- 1Division of Experimental Allergy and Immunodermatology, Faculty of Medicine and Health Sciences, Carl von Ossietzky University Oldenburg, Oldenburg, Germany
- 2Laboratory of Cellular Biology, Department of Biology, Institute of Biological Sciences (ICB), Federal University of Juiz de Fora, UFJF, Juiz de Fora, Brazil
- 3Division of Anatomy, Faculty of Medicine and Health Sciences, Carl von Ossietzky University Oldenburg, Oldenburg, Germany
- 4Department of Infection Biology, University Hospital Erlangen, Erlangen, Germany
- 5FAU Profile Center Immunomedicine (FAU I-MED), Friedrich-Alexander Universität Erlangen-Nürnberg, Erlangen, Germany
- 6Division of Allergy and Inflammation, Department of Medicine, Beth Israel Deaconess Medical Center and Harvard Medical School, Boston, MA, United States
- 7Research Center for Neurosensory Science, Carl von Ossietzky University Oldenburg, Oldenburg, Germany
- 8University Clinic of Dermatology and Allergy, Carl von Ossietzky University Oldenburg, Oldenburg, Germany
Eosinophil granulocytes, a specialized subset of white blood cells, have traditionally been associated with allergic responses and parasitic infections. However, recent research has unveiled their versatile roles in immune regulation beyond these classical functions. This review highlights the emerging field of eosinophil biology, with a particular focus on their release of extracellular vesicles (EVs) and extracellular DNA traps (EETs). It further explores potential implications of eosinophil-derived EVs and EETs for immune responses during inflammatory diseases. The release of EVs/EETs from eosinophils, which also affects the eosinophils themselves, may influence both local and systemic immune reactions, affecting the pathophysiology of conditions such as airway inflammation, chronic rhinosinusitis and atopic dermatitis.
1 Introduction
Eosinophil granulocytes are innate immune cells which compromise 2%–10% of all circulating leukocytes (1). They were first described by Paul Ehrlich in 1879 due to their bright color when stained with the acidic dye eosin (2, 3). Human eosinophils have a diameter of 12–15 µm, bilobed nuclei, and a lifespan of up to 18 h in the peripheral blood (4, 5). Once eosinophils have entered into tissues, they normally do not recirculate and have a life span of two to five days (6).
Even though eosinophils play an increasing role in allergic inflammation, they were originally considered to be a part of host defense against helminths and extracellular bacteria. When opposing these infections, eosinophils can release reactive oxygen species and toxic granule proteins such as major basic protein (MBP), eosinophil-derived neurotoxin (EDN), eosinophil peroxidase (EPX), and eosinophil cationic protein (ECP) (7). Further, extracellular DNA traps (ETs) are formed to bind and kill bacteria (7–12). Eosinophil extracellular traps (EETs) are not only associated with host defense but also with several inflammatory diseases and potentially contribute to disease persistence and progression (12, 13).
Eosinophils store and secrete a variety of inflammatory mediators such as interleukin (IL)-4, IL-5, IL-13, IL-31, neurotrophins like nerve growth factor (NGF), and brain-derived neurotrophic factor (BDNF) (10, 14–16). Furthermore, eosinophils can release different types of nanoscale membrane-bound structures, collectively termed extracellular vesicles (EVs), which have the ability to carry specific immune mediators, thus denoting the complexity of eosinophil secretory mechanisms. In human eosinophils, endosome-derived exosomes, plasma membrane-derived microvesicles/MVs, and secretory granule-derived eosinophil sombrero vesicles/EoSVs can act as EVs which are associated with inflammatory responses (17, 18). Besides eosinophils, other cells such as dendritic cells, macrophages, monocytes, neutrophils, and T cells also release EVs, contributing to inflammatory diseases such as asthma and atopic dermatitis (AD) (19, 20). This review aims to give an overview on eosinophil EVs and EETs and their contribution to inflammation.
2 Eosinophil sombrero vesicles in inflammation
Named as “microgranules” or “small granules” in the earlier eosinophil literature, EoSVs are large vesicular-tubular carriers, which are present in the cytoplasm of human eosinophils and involved in the transport of immune mediators (21–25). Due to their typical morphology, EoSVs are easily identified by transmission electron microscopy (TEM) (24, 25). EoSVs have a diameter of 150–300 nm (22) and were named due to their vesicular-tubular structure and their resemblance to a hat, although they can also take on an elongated or “C” shaped morphology (Figures 1A–C) (21, 26).
