- 1Laboratory of Immunopharmacology, Faculty of Pharmaceutical Sciences, Setsunan University, Hirakata, Japan
- 2Department of Otolaryngology, Head & Neck Surgery, Osaka Medical and Pharmaceutical University, Takatsuki, Japan
Allergen-specific immunotherapy (AIT) is the only causative treatment for allergic diseases by modification of the immune response to allergens. A key feature of AIT is to induce immunotolerance to allergens by generating antigen-specific regulatory T (Treg) cells in allergic patients. Type 1 regulatory T (Tr1) cells and forkhead box protein 3 (Foxp3)-expressing Treg cells are well known among Treg cell subsets. Foxp3 was identified as a master transcription factor of Treg cells, and its expression is necessary for their suppressive activity. In contrast to Foxp3+ Treg cells, the master transcription factor of Tr1 cells has not been elucidated. Nevertheless, Tr1 cells are generally considered as a distinct subset of Treg cells induced in the periphery during antigen exposure in tolerogenic conditions and can produce large amounts of anti-inflammatory cytokines such as interleukin-10 and transforming growth factor-β, followed by down-regulation of the function of effector immune cells independently of Foxp3 expression. Since the discovery of Tr1 cells more than 20 years ago, research on Tr1 cells has expanded our understanding of the mechanism of AIT. Although the direct precursors and true identity of these cells continues to be disputed, we and others have demonstrated that Tr1 cells are induced in the periphery by AIT, and the induced cells are re-activated by antigens, followed by suppression of allergic symptoms. In this review, we discuss the immune mechanisms for the induction of Tr1 cells by AIT and the immune-suppressive roles of Tr1 cells in AIT.
Introduction
Breaking immunotolerance to innocuous antigens causes the development of allergic diseases, such as asthma, rhinitis, and conjunctivitis. The development of allergic diseases is mainly dominated by type 2 immunity. Allergen-specific Th2 cells and type 2 innate lymphoid cells (ILC2) have essential roles in the development by producing type 2 cytokines, such as interleukin (IL)-4, IL-5 and IL-13 (1). These cytokines induce allergen-specific IgE antibody production from B cells and eosinophilic infiltration and proliferation, leading to exacerbation of allergic symptoms (1). Epithelial cells are also regulated by type 2 cytokines. IL-4 orchestrates epithelial cells toward type 2 phenotype (E2 phenotype), characterized by upregulation of chemokine ligand 26 (CCL26) and IL-24 expressions, which induce the production of mucus and anti-microbial peptides (2), leading to the development of airway remodeling (3). Conversely, interferon-γ (IFN-γ) impedes the acquisition of the E2 phenotype on epithelial cells (2). Although understanding of the mechanisms underlying allergic diseases has been advanced (4), pharmacotherapies including molecular targeted drugs have been developed for the treatment (5), the prevalence of allergic diseases has gradually increased worldwide (6, 7). Therefore, modification of the natural history of allergic diseases is crucial for a radical cure of allergic diseases.
Allergen-specific immunotherapy (AIT) is the only causative treatment for allergic diseases by induction of immune tolerance to allergens (8). Since Noon (9) first demonstrated that subcutaneous injection of a grass pollen extract was effective in modulating sensitivity to grass pollen, the mechanisms and development of further safe treatment routes have been elucidated (10). Currently, AIT has been mainly conducted in two forms: subcutaneous immunotherapy (SCIT) and sublingual immunotherapy (SLIT) (11). The clinical effectiveness and safety of SCIT and SLIT for allergic diseases, especially allergic rhinitis (12, 13) and asthma (14, 15), have been established. Moreover, AIT can prevent not only the development of allergic diseases (16) but also sensitization to new allergens (17). However, AIT remains underused mainly because (1) long-term treatment is required to acquire sustainable remission of allergic symptoms (18, 19), (2) some patients are non-responders (20, 21) and (3) rare anaphylactic reactions (22, 23). Therefore, a deeper understanding of the mechanisms associated with AIT is essential in order to develop more effective treatments.
The mechanisms of AIT have been dissected in different compartments: (1) B cell-associated changes (24): induction of regulatory B cells, an increase in allergen-specific IgG4 antibodies, and a decrease in allergen-specific IgE antibodies, (2) ILC2-related changes (25, 26): immune deviation from ILC2 toward ILC1, decreases in IL-5 and IL-13 productions, and inversely an increase in IL-10 production, and (3) T-cell associated changes (27–30): immune deviation from Th2-cell toward Th1-cell response, suppression of T follicular helper cells, trans-differentiation from Th17 cells into T regulatory 17 (Tr17) cells, and increases in regulatory T (Treg) cells. Concurrent with Treg cells by AIT, studies (31–33) have demonstrated that AIT induced T-cell exhaustion in humans and mice. Interleukin (IL)-10 and transforming growth factor (TGF)-β, which are produced by AIT-induced Treg cells, have been demonstrated to enforce T-cell exhaustion (34, 35). On the other hand, another AIT study (36) reported that the exhaustion markers, programmed cell death-1 (PD-1) and cytotoxic T-lymphocyte-associated protein 4 (CTLA-4), on Th2 cells were decreased in the up-dosing of AIT, but persisted for long-term during the maintenance phase of the treatment. Therefore, the induction of Treg cells by AIT could be crucial for maintaining immunotolerance to allergens.
Although various subsets of Treg cells have been reported, CD4+ Treg cells are well-characterized. In addition, the existence of CD8+ Treg cells (37), CD4− CD8− Treg cells (38), and γδ Treg cells (39) has also been reported. As shown in Table 1, CD4+ Treg cells can be broadly classified into two groups based on where the cells occurred: thymus-derived Treg (tTreg) cells and peripherally induced Treg (pTreg) cells (40–47). tTreg cells constitutively express the transcription factor forkhead box protein 3 (Foxp3), which controls the immunosuppressive functions of Treg cells (48). After their maturation, they move to tissues to prevent harmful immune responses against self-antigens. On the other hand, pTreg cells develop from naïve CD4+ T cells when the cells are persistently exposed to exogenous antigens, followed by the induction of tolerance toward exogenous antigens such as allergens. Moreover, pTreg cells are divided into two subsets based on whether Foxp3 is expressed in the cells: Foxp3+ pTreg cells and Foxp3− type 1 regulatory T (Tr1) cells.
More than 30 years ago, an original study on human leukocyte antigen (HLA) fully mismatched fetal liver hematopoietic stem cells successfully transplanted into a patient with severe combined immunodeficiency (SCID) led to the groundbreaking discovery of Tr1 cells (49). In this patient, immunological tolerance with mixed chimerism of donor T cells and recipient antigen-presenting cells was developed without immunosuppressive agents. However, T cells derived from this patient significantly proliferated upon exposure to host antigens in vitro. This result suggested the presence of active suppression mechanisms in peripheral tissues. The human interleukin (IL)-10 gene was subsequently isolated from CD4+ T cells derived from this successfully transplanted patient (50). Moreover, host-specific IL-10-producing CD4+ T cells were identified in an another successfully transplanted patient (51). Groux et al. (52) demonstrated that IL-10-producing CD4+ T cells can be generated by chronic antigen stimulation in the presence of IL-10 in mice and humans. These antigen-specific IL-10-producing CD4+ T cells effectively suppressed the development of colitis induced in SCID mice and were named Tr1 cells (52). A subsequent study (53) demonstrated that Tr1 cells exerted immunosuppressive functions independent of Foxp3.
Many studies have been reported that latency-associated peptide (LAP)+ CD25− CD4+ T cells (54, 55), natural killer group 2, member D (NKG2D)+ CD25− CD4+ T cells (56, 57), CD127low CD25+ CD4+ T cells (58), CD49b+ CD25− CD4+ T cells (59–62), lymphocyte activation gene 3 (LAG3)+ CD25− CD4+ T cells (63), CD44high CD62Llow IL-7 receptor (IL-7R)− LAG3+ CD49b+ LAP+ CD4+ T cells (64), and C-C chemokine receptor type 5 (CCR5)+ programmed cell death 1 (PD-1)+ CD25− CD4+ T cells (65, 66) were Tr1 cells in mice or humans (Table 2). Recently, Gagliani et al. (47) demonstrated that CD49b- and LAG3-expressing CD4+ T cells can be identified as the true phenotype of Tr1 cells in mice and humans. On the other hand, Huang et al. (67) reported that co-expression of CD49b and LAG3 were not restricted to Foxp3− Tr1 cells but also observed in Foxp3+ Treg cells. In this way, the specific markers of Tr1 cells continue to be disputed.
Although there is a lack of specific markers of Tr1 cells, the existence of various allergen-specific Tr1 cells whose characteristic features are Foxp3-negative IL-10-producing CD4+ T cells, have also been reported in allergic models of mice (68–70) and allergic patients (71–78). Several clinical studies (79–82) demonstrated that the number of Tr1 cells increased in AIT-treated allergic patients, and the number such cells correlated with the clinical score. These studies suggested that Tr1 cells have crucial roles in immune tolerance to allergens. In this review, we focused on Tr1 cells, and discussed the immune mechanisms for the induction of Tr1 cells and the immune-suppressive roles of Tr1 cells in AIT.
Clinical relevance of Tr1 cells in allergy and AIT
Breakdown of immunological tolerance to allergens results in the development or exacerbation of allergic diseases. One of the features in the breakdown of immunological tolerance is the reduction or dysfunction of Tr1 cells. Studies (74, 76) have reported that the numbers of Tr1 cells in allergic patients was significantly lower than those in healthy subjects. Our group also revealed that the numbers of Tr1 cells in peripheral blood of Japanese cedar pollinosis patients was markedly lower than those of healthy subjects (81). Han et al. (76) demonstrated that the frequency of Dermatophagoides pteronyssinus major allergen 1 (Der p 1)-specific Tr1 cells in peripheral blood was decreased in house dust mite-induced allergic rhinitis patients, and it correlated with the clinical symptom scores. Moreover, the dysfunction of Tr1 cells was observed in allergic patients. CD46 is a complement regulatory protein that is upregulated in activated leukocytes to protect these cells from autologous complement-induced lysis at inflammatory sites (83). Cross-linking of CD46 during T-cell stimulation leads to the strong induction of Tr1 cells (84). Xu et al. (71) first demonstrated that the number of CD46-induced Tr1 cells was decreased in the peripheral blood of asthmatic patients compared with that of healthy subjects. The failure of a generation of Tr1 cells was observed in asthma but also multiple sclerosis (85). Ni Choileain et al. (86) demonstrated this failure was caused by the incorrect recruitment of CD46 to the immunological synapse, which reduced the conversion of effector CD4+ T cells to Tr1 cells. More recently, it has been shown that peanut-specific Tr1 cells can be induced in healthy subjects and patients with peanut allergies, but those in patients with peanut allergies are functionally defective (73). Therefore, the lack of the generation and function of allergen-specific Tr1 cells led to the development and deterioration of allergic diseases in humans.
