- 1Department of Emergency Medicine, Camden Clark Medical Center, West Virginia University, Parkersburg, WV, United States
- 2Department of Pathology, Feinberg School of Medicine, Northwestern University, Chicago, IL, United States
Allergy and its manifestations were first appreciated in the 1870 s. Today, the mechanism by which specific substances elicit allergic reactions remains poorly understood. This is problematic from a healthcare perspective because the prevalence of allergic disease and its societal costs are substantial. Regarding mechanistic understanding of allergy, a new proposal, The Acari Hypothesis, has been forwarded. The Hypothesis, borne from consideration of alpha-gal syndrome, postulates that acarians, i.e., mites and ticks, are operative agents of allergy. By way of their pathogenic payloads and salivary pattern recognition receptor(s), acarians potentiate in human hosts the generation of IgE against acarian dietary elements. Those elements account for most, if not all, known human allergens. Inasmuch as acarian—human interactions occur on human epithelial surfaces, it is to be expected factors that influence the presence and/or operation of acarians on those surfaces influence the expression of allergic diseases. In this report, it is proposed that two adaptations of catarrhine primates, i.e., Old World monkeys, apes and humans, evolved to deter acarian species: firstly, the expansion of eccrine glands across the entirety of body surface area, and, secondly, the secretion of sweat by those glands. Contemporary hygienic practices that reduce and/or disrupt the operation of eccrine glands are likely responsible for the increase in allergic disease seen today.
1 Introduction
“The Acari Hypothesis” is a multi-installment treatise that accounts for the cardinal pathophysiologic and epidemiologic features of IgE-mediated allergic disease. It presupposes that mites and ticks are the causative agents of allergy. The first installment of The Hypothesis makes the case that most allergens are elements of acarian diets (1). The second installment provides how such dietary elements, when transmitted to a human, elicit IgE (2). The third installment relates the first two to atopic dermatitis, the prototypical allergic disease (3). This installment of The Hypothesis, the fourth, provides rationale for the ongoing allergy epidemic. Importantly, IgE-mediated diseases have increased precipitously since 1870 (4), especially within developed countries. To date, no persuasive rationale for this increase exists. In the context of The Hypothesis, however, a plausible explanation is evident; namely, encounters between acarians and humans have increased.
Just as the prevalence of allergic diseases is increasing, so, too, is the prevalence of tick-borne illnesses, examples of which include galactose-α-1,3-galactose (α-gal) hypersensitivity and Lyme disease (5–12). Although the increasing prevalence of tick-borne illnesses has been attributed to climate change and deforestation (13, 14), these factors cannot account for the rise in IgE-mediated diseases because synanthropic mites thrive in the climate-controlled human habitats of the developed world, where allergies are especially prevalent.
According to The Hypothesis, the induction of an allergic disease requires that causative acarians be present on human epithelium (1–3). In keeping with α-gal sensitization, the number of infesting organisms need not be great (15–17). Given the phylogenetic relatedness of mites and ticks, it is reasonable to assume that a change to the epithelial environment would influence the prevalence of all acarian-induced diseases.
A review of primate species and the acarian parasites that infest them supports a role for the eccrine glandular system in the anti-acarian defense of humans. Homo sapiens are subject to permanent parasitism by two lineages of acarians, Demodex spp., which inhabit pilosebaceous units and subsist on sebum, and Sarcoptes scabiei, a carnivorous mite that burrows beneath skin (18, 19). Other primate species are subject to permanent parasitism by a third acarian lineage, Psoroptidae, a family of non-burrowing carnivorous mites (20).
Modern dwellings of Homo sapiens are infested by Pyroglyphidae, a family of polyphagous acarians whose diet includes, among other things, discarded epidermal materials (21, 22). The dust mites, Dermatophagoides pteronyssinus and Dermatophagoides farinae, belong to Pyroglyphidae. Materials expressed by them are intimately associated with allergic disease (23). Phylogenetic analysis suggests that Pyroglyphidae is a sister taxon to Psoroptidae, and that both families belong within Psoroptidia, a parvorder of mites, the representatives of which are almost exclusively parasitic (24). At some time in the past, Pyroglyphidae diverged from its sister taxon and followed an exceedingly unusual evolutionary pathway from parasite to free-living scavenger (24). Consequently, Pyroglyphidae consume fungi and bacteria, and they colonize and consume stores of human foodstuffs, e.g., wheat (25–28).
One possible explanation for the apparent resistance of Homo sapiens to direct parasitism by non-burrowing Psoroptidae—as well as the continued affinity of some Pyroglyphidae for discarded human epidermal materials—is that a change to the epidermal environment of humans forced Pyroglyphidae to evolve from parasite to scavenger. Inasmuch as the eccrine glandular system distinguishes the epidermis of humans from that of other primates, it may have forced adaptation of the mite. If so, anything that interferes with the operation/function of glandular secretions, e.g., hygiene, might permit reversion of Pyroglyphidae to their parasitic ancestral mode. Consistent with a role for Pyroglyphidae in IgE-mediated diseases, the skin of patients with atopic dermatitis (AD) hosts many more Pyroglyphidae than does the skin of healthy persons (17).
As will be argued, phylogenetic analysis of evolutionary adaptations of primate species suggests secretions of eccrine glands participate in anti-acarian immunity. Further, the habitual removal and/or functional disruption of these secretions by contemporary hygienic practices increase acarian—human interactions, thereby accounting for the concomitant rise in both modern-day acarian-induced tick-borne illnesses and IgE-mediated diseases.
2 Blood types, α-gal and eccrine glands
Outcomes related to blood transfusion are informative. Among human populations there exists variability in the expression of immunoreactive carbohydrate epitopes on proteins and lipids of the outer membrane of red blood cells (29). Whereas blood type A individuals express as immunodominant antigen, N-acetylgalactosamine, blood type B individuals express as immunodominant antigen, D-galactose. Because type A individuals express the A antigen, they do not generate anti-A antibodies. Consequently, they tolerate blood products bearing the A antigen, but not ones bearing the B antigen. Likewise, type B individuals tolerate blood products bearing the B antigen, but not ones bearing the A antigen (30). In short, self-expression of a potentially immunoreactive carbohydrate protects the individual from a deleterious immune response that would otherwise be triggered by infused materials bearing that immunoreactant.