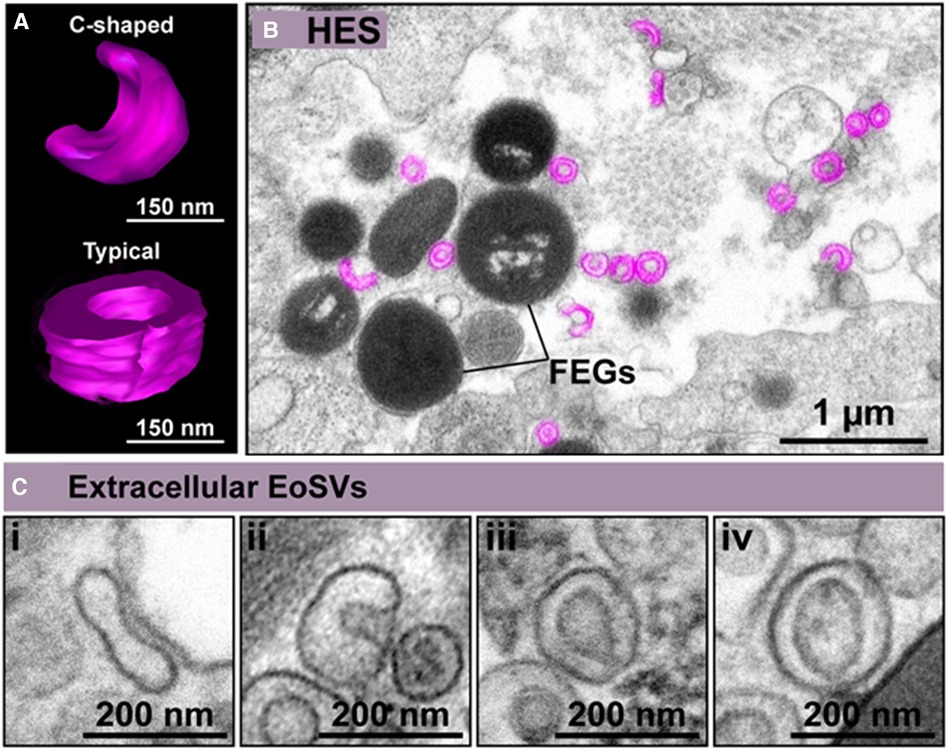
Figure 1. Extracellular eosinophil sombrero vesicles (eoSVs) are hallmarks of eosinophilic inflammation. (A) C-shaped and typical EoSVs are shown in three dimensions (3D) by electron tomography. (B) Representative electron micrograph of hypereosinophilic syndrome (HES) skin shows free EoSVs (colored in pink) in proximity to free extracellular granules (FEGs). (C) High magnification of extracellular tissue EoSVs with intact membranes and elongated, C-shaped, and typical morphologies. Reprinted from Neves et al., 2024 (26) under a Creative Commons Attribution 4.0 International (CC BY 4.0).
Remarkably, EoSVs are associated with eosinophil activation both in vivo and in vitro (27). Conventional TEM and 3D electron tomography studies have demonstrated that EoSVs are found around, and attached to secretory granules in the cytoplasm of activated eosinophils (21). This is because EoSVs can be formed from granules and are actively trafficking granule-derived products such as cytokines and cationic proteins (21). The total number of EoSVs increases when eosinophils are exposed to inflammatory stimuli. Furthermore, the number of EoSVs in contact with secretory granules also increases, thus influencing the release of the EoSV content into the extracellular medium (21). Moreover, in hypereosinophilic syndrome (HES) patients, who present with naturally activated eosinophils, the number of EoSVs is significantly higher than in healthy individuals (23).
EoSVs carry products from secretory granules to the cell surface, enabling the rapid release of specific mediators (21). This process of eosinophil secretion based on vesicular transport is termed piecemeal degranulation (PMD) and is frequently observed in vivo during numerous diseases (28). PMD is involved in the release of major basic protein (MBP) by EoSVs, which can be observed after stimulating eosinophils with C-C motif chemokine ligand 11 (CCL11). Moreover, it has been reported that the Th1 cytokine tumor necrosis factor (TNF-α) induces the release of granule contents through compound exocytosis (29). Both CCL11 and TNF-α lead to the increase of the number of EoSVs in the cytoplasm of eosinophils. Another experiment showed that these cell-activating stimuli also cause a threefold amplification of EoSVs that carry interferon-γ (IFNγ) (29). IFNγ-positive EoSVs are mostly found in the peripheral cytoplasm rather than in the adjacent cytoplasmic area deeper in the cell, suggesting a fast response to inflammatory stimuli (29). Melo et al. also demonstrated that both EoSVs and small spherical vesicles transport IL-4, a cytokine that plays a major role in atopic diseases, from specific granules to the plasma membrane upon CCL11 stimulation (22). Furthermore, it has been suggested that in human eosinophils, transferrin receptor-positive (TfnRc) recycling endosomes play an important role in trafficking the Th2 cytokines IL-9 and IL-13 to the plasma membrane for subsequent release (30).
Interestingly, cell-free EoSVs (delimited by their bilayer membranes) have also been identified in inflamed tissues of different target organs involved in eosinophilic diseases such ulcerative colitis (13, 26), eosinophilic chronic rhinosinusitis (ECRS) (13, 26), HES (Figure 1B) (26), dermatitis (26), schistosomiasis mansoni (26), and eosinophilic esophagitis (31). Intact free EoSVs are released as a result of eosinophil cytolytic degranulation and remain in the extracellular matrix in conjunction with free extracellular granules (FEGs) even after complete cell disintegration except for granules (26). For eosinophils, cytolysis including cytolysis with the release of nucleus-derived EETs (EETosis), is a form of eosinophil degranulation commonly observed in vivo (26, 32, 33). Extracellular EoSVs are mostly observed close to FEGs, but also in the proximity of EETs (26). Increased evidence suggests that the release of EETosis-generated EETs plays a crucial role in the pathophysiology of inflammatory diseases, with the resulting release of both intact FEGs and EoSVs being involved in eosinophil responses (26).
