- 1Department of Experimental Immunology, Amsterdam University Medical Centers, Location AMC, Amsterdam, Netherlands
- 2HAL Allergy BV, J.H. Oortweg, Leiden, Netherlands
- 3Division of BioTherapeutics, Leiden Academic Centre for Drug Research, Leiden University, Leiden, Netherlands
- 4Department of Biosciences, University of Salzburg, Salzburg, Austria
- 5Department of Supramolecular & Biomaterials Chemistry, Leiden Institute of Chemistry, Leiden University, Leiden, Netherlands
- 6Department of Otorhinolaryngology, Amsterdam University Medical Centers, Location AMC, Amsterdam, Netherlands
Although aluminum hydroxide (alum) is widely accepted and used as safe vaccine adjuvant, there is some concern about possible toxicity upon long-lasting repeated exposure during subcutaneous allergen immunotherapy (SCIT). Our objective was to evaluate allergen-bearing liposomes as possible alternative for alum-adsorption in SCIT. A self-assembling, coiled-coil forming peptide pair was used to anchor the major birch pollen allergen Bet v 1 to the surface of cationic liposomes. The resulting nanoparticulate liposomes were characterized with respect to their physicochemical, allergenic and immunological properties. Allergenicity was studied by ImmunoCAP inhibition and rat basophil leukemia (RBL) cell assays. Immunogenicity (immunoglobulin responses) and immune skewing (cytokine responses) were evaluated upon immunization of naïve mice, and compared to alum-adsorbed Bet v 1. Bet v 1-bearing cationic liposomes with a diameter of ∼200 nm showed a positive zeta potential. The coiled-coil conjugation of Bet v 1 to the surface of liposomes resulted in about a 15-fold lower allergenicity than soluble Bet v 1 as judged by RBL assays. Moreover, the nanoparticles induced Bet v 1-specific IgG1/IgG2a responses in mice that were several orders of magnitude higher than those induced by alum-adsorbed Bet v 1. This strong humoral response was accompanied by a relatively strong IL-10 induction upon PBMC stimulation with Bet v 1. In conclusion, their hypo-allergenic properties, combined with their capacity to induce a strong humoral immune response and a relatively strong IL-10 production, makes these allergen-covered cationic liposomes a promising alternative for aluminum salt-adsorption of allergen currently used in SCIT.
Introduction
Subcutaneous allergen immunotherapy (SCIT) has been used to treat allergies for more than 100 years (1). The treatment commonly consists of monthly subcutaneous injections of allergen extracts for 3 to 5 years to achieve optimal therapeutic effect. Therapy adherence is relatively low because of this long duration and the allergic side-effects that can occur (2). Often, aluminum hydroxide (alum) or aluminum phosphate is used as adjuvant for SCIT. Although alum has been reported to skew towards T helper (Th) 2 immune responses (3), during SCIT it has been shown to result in a more mixed Th1/Th2 cytokine response in combination with production of interleukin (IL)-10 by regulatory T- and B-cells (4, 5). Most importantly, these regulatory B-cells also produce the required protective allergen-specific immunoglobulin (Ig) G4 antibodies.
Alum has a long history of safe use in vaccines for infectious diseases but also in SCIT (3). Nevertheless, there is some concern with respect to long-term exposure to alum during allergen immunotherapy (AIT), particularly in a pediatric setting (6). Although as yet there is little or no evidence to support aluminum-associated pathology during AIT, a search for good alternatives may nevertheless be warranted. Besides directing the immune response, alum also serves as a depot for adsorption of allergens, partially shielding them from IgE antibodies and thereby reducing the risk of allergic side-effects (7). In recent years, different types of nanoparticles have drawn attention to serve as effective vaccine delivery systems (8–10). Liposomes are amongst the most promising nanoparticles to replace alum (11, 12).
Liposomes consist of one or more lipid bilayers with an aqueous core and are a versatile delivery system and adjuvant for vaccines (11–13). Antigens can be adsorbed to the lipid bilayer (14), incorporated in the lipid bilayer (15), or encapsulated in the aqueous core of the vesicle (16, 17). Recently, we described a novel antigen-anchoring method based on the interaction between two complementary α-helical peptides that form a coiled-coil (CC) structure (18). Immunization of mice with antigen attached to cationic liposomes via CC formation resulted in strong CD4+ T-cell proliferation and production of both interferon gamma (IFN-γ) and IL-10 (18). These cytokines are Th1 and regulatory T-cell responses signatures, respectively, and both are reported to be required for effective allergen immunotherapy (AIT) (8, 19–21).
The goal of this study was to design an alum-free candidate for SCIT using Bet v 1, the major allergen in birch pollen allergy, and liposomes. We produced a fusion protein between Bet v 1 and one of the two CC-forming peptides, peptide E (pepE-Bet v 1), to anchor the allergen to cationic liposomes carrying the complimentary CC-forming peptide K (pepK) at its surface. The resulting liposome nanoparticles were characterized with regard to their physicochemical, allergenic and immunological properties.
Materials and methods
Chemicals and reagents
Cholesterol, 1,2-distearoyl-sn-glycero-3-phosphocoline (DSPC) and 1,2-dioleoyl-3-trimethylammonium-propane (DOTAP) were purchased from Avanti Lipids (Birmingham, AL, United States). Recombinant Bet v 1 (isoform Bet v 1.0101, hereafter called Bet v 1) was produced by the Department of Molecular Biology of the University of Salzburg (Salzburg, Austria) (22). Dimethylformamide (DMF), piperidine, acetic anhydride, pyridine, trifluoroacetic acid (TFA) and acetonitrile (ACN) were purchased from Biosolve (Valkenswaard, The Netherlands). N,N-diisopropylethylamine (DIPEA), and ethyl cyanohydroxyiminoacetate (Oxyma) were obtained from Carl Roth (Karlsruhe, Germany). Dicholoromethane (DCM) and diethyl ether were supplied by Honeywell (Landsmeer, The Netherlands). Tentagel HL-RAM was obtained from Rapp Polymere (Tübingen, Germany). All amino acids were supplied by NovaBioChem (Darmstadt, Germany). Fmoc-NH-PEG4-COOH was purchased from Iris Biotech GmbH (Marktredwitz, Germany). Pierce BCA assay, Imject ® Alum and Fetal calf serum (FCS) were purchased from Thermo Fisher Scientific (Waltham, MA, United States). Isopropyl β-D-1-thiogalactopyranoside was obtained from Invitrogen (Carlsbad, CA, United States). Sucrose, 4-(2-hydroxyethyl)-1-piperazineethanesulfonic acid (HEPES), Triisopropylsilane (TIPS), sodium azide, Tyrodés salts, BSA, lysozyme, sodium bicarbonate, 4-methyl umbelliferyl-N-acetyl-beta-D-glucosaminide, hexafluorophosphate azabenzotriazole tetramethyl uranium (HATU), Triton X-100 and 3-(4,5-dimethylthiazol-2-yl)-2,5-diphenyltetrazolium bromide (MTT) were obtained from Sigma-Aldrich (St. Louis, MO, United States). Disodium hydrogen phosphate and sodium dihydrogen phosphate were purchased from Merck (Darmstadt, Germany). Ampicillin was obtained from Roche (Basel, Switzerland).
Mice
Six to eight weeks old female BALB/c mice were purchased from ENVIGO (Venray, The Netherlands). The animals were housed under specific pathogen-free conditions at the animal facility of the Amsterdam University Medical Centers, location AMC. All experiments were performed in compliance with the Dutch government guidelines and the Directive 2010/63/EU of the European Parliament and were approved by the Animal Ethics Committee of the AMC.
