- 1Regulatory Services Directorate, Sugar Board of Tanzania, Dar es Salaam, Tanzania
- 2Institute of Resource Assessment, Centre for Climate Change Studies, University of Dar es Salaam, Dar es Salaam, Tanzania
- 3Department of Crop Science and Beekeeping Technology, College of Agriculture and Food Technology, University of Dar es Salaam, Dar es Salaam, Tanzania
The cultivation of sugarcane (Saccharum officinarum L.) in the face of climate change requires robust strategies for managing pests, diseases, and weeds. This systematic review exposes critical deficiencies in current practices and underscores the need for climate-adaptive strategies. Climate change differentially influences pest behaviour, disease progression, and weed growth across various regions, yet the lack of region-specific responses impairs effective management. The review emphasizes the necessity for localized approaches that consider specific climatic conditions and the development of predictive models to anticipate pest and disease outbreaks. These models include Decision Support Systems (DSS), Support Vector Machines (SVM), Susceptible-Exposed-Infectious-Recovered (SEIR) models, Geographic Information Systems (GIS), Species Distribution Models (SDMs), Agricultural Production Systems sIMulator (APSIM), and Integrated Pest Management (IPM). Crucial strategies encompass integrated pest and disease management, adaptive breeding, precision agriculture, and ongoing innovation. Precision agriculture technologies, such as remote sensing and drones, enable early detection and prompt interventions. By adopting these adaptive measures and addressing existing research gaps, the sugarcane industry can bolster its resilience and maintain productivity amidst evolving climatic conditions.
Systematic review registration: https://www.bmj.com/content/372/bmj.n71.
1 Introduction
Sugarcane (Saccharum officinarum L.) stands as a vital global crop, playing a pivotal role in sugar production, bio-energy through bagasse, and livestock feed from straw (Msomba et al., 2021). Its cultivation spans 124 countries, with Brazil, India, and China dominating production. However, the sugar industry faces threats from climate change-induced shifts in temperature and weather patterns, amplifying the impact of insect pests, noxious weeds, and diseases (Bordonal et al., 2018; Sorvali et al., 2021).
Climate change exacerbates the outbreak and spread of pests like yellow sugarcane aphids (YSA) and diseases like gummosis and smut, impacting global sugarcane productivity (Cock and Allard, 2013; Lu et al., 2021). The primary driver of the challenges faced by the sugarcane industry is climate change, affecting temperature, rainfall, and humidity (Tamiru and Fekadu, 2019). The consequent impact on insect pests and diseases poses a significant threat to sugarcane cultivation globally. Yellow sugarcane aphids (YSA) (Sipha flava (Forbes)), white scales (Pseudaulacaspis pentagona (Targioni)), and white grubs (Phyllophaga sp.) are recognized culprits affecting sugarcane productivity worldwide (Ramanujam et al., 2021). The increasing prevalence of pests such as YSA, attributed to climate-driven shifts, necessitates a thorough examination of their impact and strategies for sustainable management (Way et al., 2015). It is inevitable that climate parameters significantly impact insect pests and disease pathogens in agriculture (Raid et al., 2013).
Diseases, including gummosis, ratoon stunting disease, smut, leaf scald, sugarcane mosaic virus, and leaf rust, further compound the challenges faced by the sugarcane industry (Lu et al., 2021). High temperatures are predicted to escalate the incidence of smut disease (Matthieson, 2007), while dry weather exacerbates ratoon stunting disease symptoms (Ramanujam et al., 2021). Climate-induced extreme weather events are cited as major contributors to diseases like leaf brown rust and orange rust (Raid et al., 2013). The impact of these diseases on sugarcane requires meticulous study to develop effective adaptation and mitigation strategies.
The significant sugarcane producers face challenges like insect pests infestation and disease proliferation, but comprehensive studies on the prevalence and severity of these factors are lacking (January et al., 2020). Understanding the influence of climate variability and sugarcane varieties on pests and diseases in different regions is critical for tailoring interventions. Economic thresholds, considering factors like pest density, crop variety, and market value, are essential for informed decision-making in pest management (Knutson et al., 2016). The absence of such thresholds specific to notorious pests like YSA highlights the need for focused research in this area (Bowling et al., 2016).
