- 1Multiphase Chemistry Department, Max Planck Institute for Chemistry, Mainz, Germany
- 2Department of Dermatology, University Medical Center of the Johannes Gutenberg University, Mainz, Germany
- 3Federal Institute for Materials Research and Testing (BAM), Berlin, Germany
- 4Institute of Translational Immunology, University Medical Center of the Johannes Gutenberg University, Mainz, Germany
- 5Division of Gastroenterology, Beth Israel Deaconess Medical Center, Harvard Medical School, MA, USA
The chemical modification of aeroallergens by reactive oxygen and nitrogen species (ROS/RNS) may contribute to the growing prevalence of respiratory allergies in industrialized countries. Post-translational modifications can alter the immunological properties of proteins, but the underlying mechanisms and effects are not well understood. In this study, we investigate the Toll-like receptor 4 (TLR4) activation of the major birch and grass pollen allergens Bet v 1 and Phl p 5, and how the physiological oxidant peroxynitrite (ONOO–) changes the TLR4 activation through protein nitration and the formation of protein dimers and higher oligomers. Of the two allergens, Bet v 1 exhibited no TLR4 activation, but we found TLR4 activation of Phl p 5, which increased after modification with ONOO– and may play a role in the sensitization against this grass pollen allergen. We attribute the TLR4 activation mainly to the two-domain structure of Phl p 5 which may promote TLR4 dimerization and activation. The enhanced TLR4 signaling of the modified allergen indicates that the ONOO–-induced modifications affect relevant protein-receptor interactions. This may lead to increased sensitization to the grass pollen allergen and thus contribute to the increasing prevalence of allergies in the Anthropocene, the present era of globally pervasive anthropogenic influence on the environment.
1. Introduction
The prevalence and severity of allergic diseases triggered by airborne plant pollen and other allergens are increasing worldwide (1–5). Among others, a possible driving factor for this trend is the exposure of allergens to reactive oxygen and nitrogen species (ROS/RNS) caused by air pollution (5–8). Anthropogenic air pollutants like ozone (O3), nitrogen dioxide (NO2), and particulate matter can trigger or enhance oxidative stress and inflammatory processes that lead to the formation of endogenous ROS/RNS such as peroxynitrite (ONOO–) (5, 9). The ROS/RNS react with oxidation-sensitive amino acids of proteins, especially tyrosine, forming nitrotyrosine as well as intramolecular and intermolecular dityrosine cross-links, both known as markers of inflammation and oxidative stress (9–15). Besides modification by ONOO–, the tyrosine residues of the proteins can also be modified directly in the environment. For example, air pollutants can damage the pollen cell wall and facilitate the release of allergenic proteins and other cytoplasmic substances into the environment (16–20). The allergenic proteins can thus be directly exposed to air pollutants promoting chemical protein modification before inhalation and deposition of the proteins in the respiratory tract. Especially summer smog conditions with high O3 and NO2 concentrations have been shown to efficiently nitrate and cross-link proteins within hours to days (21–24).
Changes of the protein structure and other properties due to nitration and oligomerization can alter the allergenic and inflammatory potential of a protein affecting both, the process of sensitization and the response phase of an allergy (9, 10, 25–31). The development of an IgE-mediated allergy, i.e. sensitization, is a multistep process involving interactions of the innate and adaptive immune systems. The recognition of the allergens by receptors of the airway epithelium, such as the Toll-like receptor 4 (TLR4) and other direct interactions of the allergens with the airway epithelium are the first events after allergen inhalation (32–37). The allergen interactions lead to the release of cytokines, chemokines, and danger signals that initiate the presentation of the allergen to immune cells and the production of allergen-specific IgE, crucial for the allergic response phase. Upon re-exposure to the allergen, cross-linking of IgE antibodies that are surface-bound to effector cells, in particular mast cells, induces cell degranulation and release of pro-inflammatory mediators triggering allergic symptoms (5, 38, 39).
In this study, we investigated the TLR4 activation of the major birch pollen allergen Bet v 1 and the major grass pollen allergen Phl p 5, both being key airborne allergens in Central Europe, before and after chemical modification with ONOO–. The proteins were exposed to different amounts of ONOO– in an aqueous phase, and the modifications (tyrosine nitration, oligomerization) were analyzed by liquid chromatography (RP-HPLC, C18) and SDS-PAGE. TLR4 activation and cell viability were determined simultaneously in a stable reporter cell line with bioluminescence detection. Inhibition experiments with the TLR4 antagonist TAK-242 were performed in THP-1-LuciaTM NF-B cells.
2. Materials and methods
2.1. Protein and serum samples
Recombinant pollen allergens from birch (Betula pendula) and timothy grass (Phleum pratense) pollen allergens Bet v 1.0101 and Phl p 5.0101, referred to as Bet v 1 and Phl p 5 hereafter, were obtained from Biomay AG (Vienna, Austria). Ovalbumin (OVA) was purchased from InvivoGen (Toulouse, France) and was treated the same way as the allergens to serve as a negative control in the cell culture experiments described below. Protein stock solutions (1 mg mL−1) for chemical modification were prepared with pure water as described in Backes et al. (24).