In a mechanistic sense, a tick bite is akin to a blood transfusion, albeit on a smaller scale. Just as ticks transmit pathogens from a previous blood meal to a new host, so, too, do they transmit residual blood materials from previous hosts. And just as humans can express A and/or B antigens, other tick hosts express taxon-specific immunoreactive carbohydrates. Thus, any tick taking a blood meal from a non-human host has potential to expose a subsequent human host to foreign immunoreactants.
Importantly, except for catarrhine primates, all mammals express the immunoreactive carbohydrate, α-gal (31). α-Gal linkages are catalyzed by the enzyme, N-acetyllactosaminide α-1,3-galactosyltransferase, encoded by the gene GGTA1 (32). Phylogenetic analysis indicates catarrhine primates underwent a loss-of-function mutation in GGTA1 ∼20–30 million years ago. That loss resulted in an inability to catalyze α-gal linkages (33). It is believed elimination of this non-essential carbohydrate allowed the production of anti-α-gal antibodies, which, in turn, protected ancestral primates from a near-extinction event (34).
Assuming the mechanics of IgE-mediated immunity are conserved among all mammalian species and, as posited by The Hypothesis, α-gal hypersensitivity in susceptible hosts develops following tick-directed “infusion” of α-gal-bearing material (2), only catarrhine primates should develop α-gal hypersensitivity. This raises the possibilities: (1) evolutionary pressure from acarians drove the expression of α-gal in mammalian lineages, and (2) catarrhine primates that ceased to express α-gal evolved an alternative means by which to deal with acarians.
The phylogenetic record indicates that, following emergence of the loss-of-function mutation in GGTA1, catarrhine primates evolved a trait best appreciated in Homo sapiens: full body expansion of eccrine glands (35). As elaborated next, experimental evidence indicates eccrine gland secretions deter epithelial colonization by microorganisms. Observational evidence indicates this deterrence extends to acarians.
3 Sweat, dermcidin and anti-acarian immunity
In Homo sapiens, epidermal secretions derive from 4 glands: eccrine, apocrine, apoeccrine and sebaceous (36, 37). Given that all 4 glands secrete onto the same epithelial surface, they undoubtedly act in concert. Because only eccrine gland expansion accompanied the GGTA1 loss-of-function mutation, what follows focuses solely on eccrine glands and their secretions.
Although many mammalian species have eccrine glands, the distribution of these glands in most mammals is limited to hand- and footpads, where they provide friction for mechanical gripping (38). In some species of platyrrhine primates, distribution of eccrine glands includes the tail, which is also used for gripping (35). In catarrhine primates, eccrine glands are distributed across the entirety of body surface area. Humans have 2–4 million eccrine glands, the most of any primate, with the greatest surface density being on palms and soles (36). Eccrine glands derive from embryonic ectoderm. Their number is fixed early in life (39). Consequently, the surface density of glands decreases with expansion of body surface area, e.g., normal growth and obesity (40, 41). Analyses of eccrine glands have focused primarily on thermoregulatory function, although recent focus includes the immunoregulatory functions of sweat content, especially antimicrobials, e.g., dermcidin (dcd) (42–44), and cytokines, e.g., IL-31 (45, 46). Interplay between IL-31 and keratinocytes is especially germane. Under normal physiological conditions, stratum corneum prevents IL-31 from stimulating keratinocytes (47). However, mechanical disruption of that barrier, as happens during acarian parasitism, exposes keratinocytes to the cytokine, stimulating the cells to recruit leukocytes characteristic of AD, e.g., eosinophils (48). In addition to eliciting inflammation, IL-31 stimulates itch (49). Thus, not only does IL-31 prompt localized inflammation at a site of barrier disruption, but it also facilitates mechanical removal of parasitic acarians by means of scratching. Indeed, in a mouse model of AD, the development of mite-dependent lesions occurs concurrent with IL-31-dependent pruritic inflammation (50, 51).
Eccrine glands include a secretory coil and a delivery duct (52). Hydrostatic pressure drives sweat generated in the secretory coil through the duct and onto the skin. The intraepidermal portion of the duct is termed the acrosyringium. The secretory coil is comprised of 3 cell types: myoepithelial, clear and dark (36). Myoepithelial cells maintain the structural integrity of the gland (53). Clear cells and dark cells generate sweat content. Clear cells contribute water, electrolytes and inorganic substances (54, 55). Dark cells, which contain Schiff-reactive granules, contribute glycoproteins and other macromolecules, including dcd, the most abundant protein in human sweat, Table 1 (56, 57).
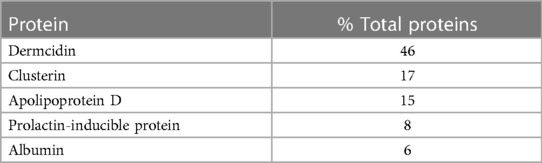
Table 1 Major proteins of eccrine gland secretions (57).
First isolated in 2001, dcd accounts for nearly half the protein in sweat of healthy individuals (57). Its precursor protein includes a 19-residue signal peptide, a 43-residue pro-domain and a 48-residue antimicrobial domain (43). Proteolytic processing of dcd within sweat generates a variety of peptides active against an array of pathogens (58–60).
Although the toxicity of dcd toward acarians has not been investigated, its toxicity toward bacteria has been. In sweat, dcd polymerizes, forming channels composed of dimeric trimers (61, 62). Such channels translocate within membranes of pathogenic bacteria, disrupting transmembrane potentials and causing bacterial death. Regarding acarians, dcd may operate similarly, disrupting the membranes of organelles critical to acarian survival, e.g., mitochondria. Alternatively, channels of dcd might compromise peritrophic membranes, rendering acarians vulnerable to naturally occurring toxins present in undigested foodstuffs (63–65).