Peptide synthesis
Triple and quadruple repeats of the KIAALKE amino acid sequence (pepK) were synthesized by standard Fmoc chemistry using solid-phase peptide synthesis with an automated microwave peptide synthesizer (CEM liberty blue). Peptide K3, CGWG-(KIAALKE)3, was synthesized for affinity purification. Peptide K4, (KIAALKE)4, was conjugated to cholesterol (forming CPK) via a poly(ethylene glycol) spacer to enable anchoring of the peptide into the lipid bilayer of liposomes and was synthesized as described elsewhere (18). In short, Fmoc-NH-PEG4-COOH was coupled to resin-bound (KIAALKE)4 in the presence of DIPEA (5 eq.) and HATU (2.5 eq.) for 2.5 h. Fmoc-deprotection was done with 20% piperidine in DMF and the reactive amine was coupled to amino-cholestene hemi-succinate (1.05 eq.) in the presence of DIPEA (5 eq.) and HATU (2.5 eq.) for 4 h at room temperature. The peptide was cleaved from the resin with a mixture of TFA:TIPS:water (95:2.5:2.5 v/v/v) and precipitated in ice-cold diethyl ether.
Crude peptides were purified using a Shimadzu RP-HPLC system comprising two LC-8A pumps and an SPD-10AVP UV-Vis detector equipped with a Kinetic Evo C18 column. A gradient of 20%–80% B, (where B is 1% (v/v) TFA in ACN, and A is 1% (v/v) TFA in water) with a flow rate of 12 ml/min was used. Collected fractions were measured on a LC-MS system (Thermo Scientific TSQ quantum access MAX mass detector connected to an Ultimate 3,000 liquid chromatography system fitted with a 50 × 4.6 mm Phenomenex Gemini 3 μm C18 column). The resulting chromatogram and spectrum are shown in Supplementary Figure S1. ACN was removed by rotary evaporation (150 mbar, 50 °C) before lyophilization and the purified peptide was stored at −20 °C until use.
Design, expression and purification of pepE-bet v 1
PepE, (EIAALEK)3, the complementary peptide of the pepE/K self-assembling peptide pair was attached to the N-terminus of Bet v 1. A detailed description of pepE-Bet v1 design is given in the supplementary Materials and Methods. In short, the pepE-Bet v 1 gene was used to transfect E. coli BL21 (DE3) cells for protein production. The harvested cell pellets were disrupted by sonication and cellular debris was removed by centrifugation. The pepE-Bet v 1 protein was purified from the supernatant by affinity purification using cross-linked agarose beads functionalized with PepK. Bound pepE-Bet v 1 was eluted by lowering the pH to 2.5 to unfold the pepE/pepK coiled coil. Elution fractions containing pepE-Bet v 1 were pooled and loaded onto a Superdex 75 pg column for polishing. Pure protein fractions were pooled and stored at −20 °C until further use. The protein concentrations of pepE-Bet v 1 and Bet v 1 were determined by BCA assay using BSA as a standard. The secondary structure of the protein was measured with circular dichroism spectroscopy (CD) and is described in detail in the supplements.
Preparation of liposomes
CPK functionalized cationic liposomes (CPK-liposomes) and non-functionalized liposomes were prepared by the dehydration-rehydration method as described elsewhere (18). In short, lipids (DSPC, DOTAP and cholesterol in a 2:1:1 molar ratio, optionally including 1 mol% CPK) were mixed and the organic solvent was evaporated using a rotary evaporator. The lipid film was hydrated in 10 mmol/L HEPES, 280 mmol/L sucrose buffer in the presence of glass beads and lyophilized overnight. The resulting lipid cake was rehydrated with filtered Milli-Q® water to a final volume of 2 ml and homogenized using a LIPEX extruder (Evonik, Canada) over a stacked 400 nm & 200 nm Whatman® Nuclepore Track-Etch membrane (GE Healthcare, Chicago, IL, United States). Figure 1 shows a schematic design of the different types of liposomes used in this study. The Bet v 1 containing liposomes were prepared by adding 50 µg of Bet v 1 (as was determined by BCA)) to a 1 mg/ml liposome suspension. The pepE-Bet v 1 containing liposomes contained an equal amount of Bet v 1. The mixtures were incubated for at least 15 min before use.
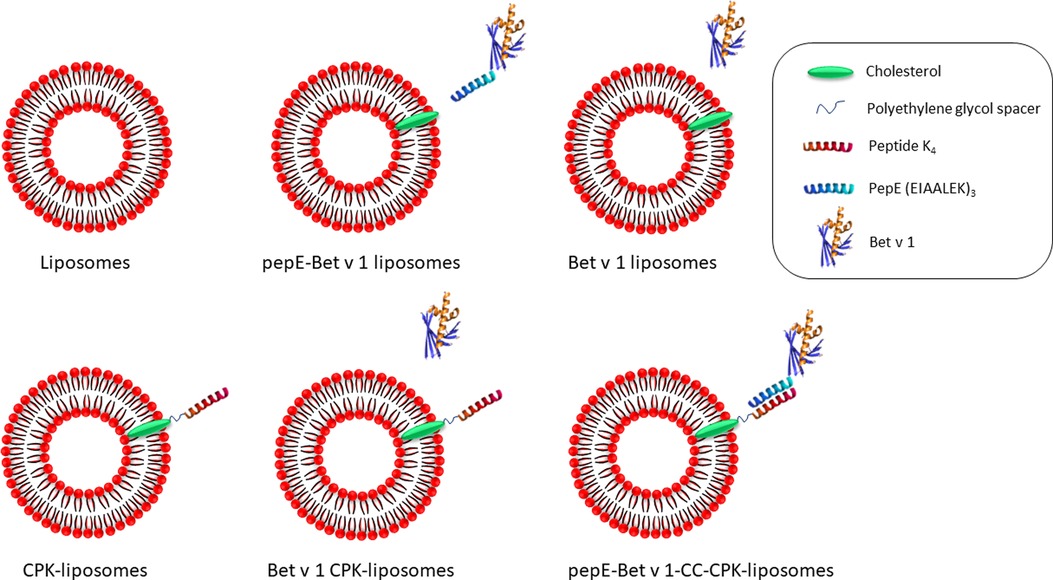
Figure 1. Schematic design of the liposomes used in this study. DSPC, DOTAP and cholesterol were mixed to generate liposomes (top left). The liposomes were functionalized with peptide K4 attached to a cholesterol anchor in the lipid bilayer via a polyethylene glycol spacer (CPK-liposomes, bottom left). PepE fused to the N-terminus of Bet v 1 enabled coiled coil mediated attachment to the CPK-liposomes (pepE-Bet v 1-CC-CPK-liposomes, bottom right). As control groups, pepE-Bet v 1 was added to liposomes (pepE-Bet v 1 liposomes, top middle), Bet v 1 was added to liposomes (Bet v 1 liposomes, top right) or Bet v 1 was added to CPK-liposomes (Bet v 1 CPK-liposomes, bottom middle).
Liposome characterization
The hydrodynamic diameter (Zave) and polydispersity (PDI) of liposome formulations and of alum-adsorbed Bet v 1 were measured by dynamic light scattering (DLS) using a Zetasizer Nano Zs (Malvern Instruments Ltd., Worcestershire, United States). The zeta potential is an electric field that exists between the surface of a particle and the surrounding liquid phase. It's an important physical characteristic of (nano)particles that affects their stability in a suspension and influences their interaction with cells (23). The zeta potential of all liposome formulations and alum-adsorbed Bet v 1 were measured using laser Doppler electrophoresis (lDe) on the same machine with a Zeta Dip Cell (Malvern Instruments Ltd.). Each sample was diluted 100-fold in 10 mmol/L HEPES buffer (pH 7.4, 0.2 µm filtered) before measurement.
ImmunoCAP IgE inhibition
IgE binding-potency of pepE-Bet v 1-CC-CPK was determined by ImmunoCap IgE inhibition assay using rBet v 1 ImmunoCAPs (t215). The starting concentration of pepE-Bet v 1-CC-CPK and the pepE-Bet v 1 control were the same concentration used for the immunization study (50 µg/ml). The included Bet v 1 and pepE-Bet v 1 control samples started at 100 µg/ml and 50 µg/ml respectively. All samples were serially diluted (10-fold dilutions) in 10 mmol/L HEPES, 280 mmol/L sucrose, pH 7.4 and pre-incubated 1:1 (v/v) for 30 min at room temperature with a serum pool. The pool, composed of 36 birch pollen allergic patient reference sera from the CREATE project (24), was pre-diluted to approximately 14 kU/L of Bet v 1 -specific IgE prior to mixing (1:1 v/v) with diluted pepE-Bet v 1-CC-CPK liposomes and control samples. For the uninhibited value serum was incubated with the HEPES/sucrose buffer.