2.2. Protein modification with peroxynitrite
Ammonium bicarbonate (98%, Ph. Eur., BP, Carl Roth, Karlsruhe, Germany) was dissolved in pure water to yield a final buffer concentration of 2 M, and the pH was adjusted to 7.8 by the addition of 1 M hydrochloric acid (37% stock solution, Merck Millipore, Darmstadt, Germany). For each reaction, 300 or L of protein solution was transferred into a brown reaction tube (Eppendorf, Hamburg, Germany), and 7.7 or L ammonium bicarbonate buffer (2 M) was added to yield a final buffer concentration of 50 mM. After being thawed on ice, sodium peroxynitrite (160–200 mM, Merck Millipore) was added to the protein solutions. To yield molar ratios of ONOO– over tyrosine residues (ONOO–/Tyr) of 1/1, 3/1, or 5/1, 0.6, 1.8, or L ONOO– were added to L samples of Bet v 1 and Phl p 5, L ONOO– to L samples of OVA (5/1), and 1, 3, or L ONOO– to L samples of Bet v 1 and Phl p 5. The reaction was performed on ice for 110 min. Afterwards, the sample was pipetted into a 10 kDa centrifugal filter (Amicon®, Merck Millipore) and centrifuged at 14 000g for 2 min (5427 R, Eppendorf). The sample was washed five times with L PBS and centrifugation at 14 000g for 2 min. For sample recovery, the filter was turned upside down, transferred into a clean microcentrifuge tube, and centrifuged at 1 000g for 2 min. To recover possible sample residues, the filter was washed with L pure water and centrifuged upside down at 1 000g for 2 min into the concentrated protein sample. Two to six independent protein samples were prepared for the different ONOO–/Tyr ratios. For mock controls of the allergens (termed mock-treated Bet v 1 and mock-treated Phl p 5 hereafter), protein solutions were treated with all buffers but without ONOO–, and were purified as described above.
2.3. HPLC-DAD analysis
The total tyrosine nitration degree (ND) of the modified samples and controls was determined by HPLC-DAD analysis as described in Selzle et al. (40). Briefly, an HPLC-DAD system (Agilent Technologies 1260 Infinity series, Waldbronn, Germany) equipped with a monomerically bound C18 column (Vydac 238TP, i.d., m, Hichrom, Berkshire, UK) was used for chromatographic separation. Gradient elution was performed at a flow rate of 0.2 mL min−1 with 0.1% (/) trifluoroacetic acid (VWR International GmbH, Darmstadt, Germany) in water and acetonitrile (Carl Roth), and absorbance was measured at wavelengths of 220 nm, 280 nm, and 357 nm. The sample injection volume was L, and each chromatographic run was performed in triplicates or duplicates. For system control and data analysis, ChemStation Software was used (Rev. C.01.07, Agilent). The ND is defined as the concentration of nitrotyrosine divided by the sum of the concentrations of nitrotyrosine and tyrosine (40). The protein concentrations of the samples were determined in parallel within the same chromatographic runs using the LC-220 method as described in Reinmuth-Selzle et al. (41).
2.4. SDS-PAGE and silver stain
Protein oligomerization was visualized and quantified by silver-stained SDS-PAGE. Protein samples were mixed with an equivalent volume of 2× Laemmli buffer, containing 65.8 mM Tris-HCl (pH 6.8, Carl Roth), 26.3% glycerol (/, Carl Roth), 2.1% SDS (Carl Roth) and 0.01% bromophenol blue (Sigma-Aldrich), and heated at 95 C for 5 min. The samples (75 ng Bet v 1 and 50 ng Phl p 5) were loaded onto a Mini-PROTEAN® TGX™ Precast Protein Gel (4–20%, Bio-Rad, Munich, Germany) together with 60 ng Color Prestained Protein Standard, Broad Range (11–245 kDa or 10–250 kDa, New-England Biolabs, Frankfurt, Germany). Electrophoresis running conditions were constant voltage of 200 V for 40 min. Following electrophoresis, the gels were stained with the Pierce Silver Stain Kit (Thermo Fisher Scientific) following the manufacturer’s protocol. For image acquisition and quantification of protein monomers, dimers, and oligomers, a ChemiDoc system (Bio-Rad) with Image Lab software 6.1 (Bio-Rad) was used. Analysis by SDS-PAGE was performed for two to three independent prepared protein samples.
2.5. Endotoxin quantification
The amount of endotoxin in native and ONOO–-modified Bet v 1 and Phl p 5 samples was quantified after dilution of the samples to a concentration of g mL−1 with endotoxin-free water using the Pierce™ LAL Chromogenic Endotoxin Quantitation Kit (Thermo Fisher Scientific) according to the manufacturer’s protocol. All samples showed less than 0.25 EU per g of protein.
2.6. HeLa TLR4 dual-luciferase reporter cells
For simultaneous determination of TLR4 activity and viability, the well-established HeLa TLR4 dual-luciferase reporter cell line was used (31). In this cell line, Renilla luciferase expressed under the control of an IL-8 promotor serves as a measure for TLR4 activity, whereas a consecutive expressed Firefly luciferase serves as a surrogate marker for cell viability. Cells were grown in Dulbecco’s Modified Eagle’s Medium (DMEM, Thermo Fisher Scientific) containing 25 mM D-glucose, and 1 mM sodium pyruvate supplemented with 10% heat-inactivated fetal calf serum (FCS, Lot #0973F, Biochrom, Berlin, Germany), 1% penicillin/streptomycin (Thermo Fisher Scientific), and g mL−1 hygromycin B (InvivoGen) in a humidified atmosphere of 5% CO2 at 37 C. For each experiment, 20 000 HeLa TLR4 dual reporter cells per well were seeded in L complete DMEM in a flat bottom 96-well plate (Greiner, Frickenhausen, Germany). On the next day, the cells were treated with native or modified allergen solutions at a final concentration of g mL−1. The samples were diluted with the culture medium to add equal volumes to the cells. Medium, mock-treated allergens, and OVA served as negative controls, and LPS from E. coli (LPS–EB, 25 ng mL−1, InvivoGen) as a positive control. After 7 h of incubation, cells were washed with L of warm Dulbecco’s PBS containing calcium and magnesium (Thermo Fisher Scientific), followed by lysing the cells using 1 passive lysis buffer (a component of the Dual-Luciferase® Reporter Assay System, Promega, Mannheim, Germany) and freezing at C overnight. The Dual-Luciferase® Reporter Assay for analysis of both Renilla and Firefly luciferase reporter activities was performed following the manufacturer’s protocol (Promega). The luminescence signals were measured in a Synergy Neo plate reader (Biotek, Bad Friedrichshall, Germany). To calculate the normalized TLR4 activity, the TLR4-driven Renilla luciferase (TLR4) signal was divided by the Firefly luciferase signal, a surrogate marker for cell viability. The resulting values were normalized to the value of the LPS-treated cells, which was set to 100%. For calculation of viability, the Firefly luciferase (viability) signal was divided by the Firefly luciferase signal of untreated cells and multiplied by 100. Two independent experiments were performed in triplicates. The dose-response relationship of TLR4 activation by Phl p 5 was investigated in additional experiments with final concentrations of 0.25, 0.5, 1, 2.5, 5, 15, 30, and g mL−1 of native Phl p 5.