In vivo induction of allergen-specific Tr1 cells has been explored to acquire immune tolerance to allergens. Repetitive administration of bee venom through bee stings in non-allergic beekeepers transformed from bee venom-specific Th2 cells into Tr1 cells (72). These Tr1 cells suppressed the proliferation of bee venom-specific T cells in vitro. This result suggested that AITs may be effective for allergic diseases because of the conversion of allergen-specific effector Th2 cells into tolerance-inducing Tr1 cells by repeated allergen administrations. Indeed, many studies [reviewed in (10, 87)] have demonstrated that AIT can be effective for various allergic diseases, and the frequency of allergen-specific Tr1 cells was correlated with the decrease in clinical scores (79–82). Taken together, these studies suggested that Tr1 cells play a critical role in the induction of immunotolerance to allergens in humans.
Several studies (88–90) demonstrated that not only Tr1 cells but also Foxp3+ Treg cells were increased in AIT-treated patients with allergies. Although which subset of Tr1 cells or Foxp3+ Treg cells is important for the improvement of allergic symptoms in AIT has not been fully proven, it may be dependent on the route of antigen administration. Our group previously demonstrated that the number of Tr1 cells but not Foxp3+ Treg cells was increased in peripheral blood and inflamed tissues of SCIT-treated allergic patients and mice (70, 81). Moreover, the induced Tr1 cells produced a large amount of IL-10 in response to antigen stimulation, followed by suppression of the development of asthma in the OVA-induced airway inflammation model of mice (91, 92). Lou et al. (79) also reported that the increase in Tr1 cells by SCIT was correlated with improvement in nasal symptoms, but the number of Foxp3+ Treg cells was not. These data imply that the increase in Tr1 cells but not Foxp3+ Treg cells could be crucial for the induction of immunotolerance to allergens by SCIT. On the other hand, our group (82) reported that both numbers of Tr1 cells and Foxp3+ Treg cells were markedly increased in the peripheral blood of Japanese cedar pollinosis patients who had received SLIT. Moreover, the number of Foxp3+ Treg cells positively correlated with improvement in nasal symptoms, whereas those of Tr1 cells did not (82). Therefore, Foxp3+ Treg cells rather than Tr1 cells may have contributed to the clinical effects of SLIT. This is also supported by a study of Xian et al. (93). Therefore, which subset of Tr1 cells or Foxp3+ Treg cells is more relevant to clinical tolerance may depend on the antigen administration route.
Induction mechanisms of Tr1 cells in AIT
High-dose allergen exposure in AIT promotes dendritic cells to produce IL-27, IL-10, and transforming growth factor-beta (TGF-β) (94, 95). Naïve CD4+ T cells can differentiate into Tr1 cells upon T cell receptor (TCR) engagement in the presence of these cytokines. The induction mechanisms of Tr1 cells in AIT are summarized in Figure 1.
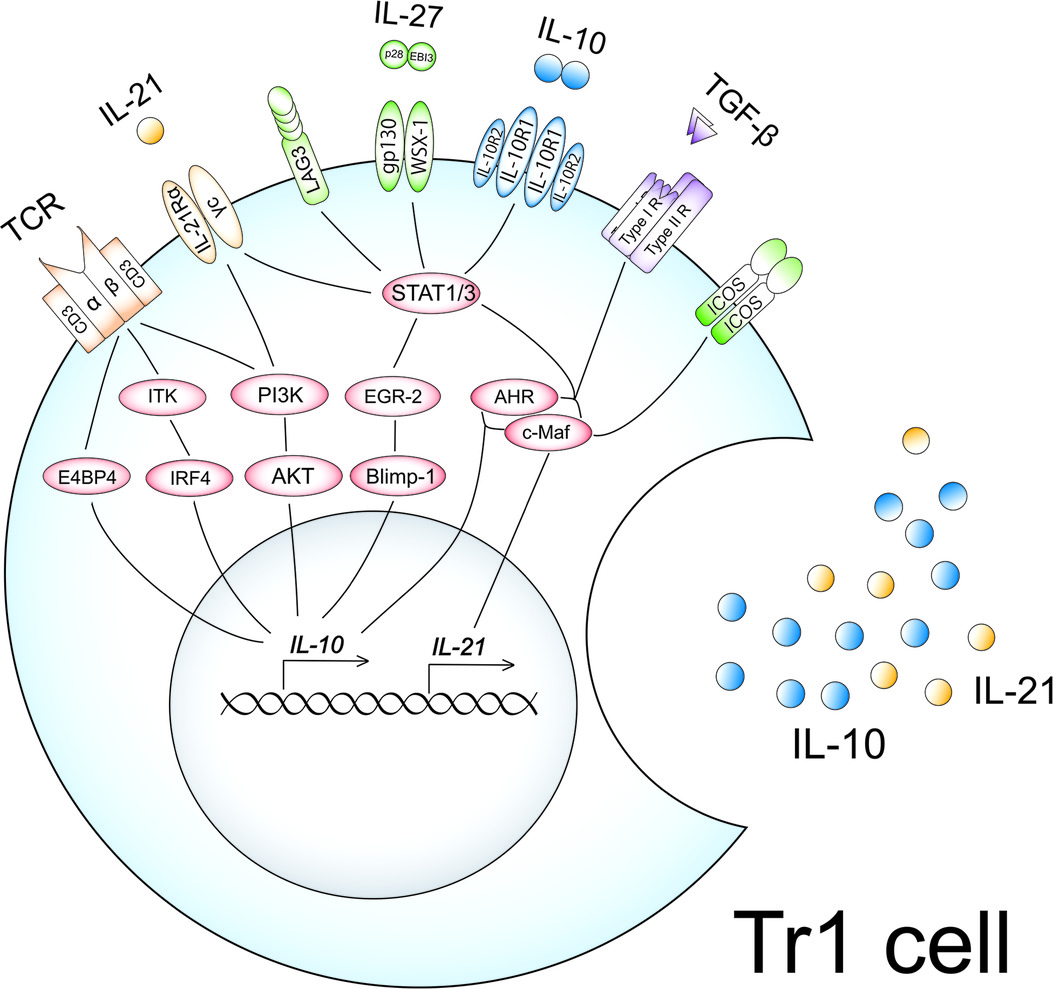
Figure 1. Induction mechanisms of Tr1 cells in AIT. High-dose allergen exposure in AIT promotes dendritic cells to produce IL-27, IL-10, and TGF-β. Naïve CD4+ T cells can differentiate into Tr1 cells upon TCR engagement in the presence of these cytokines. High-dose antigen exposure leads to the upregulation and activation of IL-10-associated molecules such as ITK, E4BP4, IRF4, PI3K, and AKT, followed by the production of IL-10. PI3K also enforces IL-21 receptor expression. IL-27 upregulates and activates IL-10-associated molecules, EGR-2, Blimp-1, AHR, and c-Maf, followed by the production of IL-10 and IL-21 in cooperation with TGF-β via activation of STAT1 and STAT3. IL-27 also upregulates the expression of ICOS and LAG3. The upregulated IL-10, IL-21, and ICOS by IL-27 amplify the productions of IL-10 and IL-21 in an autocrine manner, leading to the acquisition of a Tr1 phenotype.
Several reports (91, 96) demonstrated that antigen-specific stimulation was crucial for the generation of Tr1 cells. We also revealed that the number of Tr1 cells was increased significantly when spleen cells isolated from ovalbumin (OVA)-sensitized mice were cultured with a high concentration of OVA (10−3 g/ml) for 7 days (91). On the other hand, the induction of Tr1 cells was not observed in a culture of spleen cells derived from non-sensitized mice (91). Motomura et al. (96) also reported that chronic antigen-stimulated Th1 cells into IL-10-producing Tr1 cells via up-regulation of a transcription factor of IL-10, E4-binding protein 4 (E4BP4) in mice. One study demonstrated that the expression of E4BP4 on CD4+ T-cell populations was markedly up-regulated after AIT in mice (32). Our group also reported that the expression of E4BP4 mRNA on peripheral blood mononuclear cells in SCIT-treated Japanese cedar pollinosis patients was higher than those in non-SCIT-treated patients (81). These data suggested that antigen-specific stimulation may be essential for the induction of Tr1 cells via upregulation of E4BP4.
IL-2 inducible T cell kinase (ITK) is a non-receptor tyrosine kinase mainly observed in T cells and has a vital role in the TCR signaling (97). ITK signaling is critical for T-cell subset differentiation and the regulation of cytokine gene expression. Huang et al. (98) demonstrated that the induction of Tr1 cells was not observed in the absence of ITK in mice and humans. ITK deficiency impaired interferon regulatory factor 4 (IRF4) expression, leading to the underdevelopment of Tr1 cells in humans and mice. Overexpression of IRF4 rescued the development of these cells in ITK-deficient cells derived from both humans and mice. These findings suggest that ITK signaling components are essential for developing Tr1 cells.
The phosphatidylinositol-3 kinase (PI3K)-protein kinase B (AKT) signaling, which is a downstream of TCR activation, is also involved in the induction and maintenance of Tr1 cells. Once activated, PI3K-AKT-signaling by TCR engagement in the presence of IL-27 resulted in both upregulation of IL-10 and IL-21 receptors on naive CD4+ T cells in mice (99). These upregulated molecules are crucial for the induction and maintenance of Tr1 phenotypes, as described below in this paper. The authors also reported that FoxO1 phosphorylation was dampened in the condition of suppression of the PI3K and AKT phosphorylation, leading to impairment of Tr1 cell differentiation by IL-27 (99). Although the roles of FoxO1 in Tr1 differentiation have not been fully elucidated, FoxO1 was described as a critical molecule for the induction of IL-10, TGF-β, and CTLA-4 on CD4+ T cells in mice (100, 101). These reports suggested that PI3K-AKT-FoxO1 signaling could be crucial for the acquisition of Tr1 cell phenotypes.
IL-27 is a pleiotropic cytokine that is a heterodimer composed of Epstein-Barr virus-induced gene 3 (Ebi3) and IL-27 p28 (102). IL-27 is mainly produced by antigen-presenting cells upon Toll-like receptor stimulation (102, 103) or chronic antigen stimulation (104). IL-27 binds to the IL-27 receptor, a heterodimer composed of the orphan cytokine receptor WSX-1 and a signal transducing chain, glycoprotein 130 (gp130) (105). IL-27 induces the activation of STAT1 and STAT3, leading to the up-regulation of transcription factors such as c-Maf and aryl hydrocarbon receptor (AHR). c-Maf (106) and AHR (107) directly transactivated IL-10 gene expression through binding to a xenobiotic response element motif and a c-Maf recognition element motif in the IL-10 promoter, respectively. The interaction of AHR with c-Maf synergistically induces not only IL-10 but also IL-21 production, which is the hallmark of Tr1 cells (108). IL-27 also induces early growth response gene 2 (Egr-2) expression in naïve CD4+ T cells via activation of STAT3 (109). Egr-2 has been reported to be the transcription factor that binds to the B lymphocyte-induced maturation protein-1 (Blimp-1) promoter, leading to the upregulation of Blimp-1 (109). Blimp-1 binds to intron 1 of the IL10 locus, leading to the production of IL-10 from mouse CD4+ CD25+ Treg cells (110). Egr-2 also enforces the expression of lymphocyte activation gene 3 (LAG-3) (109). Moreover, the expression of inducible T-cell co-stimulator (ICOS) was increased in IL-27-stimulated naïve CD4+ T cells (111). ICOS is a coreceptor molecule, a member of the CD28 family, that is induced in activated T cells (112). The expression of ICOS-ligand (ICOS-L) is found in dendritic cells, macrophages, and CD4+ T cells, and the expression is further amplified upon activation of these cells (113, 114). ICOS induced the upregulation of c-Maf, followed by the production of IL-10 and IL-21 (115). Therefore, IL-27 is important for initiating the differentiation of Tr1 cells via up-regulation of STAT1 and STAT3.