Climate change affects population dynamics, distribution, and activity of pests, necessitating adaptive management strategies (Mercer, 2020). Yet, limited information exists on the effects of climate change on sugarcane pests and diseases, emphasizing the need for research and innovative management tactics (Mercer, 2020). There is also a need to evaluate the influence of agro-ecological zones and sugarcane varieties on pest and disease variability. This addresses the gap in understanding how these factors contribute to the source-sink dynamics, timing, and severity of infestations (Pekarcik and Jacobson, 2021). For instance, considering the rapid population growth potential of sugarcane aphids, economic thresholds are crucial for effective pest management and sustainable sugarcane productivity (Bowling et al., 2016; Brewer et al., 2017). Therefore, this systematic review intends to provide a comprehensive understanding of the factors influencing sugarcane production globally and their interplay with climate change. The study aims to contribute valuable insights into sustainable sugarcane cultivation strategies, considering the increasing challenges posed by climate-induced changes in pest and disease dynamics by analyzing existing literature and conducting new research. The findings can inform policymakers and practitioners, enabling evidence-based decision-making to ensure the long-term viability of the sugarcane and sugar industry worldwide.
The rationale of this review emphasizes the impact of climate change on sugarcane pests and diseases, plus explicitly addressing potential adaptation and mitigation strategies, especially climate-driven adaptation strategies. Importantly, Mercer’s (2020) suggestions modified integrated pest management tactics and monitoring climate and pest populations, but the study could benefit from a more detailed exploration of innovative approaches and practices that could enhance the industry’s resilience to climate-induced challenges. Much of the existing research focuses on the global impact of climate change on sugarcane production but somewhere lacks a detailed examination of region-specific challenges and solutions. Sugarcane cultivation is highly influenced by local agro-ecological conditions, and there may be unique factors affecting pest and disease dynamics in different regions (Lu et al., 2021). There is also a gap in the exploration of existing or potential pest and disease forecasting models in literature (Mulianga et al., 2013; Donatelli et al., 2017; Bhatt et al., 2022; Koralewski et al., 2022; Daphal and Koli, 2023; Ngcobo et al., 2023). Developing accurate and reliable models for predicting the occurrence and severity of pests and diseases can be crucial for proactive and timely management (Ngcobo et al., 2023; Sharma et al., 2023; Subedi et al., 2023). The research could delve into incorporating a more in-depth analysis of the available forecasting models and propose improvements or new models tailored to the sugarcane industry, considering the specific challenges posed by climate change. Addressing these research gaps would contribute to a more holistic and actionable understanding of the complex interplay between climate change, sugarcane pests and diseases, and sustainable cultivation practices.
Developing climate-resilient sugarcane varieties through genetic research and adaptive breeding is essential. Further studies on integrated pest and disease management (IPDM) that combine biological, chemical, and cultural practices are necessary for effective management under changing climates. Advancing precision agriculture technologies like drones, remote sensing, and can enhance early detection, monitoring, and targeted treatment of pest and disease outbreaks. Researching soil health and biodiversity, particularly the role of soil microbiomes, is vital for improving resilience. Additionally, sustainable cultivation practices such as reduced tillage, organic amendments, and crop rotation should be investigated. Finally, examining the effects of extreme weather events on pest and disease dynamics and developing mitigation strategies is crucial. These research directions can enhance the resilience and sustainability of sugarcane cultivation. It would also provide practical insights for stakeholders in the sugarcane industry, enabling them to make informed decisions and implement effective strategies to ensure long-term productivity and resilience (Ncoyini et al., 2022; Kadam et al., 2023; Shawky et al., 2023). Addressing the research gaps in understanding the interplay between climate change, sugarcane pests, diseases, and sustainable cultivation practices is crucial. Key initiatives include developing climate-resilient sugarcane varieties through genome sequencing, editing, and field trials. IPDM by creating biocontrol agents and testing related protocols can improve pest and disease management. Enhancing precision agriculture technologies with AI, machine learning, and drone integration aids in early detection and outbreak management.
Early detection of pests and diseases in sugarcane can be significantly enhanced through a variety of advanced technologies and methods (Li, 2024). One crucial tool is remote sensing, which involves the use of satellites and drones equipped with multispectral or hyperspectral sensors (Waters et al., 2024). These sensors can detect subtle changes in plant health and vigour, allowing for early identification of stress indicators associated with pest infestations or disease outbreaks. Remote sensing data is processed using sophisticated algorithms and software to generate maps that pinpoint areas of concern in sugarcane fields, enabling targeted intervention (Fresneda-Quintana et al., 2024).