Expressed primarily in sweat glands, dcd is also expressed in tears, breast milk and sebum (66–68). It is encoded by DCD, an orphan gene unique to primates (58). The phylogenetic record indicates species that evolved the loss-of-function mutation in GGTA1 acquired DCD and underwent propagation of eccrine glands (31, 35). If the loss-of-function mutation in GGTA1 made catarrhine primates vulnerable to α-gal hypersensitivity, then it seems likely acquisition of the DCD gene and expansion of the eccrine glandular system were direct responses to the evolutionary pressure exerted by that vulnerability.
Unlike other antimicrobial peptides, which are upregulated in response to inflammation and injury, dcd is expressed constitutively (69). This suggests it functions as a deterrent, preventing epidermal colonization by pathogenic microbes. Furthermore, dcd is remarkably stable, persisting on epidermis more than 72 h (70). Thus, epidermal surfaces should normally be covered by a substantial layer of microbial deterrent. Currently, however, humans living in developed countries consider sweat “unhygienic”: they wash routinely (generally using heated water and soap), their lives are mostly sedentary, and they live and work in air-conditioned environments. It stands to reason, that the layer of dcd on humans today is something significantly less than it was formerly, enabling a current situation that fosters epidermal colonization by deleterious organisms, including some acarians.
Because dcd is expressed by primates exclusively, investigation of DCD knockout phenotypes is lacking. However, there is one report that details the clinical manifestations of a human family with a hereditary loss-of-function mutation in DCD (71). As related there, affected persons are predisposed to hidradenitis suppurativa (HS), a dermatopathology of uncertain etiology. Importantly, HS is associated with elevated levels of IgE (72), the antibody class central to anti-acarian immunity. HS is also linked to demodicosis (73), further supporting a role for dcd in anti-acarian immunity.
4 Atopic dermatitis, acarians and sweat
AD is the most common chronic inflammatory disease of the skin. The incidence of AD is lowest in children born during the hot summer months, when sweating is common (74, 75). Epidermal surfaces of AD patients have a higher density of acarians than do those of healthy individuals (17, 76). Demodex follicularis and Demodex brevis, monoxenous acarians increased on the skin of AD patients, are absent from eccrine acrosyringia, suggesting even acarians adapted to human epidermis find the local environment of eccrine glands inhospitable (77).
AD impairs acetylcholine-mediated control of sweating by the sudomotor reflex (78), and prolonged latency of sympathetic skin responses in AD confirms dysfunctional sympathetic pathways in the disorder (79). Besides dysregulation of sweat volume, sweat content is also affected. Peptides derived from dcd are reduced in the sweat of AD patients (80). It is yet uncertain whether the impaired sudomotor reflex is etiological or whether it is a pathological consequence of disease. In either case, if, as proposed, dcd-containing sweat is cardinal to anti-acarian immunity, it is reasonable to assume that a decreased sudomotor reflex favors habitation of acarians on affected epidermal surfaces.
Multiple tick species have been shown to produce toxins that prevent pre-synaptic release of acetylcholine, a measure that inhibits muscle activation in mammals (81). Such inhibition paralyzes tick hosts precluding, at the very least, reflexive itching and self-removal of ticks. Should resident acarians similarly disrupt the cholinergic pathway controlling the sudomotor reflex, the resulting epidermal microenvironment would be one favorable to continued acarian colonization.
What follows next are brief descriptions of proposals by others that attempt to account for allergy on the basis of hygiene or epidermal barrier dysfunction. The observations that spawned those proposals are then re-interpreted in the context of The Acari Hypothesis. An argument is made that removal of sweat by current hygienic practices allows acarians, the operative agents of allergy, to flourish on epidermal surfaces and promote allergic disease.
5 Hygiene, allergy and the epidermal barrier in the context of the acari hypothesis
It was first recognized in 1989 that children of large families are less likely to develop hay fever and AD. Among the children of those families, the younger ones are less likely to develop the conditions (82). Initially, the reduced allergic burden was hypothesized to be due to an increased risk of infectious disease in childhood, a consequence of either: (1) transmission of pathogens from older siblings to younger ones, or (2) transmission of pathogens from mothers infected by their older children. As expressed more recently, modern hygiene reduces antigenic load thereby increasing susceptibility to allergic diseases (83). Unfortunately, studies since publication of the original proposal have not been supportive (84). Still, despite the perceived inadequacy of the hygiene proposal, the original observations that prompted its formulation remain valid and, until now, unexplained. In this regard, The Acari Hypothesis accounts for the observations that prompted the hygiene proposal; namely, hygienic practices that remove sweat increase epidermal infestation by certain acarians, which, in turn, increases the likelihood of allergic disease.
As noted earlier, the medical community did not appreciate the existence of allergic diseases until about 1870 (4). Around that same time, 1868, the first “water heater” was patented (85). Although acceptance and availability of water heaters by the general public were not immediate, the very existence of a patent for the device implies societal interest in bathing regularly in hot water.
The limited capacity of residential water heaters readily accounts for the association between sibship size and order, and allergic diseases. Given a finite supply of hot water, the volume available for bathing per family member goes down with increasing family size. Because the layer of sweat-derived materials on skin is inversely related to the availability of hot water for bathing, children of large families, especially the younger children, do not routinely “wash away” their replenishable anti-acarian barrier. Consequently, their skin is less accommodating to acarian habitation, lessening their risk of allergic disease.
The Acari Hypothesis also accounts for the many studies that demonstrate children raised on farms are at decreased risk of allergic diseases (86). Firstly, persons living in rural communities spend more time outside and use less air conditioning than their urban contemporaries (87, 88). Secondly, rural communities lack the resources necessary to support the level of hygiene practiced in urban settings (89). From these, it follows that children living in rural environments are both more likely to sweat and less likely to remove sweat than children living in urban environments. As a result, a more robust anti-acarian layer of sweat confers to children raised on farms resistance to the acarian interactions necessary for the development of allergic disease.