Rat basophil leukemia (RBL) assay
To assess the allergenicity of pepE-Bet v 1-CC-CPK-liposomes, their ability to induce mediator release from effector cells was compared to that of soluble Bet v 1 and pepE-Bet v 1. For this, rat basophil leukemia cells (RBL-2H3), transfected with the human high-affinity IgE receptor (FcεRI), were sensitized with serum of Bet v 1 sensitized birch pollen allergic patients (24), and a β-hexosaminidase mediator release assay was performed as previously described (25). A detailed description of the method can be found in the supplementary Materials and Methods.
Animal studies
Mice were immunized subcutaneously on day 0, 7 and 14 with pepE-Bet v 1-CC-CPK-liposomes or alum-adsorbed Bet v 1 (1 mg alum per dose). The groups administered with Bet v 1 containing preparations received the equivalent of 10 µg Bet v 1 per dose (200 µl). Control groups received either buffer (10 mmol/L HEPES, 280 mmol/L sucrose, pH 7.4), pepE-Bet v 1, CPK-liposomes, pepE-Bet v 1 liposomes or Bet v 1 CPK-liposomes. Serum for antibody detection was collected on days −1, 6, 13 and 20. On day 27, 28 and 29 the animals received an intranasal challenge under 3% (v/v) isoflurane anesthesia with 30 µl 100 µg/ml birch pollen extract (BPE) in PBS to further boost the immune response. On day 31, the mice were sacrificed and blood and lung draining lymph nodes were collected to analyze Bet v 1 specific levels of IgG1, IgG2a and IgE in serum and to determine the production of cytokines (IL-4, IL-5, IL-13, IL-10 and IFN-γ) upon Bet v 1-specific stimulation of cells from lung-draining lymph nodes.
Determination of bet v 1 specific antibodies
Serum was analyzed for the level of Bet v 1-specific immunoglobulins by ELISA (IgE and IgG1: Opteia, BD, San Diego, CA, United States, IgG2a: eBioscience) as previously described (26). For Bet v 1-specific IgG1 and IgG2a, Maxisorp plates were coated overnight with Bet v 1 and with purified anti-mouse IgG1 and anti-mouse IgG2a for the standards. After blocking with FCS (10%), serum samples (IgG1: 10,000-fold diluted, IgG2a: 250-fold diluted) were incubated for 2 h and followed by an HRP-conjugated anti-IgG1 or anti-IgG2a detection step, according to the manufacturer's instructions. For Bet v 1-specific IgE detection the plates were coated with an anti-IgE capture antibody, according to manufacturer's instructions. Subsequently, the wells were incubated with biotinylated Bet v 1 followed by streptavidin-horseradish peroxidase conjugate incubation to detect Bet v 1-specific IgE. Murine IgE, IgG1 and IgG2a were used as standards to quantify the immunoglobulins.
Ex vivo re-stimulation of lung draining lymph node cells
Lung draining lymph node cell suspensions were plated in 96-well round bottom plates at a density of 2 × 105 cells per well in RPMI containing 50 µg/ml gentamicin, 5% (v/v) FCS and 50 µM 2-mercaptoethanol. The cells were re-stimulated for 4 days with 10 µg/ml Bet v 1. Expression levels of cytokines (IL-4, IL-5, IL-10, IL-13, and IFN-γ) were determined in the supernatant by ELISA (eBioscience).
Statistics
Data was processed and statistically analyzed in GraphPad v9.1.0 (Prism) for Windows. The kinetic antibody levels (IgG1 and IgG2a) were analyzed with a two-way ANOVA and subsequent Tukey's multiple comparison test. Differences between group means at endpoints (IgE, cytokine levels, half-max β-hexosaminidase mediator release and antibody ratios) were compared with a one-way ANOVA and subsequent Tukey's multiple comparison test. P values < 0.05 were considered significant.
Results
Physicochemical characterization of CPK-liposomes before and after antigen adsorption
Characterization of purified CPK, peptide K3 and purification of the pepE-Bet v 1 fusion protein by affinity chromatography can be found in the supplements. Peptide K3 and CPK eluted after respectively 7.77 and 4.37 min during LC (Supplementary Figures S1 and S2). The molecular masses of peptide K3 and CPK were comparable to their theoretical values of respectively 2680.6 and 3747.2 Da. Based on its primary structure, the pepE-Bet v 1 fusion protein has a predicted molecular weight of 20.055,70 Da which was confirmed by MS (20.053 Da; data not shown). The Bet v 1 protein had a molecular mass of 17.429 Da as determined by MS (data not shown). Compared to Bet v 1 the pepE-Bet v 1 fusion protein showed a similar secondary structure as indicated by CD (Supplementary Figure S5).
PepE-Bet v 1 adsorption to CPK-liposomes via coiled coil formation increased the liposome size and polydispersity index (PDI), while the zeta potential decreased. The coupling efficiency between pepE-Bet v 1 and the CPK liposomes was determined by spinning down the liposomes in a vivaspin® column followed by measuring the amount of unbound pepE-Bet v 1 in the flow through by RP-HPLC. The coupling efficiency was typically >90% (data not shown). All liposome formulations had a hydrodynamic diameter of approximately 200 nm and a positive zeta potential (Table 1). The pepE-Bet v 1-CC-CPK-liposomes were slightly larger than the other formulations. The zeta potential was lower after coiled-coil mediated antigen adsorption. In contrast to the liposome formulations, alum-adsorbed Bet v 1 showed a slightly negative zeta potential and a hydrodynamic diameter >1000 nm.
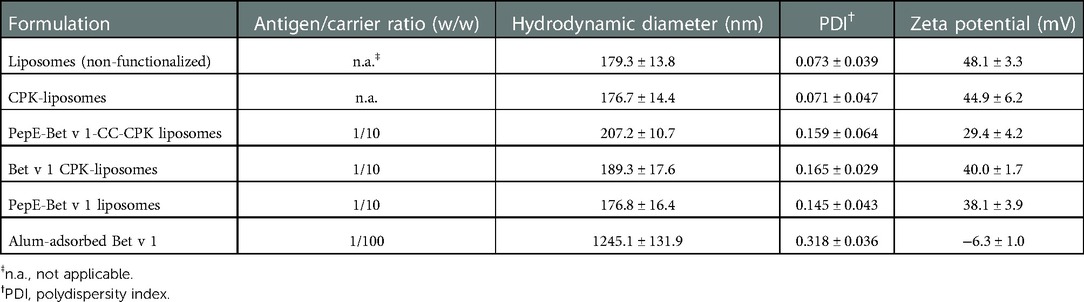
Table 1. Overview of all formulations and their physicochemical characteristics (mean values ± SD, n = 3-5). All formulations contained the same buffer composed of 10 mmol/L HEPES, 280 mmol/L sucrose, pH 7.4.
PepE-Bet v 1-cc-CPK-liposomes show hypo-allergenic IgE reactivity
Next, we characterized the IgE binding capacity of the pepE-Bet v 1-CC-CPK-liposomes by testing their potency to inhibit IgE binding to rBet v 1 ImmunoCAPs. Compared to Bet v 1 the IgE inhibition curves of pepE-Bet v 1 and pepE-Bet v 1-CC-CPK-liposomes were shifted to approximately 4- to10-fold higher inhibitor concentrations indicative of a reduced IgE binding capacity (Figure 2). Subsequently, we tested the IgE cross-linking capacity of pepE-Bet v 1-CC-CPK-liposomes by RBL mediator release assay using human sera for passive sensitization. PepE-Bet v 1 and PepE-Bet v 1-CC-CPK-liposomes showed significant 8- and 14-fold higher concentrations to reach half maximum mediator release compared to soluble Bet v 1. Thus, pepE-Bet v 1 was already less allergenic than soluble Bet v 1 but association with either liposomes or CPK-liposomes lowered the IgE cross-linking capacity even more (Figure 3). The control groups are shown in supplementary Supplementary Figure S4.