Diagnostic tools play a pivotal role in early detection as well. These include handheld devices and laboratory assays that analyze plant samples for specific pathogens or pest infestations (Vinayaka and Prasad, 2024). Polymerase chain reaction (PCR) techniques, for instance, can rapidly detect the presence of pathogens with high accuracy (Archana et al., 2024). Immunological assays and DNA-based diagnostics also contribute to precise identification and monitoring of pest and disease threat (Tu et al., 2024).Emerging technologies such as Internet of Things (IoT) devices and sensor networks are increasingly integrated into precision agriculture systems. These devices monitor environmental parameters like temperature, humidity, and soil moisture in real-time (Narayana et al., 2024). They can provide continuous data streams that are analyzed to detect anomalies indicative of pest activity or disease development.
Artificial intelligence (AI) and machine learning algorithms are transforming early detection capabilities by analyzing large datasets from remote sensing, IoT sensors, and diagnostic tools (Vinayaka and Prasad, 2024). These algorithms can identify patterns and predict potential outbreaks before visible symptoms manifest, allowing farmers to implement proactive management strategies promptly (Chaiyana et al., 2024). Collaboration between researchers, agricultural extension services, and technology developers is crucial for advancing these technologies. Funding opportunities from governmental agricultural departments, research grants, and partnerships with private sector entities may further drive innovation in early pest and disease detection technologies. By leveraging these advanced tools and methods, sugarcane growers can achieve more effective pest and disease management, leading to improved crop health and productivity. Soil microbiomes, can boost plant resistance (Xiao et al., 2024). Researching sustainable practices like reduced tillage, organic amendments, and crop rotation promotes sustainability. Examining the effects of extreme weather events on pest and disease dynamics and developing climate adaptation strategies can mitigate risks. Funding and collaboration opportunities include national agricultural departments, international organizations, private sector investments, academic institutions, NGOs, and innovation grants. These efforts can significantly enhance sugarcane resilience and sustainability.
2 Methodology for literature search
This baseline desk review of literature and synthesis of the findings spanned from January to April 2024 (See details in Table 1; Figure 1). The literature search followed the Preferred Reporting Items for Systematic Reviews and Meta-Analyses (PRISMA) framework outlined by Tlatlaa et al. (2023). PRISMA serves to enhance transparent reporting by elucidating the motivations behind the study (answering “why”)?, detailing the actions undertaken by the authors (answering “what”)?, and presenting the review findings (answering “what”)?. The PRISMA furnishes a checklist for a systematic literature review report, covering various sections such as title, abstract, introduction, methods, results, discussion, and other pertinent information, following the PRISMA 2020 checklist guidelines updated by Page et al. (2021). The PRISMA emphasizes that the title should unmistakably denote the report as a literature review, while the abstract needs to provide a structured summary. It also stresses that the introduction should discuss the rationale and objectives of the review. Methods entail specifying eligibility criteria, information sources, search strategy, selection process, and risk of bias assessment. Results involve detailing the study selection process, characteristics, risk of bias, and individual study outcomes. The discussion segment is dedicated to interpreting results, addressing limitations, and exploring implications. Additional information includes registration details, funding sources, conflicts of interest, and the availability of data and materials.