The produced IL-10 and IL-21 from induced Tr1 cells are involved in the maintenance of phenotypes of Tr1 cells in an autocrine manner. As mentioned above, IL-10 is an anti-inflammatory cytokine that forms a homodimer and exerts its function through binding to its receptor. The IL-10 receptor consists of two subunits of IL-10R1 and two subunits of IL-10 receptor 2 (IL-10R2) (116). IL-10 only binds to IL-10R1 but not IL-10R2 (117). The IL-10 binds to its receptor, followed by the activation of STAT1 and STAT3. IL-21 is a potent immunomodulatory four-alpha-helical bundle type I cytokine that has pleiotropic roles in the regulation of T-cells, B-cells, natural killer cells, and myeloid cells (118). The functional receptor of IL-21 is composed of the IL-21Rα chain and the common cytokine receptor γc chain (119). IL-21 binds to its receptor, leading to the activation of STAT3. STAT3 activated by IL-10 and IL-21 also enforces the expression of c-Maf, leading to the maintenance of Tr1 phenotypes. TGF-β is also an immunomodulatory cytokine that exacerbates the induction of Tr1 cells via upregulation of c-Maf and AHR in the presence of IL-27 (106, 108, 120).
Our group (91, 92) also demonstrated that the stimulation of allergens, IL-21, IL-27, and TGF-β of CD4+ T cells have crucial roles in the induction of allergen-specific Tr1 cells in mice. Spleen cells isolated from OVA-sensitized mice were cultured in the presence of OVA, IL-21, IL-27, and TGF-β for 7 days. After 7 days of culture, a significant increase in Foxp3-negative IL-10-producing CD4+ T cells was observed in the culture. Moreover, most of the induced Foxp3-negative IL-10-producing CD4+ T cells were double positive for CD49b and LAG3, which are surface markers of Tr1 cells. Therefore, the antigen-presenting environment in the presence of IL-21, IL-27, and TGF-β may be a suitable condition for the induction of allergen-specific Tr1 cells.
In recent years, Zissler et al. (121) reported that AIT induced upregulations of secretoglobin1A1 (SCGB1A1) and IL-7 in the airway of patients with allergic rhinitis. These factors are produced by airway epithelial cells (121, 122) and could be involved in the induction of Tr1 cells. SCGB1A1 is mainly produced by airway club cells, which are a kind of epithelial cells (123). Mandal et al. (124) demonstrated that SCGB1A1 down-regulated cyclooxygenase-2 gene expression in airway epithelial cells of asthmatic mice, followed by decreases in the production of prostaglandins (PGs). Hooper et al. (125) reported that PGE2 inhibited Tr1 cell differentiation by suppressing IL-27 production from dendritic cells in mice. These data suggested that SCGB1A1 is associated with the induction of Tr1 cells via suppressing the production of PGE2 at inflammatory milieus. IL-7 is a cytokine that is required for the development and maintenance of most major subsets of T cells (126). IL-7 also supports Tr1 cell proliferation in cooperation with IL-2 and IL-15 (127). Therefore, AIT could modulate the phenotypes of epithelial cells, leading to the induction of Tr1 cells in the inflamed tissues.
RNA interference by microRNA (miRNA) has also been associated with the mechanisms of AIT. Jakwerth et al. (128) demonstrated that the expression of miR-3595, whose target is PTGER, prostaglandin EP3 receptor, was markedly up-regulated in the sputum of AIT-treated patients with allergic rhinitis. Moreover, it was reported that the level of its ligand PGE2 in the sputum was decreased by AIT-treatment (128). As mentioned above, PGE2 signaling suppresses the induction of Tr1 cells. These data suggested that AIT could suppress the PGE2-EP3 axis via a decrease in PGE2 production and downregulation of EP3, leading to the induction of Tr1 cells.
Extracellular matrix components such as hyaluronan (HA) are also involved in the induction and maintenance of Tr1 cells. The biological functions of HA are dependent on its size (129). Although the low molecular weight of HA promotes antigen presentation and allergic responses, intact high molecular weight HA (HMW-HA) has anti-inflammatory properties and contributes to healing tissues (129). Bollyky et al. (130) demonstrated that HMW-HA promoted the induction of Tr1 cells from effector memory T-cell precursors via binding its receptor, CD44, in humans and mice. The produced IL-10 from Tr1 cells also enforces HMW-HA production from fibroblast via activating the STAT3-dependent signaling pathway in mice (131, 132). Therefore, the crosstalk of Tr1 cells and extracellular matrix such as HMW-HA could be essential for wound healing in inflamed tissues. The combination SLIT with HMW-HA markedly suppressed the development of airway hyperresponsiveness in comparison with the only SLIT-treated asthmatic mice (133). Gebe et al. (134) also reported that modified HMW-HA, which was thiolated and tethered to OVA via thiol linkages, enforced allergen-specific immune tolerance via IL-10 upregulation in the lungs of OVA-induced asthmatic model of mice. These data imply that HMW-HA is an attractive adjuvant for AIT.
Immunosuppressive roles of Tr1 cells
Tr1 cells need to be activated by specific antigen recognition via their TCR. Once activated by a specific antigen, Tr1 cells can also suppress the activities of other antigen-specific T cells in their proximity. Therefore, Tr1 cells mediate both antigen-specific and antigen-non-specific immune reactions. However, the antigen-non-specific immunosuppression is restricted to the tissue where these cells are activated (135). As immunosuppressive mechanisms by activated Tr1 cells, (1) suppression of effector cells by anti-inflammatory cytokines, (2) down-modulation of antigen-presenting cells by immune checkpoint molecules, (3) cytolysis of effector cells by granzyme B, and (4) metabolic disruption by CD39 and CD73 have been demonstrated (Figure 2). These suppressive mechanisms have been reported both for murine and human Tr1 cells (Table 3).
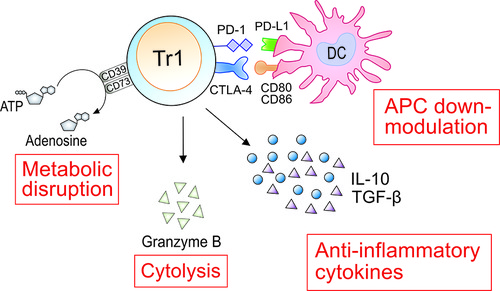
Figure 2. Immunosuppressive roles of Tr1 cells in AIT. As immunosuppressive mechanisms by activated Tr1 cells, (1) suppression of effector cells by anti-inflammatory cytokines, (2) down-modulation of antigen-presenting cells (APCs) by immune checkpoint molecules, (3) cytolysis of effector cells by granzyme B, and (4) metabolic disruption by CD39 and CD73 have been clarified. IL-10 can suppress the production of IL-5 and IL-13 from Th2 and ILC2. IL-10 and TGF-β downregulate the expression of major histocompatibility complex class II molecules and co-stimulatory molecules such as CD80 and CD86, and the production of pro-inflammatory cytokines by antigen-presenting cells, followed by suppression of activation of effector T cells. Tr1 cells suppress the functions of Th2 by down-modulating APCs through expression of CTLA-4 and PD-1. Granzyme B produced from Tr1 cells induces apoptosis of the interacted APCs. Adenosine produced by CD39 and CD73-expressed on Tr1 cells restrained the functions of Th2 cells and ILC2. The induced Tr1 cells by AIT are involved in the suppression of allergic symptoms using these four mechanisms.
Upon being activated by a specific antigen, Tr1 cells produce large amounts of anti-inflammatory cytokines, especially IL-10 and TGF-β in both mice and humans (47). We previously demonstrated that the adoptive transfer of Tr1 cells significantly suppressed the development of airway hyperresponsiveness and increased levels of eosinophils and neutrophils in the lung via the production of a large amount of IL-10 in an asthmatic model in mice (91, 92). Other studies (136, 137) demonstrated that IL-10 significantly inhibited the upregulation of vascular adhesion molecule-1 (VCAM-1) and intercellular adhesion molecule-1 (ICAM-1) on endothelial cells and leukocyte adhesion to endothelium. Our group also demonstrated that the intratracheal administration of IL-10 suppressed the infiltration of eosinophils and neutrophils into the lung via down-regulation of the expression of VCAM-1 and ICAM-1 on pulmonary vascular endothelial cells in severely asthmatic mice (138). IL-10 also induces the downregulation of the expression of major histocompatibility complex class II molecules and co-stimulatory molecules such as CD80 and CD86, and the production of pro-inflammatory cytokines by antigen-presenting cells, followed by suppression of activation of effector T cells (139). IL-10 can also directly suppress the production of type 2 cytokines such as IL-4, IL-5, and IL-13 from Th2 cells (140) and group 2 innate lymphoid cells (ILC2) (141). TGF-β also down-regulates the expression of CD80 and CD86 on dendritic cells, followed by inhibition of the interaction with effector T cells (142). In recent years, Branchett et al. (143) demonstrated that TGF-β suppressed the production of CCL8, a chemokine of Th2 and ILC2, from alveolar macrophages in a house dust mite-induced allergic airway inflammation model in mice. In addition to suppressing the functions of effector cells, TGF-β can elicit Foxp3 expression in naïve CD4+ T cells (144). However, TGF-β inversely induces the differentiation of naïve CD4+ T cells into Th9 cells (145) and Th17 cells (146) in the presence of pro-inflammatory cytokines, such as IL-4 and IL-6. Even upon allergen exposure, TGF-β1 was described as a crucial accelerator of induction of Th2, Th9 and Th17 cells in a recent publication (147). On the other hand, we previously demonstrated that TGF-β1 amplified the differentiation of Tr1 cells upon allergen exposure in cooperation with IL-27 and IL-21 in mice (91). Moreover, Th17 cell induction was not observed in this culture condition (91). As mentioned above, large amounts of IL-21, IL-27 and TGF-β1 could be produced in the milieu of Tr1 induction by AIT. Therefore, TGF-β1 may be associated with Tr1 induction rather than inflammatory Th cell differentiation in AIT.
On the other hand, many studies (51, 148–150) reported that the addition of neutralizing antibodies against IL-10 and TGF-β did not completely abrogate the suppressive functions of human Tr1 cells. These data suggested that a cell contact-dependent mechanism was associated with inhibiting immune responses by Tr1 cells. Magnani et al. (151) demonstrated that human Tr1 cells were activated by binding CD54, CD58, and CD155 on antigen-presenting cells via their own expression of lymphocyte function-associated antigen 1, CD2, and CD226, respectively, followed by the production of granzyme B. The produced granzyme B from Tr1 cells induces apoptosis of the interacting antigen-presenting cells. This cytolysis of antigen-presenting cells leads to suppression of both antigen-specific CD4+ T cells and CD8+ T cells, and non-specific T cells (152). In mice, although the expression of granzyme B on Tr1 cells was reported (153), the contribution to the immunosuppressive ability of Tr1 cells has not been elucidated.