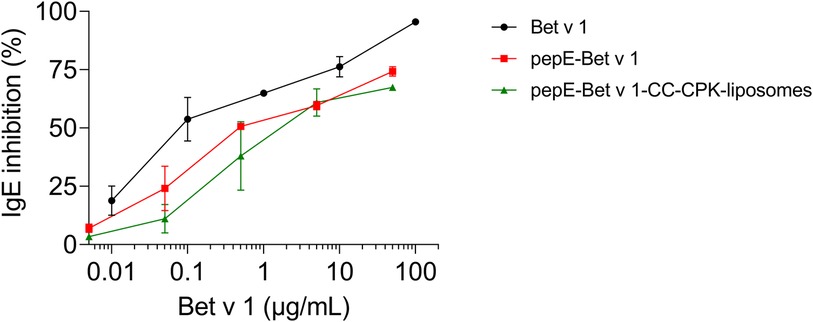
Figure 2. ImmunoCAP IgE inhibition assay. Serially diluted (10-fold) Bet v 1, pepE-Bet v 1 and pepE-Bet v 1-CC-CPK-liposomes were pre-incubated with pooled sera from birch pollen-allergic patients. After pre-incubation, the sample dilutions were loaded onto rBet v 1 ImmunoCAPs to determine the amount of uncomplexed IgE. An ImmunoCAP loaded with serum only was used as uninhibited IgE binding control. The shown graphs are normalized with regard to the uninhibited control and are an average of two independent experiments (mean ± SEM).
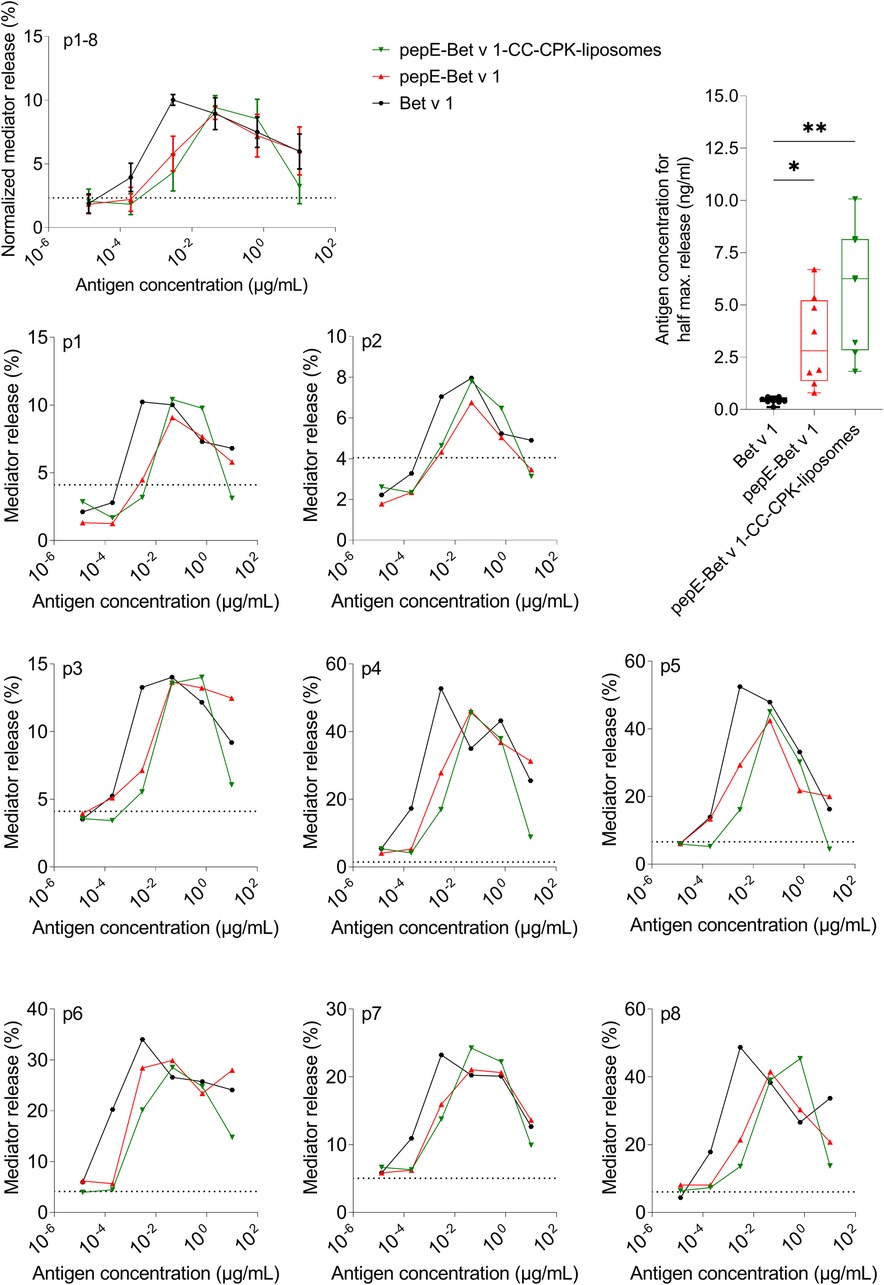
Figure 3. Allergenicity assessment by rat basophil leukemia cell assay. Rat basophils loaded with IgE from serum of Bet v 1-sensitized subjects were stimulated with different allergen formulations. Allergenicity was quantified by determining the concentration needed for half maximum mediator release. Differences between the groups were analyzed with a one-way ANOVA followed by Tukey's multiple comparisons test. Dotted line represents spontaneous mediator release by non-stimulated cells. The averaged, normalized curves of 8 patient sera (p1-8) as well as the patient specific mediator release curves showed a shift to the right of all pepE-Bet v 1 containing samples, indicating hypo-allergenicity. * = p < 0.05, ** = p < 0.01.
Coiled coil conjugation of bet v 1 triggers strong antibody responses in naïve mice
To evaluate the immune response of pepE-Bet v 1-CC-CPK-liposomes, naïve mice were immunized 3 times at weekly intervals followed by intranasal birch pollen extract challenge. PepE-Bet v 1-CC-CPK-liposomes was compared to buffer, alum-adsorbed Bet v 1, and to pepE-Bet v 1 in solution and adsorbed to liposomes (without CC). All active treatment groups received an equimolar amount of Bet v 1. Mice that received pepE-Bet v 1-CC-CPK-liposomes had 77-fold higher IgG1 and 220-fold higher IgG2a levels (Figures 4A,B, respectively) than alum-adsorbed Bet v 1 at the endpoint. Compared to soluble pepE-Bet v 1 and pepE-Bet v 1 liposomes these differences were, 31-fold and 5.6-fold (IgG1), and 73-fold and 12-fold (IgG2a), respectively. Moreover, only for the CC-conjugated Bet v 1, the induction of IgG antibodies was already detectable after the second injection. IgE levels were slightly higher upon immunization with pepE-Bet v 1-CC-CPK-liposomes (and other liposome preparations) than observed for soluble pepE-Bet v 1 and alum-adsorbed Bet v 1, but this did not reach significance (Figure 4C, Supplementary Figure S6C). In fact, the IgG1/IgE and IgG2a/IgE ratios in the pepE-Bet v 1-CC-CPK-liposomes group were significantly higher compared to alum-adsorbed Bet v 1 (IgG1: 11.2 vs. 1.72 and IgG2a: 3.78 vs. 0.25, Supplementary Figure S7). The other control groups are shown in Supplementary Figure S6.