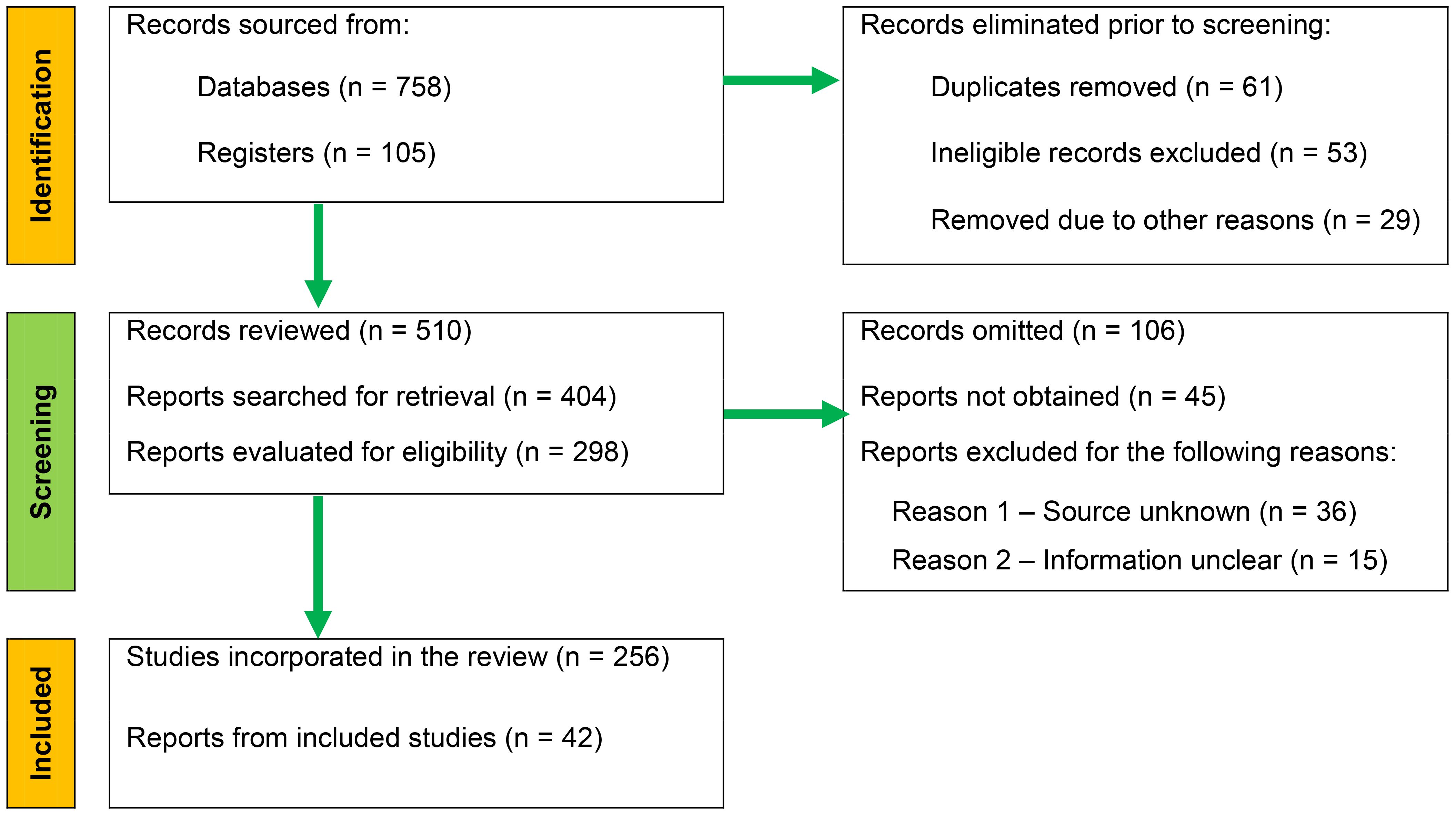
Figure 1. Quantity of studies identified and incorporated into the analysis. Adapted from Tlatlaa et al. (2023).
3 Findings of the review and synthesis
The review presents findings organized into six main sections: (1) Limited emphasis on climate-driven adaptation strategies for pest and disease control, (2) Inadequate examination of region-specific climate effects on pest and disease dynamics, (3) Sparse assessment of pest and disease forecasting models under climate change, (4) Impact of climate change on insect pests affecting sugarcane, (5) Impact of climate change on sugarcane diseases, and (6) Climate change influence on prominent sugarcane weeds.
3.1 Limited emphasis on climate-driven adaptation strategies for pest and disease control
While existing literature comprehensively acknowledges the influence of climate change on sugarcane pests and diseases, it somewhat overlooks the need for detailed exploration of climate-driven adaptation strategies. Some literature highlights the impact of climate change on the proliferation of insect pests and diseases, emphasizing the role of temperature, atmospheric carbon dioxide levels, and precipitation patterns (Delcour et al., 2015; Skendžić et al., 2021; Shivanna, 2022; Nguru and Mwongera, 2023). However, the specific measures and practices that can be implemented to adapt sugarcane cultivation to these changing conditions are not extensively discussed. Understanding how climate change alters the dynamics of pest and disease populations is crucial, but equally important is the identification of adaptive measures that farmers and stakeholders can employ (Sakdapolrak et al., 2024; Sarkar et al., 2024). For instance, the study could be required delving into the development and implementation of climate-resilient agronomic practices, pest-resistant sugarcane varieties, and precision farming technologies. Integrating these adaptation strategies into the research framework would provide a more comprehensive guide for practitioners seeking sustainable solutions in the face of climate-induced challenges (Araújo et al., 2023; Durán-Díaz, 2023).