Tr1 cells can also inhibit the activation of effector cells via binding immune checkpoint molecules such as PD-1 and CTLA-4. Chen et al. (154) demonstrated that blocking CTLA-4 or PD-1/ programmed cell death ligand 1 almost completely abolished human Tr1 cell-mediated inhibition of effector T cell proliferation. Akdis et al. (74) also reported that human Tr1 cells suppressed the production of IL-13 from Der p 1 or Bet v 1-specific Th2 by down-modulation of antigen-presenting cells through expressions of CTLA-4 and PD-1 on Tr1 cells. In mice, the expression of CTLA-4 (155, 156) and PD-1 (66, 157) on Tr1 cells has also been reported in mice, but their contribution to the immunosuppressive potential of Tr1 cells is unclear.
The expression of the ectonucleotidase CD39 and the ecto-5′-nucleotidase CD73 were observed on the surface of human Tr1 cells (158–160). In mice, the expression of CD39 but not CD73 was observed in mouse Tr1 cells (161). The extracellular adenosine triphosphates produced in inflammatory environments are sequentially hydrolyzed to 5′-adenosine monophosphate (AMP) and then to adenosine by CD39 and CD73 (162, 163). Adenosine binds to A1, A2a, A2b, and A3 receptors (R), which are expressed on the surface of various immune cells and non-immune cells (164). In immune cells, adenosine mainly binds to A2aR and A2bR, followed by suppressing the functions via upregulation of intercellular cAMP (165, 166). Csoka et al. (167) demonstrated that the interaction of adenosine with A2aR suppressed the development of Th2 cells from naïve CD4+ T cells in mice. Moreover, Csoka's group (168) reported that A2bR had suppressive roles in the production of IL-5 and IL-13 from ILC2 in mice. Xiao et al. (169) also demonstrated that adenosine restrained ILC2-driven allergic airway inflammation via binding to A2aR in mice.
Conclusion
Since the discovery of Tr1 cells, our understanding of the mechanisms of AIT has expanded through the constant efforts of many groups. As for the induction mechanisms of Tr1 cells in AIT, it has been clarified that three key events are essential for the induction and maintenance of these cells: (1) antigen presentation, (2) the production of IL-10, IL-27, and TGF-β from high-dose allergen-stimulated dendritic cells, and (3) the production of IL-10 and IL-21 from the induced Tr1 cells in an autocrine manner. When the induced Tr1 cells are reactivated by the specific antigen, the cells suppress effector cells by using anti-inflammatory cytokines, immune checkpoint molecules, granzyme B, and ectoenzymes such as CD39 and CD73. However, the master regulator of these cells is still unclear. The discovery of the master regulator of Tr1 cells may lead to the development of more-effective AIT. Therefore, understanding the biology of Tr1 cells should continue to be expanded.
Author contributions
MM, TT, KK, RK and TN wrote and revised the manuscript. All authors contributed to the article and approved the submitted version.
Funding
The present study was supported by research funding from JSPS KAKENHI grant number 17K11370 and 19K16385 (to Tetsuya Terada and Takeshi Nabe, and Masaya Matsuda, respectively), and Setsunan University (to Masaya Matsuda, Kazuyuki Kitatani and Takeshi Nabe).
Conflict of interest
Takeshi Nabe is an external evaluation committee of Ono Pharmaceutical Co., Ltd. (Osaka, Japan).
Publisher's note
All claims expressed in this article are solely those of the authors and do not necessarily represent those of their affiliated organizations, or those of the publisher, the editors and the reviewers. Any product that may be evaluated in this article, or claim that may be made by its manufacturer, is not guaranteed or endorsed by the publisher.
References
1. Boonpiyathad T, Sözener ZC, Satitsuksanoa P, Akdis CA. Immunologic mechanisms in asthma. Semin Immunol. (2019) 46:101333. doi: 10.1016/j.smim.2019.101333
2. Zissler UM, Chaker AM, Effner R, Ulrich M, Guerth F, Piontek G, et al. Interleukin-4 and interferon-γ orchestrate an epithelial polarization in the airways. Mucosal Immunol. (2016) 9:917–26. doi: 10.1038/mi.2015.110
3. Kohan M, Puxeddu I, Reich R, Levi-Schaffer F, Berkman N. Eotaxin-2/CCL24 and eotaxin-3/CCL26 exert differential profibrogenic effects on human lung fibroblasts. Ann Allergy Asthma Immunol. (2010) 104:66–72. doi: 10.1016/j.anai.2009.11.003
4. Agache I, Eguiluz-Gracia I, Cojanu C, Laculiceanu A, Del Giacco S, Zemelka-Wiacek M, et al. Advances and highlights in asthma in 2021. Allergy. (2021) 76:3390–407. doi: 10.1111/all.15054
5. Bakakos A, Schleich F, Bakakos P. Biological therapy of severe asthma and nasal polyps. J Pers Med. (2022) 12:976. doi: 10.3390/jpm12060976
6. Mazur M, Czarnobilska M, Dyga W, Czarnobilska E. Trends in the epidemiology of allergic diseases of the airways in children growing up in an urban agglomeration. J Clin Med Res. (2022) 11:2188. doi: 10.3390/jcm11082188
7. Zhang Y, Zhang L. Increasing prevalence of allergic rhinitis in China. Allergy Asthma Immunol Res. (2019) 11:156–69. doi: 10.4168/aair.2019.11.2.156
8. Jutel M, Agache I, Bonini S, Burks AW, Calderon M, Canonica W, et al. International consensus on allergy immunotherapy. J Allergy Clin Immunol. (2015) 136:556–68. doi: 10.1016/j.jaci.2015.04.047
9. Noon L. Prophylactic inoculation against hay fever. Lancet. (1911) 177:1572–3. doi: 10.1016/S0140-6736(00)78276-6
10. Passalacqua G, Bagnasco D, Canonica GW. 30 Years of sublingual immunotherapy. Allergy. (2020) 75:1107–20. doi: 10.1111/all.14113
11. Roberts G, Pfaar O, Akdis CA, Ansotegui IJ, Durham SR, Gerth van Wijk R, et al. EAACI Guidelines on allergen immunotherapy: allergic rhinoconjunctivitis. Allergy. (2018) 73:765–98. doi: 10.1111/all.13317
12. Radulovic S, Calderon MA, Wilson D, Durham S. Sublingual immunotherapy for allergic rhinitis. Cochrane Database Syst Rev. (2010) 2010:CD002893. doi: 10.1002/14651858.CD002893.pub2
13. Calderon MA, Alves B, Jacobson M, Hurwitz B, Sheikh A, Durham S. Allergen injection immunotherapy for seasonal allergic rhinitis. Cochrane Database Syst Rev. (2007) 2007:CD001936. doi: 10.1002/14651858.CD001936.pub2
14. Abramson MJ, Puy RM, Weiner JM. Injection allergen immunotherapy for asthma. Cochrane Database Syst Rev. (2010) 2010:CD001186. doi: 10.1002/14651858.CD001186.pub2
15. Fortescue R, Kew KM, Leung MST. Sublingual immunotherapy for asthma. Cochrane Database Syst Rev. (2020) 9:CD011293. doi: 10.1002/14651858.CD011293.pub3
16. Möller C, Dreborg S, Ferdousi HA, Halken S, Høst A, Jacobsen L, et al. Pollen immunotherapy reduces the development of asthma in children with seasonal rhinoconjunctivitis (the PAT-study). J Allergy Clin Immunol. (2002) 109:251–6. doi: 10.1067/mai.2002.121317
17. Pajno GB, Barberio G, De Luca F, Morabito L, Parmiani S. Prevention of new sensitizations in asthmatic children monosensitized to house dust mite by specific immunotherapy. A six-year follow-up study. Clin Exp Allergy. (2001) 31:1392–7. doi: 10.1046/j.1365-2222.2001.01161.x
18. Marogna M, Spadolini I, Massolo A, Canonica GW, Passalacqua G. Long-lasting effects of sublingual immunotherapy according to its duration: a 15-year prospective study. J Allergy Clin Immunol. (2010) 126:969–75. doi: 10.1016/j.jaci.2010.08.030
19. Scadding GW, Calderon MA, Shamji MH, Eifan AO, Penagos M, Dumitru F, et al. Effect of 2 years of treatment with sublingual grass pollen immunotherapy on nasal response to allergen challenge at 3 years among patients with moderate to severe seasonal allergic rhinitis: the GRASS randomized clinical trial. JAMA. (2017) 317:615–25. doi: 10.1001/jama.2016.21040
20. Di Lorenzo G, Mansueto P, Pacor ML, Rizzo M, Castello F, Martinelli N, et al. Evaluation of serum s-IgE/total IgE ratio in predicting clinical response to allergen-specific immunotherapy. J Allergy Clin Immunol. (2009) 123:1103–10, 1110.e1–4. doi: 10.1016/j.jaci.2009.02.012
21. Manzotti G, Lombardi C. Allergen immunotherapy as a drug: the new deal of grass allergen tablets from clinical trials to current practice. Eur Ann Allergy Clin Immunol. (2013) 45:34–42.23821831
22. Bernstein DI, Wanner M, Borish L, Liss GM, Immunotherapy Committee, American Academy of Allergy, Asthma and Immunology. Twelve-year survey of fatal reactions to allergen injections and skin testing: 1990–2001. J Allergy Clin Immunol. (2004) 113:1129–36. doi: 10.1016/j.jaci.2004.02.006
23. Cox L, Larenas-Linnemann D, Lockey RF, Passalacqua G. Speaking the same language: the world allergy organization subcutaneous immunotherapy systemic reaction grading system. J Allergy Clin Immunol. (2010) 125:569–74, 574.e1–574.e7. doi: 10.1016/j.jaci.2009.10.060
24. van de Veen W, Stanic B, Wirz OF, Jansen K, Globinska A, Akdis M. Role of regulatory B cells in immune tolerance to allergens and beyond. J Allergy Clin Immunol. (2016) 138:654–65. doi: 10.1016/j.jaci.2016.07.006
25. Eljaszewicz A, Ruchti F, Radzikowska U, Globinska A, Boonpiyathad T, Gschwend A, et al. Trained immunity and tolerance in innate lymphoid cells, monocytes, and dendritic cells during allergen-specific immunotherapy. J Allergy Clin Immunol. (2021) 147:1865–77. doi: 10.1016/j.jaci.2020.08.042