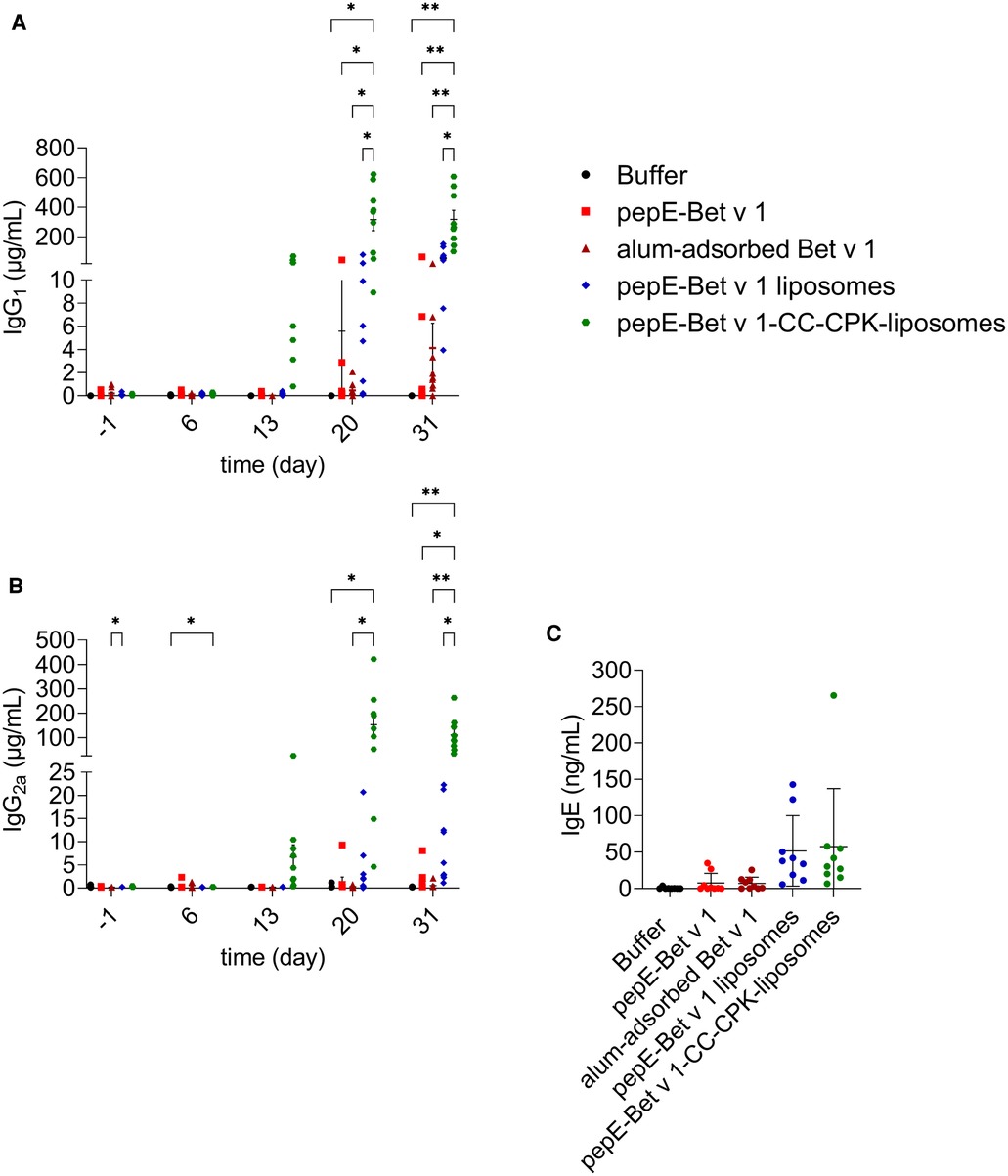
Figure 4. Murine immunogenicity. Mice (n = 9) were immunized with various formulations on day 0, 7 and 14 and received an intranasal challenge on day 27, 28 and 29 with birch pollen extract prior to the sacrifice (day 31). Bet v 1-specific IgG1 (A), IgG2a (B) and IgE (C) were measured in serum collected at day −1, 6, 13, 20 and 31. Group mean differences were analyzed with two-way ANOVA (IgG) or one-way ANOVA (IgE) followed by Tukey's multiple comparisons test. * = p < 0.05, ** = p < 0.01.
PepE-Bet v 1-cc-CPK-liposomes induce a strong mixed Th1/Th2/treg immune response
We also analyzed cytokine responses upon re-stimulation of lymph node cells (Figure 5). Compared to alum-adsorbed Bet v 1, pepE-Bet v 1-CC-CPK-liposomes induced 2.7-, 5.0-, 5.1-, 7.3- and 2.0-fold higher levels of IL-4, IL-5 and IL-13 (Th2), of IL-10 (Treg) and of IFN-γ (Th1) respectively, i.e., a strong mixed Th1/Th2/Treg response. This is further illustrated by a significantly higher IL-10/IL-4 ratio of pepE-Bet v 1-CC-CPK-liposomes compared to alum-adsorbed Bet v 1 (Supplementary Figure S9). The other control groups are shown in Supplementary Figure S8.
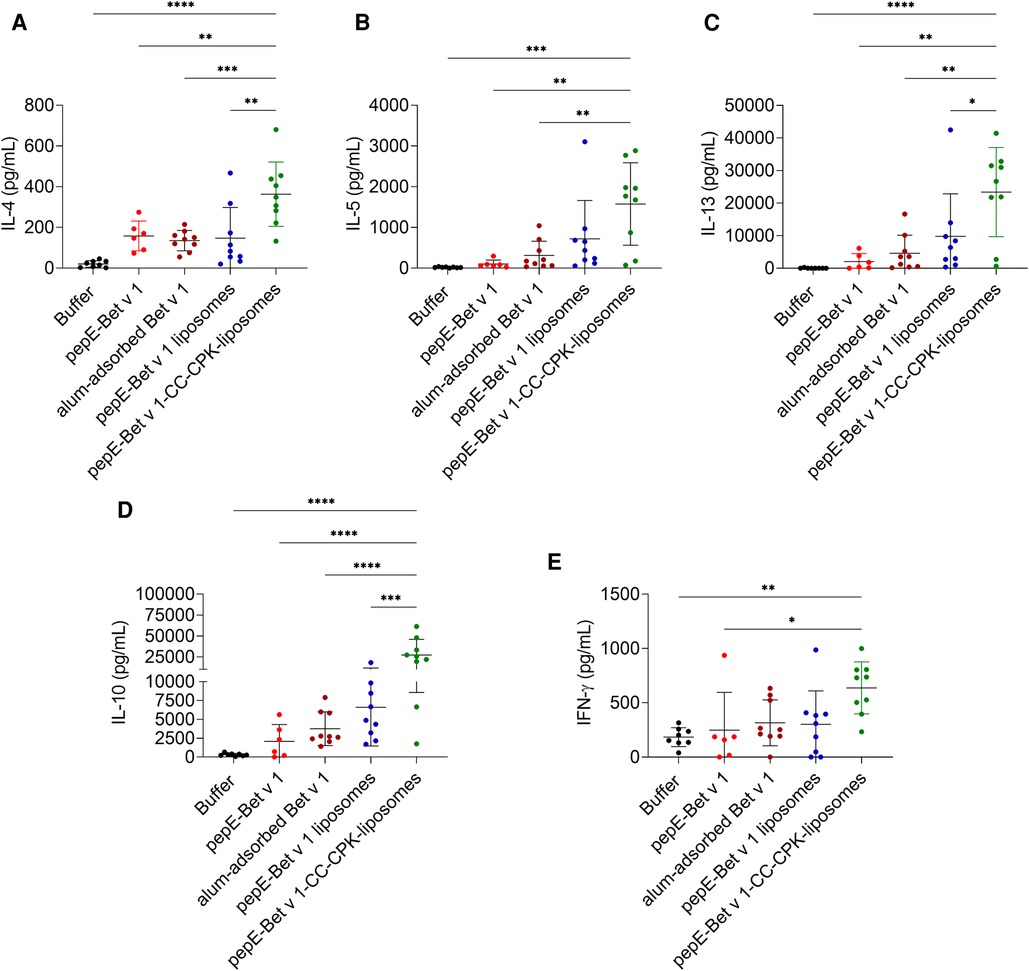
Figure 5. IL-4 (A), IL-5 (B), IL-13 (C), IL-10 (D) and IFN-γ (E) levels in supernatants of lung draining lymph node cells after ex vivo stimulation with Bet v 1. Bars represent the mean cytokine concentration and data points represent the signals for each individual mouse (n = 6-9). Group mean differences were analyzed with one-way ANOVA followed by Tukey's multiple comparisons test. * = p < 0.05, ** = p < 0.01, *** = p < 0.001, **** = p < 0.0001.