Finally, the mechanics of mite parasitism are consistent with another popular proposal on allergic disease, one that involves the barrier function of skin. Published in 2017, that proposal postulates that epidermal barrier dysfunction enables allergic sensitization and development of type 2 inflammatory disease (90). The proposal is supported by data that shows persons with mutations in epidermal structural proteins, most notably filaggrin, are at substantial risk for AD (91, 92). Filaggrin is a protein critical to the formation of corneocytes, the cells that comprise the outermost barrier of human epidermis (93). Mutations in filaggrin compromise the barrier function of the stratum corneum, thereby permitting greater penetration of antigenic material against which IgE is generated (94). In keeping with The Acari Hypothesis, pyroglyphid mites on human skin are causative agents of AD. Although the feeding habits of pyroglyphid mites on human epidermis have not been described, those of mites of their sister taxon, Psoroptidae, have been, and they are exceedingly informative (95). In order to feed, Psoroptidae first deposit antigenic material from their digestive tract onto the surface of their mammalian host. Subsequently, they abrade the host skin with their mouthparts, generating inflammation (95). The mites then feed on the resulting exudate. Importantly, secretions from the mite digestive tract contain the adjuvant-active immune complexes claimed essential per The Hypothesis (2). A weakened barrier, as occurs in persons with filaggrin mutation, undoubtedly allows for greater penetration of mite digestive secretions and, consequently, greater exposure to the adjuvant-active complexes that drive IgE formation.
6 Closing
The Acari Hypothesis posits that ancestral acarians exerted formative influence on evolution of Homo sapiens at least twice: once during the emergence of class Mammalia, the other during the emergence of catarrhine primates. Such influence likely prompted the evolution of accommodative adaptations in humans and other mammals, particularly adaptations involving epithelial surfaces. With specific regard to allergy, nonhuman mammalian species, e.g., cats, dogs, horses, etc., have evolved different adaptations to mitigate the acarian risk. Indeed, and as will be elaborated and discussed in the next installment of The Hypothesis, that risk brings clarity and uniformity to the nature of allergenicity (ACR, submitted). In the context of The Hypothesis, targeted experimentation should identify relevant human adaptations, the knowledge of which should, yield mechanistic understanding of many issues pertinent to human health and disease.
Despite sweat having already been recognized as an immunoregulatory fluid, its role in human immunity is still grossly underappreciated by scientists and clinicians alike. Although this report provides only anecdotal evidence of sweat as a deterrent to epidermal habitation by acarians, sweat is known to be active against a variety of microbes, including and especially Staphylococcus aureus. It appears disruption/removal of the microbial deterrent yields an “immunocompromised” state that enables epidermal colonization by many organisms, pathological and otherwise. If, indeed, this is the case, then it seems likely many disease processes, the emergences of which postdate contemporary hygienic practices, are consequences of disruption/removal of epidermal materials derived from eccrine glands. As one important candidate, the metabolic syndrome is eminently suited to such consideration and analysis (ACR, submitted).
Data availability statement
The original contributions presented in the study are included in the article/Supplementary Material, further inquiries can be directed to the corresponding author.
Author contributions
AR: Writing – review & editing, Writing – original draft, Investigation, Conceptualization. GR: Writing – review & editing, Resources, Funding acquisition.
Funding
The author(s) declare that financial support was received for the research, authorship, and/or publication of this article.
This research was supported in part by funding to GR from the Department of Pathology, Feinberg School of Medicine, Northwestern University.
Conflict of interest
The authors declare that the research was conducted in the absence of any commercial or financial relationships that could be construed as a potential conflict of interest.
Publisher's note
All claims expressed in this article are solely those of the authors and do not necessarily represent those of their affiliated organizations, or those of the publisher, the editors and the reviewers. Any product that may be evaluated in this article, or claim that may be made by its manufacturer, is not guaranteed or endorsed by the publisher.
References
1. Retzinger AC, Retzinger GS. Mites, ticks, anaphylaxis and allergy: the acari hypothesis. Med Hypotheses. (2020) 144:110257. doi: 10.1016/j.mehy.2020.110257