3 Eosinophil-derived microvesicles and exosomes in inflammation
Eosinophils release high numbers of MVs upon stimulation with inflammatory stimuli as shown in a study by Akuthota et al. (17). When eosinophils were exposed to CCL11 or TNF-α, the release of MVs was significantly increased when compared to unstimulated eosinophils. MVs, which by definition are EVs directly formed from the plasma membrane, were captured in situ and quantitated with the application of TEM, which unambiguously enables visualization of EVs at the cell surface (17, 18). The authors demonstrated that the type of stimulus modulates both the production and size of MVs. TNF-α resulted in greater secretion of MVs compared to CCL11, and, on average, these TNF-α-induced EVs had smaller diameters, which possibly reflects an effect of faster EV production on membrane dynamics (12). Interestingly, CD9 proved to be a better marker for eosinophil-derived MVs than CD63. This was revealed by both immunogold transmission electron microscopy (TEM) and nanoscale flow cytometry. This is in accordance with the fact that the surface of human eosinophils constitutively express high levels of CD9 (34).
Exosomes have been detected in eosinophils from patients with asthma as shown in study by Canas et al. (35). In their study, the number of exosomes from eosinophils in peripheral blood was found to be significantly increased in the asthma group when compared to healthy controls (35). Moreover, common proteins in eosinophil exosomes such as ECP, EPX, and MBP, were analyzed for both experimental groups, as well as proteins related to migration, inflammation, or adhesion. For this, eosinophils from peripheral blood were cultured with exosomes and their migration and adhesion was investigated. Eosinophils from asthma patients exhibited increased migration activity compared to eosinophils without exosome stimuli (35). Interestingly, enhanced adhesion of eosinophils was observed only after stimulation with exosomes from eosinophils of asthmatics, but not with those from healthy controls. Particularly intercellular adhesion molecule 1 (ICAM-1) and integrin α2 were responsible for adhesion, as their expression was increased in the presence of exosomes (35). Furthermore, exosomes of eosinophils were shown to induce the release of reactive oxygen species (ROS) from other eosinophils as a paracrine effect. Moreover, it was observed that eosinophils absorb exosomes from other eosinophils, which has been suggested to be an energy-dependent process (35). Interestingly, eosinophil-derived exosomes from atopic and non-atopic asthmatics also have effects on other cell types. Small airway epithelial cells showed higher apoptosis rates after stimulation with eosinophil-derived exosomes from asthma patients, but not after stimulation with exosomes from healthy controls (36). The capacity of epithelial cell monolayers to repair wounds was also impaired after exposure to asthmatic exosomes (36). Lässer et al. demonstrated that EVs can be isolated directly from lung tissue. In a model of eosinophilic airway inflammation mice were sensitized and then challenged with ovalbumin (OVA) to investigate proteins from EVs in lung tissue (37). The total numbers of eosinophils in bronchoalveolar lavage fluid (BALF), EVs, and the amount of proteins were significantly increased after sensitized mice were challenged intranasally with OVA compared to those that were treated with phosphate buffered saline (PBS) (37). The enriched proteins were mostly associated with B cell signaling pathways, innate immune responses, or immune system processes such as matrix metalloproteinase (MMP-12), pendrin, arachidonate 15-lipoxygenase (ALOX15), C-C chemokine receptor type 3 (CCR3/CD193), EPX, and eosinophil cationic protein (ECP) (37). These findings suggest that eosinophils play a crucial role in airway inflammation through the release of EVs. The association of eosinophils with Th2-driven immune responses and atopy also supports the hypothesis of eosinophil derived vesicle contribution to inflammation in other atopic disorders such as atopic dermatitis.
4 Eosinophil extracellular DNA traps in airway inflammation
As noted, eosinophils are able to release not only different subtypes of EVs but also EETs both in vitro (Figure 2) and in vivo. ETs are not only formed by eosinophils, but also by neutrophils, the so-called neutrophil extracellular traps (NETs) and other innate immune cells such as macrophages/monocytes, mast cells, basophils, and dendritic cells (38). EETs consist of DNA fibers which are embedded with granule proteins such as MBP and ECP (12), or associated with intact FEGs (23) and EoSVs (15). The release of EETs has been described from both living eosinophils and those undergoing cell lysis (EETosis), which is dependent on nicotinamide adenine dinucleotide phosphate (NADPH) oxidase activity. The process of EET release has been suggested to be influenced by external stimuli, such as Staphylococcus aureus, and also depends on the time of exposure (12). Moreover, as described above, EETosis drives the release of EETs in tissues and the secretion during several inflammatory diseases (13). The formation of EETs is associated with the formation of Charcot-Leyden crystals which are composed of the protein galectin-10, a biomarker of eosinophil involvement in asthma, allergic rhinitis, and other eosinophilic inflammation (39, 13). A recent paper focusing on inflammatory eosinophilic diseases identified that early nuclear signs of EETosis appear, before the formation of nucleus-extruded EETs. This can be helpful to detect eosinophils entering in a program of EETosis in vivo (13).