Discussion
Previously we described the functionalization of cationic liposomes with a CC-forming peptide, which enhanced the immune response to model antigen OVA323 (18). In the current study, the CC functionalized cationic liposomes were used to design a new birch pollen SCIT formulation. In this design, pepE fused to the Bet v 1 N-terminus allowed CC-facilitated conjugation of the fusion protein to the functionalized liposome surface. Our results showed that pepE attachment to Bet v 1 interfered with IgE binding as well as IgE cross-linking, i.e., promoted hypo-allergenicity. Multivalent display of pepE-Bet v 1 on liposomes contributed to further increase of hypo-allergenicity. The phenomenon of hypo-allergenicity of allergen molecules upon surface-exposure on nanoparticles has also been reported by others. For example, despite being recognized by IgE, intact Fel d 1 displayed on the surface of virus-like particles failed to efficiently cross-link IgE on mast cells (27, 28). Similarly, trimeric Der p 2 displayed at high density on plant-produced bioparticles also showed reduced IgE cross-linking potential (29). How high-density surface expression translates into hypo-allergenicity is not completely clear. The tight interaction between allergens and the particle surface could sterically mask IgE epitopes from IgE binding, thereby preventing efficient IgE cross-linking and downstream induced mediator release (29). Alternatively, combined with a lower diffusion coefficient, the effective free allergen concentration of allergen bearing nanoparticles is strongly reduced which provides another physical explanation for the reduced IgE cross-linking capacity (28). Nevertheless, these promising results should be corroborated by more studies designed to elucidate how allergen-bearing nanoparticles reduce IgE cross-linking capacity compared to soluble allergen.
Noticeably, alum-adsorbed Bet v 1 induced weak humoral responses using the subcutaneous immunization route. We have confirmed these results in several other immunization experiments. However, using the same protocol, materials, reagents and amount of allergen we found strong humoral (and T-cell) responses via the intraperitoneal administration route. This protocol is based on the sensitization protocol that we used in a previously published study (26). Our results (29)suggest that in our case the low humoral response may have been at least partly due to the chosen administration route (30). Moreover, in all experiments, IgG induction was only detected at the last time point. Therefore, the used protocol may have been too short for alum-adsorbed Bet v 1 to detect the peak of its immune response but sufficient to detect the early and strong humoral response of our pepE-Bet v 1-CC-CPK-liposomes. Our results are also in line with other murine studies that used subcutaneously administered alum-adsorbed Bet v 1, showing a relatively slow and variable induction of IgG antibodies which were mostly observed after the immunizations and not in between (31, 32).
Interestingly, all tested liposome preparations in this study were much more potent antibody inducers compared to alum-adsorbed Bet v 1, with coiled-coil liposomes clearly being superior, both quantitatively and kinetically (earlier induction). Possibly, CC formation between pepE-Bet v 1 and the liposome leads to a stable, multivalent display on the particle surface which has been associated with efficient B-cell receptor cross-linking and subsequent antibody production (33). This could explain the adjuvant potency of cationic liposomes (16, 34) and the added value of CC attachment as opposed to weaker allergen adsorption methods to induce strong antibody responses.
Although murine models can provide a lot of insight, direct translation to humans is often difficult. For example, sensitization of naïve mice to allergens often leads to relatively strong IgG1 responses while IgE responses are usually quite low and sometimes undetectable. In atopic humans, the situation is commonly reversed: The relative strong IgE response to allergens is usually accompanied with low IgG responses (35). Humoral responses also differ between mice and humans in the therapeutic setting. In mouse models of AIT, two IgG isotypes, IgG1 and IgG2a, have been associated with relief of allergic symptoms (26, 27). In humans, allergic symptom relief after SCIT is correlated with increased levels of allergen-specific IgG4 (5, 36, 37). IgG4 lacks the typical pro-inflammatory properties of the other human IgG isotypes and is capable of blocking IgE binding to allergens, thereby reducing IgE-mediated effector cell stimulation, clinical symptoms as well as IgE-facilitated antigen presentation (38). Murine IgG1 and human IgG4 are considered equivalent and could exert a similar blocking effect (39) In contrast to human IgG4, murine IgG1 antibodies also have anaphylactic properties (40). Our coiled-coil liposomes induced both a strong IgG1 and IgG2a antibody response against Bet v 1, suggesting a strong potential to induce blocking antibodies. The next step would be to study if these strong antibody responses are also induced in a mouse model of birch pollen SCIT and whether these responses associate with symptom reduction and outperform those induced by alum-adsorbed Bet v 1. Keeping the ambivalent role of mouse IgG1 in mind, it may be better to study this in a humanized mouse model (41).
The pepE-Bet v 1-CC-CPK-liposomes induced a strong but mixed Th1/Th2/Treg response compared to the Th2 biased response of alum-adsorbed Bet v 1. Although induction of Th2 cytokines could be undesirable for SCIT, human B cells require both IL-10 and IL-4 to switch to IgG4 production (35). In mice, the Th2 response induced by alum-adsorbed allergens is often used to induce allergic sensitization in SCIT models (30, 31, 42). Despite this pro-allergic potential of alum in animal studies, human clinical studies have shown that alum is capable of inducing regulatory responses and symptom relief after frequent administrations (26, 43–45). Therefore, the strong Th2 response and strong IL-10 induction of our pepE-Bet v 1-CC-CPK-liposomes could be interesting properties to achieve earlier and stronger protective immune responses during SCIT in humans.
The relatively high IL-10 expression levels induced by pepE-Bet v 1-CC-CPK-liposomes suggests a strong regulatory component. However, it is not clear which cells produced these large amounts of IL-10. In BALB/c mice IL-10 expression has been associated with Th2 immunity (46) Interestingly, mice immunized with the same CPK-liposomes bearing a pepE and OVA derived epitope conjugate showed induction of a CD4+ T-cell subset co-expressing IFN-γ and IL-10 (18). For this reason, it cannot be ruled out that at least part of the IL-10 levels observed after pepE-Bet v 1-CC-CPK-liposomes immunization was produced by similar Th1 skewed T-cells expressing both IFN-γ and IL-10.
In humans, induction of IL-10 producing regulatory T-cells capable of suppressing pro-inflammatory, type 2 T- and B-cell responses have been associated with improved clinical outcome during SCIT (8, 45). To bridge the gap between mice and humans, human based T-cell polarization studies using PBMCs or dendritic cells co-cultured with T-cells could shed more light on whether our pepE-Bet v 1-CC-CPK-liposomes are also capable of inducing similar allergen-specific IL-10 producing Tregs that are required for effective SCIT.
In summary, we have developed a novel SCIT candidate using functionalized, cationic liposomes surface-expressing Bet v 1 via CC formation. Their hypoallergenic character, strong humoral as well as mixed T-cell responses with a strong regulatory component induced by these nanoparticles are promising properties to design an alum-free birch pollen SCIT candidate.
Data availability statement
The original contributions presented in the study are included in the article/Supplementary Material, further inquiries can be directed to the corresponding author/s.
Ethics statement
The animal study was reviewed and approved by Animal Ethics Committee of the AMC, Amsterdam University Medical Center.