2. Retzinger AC, Retzinger GS. The acari hypothesis, II: interspecies operability of pattern recognition receptors. Pathogens. (2021) 10(9):1220. doi: 10.3390/pathogens10091220
3. Retzinger AC, Retzinger GS. The acari hypothesis, III: atopic dermatitis. Pathogens. (2022) 11(10):1083. doi: 10.3390/pathogens11101083
4. Platts-Mills TA. The allergy epidemics: 1870–2010. J Allergy Clin Immunol. (2015) 136(1):3–13. doi: 10.1016/j.jaci.2015.03.048
5. Eisen RJ, Kugeler KJ, Eisen L, Beard CB, Paddock CD. Tick-borne zoonoses in the United States: persistent and emerging threats to human health. ILAR J. (2017) 58(3):319–35. doi: 10.1093/ilar/ilx005
6. Radzišauskienė D, Žagminas K, Ašoklienė L, Jasionis A, Mameniškienė R, Ambrozaitis A, et al. Epidemiological patterns of tick-borne encephalitis in Lithuania and clinical features in adults in the light of the high incidence in recent years: a retrospective study. Eur J Neurol. (2018) 25(2):268–74. doi: 10.1111/ene.13486
7. Madison-Antenucci S, Kramer LD, Gebhardt LL, Kauffman E. Emerging tick-borne diseases. Clin Microbiol Rev. (2020) 33(2):e00083–18. doi: 10.1128/CMR.00083-18
8. Fang LQ, Liu K, Li XL, Liang S, Yang Y, Yao HW, et al. Emerging tick-borne infections in mainland China: an increasing public health threat. Lancet Infect Dis. (2015) 15(12):1467–79. doi: 10.1016/S1473-3099(15)00177-2
9. Sharma SR, Karim S. Tick saliva and the alpha-gal syndrome: finding a needle in a haystack. Front Cell Infect Microbiol. (2021) 11:680264. doi: 10.3389/fcimb.2021.680264
10. Lee WC, Lee MJ, Choi KH, Chung HS, Choe NH. A comparative study of the trends in epidemiological aspects of lyme disease infections in Korea and Japan, 2011–2016. J Vector Borne Dis. (2019) 56(3):268–71. doi: 10.4103/0972-9062.289396
11. Eddens T, Kaplan DJ, Anderson AJM, Nowalk AJ, Campfield BT. Insights from the geographic spread of the lyme disease epidemic. Clin Infect Dis. (2019) 68(3):426–34. doi: 10.1093/cid/ciy510
12. Dong Y, Zhou G, Cao W, Xu X, Zhang Y, Ji Z, et al. Global seroprevalence and sociodemographic characteristics of Borrelia burgdorferi sensu lato in human populations: a systematic review and meta-analysis. BMJ Glob Health. (2022) 7(6):e007744. doi: 10.1136/bmjgh-2021-007744
13. Ortiz DI, Piche-Ovares M, Romero-Vega LM, Wagman J, Troyo A. The impact of deforestation, urbanization, and changing land use patterns on the ecology of mosquito and tick-borne diseases in Central America. Insects. (2021) 13(1):20. doi: 10.3390/insects13010020
14. Caminade C, McIntyre KM, Jones AE. Impact of recent and future climate change on vector-borne diseases. Ann N Y Acad Sci. (2019) 1436(1):157–73. doi: 10.1111/nyas.13950
15. Tovey ER, Willenborg CM, Crisafulli DA, Rimmer J, Marks GB. Most personal exposure to house dust mite aeroallergen occurs during the day. PLoS One. (2013) 8(7):e69900. doi: 10.1371/journal.pone.0069900
16. Hewitt M, Barrow GI, Miller DC, Turk F, Turk S. Mites in the personal environment and their role in skin disorders. Br J Dermatol. (1973) 89(4):401–9. doi: 10.1111/j.1365-2133.1973.tb02995.x
17. Teplitsky V, Mumcuoglu KY, Babai I, Dalal I, Cohen R, Tanay A. House dust mites on skin, clothes, and bedding of atopic dermatitis patients. Int J Dermatol. (2008) 47(8):790–5. doi: 10.1111/j.1365-4632.2008.03657.x
18. Chudzicka-Strugała I, Gołębiewska I, Brudecki G, Elamin W, Zwoździak B. Demodicosis in different age groups and alternative treatment options-A review. J Clin Med. (2023) 12(4):1649. doi: 10.3390/jcm12041649
19. Sharaf MS. Scabies: immunopathogenesis and pathological changes. Parasitol Res. (2024) 123(3):149. doi: 10.1007/s00436-024-08173-6
20. Bochkov AV, Grootaert P. Mites of the genus paracoroptes lavoipierre, 1955 (acariformes: psoroptidae)–skin parasites of the African monkeys of the family cercopithecidae (primates). Zootaxa. (2014) 3887(2):225–38. doi: 10.11646/zootaxa.3887.2.5
21. Feldman-Muhsam B, Mumcuoglu Y, Osterovich T. A survey of house dust mites (acari: pyroglyphidae and cheyletidae) in Israel. J Med Entomol. (1985) 22(6):663–9. doi: 10.1093/jmedent/22.6.663
22. Arlian LG, Platts-Mills TA. The biology of dust mites and the remediation of mite allergens in allergic disease. J Allergy Clin Immunol. (2001) 107(3 Suppl):S406–13. doi: 10.1067/mai.2001.113670
23. Miller JD. The role of dust mites in allergy. Clin Rev Allergy Immunol. (2019) 57(3):312–29. doi: 10.1007/s12016-018-8693-0
24. Klimov PB, O'Connor B. Is permanent parasitism reversible?–critical evidence from early evolution of house dust mites. Syst Biol. (2013) 62(3):411–23. doi: 10.1093/sysbio/syt008
25. Molva V, Nesvorna M, Hubert J. Feeding interactions between microorganisms and the house dust mites dermatophagoides pteronyssinus and dermatophagoides farinae (Astigmata: pyroglyphidae). J Med Entomol. (2019) 56(6):1669–77. doi: 10.1093/jme/tjz089
26. Yi FC, Chen JY, Chee KK, Chua KY, Lee BW. Dust mite infestation of flour samples. Allergy. (2009) 64(12):1788–9. doi: 10.1111/j.1398-9995.2009.02116.x
27. Blanco C, Quiralte J, Castillo R, Delgado J, Arteaga C, Barber D, et al. Anaphylaxis after ingestion of wheat flour contaminated with mites. J Allergy Clin Immunol. (1997) 99(3):308–13. doi: 10.1016/S0091-6749(97)70047-2
