD-LoV, and HiD-HiV treatments than for cows on the LoD-HiV and LoD-LoV treatments for which maximum LH concentrations did not differ (Table 2). The maximum LH concentration observed was 1.4 fold greater for HiD-LoV and HiD-HiV than for the GnRH-IM treatment but did not differ. Time to the maximum LH concentration ranged from 120 to 165 min but did not differ (P = 0.29) among treatments. The proportion of cows with a surge of LH differed among treatments (P = 0.05) because all cows on the GnRH-IM, HiD-LoV, and HiD-HiV treatments had an LH surge but not all cows on the LoD-HiV and LoD-LoV treatments had an LH surge (Table 2).
Based on the TUS session at 48 h after treatment, the proportion of cows with follicle disappearance confirmed differed amongst treatments (P = 0.03; Table 2); however, no difference was observed for the proportion of cows with a CL observed 7 d after treatment (P = 0.17; Table 2). This difference was observed even though almost all cows on the GnRH-IM, HiD-LoV, and HiD-HiV treatments had a CL, whereas only half of the cows on the LoD-HiV and LoD-LoV treatments had a CL. Plasma concentrations of P4 7 d after treatment differed (P = 0.02) amongst treatments. Cows on the HiD-LoV treatment had the greatest concentration, cows on the GnRH-IM and HiD-HiV treatments were intermediate, and cows on the LoD-LoV and LoD-HiV had the smallest concentrations (Table 2).
In cattle, GnRH is one of the most widely used reproductive hormones in synchronization of ovulation protocols (De Rensis and Peters, 1999; Wiltbank and Pursley, 2014) and can also be used for inducing ovulation to resolve anovulatory conditions (Benmrad and Stevenson, 1986; McDougall et al., 2001), treat cystic ovarian disease (Kesler and Garverick, 1982; Nanda et al., 1988; López-Gatius and López-Béjar, 2002), and inducing accessory corpora lutea after insemination (Howard et al., 2006; Besbaci et al., 2020). Therefore, in a set of experiments, we tested the ability of inducing LH release in dairy cows after IVG instillation of the GnRH analogue Gonadorelin using the e-Synch device. The ability to induce a surge of LH after delivery of GnRH analogues with e-Synch is critical for enabling automated synchronization of ovulation and other uses of GnRH in cattle.
Data for all LH response outcomes in Exp 1 did not support our hypothesis that IVG instillation of 100 μg of Gonadorelin mixed with CA would generate a similar LH release response than through i.m. injection. In a first experiment presented in Wijma et al. (2017), the same dose or up to 10 times the dose of Gonadorelin delivered in the cranial portion of the vagina of lactating dairy cows with a catheter also failed to cause a rise in LH. In this previous experiment, lack of Gonadorelin absorption was proposed as the most likely reason for not observing a response after treatment. In agreement, in the second experiment presented in Wijma et al. (2017) we demonstrated that the addition of CA to solutions containing 10 times the dose used for induction of ovulation of the GnRH analogues Gonadorelin and Buserelin caused an LH surge of greater magnitude than a 100 μg i.m. injection of Gonadorelin. When added to solutions for IVG instillation of hydrophilic molecules, CA acts as an absorption enhancer through intact vaginal mucosa. Studies with rats demonstrated that CA favors paracellular transport of molecules by disrupting intercellular junctions (Okada et al., 1982; Okada et al., 1983; Fatakdawala and Uhland, 2011). Thus, in Exp 1 we expected that the addition of CA to the Gonadorelin solution would cause absorption and passage of enough GnRH into circulation to elicit a similar LH response than after i.m. injection of 100 μg of Gonadorelin. The rationale for using a 100 μg dose of the GnRH analogue Gonadorelin in 2 mL of solution was to minimize both the dose and volume needed for use with e-Synch. Demonstrating equivalency for IVG delivery and i.m. injection of 100 μg of Gonadorelin is desirable because this is one of the doses currently approved for induction of ovulation and most widely used concentration for synchronization of ovulation in cattle. On the other hand, using 2 mL of solution is considerably less than the volume used (i.e., 10 mL) to cause an LH surge of normal magnitude in our previous research (Wijma et al., 2017). Minimizing the volume of hormone solution needed to elicit a biological response, such as ovulation or luteolysis, is relevant to e-Synch development and refinement because there is a limited size of the fluid reservoirs in the device. Contrary to our hypothesis, results demonstrated that the GnRH analogue used was either not absorbed or that a smaller amount than needed to cause an LH surge is absorbed when 2 mL of a commercially available solution containing 100 μg of Gonadorelin mixed with CA is given with e-Synch.