Limited emphasis on climate-driven adaptation strategies for pest and disease control of sugarcane reflects a critical gap in addressing the evolving challenges posed by climate change in agricultural systems (Shahzad et al., 2021; Grigorieva et al., 2023). Grigorieva et al. (2023) conducted a comprehensive review of adaptation strategies and interventions proposed globally to mitigate the impact of climate change on agricultural development and production and underscored the importance of context-specific adaptation strategies, cautioning against limiting discussions to singular “top-down” or “bottom-up” approaches. Grigorieva et al. (2023) pointed out that some adaptation measures may be insufficient or even exacerbate vulnerability to climate change. In many agricultural contexts, including sugarcane cultivation, historical approaches to pest and disease control have primarily relied on conventional methods such as chemical pesticides and resistant crop varieties (Budeguer et al., 2021). While these methods have been effective to some extent, they often overlook the complex interplay between climate change and pest/disease dynamics. Pests and diseases may adapt and spread in ways that traditional management practices can no longer adequately address as climate conditions continue to change (Singh et al., 2019; Shahzad et al., 2021).
There is a pressing need for comprehensive studies to understand how climate variability influences the behaviour, life cycles, and prevalence of sugarcane pests and diseases. Without knowledge on these aspects, it becomes challenging to devise targeted adaptation strategies that can effectively mitigate risks and enhance resilience in sugarcane production systems (Grigorieva et al., 2023). Resource constraints further exacerbate the issue, as agricultural research and development budgets may prioritize other pressing concerns over climate-driven adaptation strategies for sugarcane (Khan and Akhtar, 2015). Limited funding and resources hinder the innovation and implementation of novel approaches that could bolster the resilience of sugarcane crops against pest and disease pressures exacerbated by climate change (Dixon et al., 2014).
Moreover, policy frameworks and agricultural extension programs may not adequately emphasize the importance of climate-resilient pest and disease management practices in sugarcane cultivation (Peng et al., 2020; van Zonneveld et al., 2020). There is a need for policymakers to integrate climate adaptation considerations into agricultural policies and provide incentives for adopting sustainable practices. Additionally, raising awareness among farmers and stakeholders about the implications of climate change on sugarcane production and the necessity for proactive adaptation measures is essential for fostering widespread adoption of climate-smart practices (Vermeulen et al., 2018; Adesipo et al., 2019; Kalimba and Culas, 2020; Das and Ansari, 2021; Majhi et al., 2023). Increased vulnerability to pest infestations and disease outbreaks can result in reduced yields, lower-quality produce, and economic losses for farmers and the sugar industry (Tripathi et al., 2022; Ward et al., 2022). Moreover, reliance on chemical pesticides and fungicides may contribute to environmental degradation, posing risks to soil health, water quality, and biodiversity (Boudh and Singh, 2019; Mandal et al., 2019; Alengebawy et al., 2021). Addressing this requires a concerted effort from multiple stakeholders, including researchers, policymakers, industry representatives, and farmers (Bartels et al., 2013). Investments in research and development, capacity building, policy support, and public awareness are crucial for developing and implementing effective climate-resilient pest and disease management strategies in sugarcane cultivation (Khatri-Chhetri et al., 2019; Shrestha and Thapa, 2019). By prioritizing climate adaptation in agricultural decision-making processes, stakeholders can enhance the sustainability and resilience of sugarcane production systems in the face of climate change (Andrieu et al., 2019).
3.2 Inadequate examination of region-specific climate effects on pest and disease dynamics
There is a notable research gap concerning the exploration of region-specific climate impacts on sugarcane pests and diseases. Climate change manifests differently in various geographic locations, influencing the prevalence and behaviour of pests and diseases in distinct ways (Giliba et al., 2020; Hauser et al., 2021; Skendžić et al., 2021; Singh et al., 2023). To address this gap, the research could incorporate a more nuanced examination of how local climate patterns and environmental conditions contribute to the challenges faced by sugarcane growers (Finch et al., 2021; Montalvo-Navarrete and Lasso-Palacios, 2024). This regional focus would facilitate the development of tailored recommendations and strategies, accounting for the specific interactions between climate variables and pest/disease dynamics in sugarcane fields. For instance, Nguru and Mwongera (2023) assessed the impact of climate change on the suitability of natural habitats for crop pests and diseases, and project an increased geographical spread of suitable habitats for crop pests in warmer environments by the 2030s. According to Nguru and Mwongera (2023), the importance of future-facing, long-term climate adaptation and mitigation measures create less suitable microclimates for crop pests and diseases.