28. Naegele A, Reboux G, Scherer E, Roussel S, Millon L. Fungal food choices of dermatophagoides farinae affect indoor fungi selection and dispersal. Int J Environ Health Res. (2013) 23(2):91–5. doi: 10.1080/09603123.2012.699029
29. Storry JR, Olsson ML. The ABO blood group system revisited: a review and update. Immunohematology. (2009) 25(2):48–59. PMID: 1992762019927620
30. Mitra R, Mishra N, Rath GP. Blood groups systems. Indian J Anaesth. (2014) 58(5):524–8. doi: 10.4103/0019-5049.144645
31. Galili U, Shohet SB, Kobrin E, Stults CL, Macher BA. Man, apes, and old world monkeys differ from other mammals in the expression of alpha-galactosyl epitopes on nucleated cells. J Biol Chem. (1988) 263(33):17755–62. doi: 10.1016/S0021-9258(19)77900-9
32. Huai G, Qi P, Yang H, Wang Y. Characteristics of α-gal epitope, anti-gal antibody, α1,3 galactosyltransferase and its clinical exploitation (review). Int J Mol Med. (2016) 37(1):11–20. doi: 10.3892/ijmm.2015.2397
33. Galili U. Significance of the evolutionary α1,3-galactosyltransferase (GGTA1) gene inactivation in preventing extinction of apes and old world monkeys. J Mol Evol. (2015) 80(1):1–9. doi: 10.1007/s00239-014-9652-x
34. Galili U. Paleo-immunology of human anti-carbohydrate antibodies preventing primate extinctions. Immunology. (2023) 168(1):18–29. doi: 10.1111/imm.13582
35. Best A, Kamilar JM. The evolution of eccrine sweat glands in human and nonhuman primates. J Hum Evol. (2018) 117:33–43. doi: 10.1016/j.jhevol.2017.12.003
36. Baker LB. Physiology of sweat gland function: the roles of sweating and sweat composition in human health. Temperature (Austin). (2019) 6(3):211–59. doi: 10.1080/23328940.2019.1632145
37. Zouboulis CC, Coenye T, He L, Kabashima K, Kobayashi T, Niemann C, et al. Sebaceous immunobiology - skin homeostasis, pathophysiology, coordination of innate immunity and inflammatory response and disease associations. Front Immunol. (2022) 13:1029818. doi: 10.3389/fimmu.2022.1029818
38. Adelman S, Taylor CR, Heglund NC. Sweating on paws and palms: what is its function? Am J Physiol. (1975) 229(5):1400–2. doi: 10.1152/ajplegacy.1975.229.5.1400
39. Cui CY, Schlessinger D. Eccrine sweat gland development and sweat secretion. Exp Dermatol. (2015) 24(9):644–50. doi: 10.1111/exd.12773
40. Bar-Or O, Magnusson LI, Buskirk ER. Distribution of heat-activated sweat glands in obese and lean men and women. Hum Biol. (1968) 40(2):235–48.5664189
41. Wells TR, Landing BH, Sandhu M, Lipsey AI. Microdissection study of the incidence of branched eccrine sweat glands and the number of eccrine glands per unit area of infants’ and childrens’ skin. Pediatr Pathol. (1986) 6(2-3):301–7. doi: 10.3109/15513818609037720
42. Park JH, Park GT, Cho IH, Sim SM, Yang JM, Lee DY. An antimicrobial protein, lactoferrin exists in the sweat: proteomic analysis of sweat. Exp Dermatol. (2011) 20(4):369–71. doi: 10.1111/j.1600-0625.2010.01218.x
43. Schittek B, Hipfel R, Sauer B, Bauer J, Kalbacher H, Stevanovic S, et al. Dermcidin: a novel human antibiotic peptide secreted by sweat glands. Nat Immunol. (2001) 2(12):1133–7. doi: 10.1038/ni732
44. Murakami M, Ohtake T, Dorschner RA, Schittek B, Garbe C, Gallo RL. Cathelicidin anti-microbial peptide expression in sweat, an innate defense system for the skin. J Invest Dermatol. (2002) 119(5):1090–5. doi: 10.1046/j.1523-1747.2002.19507.x
45. Cornelissen C, Lüscher-Firzlaff J, Baron JM, Lüscher B. Signaling by IL-31 and functional consequences. Eur J Cell Biol. (2012) 91(6-7):552–66. doi: 10.1016/j.ejcb.2011.07.006
46. Zhang Q, Putheti P, Zhou Q, Liu Q, Gao W. Structures and biological functions of IL-31 and IL-31 receptors. Cytokine Growth Factor Rev. (2008) 19(5-6):347–56. doi: 10.1016/j.cytogfr.2008.08.003
47. Dai X, Okazaki H, Hanakawa Y, Murakami M, Tohyama M, Shirakata Y, et al. Eccrine sweat contains IL-1α, IL-1β and IL-31 and activates epidermal keratinocytes as a danger signal. PLoS One. (2013) 8(7):e67666. doi: 10.1371/journal.pone.0067666
48. Dillon SR, Sprecher C, Hammond A, Bilsborough J, Rosenfeld-Franklin M, Presnell SR, et al. Interleukin 31, a cytokine produced by activated T cells, induces dermatitis in mice. Nat Immunol. (2004) 5(7):752–60. doi: 10.1038/ni1084
49. Sonkoly E, Muller A, Lauerma AI, Pivarcsi A, Soto H, Kemeny L, et al. IL-31: a new link between T cells and pruritus in atopic skin inflammation. J Allergy Clin Immunol. (2006) 117(2):411–7. doi: 10.1016/j.jaci.2005.10.033
50. Morita E, Kaneko S, Hiragun T, Shindo H, Tanaka T, Furukawa T, et al. Fur mites induce dermatitis associated with IgE hyperproduction in an inbred strain of mice, NC/Kuj. J Dermatol Sci. (1999) 19(1):37–43. doi: 10.1016/S0923-1811(98)00047-4
51. Takaoka A, Arai I, Sugimoto M, Honma Y, Futaki N, Nakamura A, et al. Involvement of IL-31 on scratching behavior in NC/Nga mice with atopic-like dermatitis. Exp Dermatol. (2006) 15(3):161–7. doi: 10.1111/j.1600-0625.2006.00405.x