26. Golebski K, Layhadi JA, Sahiner U, Steveling-Klein EH, Lenormand MM, Li RCY, et al. Induction of IL-10-producing type 2 innate lymphoid cells by allergen immunotherapy is associated with clinical response. Immunity. (2021) 54:291–307.e7. doi: 10.1016/j.immuni.2020.12.013
27. Zissler UM, Jakwerth CA, Guerth FM, Pechtold L, Aguilar-Pimentel JA, Dietz K, et al. Early IL-10 producing B-cells and coinciding Th/Tr17 shifts during three year grass-pollen AIT. EBioMedicine. (2018) 36:475–88. doi: 10.1016/j.ebiom.2018.09.016
28. Yao Y, Chen C-L, Yu D, Liu Z. Roles of follicular helper and regulatory T cells in allergic diseases and allergen immunotherapy. Allergy. (2021) 76:456–70. doi: 10.1111/all.14639
29. Durham SR, Ying S, Varney VA, Jacobson MR, Sudderick RM, Mackay IS, et al. Grass pollen immunotherapy inhibits allergen-induced infiltration of CD4+ T lymphocytes and eosinophils in the nasal mucosa and increases the number of cells expressing messenger RNA for interferon-gamma. J Allergy Clin Immunol. (1996) 97:1356–65. doi: 10.1016/S0091-6749(96)70205-1
30. Liu G, Liu M, Wang J, Mou Y, Che H. The role of regulatory T cells in epicutaneous immunotherapy for food allergy. Front Immunol. (2021) 12:660974. doi: 10.3389/fimmu.2021.660974
31. Okano M, Otsuki N, Azuma M, Fujiwara T, Kariya S, Sugata Y, et al. Allergen-specific immunotherapy alters the expression of B and T lymphocyte attenuator, a co-inhibitory molecule, in allergic rhinitis. Clin Exp Allergy. (2008) 38:1891–900. doi: 10.1111/j.1365-2222.2008.03116.x
32. Burton BR, Britton GJ, Fang H, Verhagen J, Smithers B, Sabatos-Peyton CA, et al. Sequential transcriptional changes dictate safe and effective antigen-specific immunotherapy. Nat Commun. (2014) 5:4741. doi: 10.1038/ncomms5741
33. Wambre E. Effect of allergen-specific immunotherapy on CD4+ T cells. Curr Opin Allergy Clin Immunol. (2015) 15:581–7. doi: 10.1097/ACI.0000000000000216
34. Brooks DG, Trifilo MJ, Edelmann KH, Teyton L, McGavern DB, Oldstone MBA. Interleukin-10 determines viral clearance or persistence in vivo. Nat Med. (2006) 12:1301–9. doi: 10.1038/nm1492
35. Tinoco R, Alcalde V, Yang Y, Sauer K, Zuniga EI. Cell-intrinsic transforming growth factor-beta signaling mediates virus-specific CD8+ T cell deletion and viral persistence in vivo. Immunity. (2009) 31:145–57. doi: 10.1016/j.immuni.2009.06.015
36. Wang S-H, Zissler UM, Buettner M, Heine S, Heldner A, Kotz S, et al. An exhausted phenotype of TH 2 cells is primed by allergen exposure, but not reinforced by allergen-specific immunotherapy. Allergy. (2021) 76:2827–39. doi: 10.1111/all.14896
37. Niederlova V, Tsyklauri O, Chadimova T, Stepanek O. CD8+ Tregs revisited: a heterogeneous population with different phenotypes and properties. Eur J Immunol. (2021) 51:512–30. doi: 10.1002/eji.202048614
38. Fischer K, Voelkl S, Heymann J, Przybylski GK, Mondal K, Laumer M, et al. Isolation and characterization of human antigen-specific TCR alpha beta+ CD4(-)CD8- double-negative regulatory T cells. Blood. (2005) 105:2828–35. doi: 10.1182/blood-2004-07-2583
39. Wesch D, Peters C, Siegers GM. Human gamma delta T regulatory cells in cancer: fact or fiction? Front Immunol. (2014) 5:598. doi: 10.3389/fimmu.2014.00598
40. Weiss JM, Bilate AM, Gobert M, Ding Y, de Lafaille MA C, Parkhurst CN, et al. Neuropilin 1 is expressed on thymus-derived natural regulatory T cells, but not mucosa-generated induced Foxp3+ T reg cells. J Exp Med. (2012) 209:1723–42, S1. doi: 10.1084/jem.20120914
41. Yadav M, Louvet C, Davini D, Gardner JM, Martinez-Llordella M, Bailey-Bucktrout S, et al. Neuropilin-1 distinguishes natural and inducible regulatory T cells among regulatory T cell subsets in vivo. J Exp Med. (2012) 209:1713–22, S1–19. doi: 10.1084/jem.20120822
42. Thornton AM, Korty PE, Tran DQ, Wohlfert EA, Murray PE, Belkaid Y, et al. Expression of Helios, an Ikaros transcription factor family member, differentiates thymic-derived from peripherally induced Foxp3+ T regulatory cells. J Immunol. (2010) 184:3433–41. doi: 10.4049/jimmunol.0904028
43. Miyara M, Yoshioka Y, Kitoh A, Shima T, Wing K, Niwa A, et al. Functional delineation and differentiation dynamics of human CD4+ T cells expressing the FoxP3 transcription factor. Immunity. (2009) 30:899–911. doi: 10.1016/j.immuni.2009.03.019
44. Giganti G, Atif M, Mohseni Y, Mastronicola D, Grageda N, Povoleri GA, et al. Treg cell therapy: how cell heterogeneity can make the difference. Eur J Immunol. (2021) 51:39–55. doi: 10.1002/eji.201948131
45. Kim YC, Bhairavabhotla R, Yoon J, Golding A, Thornton AM, Tran DQ, et al. Oligodeoxynucleotides stabilize Helios-expressing Foxp3+ human T regulatory cells during in vitro expansion. Blood. (2012) 119:2810–8. doi: 10.1182/blood-2011-09-377895
46. Feuerer M, Hill JA, Kretschmer K, von Boehmer H, Mathis D, Benoist C. Genomic definition of multiple ex vivo regulatory T cell subphenotypes. Proc Natl Acad Sci USA. (2010) 107:5919–24. doi: 10.1073/pnas.1002006107
47. Gagliani N, Magnani CF, Huber S, Gianolini ME, Pala M, Licona-Limon P, et al. Coexpression of CD49b and LAG-3 identifies human and mouse T regulatory type 1 cells. Nat Med. (2013) 19:739–46. doi: 10.1038/nm.3179
48. Hori S. FOXP3 As a master regulator of Treg cells. Nat Rev Immunol. (2021) 21:618–9. doi: 10.1038/s41577-021-00598-9
49. Roncarolo MG, Yssel H, Touraine JL, Bacchetta R, Gebuhrer L, De Vries JE, et al. Antigen recognition by MHC-incompatible cells of a human mismatched chimera. J Exp Med. (1988) 168:2139–52. doi: 10.1084/jem.168.6.2139
50. Vieira P, de Waal-Malefyt R, Dang MN, Johnson KE, Kastelein R, Fiorentino DF, et al. Isolation and expression of human cytokine synthesis inhibitory factor cDNA clones: homology to Epstein-Barr virus open reading frame BCRFI. Proc Natl Acad Sci USA. (1991) 88:1172–6. doi: 10.1073/pnas.88.4.1172
51. Bacchetta R, Bigler M, Touraine JL, Parkman R, Tovo PA, Abrams J, et al. High levels of interleukin 10 production in vivo are associated with tolerance in SCID patients transplanted with HLA mismatched hematopoietic stem cells. J Exp Med. (1994) 179:493–502. doi: 10.1084/jem.179.2.493
52. Groux H, O’Garra A, Bigler M, Rouleau M, Antonenko S, de Vries JE, et al. A CD4+ T-cell subset inhibits antigen-specific T-cell responses and prevents colitis. Nature. (1997) 389:737–42. doi: 10.1038/39614
53. Levings MK, Gregori S, Tresoldi E, Cazzaniga S, Bonini C, Roncarolo MG. Differentiation of Tr1 cells by immature dendritic cells requires IL-10 but not CD25+CD4+ Tr cells. Blood. (2005) 105:1162–9. doi: 10.1182/blood-2004-03-1211
54. Oida T, Zhang X, Goto M, Hachimura S, Totsuka M, Kaminogawa S, et al. CD4+CD25- T cells that express latency-associated peptide on the surface suppress CD4+CD45RBhigh-induced colitis by a TGF-beta-dependent mechanism. J Immunol. (2003) 170:2516–22. doi: 10.4049/jimmunol.170.5.2516
55. Ochi H, Abraham M, Ishikawa H, Frenkel D, Yang K, Basso AS, et al. Oral CD3-specific antibody suppresses autoimmune encephalomyelitis by inducing CD4+ CD25- LAP+ T cells. Nat Med. (2006) 12:627–35. doi: 10.1038/nm1408
56. Groh V, Bruhl A, El-Gabalawy H, Nelson JL, Spies T. Stimulation of T cell autoreactivity by anomalous expression of NKG2D and its MIC ligands in rheumatoid arthritis. Proc Natl Acad Sci U S A. (2003) 100:9452–7. doi: 10.1073/pnas.1632807100
57. Groh V, Smythe K, Dai Z, Spies T. Fas-ligand-mediated paracrine T cell regulation by the receptor NKG2D in tumor immunity. Nat Immunol. (2006) 7:755–62. doi: 10.1038/ni1350
58. Häringer B, Lozza L, Steckel B, Geginat J. Identification and characterization of IL-10/IFN-gamma-producing effector-like T cells with regulatory function in human blood. J Exp Med. (2009) 206:1009–17. doi: 10.1084/jem.20082238
59. Gonzalez A, Andre-Schmutz I, Carnaud C, Mathis D, Benoist C. Damage control, rather than unresponsiveness, effected by protective DX5+ T cells in autoimmune diabetes. Nat Immunol. (2001) 2:1117–25. doi: 10.1038/ni738
60. Charbonnier L-M, van Duivenvoorde LM, Apparailly F, Cantos C, Han WGH, Noël D, et al. Immature dendritic cells suppress collagen-induced arthritis by in vivo expansion of CD49b+ regulatory T cells. J Immunol. (2006) 177:3806–13. doi: 10.4049/jimmunol.177.6.3806
61. Han WGH, Schuurhuis DH, Fu N, Camps M, van Duivenvoorde LM, Louis-Plence P, et al. DC-induced CD8(+) T-cell response is inhibited by MHC class II-dependent DX5(+)CD4(+) Treg. Eur J Immunol. (2009) 39:1765–73. doi: 10.1002/eji.200838842
62. el Bannoudi H, Han WGH, Stoop JN, Louis-Plence P, Huizinga TWJ, Toes REM. DX5+ CD4+ T cells modulate CD4+ T-cell response via inhibition of IL-12 production by DCs. Eur J Immunol. (2013) 43:439–46. doi: 10.1002/eji.201242796
63. Workman CJ, Rice DS, Dugger KJ, Kurschner C, Vignali DAA. Phenotypic analysis of the murine CD4-related glycoprotein, CD223 (LAG-3). Eur J Immunol. (2002) 32:2255–63. doi: 10.1002/1521-4141(200208)32:8<2255::AID-IMMU2255>3.0.CO;2-A
64. Clemente-Casares X, Blanco J, Ambalavanan P, Yamanouchi J, Singha S, Fandos C, et al. Expanding antigen-specific regulatory networks to treat autoimmunity. Nature. (2016) 530:434–40. doi: 10.1038/nature16962
65. Facciotti F, Gagliani N, Häringer B, Alfen JS, Penatti A, Maglie S, et al. IL-10-producing forkhead box protein 3-negative regulatory T cells inhibit B-cell responses and are involved in systemic lupus erythematosus. J Allergy Clin Immunol. (2016) 137:318–21.e5. doi: 10.1016/j.jaci.2015.06.044