2.7. THP-1-LuciaTM NF-B cells and TLR4 receptor antagonist TAK-242
TLR4 inhibition experiments were performed with THP-1-LuciaTM NF-B cells (InvivoGen). This immortalized modified THP-1 cell line allows the determination of NF-B activation by measuring the activity of secreted luciferase. The cells were grown in Roswell Park Memorial Institute (RPMI) 1640 medium (Thermo Fisher Scientific) containing 25 mM D-glucose and 1 mM sodium pyruvate supplemented with 10% heat-inactivated FBS, g mL−1 Zeocin™ (InvivoGen), and 1% penicillin/streptomycin in a humidified atmosphere of 5% CO2 at 37 C. For each experiment, 100,000 cells per well were seeded in L medium in a flat-bottom 96-well plate. To inhibit TLR4 signaling, the cells were pre-incubated in duplicates with 500 nM of the TLR4 antagonist TAK-242 (25 mM in dimethyl sulfoxide (DMSO), Merck Millipore, diluted with medium) for 4 h. Medium and medium with DMSO (g mL−1, Sigma-Aldrich) were used as negative controls. LPS-EB ultrapure (25 ng mL−1, InvivoGen) served as a positive control. Subsequently, cells were incubated with g mL−1 Phl p 5 or control samples for 24 h. Activity of NF-B was measured by QUANTI-LucTM reagent (InvivoGen) according to manufacturer’s instructions. Briefly, L of cell culture supernatant was transferred into a white plate (LUMITRACTM, Greiner), and mixed with L of QUANTI-LucTM reagent. The luminescence was detected in a Synergy Neo plate reader. For each experiment, LPS-treated cells were used as positive control, and the arithmetic mean was set to 100%. This value was used to normalize the measurement results of the Phl p 5 and medium control. Arithmetic mean values and standard deviation were calculated from the normalized values of three independent experiments performed in triplicates (samples without TAK-242) or in duplicates (samples with TAK-242). Assessment of cell viability was performed using the alamarBlue™ cell viability reagent (Thermo Fisher Scientific) according to the manufacturer’s protocol. Excitation was performed at 560 nm, and emission was measured at 590 nm in a Synergy Neo plate reader. Cells treated with DMSO in medium showed no NF–B activation or toxic effects.
2.8. Statistical analysis
GraphPad Prism version 9.0.1 (GraphPad, San Diego, CA, USA) was used for statistical analysis. Unpaired t-tests were performed to observe differences between the native and ONOO–-modified proteins.
3. Results
3.1. Nitration and oligomerization
The two major allergens Bet v 1 and Phl p 5 were exposed to ONOO– in the aqueous phase. Protein nitration was analyzed by reverse-phase chromatography, and protein oligomerization was analyzed by gel electrophoresis. Protein nitration was observed in the native and modified samples of both proteins, and tyrosine nitration degrees were quantified and are summarized in Table 1. The tyrosine nitration degree (ND) is defined as the concentration of nitrotyrosine divided by the sum of the concentrations of tyrosines and nitrotyrosines (40). The native Bet v 1 and Phl p 5 samples had low average NDs of <0.5%. The modified samples were not corrected for these values. For the modified samples, the NDs increase with increasing molar ratios of ONOO– over tyrosine residues (1/1, 3/1, 5/1) and reach maximum NDs of 27% (Bet v 1) and 24% (Phl p 5). The observed NDs are in good agreement with previous studies (23, 24).
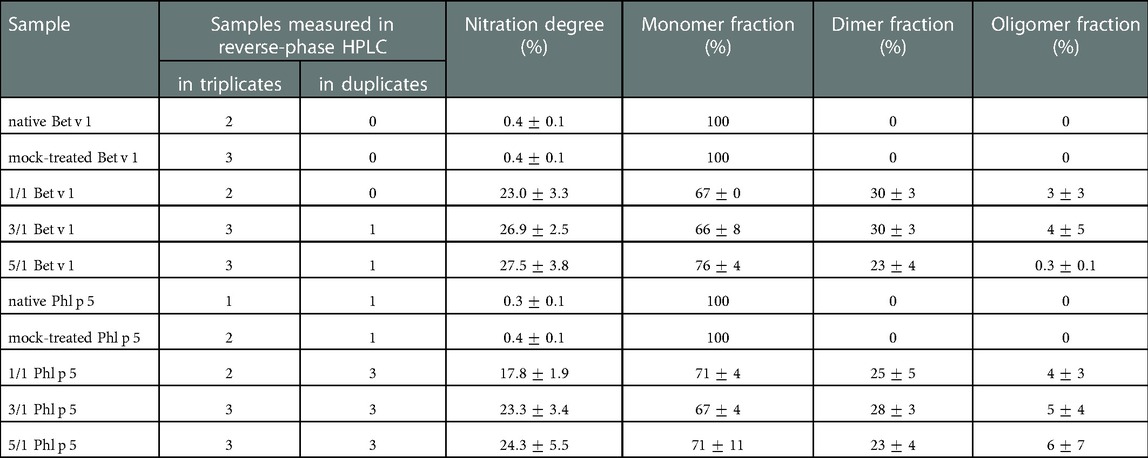
Table 1. Nitration degree and relative fractions of monomers, dimers, and oligomers of native, mock-treated, and modified Bet v 1 and Phl p 5 (arithmetic mean values and standard deviations). The allergens were modified with different molar ratios of ONOO– over tyrosine (1/1, 3/1, 5/1). Nitration degrees were determined by reversed-phase HPLC for two to six independent prepared samples measured in triplicates or duplicates, monomer/dimer/oligomer fractions were determined by SDS-PAGE for two to three independent prepared samples.