Finch et al. (2021) conducted a study focusing on the global increase in mean surface temperatures, drawing attention to the rise of approximately 0.7°C per century since 1900 and a more accelerated pace of 0.16°C per decade since 1970. Studies emphasize that the primary driver of this warming trend is the heightened concentration of greenhouse gases resulting from human activities (Jorgenson et al., 2019). Literature also addresses changes in precipitation patterns, acknowledging their variability compared to temperature changes (Alexander, 2016). Despite this variability, it is argued that future climatic changes, even under conservative emission scenarios, are likely to include further temperature increases and a significant increase in drought conditions in some regions, as highlighted by the Intergovernmental Panel on Climate Change (IPCC) in 2007 (Finch et al., 2021).
The alterations in temperature and precipitation patterns will affect invasive species in various ways. Notably, climate change is anticipated to challenge conventional perceptions of nonnative invasive species, as the impacts on some species may shift while others remain unaffected (Hume et al., 2021). Moreover, certain nonnative species could become more invasive, posing additional challenges for ecosystems. Additionally, Finch et al. (2021) anticipate shifts in the geographic ranges of native species into novel habitats due to climate change. These changes in distribution patterns among both invasive and native species are expected to have profound ecological implications (Gaertner et al., 2017). The importance of considering these dynamics is also acknowledged when addressing the challenges posed by invasive species in the context of a changing climate (Finch et al., 2021; Hume et al., 2021). The analysis by Finch et al. (2021) provides valuable insights into the interconnected impacts of climate change and invasive species, based on a robust examination of global temperature trends and their implications for ecological systems.
3.3 Sparse assessment of pest and disease forecasting models under climate change
Sparse assessment of pest and disease forecasting models under climate change encompasses the limited evaluation of predictive models designed to anticipate the emergence, spread, and impact of pests and diseases on agricultural and ecological systems amidst shifting climatic conditions (Hamdi et al., 2022; Fassnacht et al., 2024). This field of study is of paramount importance due to the significant threats posed by pests and diseases to global food security, ecosystem health, and economic stability, particularly in the face of climate change-induced alterations in temperature, precipitation patterns, and extreme weather events (Abbass et al., 2022; Mwangi et al., 2023).
Model development involves constructing predictive models based on historical data and assumptions about environmental conditions, incorporating factors such as temperature, humidity, precipitation, host plant physiology, pathogen biology, and insect behaviour to simulate the spread and impact of pests and diseases (Donatelli et al., 2017; Lee and Yun, 2023). Climate change considerations are crucial as climate change alters environmental conditions, including temperature regimes, rainfall patterns, and the frequency of extreme weather events (Lee et al., 2022). Incorporating climate change variables and scenarios into predictive models presents significant challenges due to uncertainties in future climate projections and complex interactions between climate and biological systems (Kamga et al., 2022). Data limitations contribute to sparse assessment, arising from challenges in the availability, quality, and spatial/temporal resolution of data required to calibrate, validate, and refine forecasting models (Petropoulos et al., 2022; Yang et al., 2022). Insufficient data on pest and disease occurrences, environmental variables, and management interventions can hinder the accuracy and reliability of predictive models, particularly in regions with limited monitoring infrastructure and research capacity (Akhter and Sofi, 2022; Dhanaraju et al., 2022; Taylor et al., 2023).
This systematic review emphasizes the critical role of predictive models in developing adaptive strategies for sugarcane sustainability. Decision Support Systems (DSS), such as the DSSAT framework, integrate crop growth models, weather data, and management practices to simulate crop growth and predict pest and disease outbreaks (Equation 1). Tailoring DSSAT for localized climate patterns allows for the anticipation of pest and disease threats, enabling timely interventions.
Where Y is yield, T is temperature, P is precipitation, N is nutrient availability, and W is water availability.