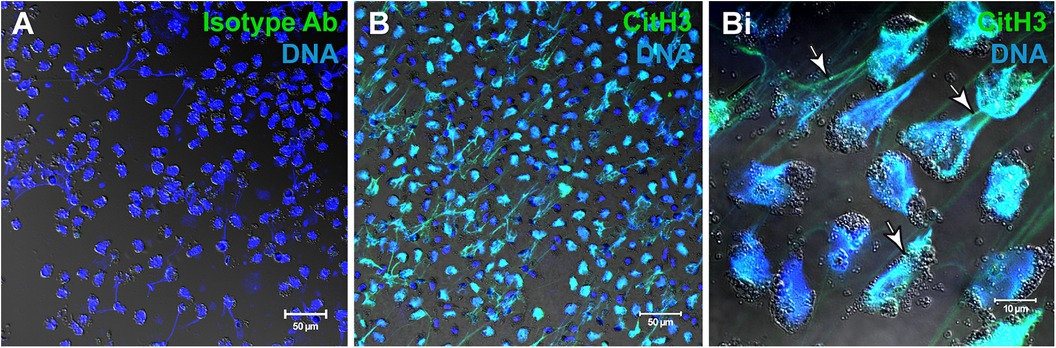
Figure 2. Human eosinophils undergo EETosis in response to stimulation with agonists. (A,B) Immunofluorescence for isotype (A) or anti-citrullinated H3 histone (CitH3) antibodies. Eosinophil extracellular traps (EETs) appear as filamentous chromatin structures immunolabeled for CitH3 (green) and DNA (Hoechst, blue). (Bi) Merged images of CitH3 +DNA-stained eosinophils in higher magnification. Arrows indicate EETs. Differential interference contrast (DIC) images were obtained by confocal microscopy. Purified eosinophils were stimulated with platelet activating factor (PAF) + IL-5. Ab, antibody. Images were reprinted from Neves et al., 2022 (13) under a Creative Commons Attribution 4.0 International (CC BY 4.0) license.
EETs are known to play a pivotal role in severe asthma. Choi et al. demonstrated that patients presenting with severe asthma have elevated EET+ eosinophil counts in comparison to those with non-severe asthma or healthy controls (40). EET formation can be induced by eosinophil activation through IL-5 and lipopolysaccharides (LPS) (40), but also through thymic stromal lymphopoietin (TSLP) (41). It has however also been shown that EETs have an autocrine effect, as they induce cell activation and the release of further EETs from eosinophils (42). The formation of EETs is dependent on adhesion (41) and ROS, as demonstrated by the fact that ROS production from peripheral blood eosinophils is inhibited by N-acetyl-L-cysteine and diphenylene iodonium chloride (41, 42). This is supported by the positive correlation of EET formation and ROS production (42). Furthermore, it has been shown that the number of EET+ eosinophils correlates with the number of type 2 innate lymphoid cells (ILC2) in severe asthma, which is also in accordance with elevated levels of IL-13, IL-33, and TSLP plasma levels (40). These findings have been confirmed by mouse experiments in which intranasally injected fluorescence-labeled EETs were found to accumulate in lung tissue. The EET treatment caused significantly increased numbers of eosinophils and neutrophils in BALF, as well as increased epithelial thickness and higher levels of infiltration into the epithelium (40). Moreover, the BALF contained elevated levels of the epithelium-derived cytokines IL1α, IL-1β, IL-33, TSLP, and the chemokine C-X-C motif ligand 1 (CXCL-1), and CCL24. Further, the percentage of IL-5 and IL-13 releasing ILC2s was increased in EET treated mice (40). These results suggest that in severe asthma, type 2 immune responses are induced through EETs.
5 EETs are associated with S. aureus colonization in chronic rhinosinusitis
The role of EETs has also been investigated in chronic rhinosinusitis with nasal polyps (43). The presence of EET releasing eosinophils was observed in the subepithelial regions of the nasal mucosa in patients but not in healthy controls. The eosinophils were mostly found in clusters with other eosinophils, rather than being present as single EET-releasing cells (43). Interestingly, the percentages of EET-releasing eosinophils were associated with higher rates of S. aureus colonization which is known to be linked to increased IL-5 levels (43, 44). Furthermore, it has been demonstrated that in vitro exposure of tissue fragments from patients with chronic rhinosinusitis to S. aureus, increased the formation of EETs in eosinophils in the subepithelial regions of nasal mucosa (43). Eosinophils further migrate to sites of epithelial defects and trap S. aureus as shown in an ex vivo model of diseased human mucosal tissue. EET formation was even enhanced when isolated eosinophils from nasal secretions were stimulated with S. aureus (43). The release of EETs in response to S. aureus is also dependent on ROS production (43). Myiabe et al. impressively demonstrated the direct role of EETs in ECRS and their therapeutic potential. They showed that the presence of EETs is linked to disease pathophysiology (45). The mucus of patients was found to be highly viscous due to the presence of aggregated cells with accumulated ETs and cell debris. The treatment with EET-degrading DNase I and Heparin caused a significant decrease in mucus viscosity which is associated with a better quality of life (45).