Including the Placebo treatment in Exp 1 was necessary to demonstrate that the presence of e-Synch in the vaginal cavity of cows does not cause LH release. In a previous experiment to evaluate feasibility of inducing CL regression with PGF in lactating dairy cows, we included a placebo treatment as a negative control; however, circulating concentrations of LH were not measured (Masello et al., 2020). Based on the circulating LH concentration dynamic observed in Exp 1, it was obvious that the mere presence of an e-Synch device in the vaginal cavity of cows for up to 8 h did not cause LH release. Based on these observations, no placebo negative control group was included in Exp 2. Inclusion of the Placebo treatment as a negative control also helped us understand the Gonadorelin absorption dynamic when using the 100 μg dose in 2 mL of solution, as similar LH concentrations for the Placebo-eS and GnRH-eS treatment suggested that either none or a very small amount of the Gonadorelin delivered by e-Synch was absorbed.
Visualization of a CL seven days after treatment on the same location in which a pre-ovulatory follicle was present indicated that cows ovulated at some point from treatment to evaluation of the ovaries by TUS. In addition, mean plasma concentrations of P4 at d 7 were 0.7 and 1.0 ng/mL in the Placebo-eS and GnRH-eS treatments, respectively. Although smaller than for the GnRH-IM treatment, P4 concentrations increased several fold from the baseline concentrations observed at the time of treatment. Collectively, data confirmed the development and presence of luteal tissue on the ovaries after treatment. Although this observation was unexpected for cows in the Placebo-eS and GnRH-eS treatments, it is not possible to rule out that ovulation occurred after delayed absorption of GnRH or ovulation after spontaneous estrus. As blood sampling was discontinued at 8 h after treatment and estrus was not monitored in this experiment, it is not possible to speculate which one of the two possible mechanisms explains the presence of luteal tissue at the time of the TUS examination. Thus, in future experiments designed to evaluate ovulatory response after administration of GnRH analogues with e-Synch, different sampling period durations and multiple criteria to confirm ovulation (included in Exp 2) might be needed.
Data for all outcomes in Exp 2 supported our hypothesis that the greater dose of Gonadorelin would elicit more LH release than the smaller dose. Moreover, the LH response, as determined by AUC, mean LH concentrations overtime, and the maximum LH concentration observed, was similar for both high dose GnRH treatments and the GnRH-IM treatment. These data supported the hypothesis that at least a similar response would be observed for a larger dose of GnRH and an i.m. injection of 100 μg of Gonadorelin. Conversely, data partially supported the hypothesis that a larger volume of solution, regardless of dose of Gonadorelin, would increase the LH response. Volume of administration had no detectable effect on LH release with the 1,000 μg dose; however, there was an effect for the 100 μg dose of Gonadorelin. Although we observed a minor increase in LH when using the 10 mL volume for the 100 μg dose, the increased volume did not compensate for the effect of dose. Although the AUC and maximum LH concentrations doubled with the increase in solution volume from 2 to 10 mL for the 100 μg dose, the LH response was several fold smaller than for the larger dose treatments. Altogether, these data suggested that more than 100 μg of the GnRH analogue Gonadorelin will be needed to induce an LH surge of sufficient magnitude to induce ovulation when administered through the IVG route with the e-Synch device. On the other hand, the small gains observed by increasing volume of solution for delivering the same dose of GnRH indicated that within the range of volume tested, absorption of GnRH might not be affected sufficiently as to justify the use of larger volumes of solution.