Protein dimers and higher oligomers were observed in the modified samples of both allergens (Table 1, Figure S1). The native proteins did not contain dimers or oligomers. Of the ONOO–-modified Bet v 1 samples, higher dimer and oligomer fractions were found for the samples modified with a lower molar amount of ONOO– over tyrosine (1/1, 3/1), which are probably mimicking more realistic scenarios in the human body. For Phl p 5, dimer and oligomer fractions after ONOO– modification exhibited similarly high values, and agree well with Backes et al. (24), who used size-exclusion chromatography for the determination of the protein oligomer mass fractions of modified Phl p 5.
Besides nitration, dimerization, and oligomerization, the reaction of proteins with oxidants can also result in oxidative side products and protein degradation. The shifts of the retention times and the broadening of the peak widths in reversed-phase chromatography after ONOO– modification indicate changes in hydrophobicity, denaturation, partial unfolding, and the formation of complex reaction products including aggregates and fragments (Figure S2).
3.2. TLR4 activation
TLR4 activation by the native and modified allergens was determined in a stable HeLa TLR4 dual-luciferase reporter cell line with simultaneous determination of cell viability. Figure 1 shows that native and ONOO–-modified Bet v 1 do not activate the TLR4. Native Phl p 5 showed TLR4 activation, and chemical modification with different amounts of ONOO– (1/1, 3/1, 5/1) increased the TLR4 activation of Phl p 5 by factors of 1.5 (1/1), 1.7 (3/1), and 2.1 (5/1) indicating changes in the protein-receptor interaction related to the ONOO– modification. All samples contained less than 0.25 EU endotoxin per g of protein so that false-positive results due to LPS content can be excluded. The mock-treated Phl p 5 showed increased TLR4 activation by a factor of 1.1, indicating that the sample handling induces changes to the protein that affect the interaction with the TLR4. The negative controls of the medium as well as native and ONOO–-modified Ovalbumin (OVA) exhibited no substantial TLR4 activation and the applied protein concentrations did not affect the viability of the cells (Figure S3). Additional experiments with different doses of native Phl p 5 showed a dose dependent increase of TLR4 activation (Figure 2) and no effect of the applied protein concentrations on cell viability (Figure S4). The TLR4 activation of Phl p 5 at the highest concentration (46% at g mL−1) and 5/1 ONOO–-modified Phl p 5 (43% at g mL−1) are similarly high (Figures 1 and 2). Inhibition of the TLR4 by the antagonist TAK-242 in THP-1-LuciaTM NF-B cells reduced the NF-B response by 77% (Figure 3) confirming that NF-B activation induced by Phl p 5 is mediated by the TLR4.
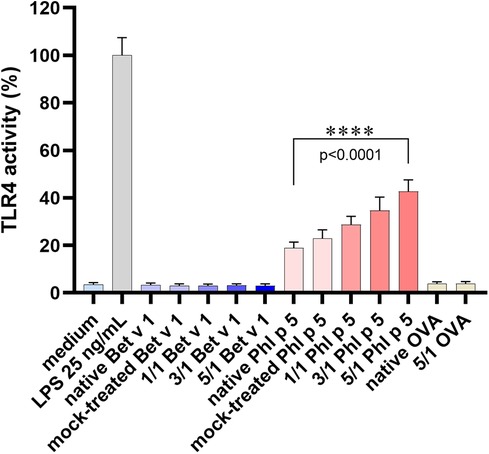
Figure 1. TLR4 activation of native and ONOO–-modified allergens. TLR4 activity in HeLa TLR4 dual-luciferase reporter cells determined for Bet v 1, Phl p 5, and OVA after modification with different molar ratios of ONOO– over tyrosine (1/1, 3/1, 5/1) and 7 h of incubation, normalized to LPS. Arithmetic mean values and standard deviations of two independent experiments performed in triplicates.
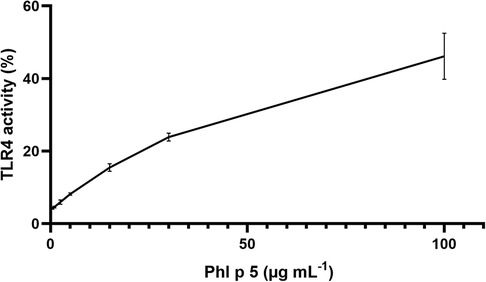
Figure 2. TLR4 activation by different concentrations of native Phl p 5 determined in HeLa dual-luciferase reporter cells and normalized to LPS. Arithmetic mean values and standard deviations for two independent experiments performed in triplicates. The line is to guide the eye.