6 EET link to S. aureus colonization indicates EET contribution to skin inflammation
The link between the release of EETs and S. aureus further suggests a possible role of eosinophil traps in inflamed skin such as in AD. Even though EETs could not be detected in tissue samples of AD skin in first experiments, they have been found in skin diseases related to exogenous triggers such as allergic contact dermatitis, drug hypersensitivity, and also after atopy patch tests which resemble the acute exacerbation observed in AD (46). Exposure to S. aureus induces itch which in turn incites scratching behavior and resulting epicutaneous skin damage, and is known to promote skin inflammation that correlates with AD severity (47–49). It has been demonstrated that IL-5 gene expression is increased in ILC2s and that IL-5 protein levels are elevated after stimulation with S. aureus lysate in nasal tissue of patients with chronic rhinosinusitis (50). These findings lead to the hypothesis that in inflamed skin, elevated IL-5 levels as a result of S. aureus colonization, induces the release of EETs from eosinophils. This assumption is supported by increased IL-5 expression in skin, and higher IL-5 serum levels in AD patients (51, 52). Furthermore, IL-5 was found to be expressed under conditions of EET formation as found in allergic contact dermatitis (46). Interestingly, NETs are released in the skin in response to S. aureus and have been observed to enhance colonization of this pathogen on human and mouse skin by inducing oxidative stress and the expression of the high-mobility-group-protein B1 (HMGB1) from keratinocytes (53). HMGB1 in turn promotes skin barrier dysfunctions by downregulating epidermal barrier genes, further promoting the spread of S. aureus (53). The microRNA-223 has been further reported to be reduced in AD patients, enhancing the release of NETs from neutrophils in AD (54). All these findings suggest that EETs might have a similar role to NETs in skin inflammation such as AD.
7 EVs and EETs in autoimmune disease bullous pemphigoid
EETs have further been found in skin biopsies of patients with the autoimmune blistering disease bullous pemphigoid (BP) (46) in which EVs also play a pivotal role. EVs have been reported to carry tissue-specific autoantigens in autoimmune diseases (55, 10). In a recent pilot study, BP180, a hemidesmosomal protein and the most important autoantigen in BP, was found in EVs of blister fluid in 50% of all BP patients (56). Furthermore, eosinophils have been described to be the major cellular component of BP blister fluid (57). Blister fluid exosomes from BP patients have been reported to induce the recruitment of neutrophils through IL-8 (58). It has been further hypothesized that keratinocytes and skin infiltrating granulocytes such as neutrophils, are a possible source of exosomes in BP blister fluids (58, 10). As enhanced release of TNF-α from keratinocytes has been observed after stimulation with blister fluid derived exosomes (58), it is possible that TNF-α in turn initiates the release of EoSVs from eosinophils, contributing to the pathogenesis of BP.
8 Final remarks and questions for the future
In summary, increasing evidence has been demonstrating that eosinophil EVs and EETs play a pivotal role in inflammation during a variety of diseases associated with eosinophilia, including in the upper and lower airways and the skin. However, the question arises if there is there also a marker for eosinophilic diseases as a result of EET release. A promising candidate is Galectin-10, as it is released in large amounts by EETosis rather than the secretory system (59). Furthermore, the role of ETs in mucus and tissues of patients with eosinophilic diseases poses another question that has not yet been answered. Further research is required to answer these questions and to gain a comprehensive understanding of EETs and EVs in eosinophilic inflammatory diseases, which may lead to novel and therapeutic treatment approaches in the future.
Author contributions
TW: Visualization, Writing – original draft. RM: Visualization, Writing – review & editing. NG: Writing – review & editing. DV: Writing – review & editing. PW: Writing – review & editing. UR: Conceptualization, Writing – review & editing.
Funding
The author(s) declare financial support was received for the research, authorship, and/or publication of this article.
This work was funded by the German Research Foundation DFG (FOR2690-PruSEARCH Translational Pruritus Research, grant RA-1026/3-2) to UR, the Conselho Nacional de Desenvolvimento Científico e Tecnológico (CNPq, Brazil, grants 307270/2022-0 and 409301/2023-0), and Fundação de Amparo a Pesquisa do Estado de Minas Gerais (FAPEMIG, Brazil, grants APQ-02930-21 and APQ-00754-23) to RM.
Conflict of interest
The authors declare that the research was conducted in the absence of any commercial or financial relationships that could be construed as a potential conflict of interest.
The author(s) declared that they were an editorial board member of Frontiers, at the time of submission. This had no impact on the peer review process and the final decision.
Publisher's note
All claims expressed in this article are solely those of the authors and do not necessarily represent those of their affiliated organizations, or those of the publisher, the editors and the reviewers. Any product that may be evaluated in this article, or claim that may be made by its manufacturer, is not guaranteed or endorsed by the publisher.
References
1. Raap U, Wardlaw AJ. A new paradigm of eosinophil granulocytes: neuroimmune interactions. Exp Dermatol. (2008) 17(9):731–8. doi: 10.1111/j.1600-0625.2008.00741.x
2. Blanchard C, Rothenberg ME. Biology of the eosinophil. Adv Immunol. (2009) 101:81–121. doi: 10.1016/S0065-2776(08)01003-1
3. Ehrlich P. Ueber die spezifischen granulationen des blutes. Arch Anat Physiol Physiol Abt. (1879):571–9.
4. Dorosz A, Grosicki M, Dybas J, Matuszyk E, Rodewald M, Meyer T, et al. Eosinophils and neutrophils-molecular differences revealed by spontaneous Raman, CARS and fluorescence microscopy. Cells. (2020) 9(9):2041. doi: 10.3390/cells9092041
5. Melo RCN. Eosinophil Ultrastructure: Atlas of Eosinophil Cell Biology and Pathology. San Diego: Elsevier Science & Technology (2022).