52. Groscurth P. Anatomy of sweat glands. Curr Probl Dermatol. (2002) 30:1–9. doi: 10.1159/000060678
53. Sato K, Nishiyama A, Kobayashi M. Mechanical properties and functions of the myoepithelium in the eccrine sweat gland. Am J Physiol. (1979) 237(3):C177–84. doi: 10.1152/ajpcell.1979.237.3.C177
54. Montagna W, Chase HB, Lobitz WC Jr. Histology and cytochemistry of human skin. IV. the Eccrine Sweat Glands. J Invest Dermatol. (1953) 20(6):415–23. doi: 10.1038/jid.1953.52
55. Munger BL. The ultrastructure and histophysiology of human eccrine sweat glands. J Biophys Biochem Cytol. (1961) 11(2):385–402. doi: 10.1083/jcb.11.2.385
56. Yanagawa S, Yokozeki H, Sato K. Origin of periodic acid-schiff-reactive glycoprotein in human eccrine sweat. J Appl Physiol (1985). (1986) 60(5):1615–22. doi: 10.1152/jappl.1986.60.5.1615
57. Csősz É, Emri G, Kalló G, Tsaprailis G, Tőzsér J. Highly abundant defense proteins in human sweat as revealed by targeted proteomics and label-free quantification mass spectrometry. J Eur Acad Dermatol Venereol. (2015) 29(10):2024–31. doi: 10.1111/jdv.13221
58. Steffen H, Rieg S, Wiedemann I, Kalbacher H, Deeg M, Sahl HG, et al. Naturally processed dermcidin-derived peptides do not permeabilize bacterial membranes and kill microorganisms irrespective of their charge. Antimicrob Agents Chemother. (2006) 50(8):2608–20. doi: 10.1128/AAC.00181-06
59. Paulmann M, Arnold T, Linke D, Özdirekcan S, Kopp A, Gutsmann T, et al. Structure-activity analysis of the dermcidin-derived peptide DCD-1l, an anionic antimicrobial peptide present in human sweat. J Biol Chem. (2012) 287(11):8434–43. doi: 10.1074/jbc.M111.332270
60. Schittek B. The multiple facets of dermcidin in cell survival and host defense. J Innate Immun. (2012) 4(4):349–60. doi: 10.1159/000336844
61. Nguyen VS, Tan KW, Ramesh K, Chew FT, Mok YK. Structural basis for the bacterial membrane insertion of dermcidin peptide, DCD-1l. Sci Rep. (2017) 7(1):13923. doi: 10.1038/s41598-017-13600-z
62. Zeth K, Sancho-Vaello E. The human antimicrobial peptides dermcidin and LL-37 show novel distinct pathways in membrane interactions. Front Chem. (2017) 5:86. doi: 10.3389/fchem.2017.00086
63. Wharton GW, Brody AR. The peritrophic membrane of the mite, dermatophagoides farinae: acariformes. J Parasitol. (1972) 58(4):801–4. doi: 10.2307/3278321
64. Zhu Z, Gern L, Aeschlimann A. The peritrophic membrane of ixodes ricinus. Parasitol Res. (1991) 77(7):635–41. doi: 10.1007/BF00931028
65. Terra WR. The origin and functions of the insect peritrophic membrane and peritrophic gel. Arch Insect Biochem Physiol. (2001) 47(2):47–61. doi: 10.1002/arch.1036
66. You J, Fitzgerald A, Cozzi PJ, Zhao Z, Graham P, Russell PJ, et al. Post-translation modification of proteins in tears. Electrophoresis. (2010) 31(11):1853–61. doi: 10.1002/elps.200900755
67. Chow BD, Reardon JL, Perry EO, Laforce-Nesbitt SS, Tucker R, Bliss JM. Host defense proteins in breast milk and neonatal yeast colonization. J Hum Lact. (2016) 32(1):168–73. doi: 10.1177/0890334415592402
68. Dahlhoff M, Zouboulis CC, Schneider MR. Expression of dermcidin in sebocytes supports a role for sebum in the constitutive innate defense of human skin. J Dermatol Sci. (2016) 81(2):124–6. doi: 10.1016/j.jdermsci.2015.11.013
69. Rieg S, Garbe C, Sauer B, Kalbacher H, Schittek B. Dermcidin is constitutively produced by eccrine sweat glands and is not induced in epidermal cells under inflammatory skin conditions. Br J Dermatol. (2004) 151(3):534–9. doi: 10.1111/j.1365-2133.2004.06081.x
70. Rieg S, Seeber S, Steffen H, Humeny A, Kalbacher H, Stevanovic S, et al. Generation of multiple stable dermcidin-derived antimicrobial peptides in sweat of different body sites. J Invest Dermatol. (2006) 126(2):354–65. doi: 10.1038/sj.jid.5700041
71. Tricarico PM, Gratton R, Dos Santos-Silva CA, de Moura RR, Ura B, Sommella E, et al. A rare loss-of-function genetic mutation suggest a role of dermcidin deficiency in hidradenitis suppurativa pathogenesis. Front Immunol. (2022) 13:1060547. doi: 10.3389/fimmu.2022.1060547
72. Pascual JC, García-Martínez FJ, Martorell A, González I, Hispan P. Increased total serum IgE levels in moderate-to-severe hidradenitis suppurativa. Br J Dermatol. (2016) 175(5):1101–2. doi: 10.1111/bjd.14870
73. Ünal E, Güvendi Akçınar U, Arduç A. Hidradenitis suppurativa, metabolic syndrome, and demodex spp. infestation. Turkiye Parazitol Derg. (2018) 42(2):171–4. doi: 10.5152/tpd.2018.5330
74. Calov M, Alinaghi F, Hamann CR, Silverberg J, Egeberg A, Thyssen JP. The association between season of birth and atopic dermatitis in the Northern hemisphere: a systematic review and meta-analysis. J Allergy Clin Immunol Pract. (2020) 8(2):674–680.e5. doi: 10.1016/j.jaip.2019.10.007
75. Kuwabara Y, Nii R, Tanaka K, Ishii E, Nagao M, Fujisawa T. Season of birth is associated with increased risk of atopic dermatitis in Japanese infants: a retrospective cohort study. Allergy Asthma Clin Immunol. (2020) 16:44. doi: 10.1186/s13223-020-00443-z