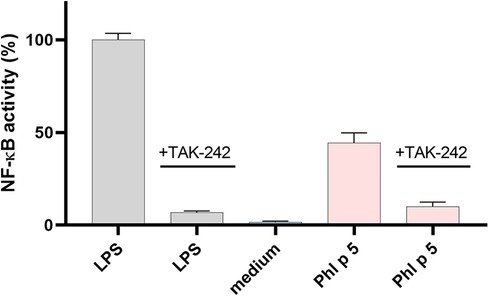
Figure 3. NF-B activation by native Phl p 5. NF-B activity in THP-1-LuciaTM NF-B cells normalized to LPS and inhibition experiments with TLR4 antagonist TAK-242. Arithmetic mean values and standard deviations of three independent experiments performed in triplicates (samples without TAK-242) or in duplicates (samples with TAK-242).
4. Discussion
The TLR4 plays a central role in inflammatory processes by recognizing a broad range of pathogen- and damage-associated molecules including bacterial Lipopolysaccharides (LPS) resulting in the release of proinflammatory mediators. Numerous studies indicate a role of the TLR4 in the pathogenesis of allergic diseases (32, 35, 42–46). Direct TLR4 activation by allergens has been reported for the house dust mite proteins Der p 2 and Der p 38 (47–49), wheat amylase trypsin inhibitors (ATI, Baker’s asthma) (50) and for the metal nickel (51). We found TLR4 activation for the grass pollen allergen Phl p 5, but not for the birch pollen allergen Bet v 1. Peroxynitrite modification enhanced the TLR4 activation of Phl p 5, which is in agreement with former studies where we showed that ONOO– modification leads to increased TLR4 activation of the TLR4 stimulating proteins ATI, -synuclein, heat shock protein 60, and high-mobility group box 1 protein (9, 31). Phl p 5 might act as a multivalent TLR4 ligand due to its two-domain structure and might thus efficiently promote TLR4 dimerization and activation. The enhancement of TLR4 activation of the mock-treated Phl p 5 could indicate that folding changes or protein denaturation contribute to better TLR4 interaction as the mock-treated Phl p 5 exhibited a ND similar low as the native Phl p 5 and behaves as a monomer in the non-reducing SDS-PAGE (Table 1, Figure S1). Also the increase of TLR4 activation by Phl p 5 modified with increasing ONOO– over tyrosine ratios could result from enhanced protein degradation associated with the ONOO– concentrations (23, 40, 52). Dimer and oligomers are less expected to play a role in the enhanced TLR4 activation as the dimer and oligomer fractions exhibited similarly high values for the Phl p 5 modified by the three different ONOO– over tyrosine ratios (Table 1). The concomitant increase of TLR4 activation and nitration degree with increasing amounts of applied ONOO– (Figure 1, Table 1), however, suggests that besides degradation and conformational changes, also tyrosine nitration could play a role in the enhanced TLR4 activation of ONOO–-modified Phl p 5. As nitrotyrosine is more acidic than tyrosine, the chemical and physiological properties of a protein such as the isoelectric point and binding to receptors and ligands can change upon nitration (25, 27, 30, 31, 53). Further investigations will be required to determine if and how nitrotyrosine contributes to the enhancement of TLR4 activation by Phl p 5.
The results show that the grass pollen allergen Phl p 5 directly activates the TLR4 and that chemical modification by ONOO– enhances the TLR4 activation and thus the inflammatory potential of Phl p 5. The direct TLR4 activation by Phl p 5 might play a role in the sensitization against the grass pollen allergen and become particularly important during oxidative stress and inflammation. If Phl p 5 is chemically modified by ROS/RNS formed during oxidative stress, innate immune responses can be enhanced through positive feedback loops via TLR4 signaling (9, 54). This amplification of innate immune responses may contribute to increased sensitization to the grass pollen allergen. Further studies are required to better understand and identify the early and most important interactions of the modified allergens with the broad spectrum of pattern recognition receptors on epithelial cells that might contribute to sensitization. Moreover, it is also necessary to analyze how the binding of IgE antibodies and allergic responses are modulated by chemical modification of allergens. The understanding of how environmental risk factors like air pollution, either directly or indirectly via oxidative stress, affect the allergenic potential of proteins is crucial for the protection of public health in the Anthropocene, i.e. the present era of globally pervasive anthropogenic influence on planet Earth and, thus, on the entire human environment (5, 55, 56). Moreover, deeper insights into chemical modifications of allergens and related immune responses can also help in the development of treatments for immune therapy.
Data availability statement
The datasets presented in this study can be found in online repositories. The names of the repository/repositories and accession number(s) can be found below: The datasets for this study are available at Edmond–the Open Access Data Repository of the Max Planck Society, under https://doi.org/10.17617/3.98W1BB (57).
Author contributions
KR-S, KL, UP, and JF-N designed the experiments. KR-S, ALL, ATB, NB, and KZ performed the experiments. KR-S, IB, MW, KL, UP, and JF-N analyzed and interpreted the data. KR-S wrote the first draft and JF-N wrote the final version of the manuscript. All authors were involved in the editing and proofreading of the manuscript. All authors contributed to the article and approved the submitted version.
Funding
The work was funded by the Max Planck Society. IB was supported by the Deutsche Forschungsgemeinschaft (DFG) grant BE 4504/3-3.
Acknowledgments
The authors acknowledge helpful discussions with the members of the Mainz Program for Chemical Allergology (MPCA) and technical support by C. S. Krevert and N.-M. Kropf.
Conflict of interest
The authors declare that the research was conducted in the absence of any commercial or financial relationships that could be construed as a potential conflict of interest.
Publisher's note
All claims expressed in this article are solely those of the authors and do not necessarily represent those of their affiliated organizations, or those of the publisher, the editors and the reviewers. Any product that may be evaluated in this article, or claim that may be made by its manufacturer, is not guaranteed or endorsed by the publisher.
Supplementary material
The Supplementary Material for this article can be found online at: https://www.frontiersin.org/articles/10.3389/falgy.2023.1066392/full#supplementary-material.