6. Park YM, Bochner BS. Eosinophil survival and apoptosis in health and disease. Allergy Asthma Immunol Res. (2010) 2(2):87–101. doi: 10.4168/aair.2010.2.2.87
7. Weller PF. The immunobiology of eosinophils. N Engl J Med. (1991) 324(16):1110–8. doi: 10.1056/NEJM199104183241607
8. Radonjic-Hoesli S, Brüggen M-C, Feldmeyer L, Simon H-U, Simon D. Eosinophils in skin diseases. Semin Immunopathol. (2021) 43(3):393–409. doi: 10.1007/s00281-021-00868-7
9. Yousefi S, Gold JA, Andina N, Lee JJ, Kelly AM, Kozlowski E, et al. Catapult-like release of mitochondrial DNA by eosinophils contributes to antibacterial defense. Nat Med. (2008) 14(9):949–53. doi: 10.1038/nm.1855
10. Limberg MM, Weihrauch T, Gray N, Ernst N, Hartmann K, Raap U. Eosinophils, basophils, and neutrophils in bullous pemphigoid. Biomolecules. (2023) 13(7):1019. doi: 10.3390/biom13071019
11. Ueki S, Konno Y, Takeda M, Moritoki Y, Hirokawa M, Matsuwaki Y, et al. Eosinophil extracellular trap cell death-derived DNA traps: their presence in secretions and functional attributes. J Allergy Clin Immunol. (2016) 137(1):258–67. doi: 10.1016/j.jaci.2015.04.041
12. Mukherjee M, Lacy P, Ueki S. Eosinophil extracellular traps and inflammatory pathologies-untangling the web! Front Immunol. (2018) 9:2763. doi: 10.3389/fimmu.2018.02763
13. Neves VH, Palazzi C, Bonjour K, Ueki S, Weller PF, Melo RCN. in vivo ETosis of human eosinophils: the ultrastructural signature captured by TEM in eosinophilic diseases. Front Immunol. (2022) 13:938691. doi: 10.3389/fimmu.2022.938691
14. Weihrauch T, Limberg MM, Gray N, Schmelz M, Raap U. Neurotrophins: neuroimmune interactions in human atopic diseases. Int J Mol Sci. (2023) 24(7):6105. doi: 10.3390/ijms24076105
15. Kunsleben N, Rüdrich U, Gehring M, Novak N, Kapp A, Raap U. IL-31 induces chemotaxis, calcium mobilization, release of reactive oxygen species, and CCL26 in eosinophils, which are capable to release IL-31. J Invest Dermatol. (2015) 135(7):1908–11. doi: 10.1038/jid.2015.106
16. Spencer LA, Bonjour K, Melo RCN, Weller PF. Eosinophil secretion of granule-derived cytokines. Front Immunol. (2014) 5:496. doi: 10.3389/fimmu.2014.00496
17. Akuthota P, Carmo LAS, Bonjour K, Murphy RO, Silva TP, Gamalier JP, et al. Extracellular microvesicle production by human eosinophils activated by “inflammatory” stimuli. Front Cell Dev Biol. (2016) 4:117. doi: 10.3389/fcell.2016.00117
18. Melo RCN, Silva TP. Eosinophil activation during immune responses: an ultrastructural view with an emphasis on viral diseases. J Leukoc Biol. (2024) 116(2):321–34. doi: 10.1093/jleuko/qiae058
19. Alashkar Alhamwe B, Potaczek DP, Miethe S, Alhamdan F, Hintz L, Magomedov A, et al. Extracellular vesicles and asthma-more than just a co-existence. Int J Mol Sci. (2021) 22(9):4984. doi: 10.3390/ijms22094984
20. Grueso-Navarro E, Navarro P, Laserna-Mendieta EJ, Lucendo AJ, Arias-González L. Blood-based biomarkers for eosinophilic esophagitis and concomitant atopic diseases: a look into the potential of extracellular vesicles. Int J Mol Sci. (2023) 24(4):3669. doi: 10.3390/ijms24043669
21. Melo RCN, Weller PF. Vesicular trafficking of immune mediators in human eosinophils revealed by immunoelectron microscopy. Exp Cell Res. (2016) 347(2):385–90. doi: 10.1016/j.yexcr.2016.08.016
22. Melo RCN, Spencer LA, Perez SAC, Ghiran I, Dvorak AM, Weller PF. Human eosinophils secrete preformed, granule-stored interleukin-4 through distinct vesicular compartments. Traffic. (2005) 6(11):1047–57. doi: 10.1111/j.1600-0854.2005.00344.x
23. Melo RCN, Spencer LA, Perez SAC, Neves JS, Bafford SP, Morgan ES, et al. Vesicle-mediated secretion of human eosinophil granule-derived major basic protein. Lab Invest. (2009) 89(7):769–81. doi: 10.1038/labinvest.2009.40
24. Melo RCN, Spencer LA, Dvorak AM, Weller PF. Mechanisms of eosinophil secretion: large vesiculotubular carriers mediate transport and release of granule-derived cytokines and other proteins. J Leukoc Biol. (2008) 83(2):229–36. doi: 10.1189/jlb.0707503
25. Melo RCN, Weller PF. Contemporary understanding of the secretory granules in human eosinophils. J Leukoc Biol. (2018) 104(1):85–93. doi: 10.1002/JLB.3MR1217-476R
26. Neves VH, Palazzi C, Malta KK, Bonjour K, Kneip F, Dias FF, et al. Extracellular sombrero vesicles are hallmarks of eosinophilic cytolytic degranulation in tissue sites of human diseases. J Leukoc Biol. (2024) 116(2):398–408. doi: 10.1093/jleuko/qiae079