76. Edslev SM, Andersen PS, Agner T, Saunte DML, Ingham AC, Johannesen TB, et al. Identification of cutaneous fungi and mites in adult atopic dermatitis: analysis by targeted 18S rRNA amplicon sequencing. BMC Microbiol. (2021) 21(1):72. doi: 10.1186/s12866-021-02139-9
77. Plewig G, Kligman AM. The role of demodex. Acne and Rosacea. Berlin, Heidelberg: Springer (1993). p. 482. ISBN-13: 978-3-642-97236-2
78. Eishi K, Lee JB, Bae SJ, Takenaka M, Katayama I. Impaired sweating function in adult atopic dermatitis: results of the quantitative sudomotor axon reflex test. Br J Dermatol. (2002) 147(4):683–8. doi: 10.1046/j.1365-2133.2002.04765.x
79. Cicek D, Kandi B, Berilgen MS, Bulut S, Tekatas A, Dertlioglu SB, et al. Does autonomic dysfunction play a role in atopic dermatitis? Br J Dermatol. (2008) 159(4):834–8. doi: 10.1111/j.1365-2133.2008.08756.x
80. Rieg S, Steffen H, Seeber S, Humeny A, Kalbacher H, Dietz K, et al. Deficiency of dermcidin-derived antimicrobial peptides in sweat of patients with atopic dermatitis correlates with an impaired innate defense of human skin in vivo. J Immunol. (2005) 174(12):8003–10. doi: 10.4049/jimmunol.174.12.8003
81. Chand KK, Lee KM, Lavidis NA, Rodriguez-Valle M, Ijaz H, Koehbach J, et al. Tick holocyclotoxins trigger host paralysis by presynaptic inhibition. Sci Rep. (2016) 6:29446. doi: 10.1038/srep29446. Erratum in: Sci Rep. (2022) 12(1):20636. doi: 10.1038/s41598-022-24528-4
82. Strachan DP. Hay fever, hygiene, and household size. Br Med J. (1989) 299(6710):1259–60. doi: 10.1136/bmj.299.6710.1259
83. Perkin MR, Strachan DP. The hygiene hypothesis for allergy - conception and evolution. Front Allergy. (2022) 3:1051368. doi: 10.3389/falgy.2022.1051368
84. Strachan DP. Family size, infection and atopy: the first decade of the “hygiene hypothesis”. Thorax. (2000) 55(Suppl 1):S2–S10. doi: 10.1136/thorax.55.suppl_1.S2
85. Yarwood D. Maughan, benjamin waddy. In: Day L, McNeil I, editors. Biographical Dictionary of the History of Technology. 2nd ed New York: Routledge (1996). p. 818–9.
86. von Mutius E. 99th Dahlem conference on infection, inflammation and chronic inflammatory disorders: farm lifestyles and the hygiene hypothesis. Clin Exp Immunol. (2010) 160(1):130–5. doi: 10.1111/j.1365-2249.2010.04138.x
87. Pavanello F, De Cian E, Davide M, Mistry M, Cruz T, Bezerra P, et al. Air-conditioning and the adaptation cooling deficit in emerging economies. Nat Commun. (2022) 13(1):1978. doi: 10.1038/s41467-022-29692-9. Erratum for: Nat Commun. (2021) 12(1):6460. doi: 10.1038/s41467-021-26592-2
88. Matz CJ, Stieb DM, Brion O. Urban-rural differences in daily time-activity patterns, occupational activity and housing characteristics. Environ Health. (2015) 14:88. doi: 10.1186/s12940-015-0075-y
89. Quispe-Coica A, Pérez-Foguet A. A new measure of hygiene inequality applied to urban-rural comparison. Int J Hyg Environ Health. (2022) 239:113876. doi: 10.1016/j.ijheh.2021.113876
90. Pothoven KL, Schleimer RP. The barrier hypothesis and oncostatin M: restoration of epithelial barrier function as a novel therapeutic strategy for the treatment of type 2 inflammatory disease. Tissue Barriers. (2017) 5(3):e1341367. doi: 10.1080/21688370.2017.1341367
91. Palmer CN, Irvine AD, Terron-Kwiatkowski A, Zhao Y, Liao H, Lee SP, et al. Common loss-of-function variants of the epidermal barrier protein filaggrin are a major predisposing factor for atopic dermatitis. Nat Genet. (2006) 38(4):441–6. doi: 10.1038/ng1767
92. Gupta J, Margolis DJ. Filaggrin gene mutations with special reference to atopic dermatitis. Curr Treat Options Allergy. (2020) 7(3):403–13. doi: 10.1007/s40521-020-00271-x
93. Hoober JK, Eggink LL. The discovery and function of filaggrin. Int J Mol Sci. (2022) 23(3):1455. doi: 10.3390/ijms23031455
94. De Benedetto A, Kubo A, Beck LA. Skin barrier disruption: a requirement for allergen sensitization? J Invest Dermatol. (2012) 132(3 Pt 2):949–63. doi: 10.1038/jid.2011.435
Keywords: the acari hypothesis, allergy, mites and ticks, α-gal hypersensitivity, human evolution, eccrine glands, sweat, hygiene
Citation: Retzinger AC and Retzinger GS (2024) The Acari Hypothesis, IV: revisiting the role of hygiene in allergy. Front. Allergy 5:1415124. doi: 10.3389/falgy.2024.1415124
Received: 10 April 2024; Accepted: 21 June 2024;
Published: 10 July 2024.
Edited by:
Nikolaos (Nikos) G. Papadopoulos, National and Kapodistrian University of Athens, GreeceReviewed by:
Wayne Robert Thomas, University of Western Australia, AustraliaKarin Fieten, University of Zurich, Switzerland
© 2024 Retzinger and Retzinger. This is an open-access article distributed under the terms of the Creative Commons Attribution License (CC BY). The use, distribution or reproduction in other forums is permitted, provided the original author(s) and the copyright owner(s) are credited and that the original publication in this journal is cited, in accordance with accepted academic practice. No use, distribution or reproduction is permitted which does not comply with these terms.
*Correspondence: Andrew C. Retzinger, YW5kcmV3LnJldHppbmdlckBnbWFpbC5jb20=