References
1. Pawankar R, Baena-Cagnani CE, Bouquet J, Canonica GW, Cruz AA, Kaliner MA, et al. State of world allergy report 2008: allergy, chronic respiratory diseases. Alergie. (2008) 10:2. doi: 10.1186/1939-4551-1-s1-s4
2. Ring J, Akdis C, Lauener R, Schäppi G, Traidl-Hoffmann C, Akdis M, et al. Global allergy forum, second Davos declaration 2013 allergy: barriers to cure - challenges and actions to be taken. Allergy. (2014) 69:978–82. doi: 10.1111/all.12406
3. D’Amato G, Cecchi L, Bonini S, Nunes C, Annesi-Maesano I, Behrendt H. Allergenic pollen and pollen allergy in Europe, et al. Allergy Eur J Allergy Clin Immunol. (2007) 62:976–90. doi: 10.1111/j.1398-9995.2007.01393.x
4. Biedermann T, Winther L, Till SJ, Panzner P, Knulst A, Valovirta E. Birch pollen allergy in Europe. Allergy Eur J Allergy Clin Immunol. (2019) 74:1237–48. doi: 10.1111/all.13758
5. Reinmuth-Selzle K, Kampf CJ, Lucas K, Lang-Yona N, Fröhlich-Nowoisky J, Shiraiwa M, et al. Air pollution and climate change effects on allergies in the anthropocene: abundance, interaction, and modification of allergens and adjuvants. Environ Sci Technol. (2017) 51:4119–41. doi: 10.1021/acs.est.6b04908
6. Saxon A, Diaz-Sanchez D. Air pollution and allergy: you are what you breathe. Nat Immunol. (2005) 6:223–6. doi: 10.1038/ni0305-223
7. D’Amato G. Effects of climatic changes and urban air pollution on the rising trends of respiratory allergy and asthma. Multidiscip Respir Med. (2011) 6:28. doi: 10.1186/2049-6958-6-1-28
8. D’Amato G, Baena-Cagnani CE, Cecchi L, Annesi-Maesano I, Nunes C, Ansotegui I, et al. Climate change, air pollution and extreme events leading to increasing prevalence of allergic respiratory diseases. Multidiscip Respir Med. (2013) 8:12. doi: 10.1186/2049-6958-8-12
9. Ziegler K, Kunert AT, Reinmuth-Selzle K, Leifke AL, Widera D, Weller MG, et al. Chemical modification of pro-inflammatory proteins by peroxynitrite increases activation of TLR4 and NF-B: Implications for the health effects of air pollution and oxidative stress. Redox Biol. (2020) 37:101581. doi: 10.1016/j.redox.2020.101581
10. Ischiropoulos H. Protein tyrosine nitration-An update. Arch Biochem Biophys. (2009) 484:117–21. doi: 10.1016/j.abb.2008.10.034
11. Bachi A, Dalle-Donne I, Scaloni A. Redox proteomics: chemical principles, methodological approaches, biological/biomedical promises. Chem Rev. (2013) 113:596–698. doi: 10.1021/cr300073p
12. Radi R. Protein tyrosine nitration: Biochemical mechanisms, structural basis of functional effects. Acc Chem Res. (2013) 46:550–9. doi: 10.1021/ar300234c
13. Shiraiwa M, Selzle K, Yang H, Sosedova Y, Ammann M, Pöschl U. Multiphase chemical kinetics of the nitration of aerosolized protein by ozone and nitrogen dioxide. Environ Sci Technol. (2012) 46:6672–80. doi: 10.1021/es300871b
14. Kampf CJ, Liu F, Reinmuth-Selzle K, Berkemeier T, Meusel H, Shiraiwa M, et al. Protein cross-linking and oligomerization through dityrosine formation upon exposure to ozone. Environ Sci Technol. (2015) 49:10859–66. doi: 10.1021/acs.est.5b02902
15. Liu F, Reinmuth-Selzle K, Lai S, Weller MG, Pöschl U, Kampf CJ. Simultaneous determination of nitrated and oligomerized proteins by size exclusion high-performance liquid chromatography coupled to photodiode array detection. J Chromatogr A. (2017) 1495:76–82. doi: 10.1016/j.chroma.2017.03.015
16. Taylor P, Flagan R, Miguel A, Valenta R, Glovsky M. Birch pollen rupture and the release of aerosols of respirable allergens. Clin Exp Allergy. (2004) 34:1591–6. doi: 10.1111/j.1365-2222.2004.02078.x
17. Bryce M, Drews O, Schenk MF, Menzel A, Estrella N, Weichenmeier I, et al. Impact of urbanization on the proteome of birch pollen and its chemotactic activity on human granulocytes. Int Arch Allergy Immunol. (2009) 151:46–55. doi: 10.1159/000232570
18. Motta A, Marliere M, Peltre G, Sterenberg P, Lacroix G. Traffic-related air pollutants induce the release of allergen-containing cytoplasmic granules from grass pollen. Int Arch Allergy Immunol. (2006) 139:294–8. doi: 10.1159/000091600
19. Ouyang Y, Xu Z, Fan E, Li Y, Zhang L. Effect of nitrogen dioxide and sulfur dioxide on viability and morphology of oak pollen. Int Forum Allergy Rhinol. (2016) 6:95–100. doi: 10.1002/alr.21632
20. Sedghy F, Varasteh AR, Sankian M, Moghadam M. Interaction between air pollutants and pollen grains: the role on the rising trend in allergy. Reports Biochem Mol Biol. (2018) 6:219–24. PMID: 29766006
21. Franze T, Weller MG, Niessner R, Pöschl U. Enzyme immunoassays for the investigation of protein nitration by air pollutants. Analyst. (2003) 128:824–31. doi: 10.1039/B303132B