66. Alfen JS, Larghi P, Facciotti F, Gagliani N, Bosotti R, Paroni M, et al. Intestinal IFN-γ-producing type 1 regulatory T cells coexpress CCR5 and programmed cell death protein 1 and downregulate IL-10 in the inflamed guts of patients with inflammatory bowel disease. J Allergy Clin Immunol. (2018) 142:1537–47.e8. doi: 10.1016/j.jaci.2017.12.984
67. Huang W, Solouki S, Carter C, Zheng S-G, August A. Beyond type 1 regulatory T cells: co-expression of LAG3 and CD49b in IL-10-producing T cell lineages. Front Immunol. (2018) 9:2625. doi: 10.3389/fimmu.2018.02625
68. Tsuji NM, Mizumachi K, Antigen-specific KJ-I. CD4+CD25+regulatory T cell clones induced in Peyer's Patches. Int Immunol. (2003) 15:525–34. doi: 10.1093/intimm/dxg051
69. Böhm L, Maxeiner J, Meyer-Martin H, Reuter S, Finotto S, Klein M, et al. IL-10 and regulatory T cells cooperate in allergen-specific immunotherapy to ameliorate allergic asthma. J Immunol. (2015) 194:887–97. doi: 10.4049/jimmunol.1401612
70. Matsuda M, Morie Y, Oze H, Doi K, Tsutsumi T, Hamaguchi J, et al. Phenotype analyses of IL-10-producing Foxp3- CD4+ T cells increased by subcutaneous immunotherapy in allergic airway inflammation. Int Immunopharmacol. (2018) 61:297–305. doi: 10.1016/j.intimp.2018.06.014
71. Xu Y-Q, Gao Y-D, Yang J, Guo W. A defect of CD4+CD25+regulatory T cells in inducing interleukin-10 production from CD4+ T cells under CD46 costimulation in asthma patients. J Asthma. (2010) 47:367–73. doi: 10.3109/02770903.2010.481340
72. Meiler F, Zumkehr J, Klunker S, Rückert B, Akdis CA, Akdis M. In vivo switch to IL-10-secreting T regulatory cells in high dose allergen exposure. J Exp Med. (2008) 205:2887–98. doi: 10.1084/jem.20080193
73. Pellerin L, Jenks JA, Chinthrajah S, Dominguez T, Block W, Zhou X, et al. Peanut-specific type 1 regulatory T cells induced in vitro from allergic subjects are functionally impaired. J Allergy Clin Immunol. (2018) 141:202–13.e8. doi: 10.1016/j.jaci.2017.05.045
74. Akdis M, Verhagen J, Taylor A, Karamloo F, Karagiannidis C, Crameri R, et al. Immune responses in healthy and allergic individuals are characterized by a fine balance between allergen-specific T regulatory 1 and T helper 2 cells. J Exp Med. (2004) 199:1567–75. doi: 10.1084/jem.20032058
75. Pacciani V, Gregori S, Chini L, Corrente S, Chianca M, Moschese V, et al. Induction of anergic allergen-specific suppressor T cells using tolerogenic dendritic cells derived from children with allergies to house dust mites. J Allergy Clin Immunol. (2010) 125:727–36. doi: 10.1016/j.jaci.2009.12.004
76. Han D, Wang C, Lou W, Gu Y, Wang Y, Zhang L. Allergen-specific IL-10-secreting type I T regulatory cells, but not CD4(+)CD25(+)Foxp3(+) T cells, are decreased in peripheral blood of patients with persistent allergic rhinitis. Clin Immunol. (2010) 136:292–301. doi: 10.1016/j.clim.2010.03.006
77. Lou H, Huang Y, Ouyang Y, Zhang Y, Xi L, Chu X, et al. Artemisia annua-sublingual immunotherapy for seasonal allergic rhinitis: a randomized controlled trial. Allergy. (2020) 75:2026–36. doi: 10.1111/all.14218
78. Möbs C, Ipsen H, Mayer L, Slotosch C, Petersen A, Würtzen PA, et al. Birch pollen immunotherapy results in long-term loss of Bet v 1–specific TH2 responses, transient TR1 activation, and synthesis of IgE-blocking antibodies. J Allergy Clin Immunol. (2012) 130:1108–16.e6. doi: 10.1016/j.jaci.2012.07.056
79. Lou W, Wang C, Wang Y, Han D, Zhang L. Responses of CD4(+) CD25(+) Foxp3(+) and IL-10-secreting type I T regulatory cells to cluster-specific immunotherapy for allergic rhinitis in children. Pediatr Allergy Immunol. (2012) 23:140–9. doi: 10.1111/j.1399-3038.2011.01249.x
80. Yamanaka K, Yuta A, Kakeda M, Kitagawa H, Ogihara H, Gabazza EC, et al. SLIT Improves cedar pollinosis by restoring IL-10 production from Tr1 and Monocytes∼IL-10 productivity is critical for becoming allergic∼. Allergol Int. (2011) 60:45–51. doi: 10.2332/allergolint.10-OA-0198
81. Matsuda M, Terada T, Tsujimoto N, Morie Y, Ishida T, Takahashi H, et al. Regulatory T and B cells in peripheral blood of subcutaneous immunotherapy-treated Japanese cedar pollinosis patients. Immunotherapy. (2019) 11:473–82. doi: 10.2217/imt-2018-0170
82. Terada T, Matsuda M, Inaba M, Hamaguchi J, Takemoto N, Kikuoka Y, et al. Sublingual immunotherapy for 4 years increased the number of Foxp3+ Treg cells, which correlated with clinical effects. Inflamm Res. (2021) 70:581–9. doi: 10.1007/s00011-021-01460-3
83. Riley-Vargas RC, Gill DB, Kemper C, Liszewski MK, Atkinson JP. CD46: expanding beyond complement regulation. Trends Immunol. (2004) 25:496–503. doi: 10.1016/j.it.2004.07.004
84. Le Buanec H, Gougeon M-L, Mathian A, Lebon P, Dupont J-M, Peltre G, et al. IFN-α and CD46 stimulation are associated with active lupus and skew natural T regulatory cell differentiation to type 1 regulatory T (Tr1) cells. Proc Natl Acad Sci U S A. (2011) 108:18995–9000. doi: 10.1073/pnas.1113301108
85. Astier AL, Meiffren G, Freeman S, Hafler DA. Alterations in CD46-mediated Tr1 regulatory T cells in patients with multiple sclerosis. J Clin Invest. (2006) 116:3252–7. doi: 10.1172/JCI29251
86. Ni Choileain S, Hay J, Thomas J, Williams A, Vermeren MM, Benezech C, et al. TCR-stimulated changes in cell surface CD46 expression generate type 1 regulatory T cells. Sci Signal. (2017) 10:eaah6163. doi: 10.1126/scisignal.aah6163
87. Farraia M, Paciência I, Castro Mendes F, Cavaleiro Rufo J, Shamji M, Agache I, et al. Allergen immunotherapy for asthma prevention: a systematic review and meta-analysis of randomized and non-randomized controlled studies. Allergy. (2022) 77:1719–35. doi: 10.1111/all.15295
88. Radulovic S, Jacobson MR, Durham SR, Nouri-Aria KT. Grass pollen immunotherapy induces Foxp3-expressing CD4+ CD25+ cells in the nasal mucosa. J Allergy Clin Immunol. (2008) 121:1467–72, 1472.e1. doi: 10.1016/j.jaci.2008.03.013
89. Suárez-Fueyo A, Ramos T, Galán A, Jimeno L, Wurtzen PA, Marin A, et al. Grass tablet sublingual immunotherapy downregulates the TH2 cytokine response followed by regulatory T-cell generation. J Allergy Clin Immunol. (2014) 133:130–8.e1–2. doi: 10.1016/j.jaci.2013.09.043
90. Zhang Y, Li L, Genest G, Zhao W, Ke D, Bartolucci S, et al. Successful milk oral immunotherapy promotes generation of casein-specific CD137+ FOXP3+ regulatory T cells detectable in peripheral blood. Front Immunol. (2021) 12:705615. doi: 10.3389/fimmu.2021.705615
91. Matsuda M, Doi K, Tsutsumi T, Fujii S, Kishima M, Nishimura K, et al. Regulation of allergic airway inflammation by adoptive transfer of CD4+ T cells preferentially producing IL-10. Eur J Pharmacol. (2017) 812:38–47. doi: 10.1016/j.ejphar.2017.06.037
92. Matsuda M, Doi K, Tsutsumi T, Inaba M, Hamaguchi J, Terada T, et al. Adoptive transfer of type 1 regulatory T cells suppressed the development of airway hyperresponsiveness in ovalbumin-induced airway inflammation model mice. J Pharmacol Sci. (2019) 141:139–45. doi: 10.1016/j.jphs.2019.10.004
93. Xian M, Feng M, Dong Y, Wei N, Su Q, Li J. Changes in CD4+CD25+FoxP3+ regulatory T cells and Serum cytokines in sublingual and subcutaneous immunotherapy in allergic rhinitis with or without asthma. Int Arch Allergy Immunol. (2020) 181:71–80. doi: 10.1159/000503143
94. Angelini F, Pacciani V, Corrente S, Silenzi R, Di Pede A, Polito A, et al. Dendritic cells modification during sublingual immunotherapy in children with allergic symptoms to house dust mites. World J Pediatr. (2011) 7:24–30. doi: 10.1007/s12519-011-0242-3
95. Allam J-P, Würtzen PA, Reinartz M, Winter J, Vrtala S, Chen K-W, et al. Phl p 5 resorption in human oral mucosa leads to dose-dependent and time-dependent allergen binding by oral mucosal Langerhans cells, attenuates their maturation, and enhances their migratory and TGF-beta1 and IL-10-producing properties. J Allergy Clin Immunol. (2010) 126:638–45.e1. doi: 10.1016/j.jaci.2010.04.039
96. Motomura Y, Kitamura H, Hijikata A, Matsunaga Y, Matsumoto K, Inoue H, et al. The transcription factor E4BP4 regulates the production of IL-10 and IL-13 in CD4+ T cells. Nat Immunol. (2011) 12:450–9. doi: 10.1038/ni.2020
97. Andreotti AH, Schwartzberg PL, Joseph RE, Berg LJ. T-cell signaling regulated by the Tec family kinase, Itk. Cold Spring Harb Perspect Biol. (2010) 2:a002287. doi: 10.1101/cshperspect.a002287
98. Huang W, Solouki S, Koylass N, Zheng S-G, August A. ITK Signalling via the Ras/IRF4 pathway regulates the development and function of Tr1 cells. Nat Commun. (2017) 8:15871. doi: 10.1038/ncomms15871
99. Nadya NA, Tezuka H, Ohteki T, Matsuda S, Azuma M, Nagai S. PI3K-Akt Pathway enhances the differentiation of interleukin-27-induced type 1 regulatory T cells. Immunology. (2017) 152:507–16. doi: 10.1111/imm.12789
100. Ouyang W, Liao W, Luo CT, Yin N, Huse M, Kim MV, et al. Novel Foxo1-dependent transcriptional programs control T(reg) cell function. Nature. (2012) 491:554–9. doi: 10.1038/nature11581
101. Graves DT, Milovanova TN. Mucosal immunity and the FOXO1 transcription factors. Front Immunol. (2019) 10:2530. doi: 10.3389/fimmu.2019.02530