Author contributions
HW, RL, JS, HS, BS, WJ, RR, AK conceptualized and designed the study. HW wrote the manuscript. WJ, AK and RR critically reviewed the draft version of the manuscript. HW synthesized pepE-Bet v 1, RL performed the synthesis and characterization of all the liposomes. JS synthesized CPK. AL, HW and LR performed the animal experiments. AB and LA performed the RBL tests. All authors contributed to the article and approved the submitted version.
Funding
This work was supported by the Nederlandse Organisatie voor Wetenschappelijk Onderzoek (TKI-NCI, grant 731.014.207), the Austrian Science Funds (Projects P32189) and by the University of Salzburg priority program “Allergy-Cancer-BioNano Research Centre”.
Acknowledgment
We thank FF for the opportunity to perform the RBL assay at the Division of Allergy and Immunology, Department of Biosciences, Paris Lodron University of Salzburg.
Conflict of interest
HW and HS are employees of HAL Allergy BV. RR is a consultant for HAL Allergy BV, Citeq BV, Reacta Healthcare Ltd., Angany Inc., Mission MightyMe and AB Enzymes GmbH. RR reports speaker fees from HAL Allergy BV, ThermoFisher Scientific and ALK and has stock options of Angany Inc. The remaining authors declare that the research was conducted in the absence of any commercial or financial relationships that could be construed as a potential conflict of interest.
Publisher's note
All claims expressed in this article are solely those of the authors and do not necessarily represent those of their affiliated organizations, or those of the publisher, the editors and the reviewers. Any product that may be evaluated in this article, or claim that may be made by its manufacturer, is not guaranteed or endorsed by the publisher.
Supplementary material
The Supplementary Material for this article can be found online at: https://www.frontiersin.org/articles/10.3389/falgy.2022.1092262/full#supplementary-material.
References
1. Finegold I, Dockhorn RJ, Ein D, Dolen WK, Oppenheimer J, Potter LH. Immunotherapy throughout the decades: from noon to now. Ann Allergy, Asthma & Immunol. (2010) 105(5):328–36; quiz 37, 58. doi: 10.1016/j.anai.2010.08.008
2. Allam JP, Andreasen JN, Mette J, Serup-Hansen N, Wustenberg EG. Comparison of allergy immunotherapy medication persistence with a sublingual immunotherapy tablet versus subcutaneous immunotherapy in Germany. J Allergy Clin Immunol. (2018) 141(5):1898–901e5. doi: 10.1016/j.jaci.2017.12.999
3. He P, Zou Y, Hu Z. Advances in aluminum hydroxide-based adjuvant research and its mechanism. Hum Vaccin Immunother. (2015) 11(2):477–88. doi: 10.1080/21645515.2014.1004026
4. Klimek L, Brehler R, Hamelmann E, Kopp M, Ring J, Treudler R, et al. Evolution of subcutaneous allergen immunotherapy (part 1): from first developments to mechanism-driven therapy concepts. Allergo J Int. (2019) 28(3):78–95. doi: 10.1007/s40629-019-0092-4
5. Shamji MH, Durham SR. Mechanisms of allergen immunotherapy for inhaled allergens and predictive biomarkers. J Allergy Clin Immunol. (2017) 140(6):1485–98. doi: 10.1016/j.jaci.2017.10.010
6. Alvaro-Lozano M, Akdis CA, Akdis M, Alviani C, Angier E, Arasi S, et al. Eaaci allergen immunotherapy User's Guide. Pediatr Allergy and Immunol. (2020) 31(Suppl 25):1–101. doi: 10.1111/pai.13189
7. van der Kleij HPM, Warmenhoven HJM, van Ree R, Versteeg SA, Pieters RHH, Dreskin SC, et al. Chemically modified peanut extract shows increased safety while maintaining immunogenicity. Allergy. (2019) 74(5):986–95. doi: 10.1111/all.13687
8. Pfaar O, Agache I, de Blay F, Bonini S, Chaker AM, Durham SR, et al. Perspectives in allergen immunotherapy: 2019 and beyond. Allergy. (2019) 74(Suppl 108):3–25. doi: 10.1111/all.14077
9. Patil SU, Shreffler WG. Novel vaccines: technology and development. J Allergy Clin Immunol. (2019) 143(3):844–51. doi: 10.1016/j.jaci.2018.05.021
10. Gunawardana NC, Durham SR. New approaches to allergen immunotherapy. Ann Allergy, Asthma & Immunol. (2018) 121(3):293–305. doi: 10.1016/j.anai.2018.07.014
11. Perrie Y, Crofts F, Devitt A, Griffiths HR, Kastner E, Nadella V. Designing liposomal adjuvants for the next generation of vaccines. Adv Drug Delivery Rev. (2016) 99(Pt A):85–96. doi: 10.1016/j.addr.2015.11.005
12. Schwendener RA. Liposomes as vaccine delivery systems: a review of the recent advances. Ther Adv Vaccine. (2014) 2(6):159–82. doi: 10.1177/2051013614541440
13. Kratzer B, Köhler C, Hofer S, Smole U, Trapin D, Iturri J, et al. Prevention of allergy by virus-like nanoparticles (vnp) delivering shielded versions of Major allergens in a humanized murine allergy model. Allergy. (2019) 74(2):246–60. doi: 10.1111/all.13573
14. Watson DS, Endsley AN, Huang L. Design considerations for liposomal vaccines: influence of formulation parameters on antibody and cell-mediated immune responses to liposome associated antigens. Vaccine. (2012) 30(13):2256–72. doi: 10.1016/j.vaccine.2012.01.070
15. Hanson MC, Abraham W, Crespo MP, Chen SH, Liu H, Szeto GL, et al. Liposomal vaccines incorporating molecular adjuvants and intrastructural T-cell help promote the immunogenicity of hiv membrane-proximal external region peptides. Vaccine. (2015) 33(7):861–8. doi: 10.1016/j.vaccine.2014.12.045
16. Heuts J, Varypataki EM, van der Maaden K, Romeijn S, Drijfhout JW, van Scheltinga AT, et al. Cationic liposomes: a flexible vaccine delivery system for physicochemically diverse antigenic peptides. Pharm Res. (2018) 35(11):207. doi: 10.1007/s11095-018-2490-6
17. Colletier JP, Chaize B, Winterhalter M, Fournier D. Protein encapsulation in liposomes: efficiency Depends on interactions between protein and phospholipid bilayer. BMC Biotechnol. (2002) 2(1):9. doi: 10.1186/1472-6750-2-9
18. Leboux RJT, Benne N, van Os WL, Bussmann J, Kros A, Jiskoot W, et al. High-Affinity antigen association to cationic liposomes via coiled coil-forming peptides induces a strong antigen-specific Cd4(+) T-cell response. Eur J Pharm and Biopharm. (2021) 158:96–105. doi: 10.1016/j.ejpb.2020.11.005
19. Akdis M. Immune tolerance in allergy. Curr Opin Immunol. (2009) 21(6):700–7. doi: 10.1016/j.coi.2009.07.012
20. Akdis M, Akdis CA. Mechanisms of allergen-specific immunotherapy. J Allergy Clin Immunol. (2007) 119(4):780–91. doi: 10.1016/j.jaci.2007.01.022
21. Larche M, Akdis CA, Valenta R. Immunological mechanisms of allergen-specific immunotherapy. Nat Rev Immunol. (2006) 6(10):761–71. doi: 10.1038/nri1934
22. Soh WT, Aglas L, Mueller GA, Gilles S, Weiss R, Scheiblhofer S, et al. Multiple roles of bet V 1 ligands in allergen stabilization and modulation of endosomal protease activity. Allergy. (2019) 74(12):2382–93. doi: 10.1111/all.13948
23. Rasmussen MK, Pedersen JN, Marie R. Size and surface charge characterization of nanoparticles with a salt gradient. Nat Commun. (2020) 11(1):2337. doi: 10.1038/s41467-020-15889-3