22. Franze T, Weller MG, Niessner R, Pöschl U. Protein nitration by polluted air. Environ Sci Technol. (2005) 39:1673–78. doi: 10.1021/es0488737
23. Reinmuth-Selzle K, Kampf CJ, Samonig M, Shiraiwa M, Kofler S, Yang H, et al. Nitration of the Birch Pollen Allergen Bet v 1. 0101: efficiency, site-selectivity of liquid, gaseous nitrating agents. J Proteome Res. (2014) 13:1570–7. doi: 10.1021/pr401078h
24. Backes AT, Reinmuth-Selzle K, Leifke AL, Ziegler K, Krevert CS, Tscheuschner G, et al. Oligomerization and nitration of the grass pollen allergen Phl p 5 by ozone, nitrogen dioxide and peroxynitrite: reaction products, kinetics and health effects. Int J Mol Sci. (2021) 22:7616. doi: 10.3390/ijms22147616
25. Greenacre S, Ischiropoulos H. Tyrosine nitration: localisation, quantification, consequences for protein function, signal transduction. Free Radic Res. (2001) 34:541–81. doi: 10.1080/10715760100300471
26. Gruijthuijsen YK, Grieshuber I, Stöcklinger A, Tischler U, Fehrenbach T, Weller MG, et al. Nitration enhances the allergenic potential of proteins. Int Arch Allergy Immunol. (2006) 141:265–75. doi: 10.1159/000095296
27. Abello N, Kerstjens HA, Postma DS, Bischoff R. Protein tyrosine nitration: selectivity, physicochemical, biological consequences, denitration, and proteomics methods for the identification of tyrosine-nitrated proteins. J Proteome Res. (2009) 8:3222–38. doi: 10.1021/pr900039c
28. Untersmayr E, Diesner SC, Oostingh GJ, Selzle K, Pfaller T, Schultz C, et al. Nitration of the egg-allergen ovalbumin enhances protein allergenicity but reduces the risk for oral sensitization in a murine model of food allergy. PLoS ONE. (2010) 5:1–10. doi: 10.1371/journal.pone.0014210
29. Karle AC, Oostingh GJ, Mutschlechner S, Ferreira F, Lackner P, Bohle B, et al. Nitration of the pollen allergen Bet v 1.0101 enhances the presentation of Bet v 1-derived peptides by HLA-DR on human dendritic cells. PLoS ONE. (2012) 7:e31483. doi: 10.1371/journal.pone.0031483
30. Ackaert C, Kofler S, Horejs-Hoeck J, Zulehner N, Asam C, Von Grafenstein S, et al. The impact of nitration on the structure and immunogenicity of the major birch pollen allergen Bet v 1.0101. PLoS ONE. (2014) 9:1–11. doi: 10.1371/journal.pone.0104520
31. Ziegler K, Neumann J, Liu F, Fröhlich-Nowoisky J, Cremer C, Saloga J, et al. Nitration of wheat amylase trypsin inhibitors increases their innate and adaptive immunostimulatory potential in vitro. Front Immunol. (2019) 10:1–10. doi: 10.3389/fimmu.2018.03174
32. Gandhi VD, Vliagoftis H. Airway epithelium interactions with aeroallergens: Role of secreted cytokines and chemokines in innate immunity. Front Immunol. (2015) 6:1–13. doi: 10.3389/fimmu.2015.00147
33. Lambrecht BN, Hammad H. Allergens and the airway epithelium response: gateway to allergic sensitization. J Allergy Clin Immunol. (2014) 134:499–507. doi: 10.1016/j.jaci.2014.06.036
34. Li DQ, Zhang L, Pflugfelder SC, De Paiva CS, Zhang X, Zhao G, et al. Short ragweed pollen triggers allergic inflammation through Toll-like receptor 4-dependent thymic stromal lymphopoietin/OX40 ligand/OX40 signaling pathways. J Allergy Clin Immunol. (2011) 128:1318–25.e2. doi: 10.1016/j.jaci.2011.06.041
35. Hosoki K, Aguilera-Aguirre L, Brasier AR, Kurosky A, Boldogh I, Sur S. Facilitation of allergic sensitization and allergic airway inflammation by pollen-induced innate neutrophil recruitment. Am J Respir Cell Mol Biol. (2016) 54:81–90. doi: 10.1165/rcmb.2015-0044OC
36. Li J, Zhang L, Chen X, Chen D, Hua X, Bian F, et al. Pollen/TLR4 innate immunity signaling initiates IL-33/ST2/Th2 pathways in allergic inflammation. Sci Rep. (2016) 6:1–10. doi: 10.1038/srep36150
37. Salazar F, Ghaemmaghami AM. Allergen recognition by innate immune cells: critical role of dendritic and epithelial cells. Front Immunol. (2013) 4:1–10. doi: 10.3389/fimmu.2013.00356
38. Van Ree R, Hummelshøj L, Plantinga M, Poulsen LK, Swindle E. Allergic sensitization: host-immune factors. Clin Transl Allergy. (2014) 4:1–9. doi: 10.1186/2045-7022-4-12
39. Valenta R, Karaulov A, Niederberger V, Gattinger P, van Hage M, Flicker S, et al. Molecular aspects of allergens and allergy, vol. 138, 1st edn. Elsevier (2018). p. 195–256. doi: 10.1016/bs.ai.2018.03.002
40. Selzle K, Ackaert C, Kampf CJ, Kunert AT, Duschl A, Oostingh GJ, et al. Determination of nitration degrees for the birch pollen allergen Bet v 1. Anal Bioanal Chem. (2013) 405:8945–9. doi: 10.1007/s00216-013-7324-0
41. Reinmuth-Selzle K, Tchipilov T, Backes AT, Tscheuschner G, Tang K, Ziegler K, et al. Determination of the protein content of complex samples by aromatic amino acid analysis, liquid chromatography-UV absorbance, and colorimetry. Anal Bioanal Chem. (2022) 414(15):4457–70. doi: 10.1007/s00216-022-03910-1