Results from these experiments have several implications for future e-Synch design and implementation in the field. In Exp 1, we confirmed a lack of response to the dose typically used for inducing ovulation after i.m. injection of GnRH analogues, which indicated that larger doses of the GnRH analogue Gonadorelin are needed to cause an LH surge of at least similar magnitude when given via e-Synch. It remains to be determined if the full 1,000 μg dose is necessary, or if it is possible to elicit an LH surge of sufficient magnitude to induce ovulation with < 1,000 μg of Gonadorelin. Although not statistically significant, in both experiments the total amount of LH released (i.e., AUC) over the sampling period was 1.1 to 1.5 fold greater for the 1,000 μg dose given vaginally. Based on these observations and observations from our previous experiment in which a 1,000 μg dose of Gonadorelin was used (Wijma et al., 2017), it seems plausible that doses of Gonadorelin of < 1,000 μg delivered by e-Synch could cause a similar response as a 100 μg i.m. injection. The need for a larger dose of a hormone to induce a desired physiological response after administration with e-Synch does not seem to be unique to GnRH analogues. In a previous experiment (Masello et al., 2020), we observed the same P4 concentration profile and proportion of cows with complete luteal regression after administration of four times the dose of the natural form of PGF approved for inducing luteolysis in cattle in the US (i.e., 25 mg of Dinoprost). The latter suggested that four times the dose of PGF given with e-Synch was equivalent to one time the dose given by the i.m. route. Ultimately, optimizing the dose of GnRH for IVG administration with e-Synch or any other method would be relevant to minimize cost of implementing automated synchronization of ovulation by commercial farms.
Another important implication of the results of Exp 2 was that it was not necessary to increase the volume of solution when using a dose of Gonadorelin that induced an LH surge of similar magnitude to that of a 100 μg i.m. injection. This is relevant because minimizing the total volume needed for delivery of an effective hormonal treatment is critical to enable device use in the field and minimize device dimensions. Delivering the dose of GnRH analogue needed in the least amount of solution is relevant because some synchronization of ovulation protocols used for timed AI may require up three to four separate GnRH treatments (Bello et al., 2006; Souza et al., 2008; Giordano et al., 2012b). Minimizing device size would also be ideal for ease of insertion and best cow comfort.
Although the limited sample size of Exp 2 precludes a valid interpretation of results for binary outcomes, the results for ovulation after treatment were more in line with expectations based on the LH concentration dynamics for the different treatments. Only a third of the cows had ovulation (i.e., 2 out 6) confirmed by follicle disappearance in the treatments that received the low dose of Gonadorelin, which did not have an LH surge. In contrast, 75% (i.e., 3 out 4 ovulated) and 83% (i.e., 5 out 6 ovulated) of the cows that received the larger dose of GnRH with e-Synch and 100% (i.e., 7 out 7 ovulated) of cows in the GnRH-IM treatment presented evidence of ovulation and had a surge of LH. Results for circulating concentrations of P4 were also in general agreement with results for ovulation and the LH dynamics, as cows that received the larger dose had or tended to have greater circulating concentrations of P4 7 d after treatment. The few more cows with apparent ovulation from 2 to 7 d after treatment confirmed that some cows must have had a surge of LH either after estrus or, although less likely, after delayed absorption of GnRH. In this regard, a limitation of our experiments was discontinuation of blood sampling at 8 h after treatment. This duration of sampling precluded evaluating if Gonadorelin was absorbed and caused LH release after sampling concluded. The reasoning for the approach used was that LH concentrations typically return to baseline levels by 6 to 8 h after i.m. injection of GnRH analogues (Colazo et al., 2009; Giordano et al., 2012a; Armengol-Gelonch et al., 2017). Secondly, we aimed to minimize the number of samples collected because blood was drawn by puncture of the tail blood vessels rather than through jugular catheters. Therefore, future experiments should include sampling beyond 8 h of treatment to rule out or confirm delayed absorption of GnRH.
We conclude that the current e-Synch device can be programmed to automatically release Gonadorelin solution and CA as an absorption enhancer in a dose and volume that successfully induces a surge of LH of at least similar magnitude as a 100 μg i.m. injection. Further research is needed to determine the minimum dose of GnRH delivered by e-Synch that optimizes LH release to achieve the same ovulatory response as an i.m. injection of the dose of GnRH analogues typically used in synchronization of ovulation protocols for cattle. We also conclude that the dose of GnRH delivered is more critical than the volume in which a set dose of GnRH is delivered. Volume of administration was also less relevant for doses that successfully induced a surge of LH. Minimizing the volume required to deliver a hormone dose that causes a desired biological response is of utmost importance for reducing the size of the e-Synch device or other devices for IVG delivery of hormones.