24. van Ree R, Chapman MD, Ferreira F, Vieths S, Bryan D, Cromwell O, et al. The create project: development of certified reference materials for allergenic products and validation of methods for their quantification. Allergy. (2008) 63(3):310–26. doi: 10.1111/j.1398-9995.2007.01612.x
25. Vogel L, Luttkopf D, Hatahet L, Haustein D, Vieths S. Development of a functional in vitro assay as a novel tool for the standardization of allergen extracts in the human system. Allergy. (2005) 60(8):1021–8. doi: 10.1111/j.1398-9995.2005.00803.x
26. van Rijt LS, Gouveia L, Logiantara A, Canbaz D, Opstelten DJ, van der Kleij HP, et al. Birch pollen immunotherapy in mice: inhibition of Th2 inflammation is not sufficient to decrease airway hyper-reactivity. Int Arch Allergy Appl Immunol. (2014) 165(2):128–39. doi: 10.1159/000368777
27. Schmitz N, Dietmeier K, Bauer M, Maudrich M, Utzinger S, Muntwiler S, et al. Displaying fel D1 on virus-like particles prevents reactogenicity despite greatly enhanced immunogenicity: a novel therapy for cat allergy. J Exp Med. (2009) 206(9):1941–55. doi: 10.1084/jem.20090199
28. Engeroff P, Caviezel F, Storni F, Thoms F, Vogel M, Bachmann MF. Allergens displayed on virus-like particles are highly immunogenic but fail to activate human mast cells. Allergy. (2018) 73(2):341–9. doi: 10.1111/all.13268
29. Gomord V, Stordeur V, Fitchette AC, Fixman ED, Tropper G, Garnier L, et al. Design, production and immunomodulatory potency of a novel allergen bioparticle. PloS one. (2020) 15(12):e0242867. doi: 10.1371/journal.pone.0242867
30. Repa A, Wild C, Hufnagl K, Winkler B, Bohle B, Pollak A, et al. Influence of the route of sensitization on local and systemic immune responses in a murine model of type I allergy. Clin Exp Immunol. (2004) 137(1):12–8. doi: 10.1111/j.1365-2249.2004.02519.x
31. Wallner M, Hauser M, Himly M, Zaborsky N, Mutschlechner S, Harrer A, et al. Reshaping the bet V 1 fold modulates T(H) polarization. J Allergy Clin Immunol. (2011) 127(6):1571–8 e9. doi: 10.1016/j.jaci.2011.01.064
32. Pichler U, Asam C, Weiss R, Isakovic A, Hauser M, Briza P, et al. The fold variant Bm4 is beneficial in a therapeutic bet V 1 mouse model. BioMed Res Int. (2013) 2013:832404. doi: 10.1155/2013/832404
33. Bachmann MF, Jennings GT. Vaccine delivery: a matter of size, geometry, kinetics and molecular patterns. Nat Rev Immunol. (2010) 10(11):787–96. doi: 10.1038/nri2868
34. Christensen D, Korsholm KS, Rosenkrands I, Lindenstrom T, Andersen P, Agger EM. Cationic liposomes as vaccine adjuvants. Expert Rev Vaccines. (2007) 6(5):785–96. doi: 10.1586/14760584.6.5.785
35. Aalberse RC, Platts-Mills TA, Rispens T. The developmental history of ige and Igg4 antibodies in relation to atopy, eosinophilic esophagitis, and the modified Th2 response. Curr Allergy Asthma Rep. (2016) 16(6):45. doi: 10.1007/s11882-016-0621-x
36. Akdis M, Akdis CA. Mechanisms of allergen-specific immunotherapy: multiple suppressor factors at work in immune tolerance to allergens. J Allergy Clin Immunol. (2014) 133(3):621–31. doi: 10.1016/j.jaci.2013.12.1088
37. Geroldinger-Simic M, Zelniker T, Aberer W, Ebner C, Egger C, Greiderer A, et al. Birch pollen-related food allergy: clinical aspects and the role of allergen-specific ige and Igg4 antibodies. J Allergy Clin Immunol. (2011) 127(3):616–22 e1. doi: 10.1016/j.jaci.2010.10.027
38. Davies AM, Sutton BJ. Human Igg4: a structural perspective. Immunol Rev. (2015) 268(1):139–59. doi: 10.1111/imr.12349
39. Vrtala S, Ball T, Spitzauer S, Pandjaitan B, Suphioglu C, Knox B, et al. Immunization with purified natural and recombinant allergens induces mouse Igg1 antibodies that recognize similar epitopes as human ige and inhibit the human ige-allergen interaction and allergen-induced basophil degranulation. J Immunol. (1998) 160(12):6137–44. doi: 10.4049/jimmunol.160.12.6137
40. Beutier H, Gillis CM, Iannascoli B, Godon O, England P, Sibilano R, et al. Igg subclasses determine pathways of anaphylaxis in mice. J Allergy Clin Immunol. (2017) 139(1):269–80 e7. doi: 10.1016/j.jaci.2016.03.028
41. Ito R, Maruoka S, Gon Y, Katano I, Takahashi T, Ito M, et al. Recent advances in allergy research using humanized mice. Int J Mol Sci. (2019) 20(11):2740. doi: 10.3390/ijms20112740
42. Siebeneicher S, Reuter S, Krause M, Wangorsch A, Maxeiner J, Wolfheimer S, et al. Epicutaneous immune modulation with bet V 1 plus R848 suppresses allergic asthma in a murine model. Allergy. (2014) 69(3):328–37. doi: 10.1111/all.12326
43. Tourdot S, Airouche S, Berjont N, Moussu H, Betbeder D, Nony E, et al. Efficacy of sublingual vectorized recombinant bet V 1a in a mouse model of birch pollen allergic asthma. Vaccine. (2013) 31(23):2628–37. doi: 10.1016/j.vaccine.2013.03.041
44. Mondoulet L, Dioszeghy V, Ligouis M, Dhelft V, Dupont C, Benhamou PH. Epicutaneous immunotherapy on intact skin using a new delivery system in a murine model of allergy. Clin and Exp Allergy. (2010) 40(4):659–67. doi: 10.1111/j.1365-2222.2009.03430.x
45. Akdis CA, Akdis M. Mechanisms of allergen-specific immunotherapy and immune tolerance to allergens. World Allergy Organ J. (2015) 8(1):17. doi: 10.1186/s40413-015-0063-2
Keywords: aluminum, bet v 1, hypo-allergenic, liposomes, mice
Citation: Warmenhoven H, Leboux R, Bethanis A, van Strien J, Logiantara A, van Schijndel H, Aglas L, van Rijt L, Slütter B, Kros A, Jiskoot W and van Ree R (2023) Cationic liposomes bearing Bet v 1 by coiled coil-formation are hypo-allergenic and induce strong immunogenicity in mice. Front. Allergy 3:1092262. doi: 10.3389/falgy.2022.1092262
Received: 7 November 2022; Accepted: 19 December 2022;
Published: 10 January 2023.
Edited by:
Franziska Roth-Walter, University of Veterinary Medicine Vienna, AustriaReviewed by:
Cristina Gomez-Casado, Heinrich Heine University of Düsseldorf, GermanyPia Gattinger, Medical University of Vienna, Austria
© 2023 Warmenhoven, Leboux, Bethanis, van Strien, Logiantara, van Schijndel, Aglas, van Rijt, Slütter, Kros, Jiskoot and van Ree. This is an open-access article distributed under the terms of the Creative Commons Attribution License (CC BY). The use, distribution or reproduction in other forums is permitted, provided the original author(s) and the copyright owner(s) are credited and that the original publication in this journal is cited, in accordance with accepted academic practice. No use, distribution or reproduction is permitted which does not comply with these terms.
*Correspondence: Ronald van Ree ci52YW5yZWVAYW1zdGVyZGFtdW1jLm5s
†These authors have contributed equally to this work and share first authorship
‡Passed away on August 22nd, 2021
Specialty Section: This article was submitted to Allergen Immunotherapy, a section of the journal Frontiers in Allergy