42. Tan AM, Chen HC, Pochard P, Eisenbarth SC, Herrick CA, Bottomly HK. TLR4 signaling in stromal cells is critical for the initiation of allergic Th2 responses to inhaled antigen. J Immunol. (2010) 184:3535–44. doi: 10.4049/jimmunol.0900340
43. Radman M, Golshiri A, Shamsizadeh A, Zainodini N, Bagheri V, Arababadi MK, et al. Toll-like receptor 4 plays significant roles during allergic rhinitis. Allergol Immunopathol (Madr). (2015) 43:416–20. doi: 10.1016/j.aller.2014.04.006
44. Xu H, Shu H, Zhu J, Song J. Inhibition of TLR4 inhibits allergic responses in murine allergic rhinitis by regulating the NF-B pathway. Exp Ther Med. (2019) 18(1):761–8. doi: 10.3892/etm.2019.7631
45. Pointner L, Bethanis A, Thaler M, Traidl-Hoffmann C, Gilles S, Ferreira F, et al. Initiating pollen sensitization - complex source, complex mechanisms. Clin Transl Allergy. (2020) 10:1–18. doi: 10.1186/s13601-020-00341-y
46. Pointner L, Kraiem A, Thaler M, Richter F, Wenger M, Bethanis A, et al. Birch pollen induces toll-like receptor 4-dependent dendritic cell activation favoring T cell responses. Front Allergy. (2021) 2:1–20. doi: 10.3389/falgy.2021.680937
47. Hammad H, Chieppa M, Perros F, Willart MA, Germain RN, Lambrecht BN. House dust mite allergen induces asthma via Toll-like receptor 4 triggering of airway structural cells. Nat Med. (2009) 15:410–6. doi: 10.1038/nm.1946
48. Trompette A, Divanovic S, Visintin A, Blanchard C, Hegde RS, Madan R, et al. Allergenicity resulting from functional mimicry of a Toll-like receptor complex protein. Nature. (2009) 457:585–8. doi: 10.1038/nature07548
49. Kim G, Hong M, Kashif A, Hong Y, Park BS, Mun JY, et al. Der f 38 is a novel TLR4-binding allergen related to allergy pathogenesis from dermatophagoides farinae. Int J Mol Sci. (2021) 22:1–16. doi: 10.3390/ijms22168440
50. Schuppan D, Zevallos V. Wheat amylase trypsin inhibitors as nutritional activators of innate immunity. Dig Dis. (2015) 33:260–3. doi: 10.1159/000371476
51. Schmidt M, Raghavan B, Müller V, Vogl T, Fejer G, Tchaptchet S, et al. Crucial role for human Toll-like receptor 4 in the development of contact allergy to nickel. Nat Immunol. (2010) 11:814–9. doi: 10.1038/ni.1919
52. Ferrer-Sueta G, Radi R. Chemical biology of peroxynitrite: kinetics, diffusion, and radicals. ACS Chem Biol. (2009) 4:161–77. doi: 10.1021/cb800279q
53. Ferrer-Sueta G, Campolo N, Trujillo M, Bartesaghi S, Carballal S, Romero N, et al. Biochemistry of peroxynitrite and protein tyrosine nitration. Chem Rev. (2018) 118:1338–408. doi: 10.1021/acs.chemrev.7b00568
54. Lucas K, Maes M. Role of the toll like receptor (TLR) radical cycle in chronic inflammation: possible treatments targeting the TLR4 pathway. Mol Neurobiol. (2013) 48:190–204. doi: 10.1007/s12035-013-8425-7
55. Pöschl U, Shiraiwa M. Multiphase chemistry at the atmosphere-biosphere interface influencing climate and public health in the anthropocene. Chem Rev. (2015) 115:4440–75. doi: 10.1021/cr500487s
Keywords: allergy, Bet v 1, Phl p 5, nitration, dimers, oligomers, peroxynitrite, air pollution
Citation: Reinmuth-Selzle K, Bellinghausen I, Leifke AL, Backes AT, Bothen N, Ziegler K, Weller MG, Saloga J, Schuppan D, Lucas K, Pöschl U and Fröhlich-Nowoisky J (2023) Chemical modification by peroxynitrite enhances TLR4 activation of the grass pollen allergen Phl p 5. Front. Allergy 4:1066392. doi: 10.3389/falgy.2023.1066392
Received: 10 October 2022; Accepted: 12 January 2023;
Published: 15 February 2023.
Edited by:
Andreas L. Lopata James Cook University, AustraliaReviewed by:
Ulrich Matthias Zissler University Hospital rechts der Isar, Technical University of Munich, Germany,Wenting Luo First Affiliated Hospital of Guangzhou Medical University, China
© 2023 Reinmuth-Selzle, Bellinghausen, Leifke, Backes, Bothen, Ziegler, Weller, Saloga, Schuppan, Lucas, Pöschl and Fröhlich-Nowoisky. This is an open-access article distributed under the terms of the Creative Commons Attribution License (CC BY). The use, distribution or reproduction in other forums is permitted, provided the original author(s) and the copyright owner(s) are credited and that the original publication in this journal is cited, in accordance with accepted academic practice. No use, distribution or reproduction is permitted which does not comply with these terms.
*Correspondence: Janine Fröhlich-Nowoisky j.frohlich@mpic.de
Specialty Section: This article was submitted to Environmental & Occupational Determinants, a section of the journal Frontiers in Allergy