27. Melo RC, Dvorak AM, Weller PF. Contributions of electron microscopy to understand secretion of immune mediators by human eosinophils. Microsc Microanal. (2010) 16(6):653–60. doi: 10.1017/S1431927610093864
28. Melo RC, Weller PF. Piecemeal degranulation in human eosinophils: a distinct secretion mechanism underlying inflammatory responses. Histol Histopathol. (2010) 25(10):1341–54. doi: 10.14670/HH-25.1341
29. Carmo LAS, Bonjour K, Spencer LA, Weller PF, Melo RCN. Single-cell analyses of human eosinophils at high resolution to understand compartmentalization and vesicular trafficking of interferon-gamma. Front Immunol. (2018) 9:1542. doi: 10.3389/fimmu.2018.01542
30. Almas S, Fayad N, Srivastava O, Siddique M, Touret N, Lacy P. Cytokine trafficking of IL-9 and IL-13 through TfnRc+ vesicles in activated human eosinophils. J Leukoc Biol. (2021) 109(4):753–62. doi: 10.1002/JLB.2MA0820-320RR
31. Saffari H, Hoffman LH, Peterson KA, Fang JC, Leiferman KM, Pease LF, et al. Electron microscopy elucidates eosinophil degranulation patterns in patients with eosinophilic esophagitis. J Allergy Clin Immunol. (2014) 133(6):1728–34.e1. doi: 10.1016/j.jaci.2013.11.024
32. Weller PF, Spencer LA. Functions of tissue-resident eosinophils. Nat Rev Immunol. (2017) 17(12):746–60. doi: 10.1038/nri.2017.95
33. Fukuchi M, Kamide Y, Ueki S, Miyabe Y, Konno Y, Oka N, et al. Eosinophil ETosis-mediated release of galectin-10 in eosinophilic granulomatosis with polyangiitis. Arthritis Rheumatol. (2021) 73(9):1683–93. doi: 10.1002/art.41727
34. Akuthota P, Melo RCN, Spencer LA, Weller PF. MHC Class II and CD9 in human eosinophils localize to detergent-resistant membrane microdomains. Am J Respir Cell Mol Biol. (2012) 46(2):188–95. doi: 10.1165/rcmb.2010-0335OC
35. Cañas JA, Sastre B, Mazzeo C, Fernández-Nieto M, Rodrigo-Muñoz JM, González-Guerra A, et al. Exosomes from eosinophils autoregulate and promote eosinophil functions. J Leukoc Biol. (2017) 101(5):1191–9. doi: 10.1189/jlb.3AB0516-233RR
36. Cañas JA, Sastre B, Rodrigo-Muñoz JM, Fernández-Nieto M, Barranco P, Quirce S, et al. Eosinophil-derived exosomes contribute to asthma remodelling by activating structural lung cells. Clin Exp Allergy. (2018) 48(9):1173–85. doi: 10.1111/cea.13122
37. Lässer C, Kishino Y, Park K, Shelke GV, Karimi N, Suzuki S, et al. Immune-associated proteins are enriched in lung tissue-derived extracellular vesicles during allergen-induced eosinophilic airway inflammation. Int J Mol Sci. (2021) 22(9):4718. doi: 10.3390/ijms22094718
38. Huang SU-S, O’Sullivan KM. The expanding role of extracellular traps in inflammation and autoimmunity: the new players in casting dark webs. Int J Mol Sci. (2022) 23(7):3793. doi: 10.3390/ijms23073793
39. Ueki S, Tokunaga T, Melo RCN, Saito H, Honda K, Fukuchi M, et al. Charcot-Leyden crystal formation is closely associated with eosinophil extracellular trap cell death. Blood. (2018) 132(20):2183–7. doi: 10.1182/blood-2018-04-842260
40. Choi Y, Kim Y-M, Lee H-R, Mun J, Sim S, Lee D-H, et al. Eosinophil extracellular traps activate type 2 innate lymphoid cells through stimulating airway epithelium in severe asthma. Allergy. (2020) 75(1):95–103. doi: 10.1111/all.13997
41. Morshed M, Yousefi S, Stöckle C, Simon H-U, Simon D. Thymic stromal lymphopoietin stimulates the formation of eosinophil extracellular traps. Allergy. (2012) 67(9):1127–37. doi: 10.1111/j.1398-9995.2012.02868.x
42. Choi Y, Le Pham D, Lee D-H, Lee S-H, Kim S-H, Park H-S. Biological function of eosinophil extracellular traps in patients with severe eosinophilic asthma. Exp Mol Med. (2018) 50(8):1–8. doi: 10.1038/s12276-018-0136-8
43. Gevaert E, Zhang N, Krysko O, Lan F, Holtappels G, de Ruyck N, et al. Extracellular eosinophilic traps in association with Staphylococcus aureus at the site of epithelial barrier defects in patients with severe airway inflammation. J Allergy Clin Immunol. (2017) 139(6):1849–1860.e6. doi: 10.1016/j.jaci.2017.01.019
44. van Zele T, Gevaert P, Watelet J-B, Claeys G, Holtappels G, Claeys C, et al. Staphylococcus aureus colonization and IgE antibody formation to enterotoxins is increased in nasal polyposis. J Allergy Clin Immunol. (2004) 114(4):981–3. doi: 10.1016/j.jaci.2004.07.013