102. Pflanz S, Timans JC, Cheung J, Rosales R, Kanzler H, Gilbert J, et al. IL-27, a heterodimeric cytokine composed of EBI3 and p28 protein, induces proliferation of naive CD4+ T cells. Immunity. (2002) 16:779–90. doi: 10.1016/S1074-7613(02)00324-2
103. Kong W, Yen J-H, Vassiliou E, Adhikary S, Toscano MG, Ganea D. Docosahexaenoic acid prevents dendritic cell maturation and in vitro and in vivo expression of the IL-12 cytokine family. Lipids Health Dis. (2010) 9:12. doi: 10.1186/1476-511X-9-12
104. Suwanpradid J, Lee MJ, Hoang P, Kwock J, Floyd LP, Smith JS, et al. IL-27 Derived from macrophages facilitates IL-15 production and T cell maintenance following allergic hypersensitivity responses. Front Immunol. (2021) 12:713304. doi: 10.3389/fimmu.2021.713304
105. Caveney NA, Glassman CR, Jude KM, Tsutsumi N, Garcia KC. Structure of the IL-27 quaternary receptor signaling complex. Elife. (2022) 11:e78463. doi: 10.7554/eLife.78463
106. Xu J, Yang Y, Qiu G, Lal G, Wu Z, Levy DE, et al. Ding Y. c-Maf regulates IL-10 expression during Th17 polarization. J Immunol. (2009) 182:6226–36. doi: 10.4049/jimmunol.0900123
107. Gandhi R, Kumar D, Burns EJ, Nadeau M, Dake B, Laroni A, et al. Activation of the aryl hydrocarbon receptor induces human type 1 regulatory T cell-like and Foxp3(+) regulatory T cells. Nat Immunol. (2010) 11:846–53. doi: 10.1038/ni.1915
108. Apetoh L, Quintana FJ, Pot C, Joller N, Xiao S, Kumar D, et al. The aryl hydrocarbon receptor interacts with c-Maf to promote the differentiation of type 1 regulatory T cells induced by IL-27. Nat Immunol. (2010) 11:854–61. doi: 10.1038/ni.1912
109. Iwasaki Y, Fujio K, Okamura T, Yanai A, Sumitomo S, Shoda H, et al. Egr-2 transcription factor is required for Blimp-1-mediated IL-10 production in IL-27-stimulated CD4+ T cells. Eur J Immunol. (2013) 43:1063–73. doi: 10.1002/eji.201242942
110. Cretney E, Xin A, Shi W, Minnich M, Masson F, Miasari M, et al. The transcription factors Blimp-1 and IRF4 jointly control the differentiation and function of effector regulatory T cells. Nat Immunol. (2011) 12:304–11. doi: 10.1038/ni.2006
111. Pot C, Jin H, Awasthi A, Liu SM, Lai C-Y, Madan R, et al. Cutting edge: IL-27 induces the transcription factor c-Maf, cytokine IL-21, and the costimulatory receptor ICOS that coordinately act together to promote differentiation of IL-10-producing Tr1 cells. J Immunol. (2009) 183:797–801. doi: 10.4049/jimmunol.0901233
112. Hutloff A, Dittrich AM, Beier KC, Eljaschewitsch B, Kraft R, Anagnostopoulos I, et al. ICOS Is an inducible T-cell co-stimulator structurally and functionally related to CD28. Nature. (1999) 397:263–6. doi: 10.1038/16717
113. Subudhi SK, Alegre M-L, Fu Y-X. The balance of immune responses: costimulation verse coinhibition. J Mol Med. (2005) 83:193–202. doi: 10.1007/s00109-004-0617-1
114. Ling V, Wu PW, Finnerty HF, Bean KM, Spaulding V, Fouser LA, et al. Cutting edge: identification of GL50, a novel B7-like protein that functionally binds to ICOS receptor. J Immunol. (2000) 164:1653–7. doi: 10.4049/jimmunol.164.4.1653
115. Bauquet AT, Jin H, Paterson AM, Mitsdoerffer M, Ho I-C, Sharpe AH, et al. The costimulatory molecule ICOS regulates the expression of c-Maf and IL-21 in the development of follicular T helper cells and TH-17 cells. Nat Immunol. (2009) 10:167–75. doi: 10.1038/ni.1690
116. Ding Y, Qin L, Zamarin D, Kotenko SV, Pestka S, Moore KW, et al. Differential IL-10R1 expression plays a critical role in IL-10-mediated immune regulation. J Immunol. (2001) 167:6884–92. doi: 10.4049/jimmunol.167.12.6884
117. Zhu L, Shi T, Zhong C, Wang Y, Chang M, Liu X. IL-10 and IL-10 receptor mutations in very early onset inflammatory bowel disease. Gastroenterol Res Pract. (2017) 10:65–9. doi: 10.14740/gr740w
118. Spolski R, Leonard WJ. Interleukin-21: basic biology and implications for cancer and autoimmunity. Annu Rev Immunol. (2008) 26:57–79. doi: 10.1146/annurev.immunol.26.021607.090316
119. Parrish-Novak J, Dillon SR, Nelson A, Hammond A, Sprecher C, Gross JA, et al. Interleukin 21 and its receptor are involved in NK cell expansion and regulation of lymphocyte function. Nature. (2000) 408:57–63. doi: 10.1038/35040504
120. Veldhoen M, Hirota K, Westendorf AM, Buer J, Dumoutier L, Renauld J-C, et al. The aryl hydrocarbon receptor links TH17-cell-mediated autoimmunity to environmental toxins. Nature. (2008) 453:106–9. doi: 10.1038/nature06881
121. Zissler UM, Jakwerth CA, Guerth F, Lewitan L, Rothkirch S, Davidovic M, et al. Allergen-specific immunotherapy induces the suppressive secretoglobin 1A1 in cells of the lower airways. Allergy. (2021) 76:2461–74. doi: 10.1111/all.14756
122. Jin J-O, Yu Q. Systemic administration of TLR3 agonist induces IL-7 expression and IL-7-dependent CXCR3 ligand production in the lung. J Leukoc Biol. (2013) 93:413–25. doi: 10.1189/jlb.0712360
123. Rawlins EL, Okubo T, Xue Y, Brass DM, Auten RL, Hasegawa H, et al. The role of Scgb1a1+ Clara cells in the long-term maintenance and repair of lung airway, but not alveolar, epithelium. Cell Stem Cell. (2009) 4:525–34. doi: 10.1016/j.stem.2009.04.002
124. Mandal AK, Zhang Z, Ray R, Choi MS, Chowdhury B, Pattabiraman N, et al. Uteroglobin represses allergen-induced inflammatory response by blocking PGD2 receptor-mediated functions. J Exp Med. (2004) 199:1317–30. doi: 10.1084/jem.20031666
125. Hooper KM, Yen J-H, Kong W, Rahbari KM, Kuo P-C, Gamero AM, et al. Prostaglandin E2 inhibition of IL-27 production in murine dendritic cells: a novel mechanism that involves IRF1. J Immunol. (2017) 198:1521–30. doi: 10.4049/jimmunol.1601073
126. Chen D, Tang T-X, Deng H, Yang X-P, Tang Z-H. Interleukin-7 biology and its effects on immune cells: mediator of generation, differentiation, survival, and homeostasis. Front Immunol. (2021) 12:747324. doi: 10.3389/fimmu.2021.747324
127. Bacchetta R, Sartirana C, Levings MK, Bordignon C, Narula S, Roncarolo M-G. Growth and expansion of human T regulatory type 1 cells are independent from TCR activation but require exogenous cytokines. Eur J Immunol. (2002) 32:2237–45. doi: 10.1002/1521-4141(200208)32:8<2237::AID-IMMU2237>3.0.CO;2-2
128. Jakwerth CA, Chaker AM, Guerth F, Oelsner M, Pechtold L, Zur Bonsen LS, et al. Sputum microRNA-screening reveals Prostaglandin EP3 receptor as selective target in allergen-specific immunotherapy. Clin Exp Allergy. (2021) 51:1577–91. doi: 10.1111/cea.14013
129. Stern R, Asari AA, Sugahara KN. Hyaluronan fragments: an information-rich system. Eur J Cell Biol. (2006) 85:699–715. doi: 10.1016/j.ejcb.2006.05.009
130. Bollyky PL, Wu RP, Falk BA, Lord JD, Long SA, Preisinger A, et al. ECM Components guide IL-10 producing regulatory T-cell (TR1) induction from effector memory T-cell precursors. Proc Natl Acad Sci USA. (2011) 108:7938–43. doi: 10.1073/pnas.1017360108
131. Balaji S, Wang X, King A, Le LD, Bhattacharya SS, Moles CM, et al. Interleukin-10-mediated regenerative postnatal tissue repair is dependent on regulation of hyaluronan metabolism via fibroblast-specific STAT3 signaling. FASEB J. (2017) 31:868–81. doi: 10.1096/fj.201600856R
132. Short WD, Wang X, Li H, Yu L, Kaul A, Calderon GA, et al. Interleukin-10 producing T lymphocytes attenuate dermal scarring. Ann Surg. (2021) 274:627–36. doi: 10.1097/SLA.0000000000004984
133. Katoh S, Uesaka T, Tanaka H, Matsuhara H, Ohashi-Doi K, Oga T. CD44 Is critical for the enhancing effect of hyaluronan in allergen-specific sublingual immunotherapy in a murine model of chronic asthma. Clin Exp Immunol. (2022) 208:202–11. doi: 10.1093/cei/uxac024
134. Gebe JA, Yadava K, Ruppert SM, Marshall P, Hill P, Falk BA, et al. Modified high-molecular-weight hyaluronan promotes allergen-specific immune tolerance. Am J Respir Cell Mol Biol. (2017) 56:109–20. doi: 10.1165/rcmb.2016-0111OC
135. Roncarolo MG, Gregori S, Bacchetta R, Battaglia M, Gagliani N. The biology of T regulatory type 1 cells and their therapeutic application in immune-mediated diseases. Immunity. (2018) 49:1004–19. doi: 10.1016/j.immuni.2018.12.001
136. Li H-D, Zhang Q-X, Mao Z, Xu X-J, Li N-Y, Zhang H. Exogenous interleukin-10 attenuates hyperoxia-induced acute lung injury in mice. Exp Physiol. (2015) 100:331–40. doi: 10.1113/expphysiol.2014.083337
137. Huet O, Laemmel E, Fu Y, Dupic L, Aprico A, Andrews KL, et al. Interleukin 10 antioxidant effect decreases leukocytes/endothelial interaction induced by tumor necrosis factor α. Shock. (2013) 39:83–8. doi: 10.1097/SHK.0b013e318278ae36
138. Matsuda M, Inaba M, Hamaguchi J, Tomita H, Omori M, Shimora H, et al. Local IL-10 replacement therapy was effective for steroid-insensitive asthma in mice. Int Immunopharmacol. (2022) 110:109037. doi: 10.1016/j.intimp.2022.109037
139. Cavani A, Nasorri F, Prezzi C, Sebastiani S, Albanesi C, Girolomoni G. Human CD4+ T lymphocytes with remarkable regulatory functions on dendritic cells and nickel-specific Th1 immune responses. J Invest Dermatol. (2000) 114:295–302. doi: 10.1046/j.1523-1747.2000.00881.x
140. Coomes SM, Kannan Y, Pelly VS, Entwistle LJ, Guidi R, Perez-Lloret J, et al. CD4+ Th2 cells are directly regulated by IL-10 during allergic airway inflammation. Mucosal Immunol. (2017) 10:150–61. doi: 10.1038/mi.2016.47