The authors have filed a patent application US 63/016,235 for the system(s), device(s) and method(s) published in this paper.
This article is the second part of a set of companion articles. The companion article is Ren, Y., Duhatschek, D., Bartolomeu, C. C., Erickson, D., and Giordano, J. O. (2023). An automated system for cattle reproductive management under the IoT framework. Part I: The e-synch system and cow responses. Front. Anim. Sci 4, 1093851. doi: 10.3389/fanim.2023.1093851.
The original contributions presented in the study are included in the article/supplementary materials. Further inquiries can be directed to the corresponding author.
The animal study was reviewed and approved by the Animal Care and Use Committee of Cornell University (Ithaca, NY) under protocols 2016-0093 and 2021-0010.
YR, DE, and JG designed the e-Synch device. YR, DD, DE, CB, and JG contributed to the design of the experiments conducted. YR, DD, CB, AL, CR, MP, and JG carried out the experiments. YR and DD wrote a first draft of the manuscript and DE and JG revised and edited the manuscript. All authors contributed to the article and approved the submitted version.
This project was supported by Agriculture and Food Research Initiative Competitive Grant no. 2021-67015-34503 from the USDA National Institute of Food and Agriculture (Washington, DC). Any opinions, findings, conclusions, or recommendations expressed in this publication are those of the authors and do not necessarily reflect the view of the National Institute of Food and Agriculture (NIFA) or the USDA. Partial support was also provided by project numbers 1014955 and 1007421 from the USDA National Institute of Food and Agriculture and the Research Innovation Fund of the Cornell Initiative for Digital Agriculture (Cornell University, Ithaca, NY).
We thank Sheridan Tompkins from the Department of Animal Science at Cornell University (Ithaca, NY) for her help with making the GnRH solutions. We thank personnel from the Dairy Unit of the Cornell University Ruminant Center (Harford, NY) for helping with cow management.
The authors have filed a patent application US 63/016,235 for the systems, devices and methods published in this paper.
The authors declare that the research was conducted in the absence of any commercial or financial relationships that could be construed as a potential conflict of interest.
All claims expressed in this article are solely those of the authors and do not necessarily represent those of their affiliated organizations, or those of the publisher, the editors and the reviewers. Any product that may be evaluated in this article, or claim that may be made by its manufacturer, is not guaranteed or endorsed by the publisher.
Aquilani C., Confessore A., Bozzi R., Sirtori F., Pugliese C. (2022). Review: Precision livestock farming technologies in pasture-based livestock systems. Animal 16, 100429. doi: 10.1016/j.animal.2021.100429
Armengol-Gelonch R., Mallo J. M., Ponté D., Jimenez A., Valenza A., Souza A. H. (2017). Impact of phase of the estrous cycle and season on LH surge profile and fertility in dairy cows treated with different GnRH analogs (gonadorelin vs. buserelin). Theriogenology 91, 121–126. doi: 10.1016/j.theriogenology.2017.01.001
Bello N. M., Steibel J. P., Pursley J. R. (2006). Optimizing ovulation to first GnRH improved outcomes to each hormonal injection of ovsynch in lactating dairy cows. J. Dairy Sci. 89, 3413–3424. doi: 10.3168/jds.S0022-0302(06)72378-5
Benmrad M., Stevenson J. S. (1986). Gonadotropin-releasing hormone and prostaglandin F2 alpha for postpartum dairy cows: Estrous, ovulation, and fertility traits. J. Dairy Sci. 69, 800–811. doi: 10.3168/jds.S0022-0302(86)80469-6
Berckmans D. (2017). General introduction to precision livestock farming. Anim. Front. 7, 6–11. doi: 10.2527/af.2017.0102%JAnimalFrontiers