45. Miyabe Y, Fukuchi M, Tomizawa H, Nakamura Y, Jikei M, Matsuwaki Y, et al. Aggregated eosinophils and neutrophils characterize the properties of mucus in chronic rhinosinusitis. J Allergy Clin Immunol. (2024) 153(5):1306–18. doi: 10.1016/j.jaci.2023.11.925
46. Simon D, Hoesli S, Roth N, Staedler S, Yousefi S, Simon H-U. Eosinophil extracellular DNA traps in skin diseases. J Allergy Clin Immunol. (2011) 127(1):194–9. doi: 10.1016/j.jaci.2010.11.002
47. Leung AD, Schiltz AM, Hall CF, Liu AH. Severe atopic dermatitis is associated with a high burden of environmental Staphylococcus aureus. Clin Exp Allergy. (2008) 38(5):789–93. doi: 10.1111/j.1365-2222.2008.02964.x
48. Huang JT, Abrams M, Tlougan B, Rademaker A, Paller AS. Treatment of Staphylococcus aureus colonization in atopic dermatitis decreases disease severity. Pediatrics. (2009) 123(5):e808–14. doi: 10.1542/peds.2008-2217
49. Deng L, Costa F, Blake KJ, Choi S, Chandrabalan A, Yousuf MS, et al. S. aureus drives itch and scratch-induced skin damage through a V8 protease-PAR1 axis. Cell. (2023) 186(24):5375–5393.e25. doi: 10.1016/j.cell.2023.10.019
50. Du K, Zhao Y, Zhang X, Li C, Hao Y, Du X, et al. Staphylococcus aureus lysate induces an IgE response via memory B cells in nasal polyps. J Allergy Clin Immunol. (2024) 153(3):718–31.e11. doi: 10.1016/j.jaci.2023.10.033
51. Çetinarslan T, Kümper L, Fölster-Holst R. The immunological and structural epidermal barrier dysfunction and skin microbiome in atopic dermatitis-an update. Front Mol Biosci. (2023) 10:1159404. doi: 10.3389/fmolb.2023.1159404
52. Kondo S, Yazawa H, Jimbow K. Reduction of serum interleukin-5 levels reflect clinical improvement in patients with atopic dermatitis. J Dermatol. (2001) 28(5):237–43. doi: 10.1111/j.1346-8138.2001.tb00124.x
53. Focken J, Scheurer J, Jäger A, Schürch CM, Kämereit S, Riel S, et al. Neutrophil extracellular traps enhance S. aureus skin colonization by oxidative stress induction and downregulation of epidermal barrier genes. Cell Rep. (2023) 42(10):113148. doi: 10.1016/j.celrep.2023.113148
54. Liao T-L, Lin C-C, Chen Y-H, Tang K-T. Reduced miR-223 increases blood neutrophil extracellular trap and promotes skin inflammation in atopic dermatitis. Allergy. (2023) 78(12):3252–4. doi: 10.1111/all.15794
55. Lu M, DiBernardo E, Parks E, Fox H, Zheng S-Y, Wayne E. The role of extracellular vesicles in the pathogenesis and treatment of autoimmune disorders. Front. Immunol. (2021) 12:566299. doi: 10.3389/fimmu.2021.566299
56. Gasparini G, Tasso R, Palamà MEF, Ciferri MC, Gentili C, Di Zenzo G, et al. Pilot study investigating BP-180 in extracellular vesicles derived from blister fluid of bullous pemphigoid patients. Arch Dermatol Res. (2023) 315(6):1837–41. doi: 10.1007/s00403-023-02560-2
57. Rüdrich U, Gehring M, Papakonstantinou E, Illerhaus A, Engmann J, Kapp A, et al. Eosinophils are a major source of interleukin-31 in bullous pemphigoid. Acta Derm Venereol. (2018) 98(8):766–71. doi: 10.2340/00015555-2951
58. Fang H, Shao S, Jiang M, Dang E, Shen S, Zhang J, et al. Proinflammatory role of blister fluid-derived exosomes in bullous pemphigoid. J Pathol. (2018) 245(1):114–25. doi: 10.1002/path.5061
Keywords: extracellular vesicles, eosinophil activation, sombrero vesicles, EETosis, inflammation, eosinophilic diseases
Citation: Weihrauch T, Melo RCN, Gray N, Voehringer D, Weller PF and Raap U (2024) Eosinophil extracellular vesicles and DNA traps in allergic inflammation. Front. Allergy 5: 1448007. doi: 10.3389/falgy.2024.1448007
Received: 12 June 2024; Accepted: 23 July 2024;
Published: 1 August 2024.
Edited by:
Andaç Salman, Acıbadem Healthcare Group Altunizade Hospital, TürkiyeReviewed by:
Daniel P. Potaczek, University of Marburg, Germany© 2024 Weihrauch, Melo, Gray, Voehringer, Weller and Raap. This is an open-access article distributed under the terms of the Creative Commons Attribution License (CC BY). The use, distribution or reproduction in other forums is permitted, provided the original author(s) and the copyright owner(s) are credited and that the original publication in this journal is cited, in accordance with accepted academic practice. No use, distribution or reproduction is permitted which does not comply with these terms.
*Correspondence: Ulrike Raap, cmFhcC51bHJpa2VAa2xpbmlrdW0tb2xkZW5idXJnLmRl