141. Rigas D, Lewis G, Aron JL, Wang B, Banie H, Sankaranarayanan I, et al. Type 2 innate lymphoid cell suppression by regulatory T cells attenuates airway hyperreactivity and requires inducible T-cell costimulator-inducible T-cell costimulator ligand interaction. J Allergy Clin Immunol. (2017) 139:1468–77.e2. doi: 10.1016/j.jaci.2016.08.034
142. Kurilin VV, Khantakova JN, Tereschenko VP, Lopatnikova JA, Obleukhova IA, Sennikov SV. The effects of immunosuppressive factors on primary dendritic cells from C57BL/6 and CBA mice. J Immunol Res. (2019) 2019:7029726. doi: 10.1155/2019/7029726
143. Branchett WJ, Cook J, Oliver RA, Bruno N, Walker SA, Stölting H, et al. Airway macrophage-intrinsic TGF-β1 regulates pulmonary immunity during early-life allergen exposure. J Allergy Clin Immunol. (2021) 147:1892–906. doi: 10.1016/j.jaci.2021.01.026
144. Chen W, Jin W, Hardegen N, Lei K-J, Li L, Marinos N, et al. Conversion of peripheral CD4+CD25- naive T cells to CD4+CD25+regulatory T cells by TGF-beta induction of transcription factor Foxp3. J Exp Med. (2003) 198:1875–86. doi: 10.1084/jem.20030152
145. Dardalhon V, Awasthi A, Kwon H, Galileos G, Gao W, Sobel RA, et al. IL-4 inhibits TGF-beta-induced Foxp3+ T cells and, together with TGF-beta, generates IL-9+ IL-10+ Foxp3(-) effector T cells. Nat Immunol. (2008) 9:1347–55. doi: 10.1038/ni.1677
146. Bettelli E, Carrier Y, Gao W, Korn T, Strom TB, Oukka M, et al. Reciprocal developmental pathways for the generation of pathogenic effector TH17 and regulatory T cells. Nature. (2006) 441:235–8. doi: 10.1038/nature04753
147. Musiol S, Alessandrini F, Jakwerth CA, Chaker AM, Schneider E, Guerth F, et al. TGF-β1 Drives inflammatory th cell but not treg cell compartment upon allergen exposure. Front Immunol. (2021) 12:763243. doi: 10.3389/fimmu.2021.763243
148. Levings MK, Sangregorio R, Galbiati F, Squadrone S, de Waal Malefyt R, Roncarolo MG. IFN-alpha and IL-10 induce the differentiation of human type 1 T regulatory cells. J Immunol. (2001) 166:5530–9. doi: 10.4049/jimmunol.166.9.5530
149. Bacchetta R, Gregori S, Serafini G, Sartirana C, Schulz U, Zino E, et al. Molecular and functional characterization of allogantigen-specific anergic T cells suitable for cell therapy. Haematologica. (2010) 95:2134–43. doi: 10.3324/haematol.2010.025825
150. Andolfi G, Fousteri G, Rossetti M, Magnani CF, Jofra T, Locafaro G, et al. Enforced IL-10 expression confers type 1 regulatory T cell (Tr1) phenotype and function to human CD4+ T cells. Mol Ther. (2012) 20:1778–90. doi: 10.1038/mt.2012.71
151. Magnani CF, Alberigo G, Bacchetta R, Serafini G, Andreani M, Roncarolo MG, et al. Killing of myeloid APCs via HLA class I, CD2 and CD226 defines a novel mechanism of suppression by human Tr1 cells. Eur J Immunol. (2011) 41:1652–62. doi: 10.1002/eji.201041120
152. Tree TIM, Lawson J, Edwards H, Skowera A, Arif S, Roep BO, et al. Naturally arising human CD4 T-cells that recognize islet autoantigens and secrete interleukin-10 regulate proinflammatory T-cell responses via linked suppression. Diabetes. (2010) 59:1451–60. doi: 10.2337/db09-0503
153. Asnagli H, Martire D, Belmonte N, Quentin J, Bastian H, Boucard-Jourdin M, et al. Type 1 regulatory T cells specific for collagen type II as an efficient cell-based therapy in arthritis. Arthritis Res Ther. (2014) 16:R115. doi: 10.1186/ar4567
154. Chen PP, Cepika A-M, Agarwal-Hashmi R, Saini G, Uyeda MJ, Louis DM, et al. Alloantigen-specific type 1 regulatory T cells suppress through CTLA-4 and PD-1 pathways and persist long-term in patients. Sci Transl Med. (2021) 13:eabf5264. doi: 10.1126/scitranslmed.abf5264
155. Jeon Y-W, Lim J-Y, Im K-I, Kim N, Nam Y-S, Song Y-J, et al. Enhancement of graft-versus-host disease control efficacy by adoptive transfer of type 1 regulatory T cells in bone marrow transplant model. Stem Cells Dev. (2019) 28:129–40. doi: 10.1089/scd.2018.0113
156. Ahangarani RR, Janssens W, VanderElst L, Carlier V, VandenDriessche T, Chuah M, et al. In vivo induction of type 1-like regulatory T cells using genetically modified B cells confers long-term IL-10-dependent antigen-specific unresponsiveness. J Immunol. (2009) 183:8232–43. doi: 10.4049/jimmunol.0901777
157. Ma Y, Bauer V, Riedel T, Ahmetlić F, Hömberg N, Hofer TP, et al. Interleukin-10 counteracts T-helper type 1 responses in B-cell lymphoma and is a target for tumor immunotherapy. Cancer Lett. (2021) 503:110–6. doi: 10.1016/j.canlet.2021.01.022
158. Schuler PJ, Saze Z, Hong C-S, Muller L, Gillespie DG, Cheng D, et al. Human CD4+ CD39+regulatory T cells produce adenosine upon co-expression of surface CD73 or contact with CD73+exosomes or CD73+cells. Clin Exp Immunol. (2014) 177:531–43. doi: 10.1111/cei.12354
159. Mandapathil M, Szczepanski MJ, Szajnik M, Ren J, Jackson EK, Johnson JT, et al. Adenosine and prostaglandin E2 cooperate in the suppression of immune responses mediated by adaptive regulatory T cells. J Biol Chem. (2010) 285:27571–80. doi: 10.1074/jbc.M110.127100
160. Mandapathil M, Szczepanski MJ, Jackson EK, Lang S, Whiteside TL. Breast cancer cell-derived adenosine enhances generation and suppressor function of human adaptive regulatory T cells. J Pers Med. (2021) 11:754. doi: 10.3390/jpm11080754
161. Mascanfroni ID, Takenaka MC, Yeste A, Patel B, Wu Y, Kenison JE, et al. Metabolic control of type 1 regulatory T cell differentiation by AHR and HIF1-α. Nat Med. (2015) 21:638–46. doi: 10.1038/nm.3868
162. Deaglio S, Dwyer KM, Gao W, Friedman D, Usheva A, Erat A, et al. Adenosine generation catalyzed by CD39 and CD73 expressed on regulatory T cells mediates immune suppression. J Exp Med. (2007) 204:1257–65. doi: 10.1084/jem.20062512
163. Borsellino G, Kleinewietfeld M, Di Mitri D, Sternjak A, Diamantini A, Giometto R, et al. Expression of ectonucleotidase CD39 by Foxp3+ Treg cells: hydrolysis of extracellular ATP and immune suppression. Blood. (2007) 110:1225–32. doi: 10.1182/blood-2006-12-064527
164. Wang J, Bhattarai A, Do HN, Akhter S, Miao Y. Molecular simulations and drug discovery of adenosine receptors. Molecules. (2022) 27:2054. doi: 10.3390/molecules27072054
165. Ohta A, Sitkovsky M. Role of G-protein-coupled adenosine receptors in downregulation of inflammation and protection from tissue damage. Nature. (2001) 414:916–20. doi: 10.1038/414916a
166. Raskovalova T, Huang X, Sitkovsky M, Zacharia LC, Jackson EK, Gorelik E. Gs protein-coupled adenosine receptor signaling and lytic function of activated NK cells. J Immunol. (2005) 175:4383–91. doi: 10.4049/jimmunol.175.7.4383
167. Csóka B, Himer L, Selmeczy Z, Vizi ES, Pacher P, Ledent C, et al. Adenosine A2A receptor activation inhibits T helper 1 and T helper 2 cell development and effector function. FASEB J. (2008) 22:3491–9. doi: 10.1096/fj.08-107458
168. Csóka B, Németh ZH, Duerr CU, Fritz JH, Pacher P, Haskó G. Adenosine receptors differentially regulate type 2 cytokine production by IL-33-activated bone marrow cells, ILC2s, and macrophages. FASEB J. (2018) 32:829–37. doi: 10.1096/fj.201700770R
169. Xiao Q, Han X, Liu G, Zhou D, Zhang L, He J, et al. Adenosine restrains ILC2-driven allergic airway inflammation via A2A receptor. Mucosal Immunol. (2022) 15:338–50. doi: 10.1038/s41385-021-00475-7
170. Cottrez F, Hurst SD, Coffman RL, Groux H. T regulatory cells 1 inhibit a Th2-specific response in vivo. J Immunol. (2000) 165:4848–53. doi: 10.4049/jimmunol.165.9.4848
171. Yao Y, Vent-Schmidt J, McGeough MD, Wong M, Hoffman HM, Steiner TS, et al. Tr1 cells, but not Foxp3+ regulatory T cells, suppress NLRP3 inflammasome activation via an IL-10-dependent mechanism. J Immunol. (2015) 195:488–97. doi: 10.4049/jimmunol.1403225
172. Guipouy D, Gertner-Dardenne J, Pfajfer L, German Y, Belmonte N, Dupré L. Granulysin- and granzyme-dependent elimination of myeloid cells by therapeutic ova-specific type 1 regulatory T cells. Int Immunol. (2019) 31:239–50. doi: 10.1093/intimm/dxy083
173. Locafaro G, Andolfi G, Russo F, Cesana L, Spinelli A, Camisa B, et al. IL-10-Engineered human CD4+ Tr1 cells eliminate myeloid leukemia in an HLA class I-dependent mechanism. Mol Ther. (2017) 25:2254–69. doi: 10.1016/j.ymthe.2017.06.029
Keywords: allergy, allergen, interleukin-10, immunotherapy, Tr1 cells
Citation: Matsuda M, Terada T, Kitatani K, Kawata R and Nabe T (2022) Roles of type 1 regulatory T (Tr1) cells in allergen-specific immunotherapy. Front. Allergy 3:981126. doi: 10.3389/falgy.2022.981126
Received: 29 June 2022; Accepted: 20 July 2022;
Published: 3 August 2022.
Edited by:
Dong-Ho Nahm, Ajou University, South KoreaReviewed by:
Young-Il Koh, Chonnam National University Medical School, South KoreaUlrich Matthias Zissler, University Hospital rechts der Isar, Technical University of Munich, Germany
© 2022 Matsuda, Terada, Kitatani, Kawata and Nabe. This is an open-access article distributed under the terms of the Creative Commons Attribution License (CC BY). The use, distribution or reproduction in other forums is permitted, provided the original author(s) and the copyright owner(s) are credited and that the original publication in this journal is cited, in accordance with accepted academic practice. No use, distribution or reproduction is permitted which does not comply with these terms.
*Correspondence: Takeshi Nabe t-nabe@pharm.setsunan.ac.jp
Specialty Section: This article was submitted to Allergen Immunotherapy, a section of the journal Frontiers in Allergy