Besbaci M., Abdelli A., Minviel J. J., Belabdi I., Kaidi R., Raboisson D. (2020). Association of pregnancy per artificial insemination with gonadotropin-releasing hormone and human chorionic gonadotropin administered during the luteal phase after artificial insemination in dairy cows: A meta-analysis. J. Dairy Sci. 103, 2006–2018. doi: 10.3168/jds.2019-16439
Colazo M. G., Ree T. O., Emmanuel D. G. V., Ambrose D. J. (2009). Plasma luteinizing hormone concentrations in cows given repeated treatments or three different doses of gonadotropin releasing hormone. Theriogenology 71, 984–992. doi: 10.1016/j.theriogenology.2008.11.002
De Rensis F., Peters A. R. (1999). The control of follicular dynamics by PGF2α, gnrh, hCG and oestrus synchronization in cattle. Reprod. Domest. Anim. 34, 49–59. doi: 10.1111/j.1439-0531.1999.tb01383.x
Edmonson A. J., Lean I. J., Weaver L. D., Farver T., Webster G. (1989). A body condition scoring chart for Holstein dairy cows. J. Dairy Sci. 72, 68–78. doi: 10.3168/jds.S0022-0302(89)79081-0
Fatakdawala H., Uhland S. A. (2011). Hydrogen peroxide mediated transvaginal drug delivery. Int. J. Pharm. 409, 121–127. doi: 10.1016/j.ijpharm.2011.02.052
Giordano J. O., Fricke P. M., Guenther J. N., Lopes G. Jr., Herlihy M. M., Nascimento A. B., et al. (2012a). Effect of progesterone on magnitude of the luteinizing hormone surge induced by two different doses of gonadotropin-releasing hormone in lactating dairy cows. J. Dairy Sci. 95, 3781–3793. doi: 10.3168/jds.2011-5155
Giordano J. O., Wiltbank M. C., Guenther J. N., Pawlisch R., Bas S., Cunha A. P., et al. (2012b). Increased fertility in lactating dairy cows resynchronized with double-ovsynch compared with ovsynch initiated 32 d after timed artificial insemination. J. Dairy Sci. 95, 639–653. doi: 10.3168/jds.2011-4418
Halachmi I., Guarino M., Bewley J., Pastell M. J. A. R. A. B. (2019). Smart animal agriculture: Application of real-time sensors to improve animal well-being and production 7, 403–425. doi: 10.1146/annurev-animal-020518-114851
Howard J. M., Manzo R., Dalton J. C., Frago F., Ahmadzadeh A. (2006). Conception rates and serum progesterone concentration in dairy cattle administered gonadotropin releasing hormone 5 days after artificial insemination. Anim. Reprod. Sci. 95, 224–233. doi: 10.1016/j.anireprosci.2005.10.010
Kesler D. J., Garverick H. A. (1982). Ovarian cysts in dairy cattle: A review. J. Anim. Sci. 55, 1147–1159. doi: 10.2527/jas1982.5551147x
López-Gatius F., López-Béjar M. (2002). Reproductive performance of dairy cows with ovarian cysts after different GnRH and cloprostenol treatments. Theriogenology 58, 1337–1348. doi: 10.1016/s0093-691x(02)00952-4
Masello M., Ren Y., Erickson D., Giordano J. O. (2020). An automated controlled-release device for intravaginal hormone delivery. JDS Commun. 1, 15–20. doi: 10.3168/jdsc.2020-18816
McDougall S., Cullum A. A., Annis F. M., Rhodes F. M. (2001). Treatment of anovulatory anoestrous postpartum dairy cows with a gonadotropin-releasing hormone (GnRH), prostaglandin F2 alpha, GnRH regimen or with progesterone and oestradiol benzoate. New Z. Vet. J. 49, 168–172. doi: 10.1080/00480169.2001.36228
Motta J. C. L., Madureira G., Silva L. O., Alves R., Silvestri M., Drum J. N., et al. (2020). Interactions of circulating estradiol and progesterone on changes in endometrial area and pituitary responsiveness to GnRH†. Biol. Reprod. 103, 643–653. doi: 10.1093/biolre/ioaa065
Nanda A. S., Ward W. R., Williams P. C., Dobson H. (1988). Retrospective analysis of the efficacy of different hormone treatments of cystic ovarian disease in cattle. Vet. Rec. 122, 155–158. doi: 10.1136/vr.122.7.155
Niswender G. D., Reichert L. E. Jr., Midgley A. R. Jr., Nalbandov A. V. (1969). Radioimmunoassay for bovine and ovine luteinizing hormone. Endocrinology 84, 1166–1173. doi: 10.1210/endo-84-5-1166
Okada H., Yamazaki I., Ogawa Y., Hirai S., Yashiki T., Mima H. (1982). Vaginal absorption of a potent luteinizing hormone-releasing hormone analog (leuprolide) in rats I: Absorption by various routes and absorption enhancement. J. Pharm. Sci. 71, 1367–1371. doi: 10.1002/jps.2600711214
Okada H., Yashiki T., Mima H. (1983). Vaginal absorption of a potent luteinizing hormone-releasing hormone analogue (leuprolide) in rats III: Effect of estrous cycle on vaginal absorption of hydrophilic model compounds. J. Pharm. Sci. 72, 173–176. doi: 10.1002/jps.2600720219
Ren Y., Duhatschek D., Bartolomeu C. C., Erickson D., Giordano J. O. (2023). An automated system for cattle reproductive management under the IoT framework. Part I: The e-synch system and cow responses. Front. Anim. Sci 4, 1093851. doi: 10.3389/fanim.2023.1093851
Rial C., Laplacette A., Giordano J. O. (2022). Effect of a targeted reproductive management program designed to prioritize insemination at detected estrus and optimize time to insemination on the reproductive performance of lactating dairy cows. J. Dairy Sci. 105, 8411–8425. doi: 10.3168/jds.2022-22082
Souza A. H., Ayres H., Ferreira R. M., Wiltbank M. C. (2008). A new presynchronization system (Double-ovsynch) increases fertility at first postpartum timed AI in lactating dairy cows. Theriogenology 70, 208–215. doi: 10.1016/j.theriogenology.2008.03.014
Wijma R., Stangaferro M. L., Masello M., Elmetwally M. A., Granados G. E., Amovilli F., et al. (2017). Intravaginal instillation of gonadotropin-releasing hormone analogues with an absorption enhancer induced a surge of luteinizing hormone in lactating dairy cows. J. Dairy Sci. 100, 7626–7637. doi: 10.3168/jds.2016-12513
Wiltbank M. C., Baez G. M., Cochrane F., Barletta R. V., Trayford C. R., Joseph R. T. (2015). Effect of a second treatment with prostaglandin F2α during the ovsynch protocol on luteolysis and pregnancy in dairy cows. J. Dairy Sci. 98, 8644–8654. doi: 10.3168/jds.2015-9353
Keywords: automation, dairy cow, internet of things, synchronization of ovulation, gonadotropin releasing hormone (GnRH)
Citation: Ren Y, Duhatschek D, Bartolomeu CC, Laplacette AL, Perez MM, Rial C, Erickson D and Giordano JO (2023) An automated system for cattle reproductive management under the IoT framework. Part II: Induction of luteinizing hormone release after gonadotropin releasing hormone analogue delivery with e-Synch. Front. Anim. Sci. 4:1093857. doi: 10.3389/fanim.2023.1093857
Received: 09 November 2022; Accepted: 23 February 2023;
Published: 17 March 2023.
Edited by:
Guilherme J. M. Rosa, University of Wisconsin-Madison, United StatesReviewed by:
Joao Henrique Moreira Viana, Brazilian Agricultural Research Corporation (EMBRAPA), BrazilCopyright © 2023 Ren, Duhatschek, Bartolomeu, Laplacette, Perez, Rial, Erickson and Giordano. This is an open-access article distributed under the terms of the Creative Commons Attribution License (CC BY). The use, distribution or reproduction in other forums is permitted, provided the original author(s) and the copyright owner(s) are credited and that the original publication in this journal is cited, in accordance with accepted academic practice. No use, distribution or reproduction is permitted which does not comply with these terms.
*Correspondence: Julio O. Giordano, am9nMjVAY29ybmVsbC5lZHU=
Disclaimer: All claims expressed in this article are solely those of the authors and do not necessarily represent those of their affiliated organizations, or those of the publisher, the editors and the reviewers. Any product that may be evaluated in this article or claim that may be made by its manufacturer is not guaranteed or endorsed by the publisher.
Research integrity at Frontiers
Learn more about the work of our research integrity team to safeguard the quality of each article we publish.