Machine learning models, including Random Forest and Support Vector Machines (SVM), analyze large datasets, including climate variables and historical disease incidence, to forecast future disease outbreaks (Equation 2). These models, trained on localized climate data, are effective in predicting specific disease threats.
Where ŷ is the predicted outcome, and ŷi are the predictions from individual trees in the forest.
Epidemiological models like Susceptible-Exposed-Infectious-Recovered (SEIR) can be adapted to predict the spread of sugarcane diseases by incorporating local climate variables, pest population dynamics, and crop growth stages, thus helping devise targeted control measures (Equation 3).
Where S is susceptible, E is exposed, I is infectious, R is recovered, β is the transmission rate, σ is the incubation rate, and γ is the recovery rate.
Geographic Information Systems (GIS) combined with Species Distribution Models (SDMs) predict pest infestations by analyzing spatial and climatic data (Equation 4), identifying high-risk areas for pest outbreaks and enabling proactive pest management strategies.
Where P(x) is the probability of species presence, β0 is the intercept, βi are coefficients, and xi are the predictor variables.
Climate-driven crop simulation models, such as Agricultural Production Systems sIMulator (APSIM), simulate the growth and yield of sugarcane under different climate scenarios (Equation 5). The APSIM predicts the impact of climate variables on sugarcane growth and the likelihood of pest and disease problems, facilitating the development of adaptive strategies to enhance crop resilience by incorporating localized climate data.
Where G is growth, Gmax is maximum growth, T is temperature, Topt is optimal temperature, Tmin is minimum temperature, and P is precipitation.
Bioeconomic models, which combine climate data with pest and disease management costs, optimize Integrated Pest Management (IPM) strategies by predicting the most cost-effective and sustainable approaches to pest and disease control, tailored to localized climate patterns (Equation 6). These predictive models present the necessity of adapting to localized climate patterns to effectively manage pest and disease threats, ensuring the resilience and productivity of sugarcane cultivation amidst evolving climate conditions.
Where NPV is the net present value, Bt are benefits in year t, Ct are costs in year t, r is the discount rate, and T is the time horizon.
While literature explains upon the importance of monitoring climate and pest populations, there is a notable gap in the exploration of existing or potential pest and disease forecasting models. Climate change not only affects the prevalence of pests and diseases of sugarcane but also alters their spatial and temporal distribution (Srinivasan et al., 2024). Robust forecasting models can play a pivotal role in predicting the occurrence and severity of outbreaks, enabling proactive and targeted management strategies (Subedi et al., 2023).
There exists complex relationship between the explosive growth of the global population, technological advancements, and the challenges posed by climate change to agricultural e production (Subedi et al., 2023). Subedi et al. (2023) indicated that the confluence of these factors has given rise to a complex scenario where the progress that has fuelled increased food demand is under threat due to the adverse impacts of climate change. The significantly acknowledged issues arising from climate change include rising carbon dioxide levels, frequent droughts, and temperature fluctuations (Waheed et al., 2023; Yanagi, 2024). These environmental shifts pose substantial challenges to crop production and, consequently, jeopardize global producing systems. The focus of much literature in this discipline lies in understanding how these climatic variables affect the biology and ecology of insect pests, which play a pivotal role in the delicate balance of agricultural ecosystems (Skendžić et al., 2021; Abbass et al., 2022). Given the dependence of insect pests on climate factors, any alterations in these variables have far-reaching implications for crop productivity (Subedi et al., 2023). The interconnectedness of insect pests, climate change, and crop yields underscores the urgency of comprehending the impact of climate change on insect pest dynamics (Table 2). A key contribution of literature lies in its exploration of modern pest monitoring technologies and prediction tools. Literature advocates for the integration of these advanced tools to enhance ability to monitor and predict pest behaviour in the face of changing climatic conditions. Despite the valuable insights provided by literature, there remain certain research gaps that merit further investigation. One crucial aspect is the need for more extensive field studies to validate the efficacy of the proposed pest monitoring technologies and prediction tools in diverse agricultural settings (Chawade et al., 2019; Preti et al., 2021). Additionally, understanding the socio-economic impacts of implementing these technologies, especially in resource-limited regions, is crucial for ensuring the practicality and accessibility of these strategies (Maduka et al., 2023). Furthermore, studies emphasize the importance of climate variables in influencing insect pest biology, but there is room for additional research into the potential feedback loops between insect pests and climate change (Barton et al., 2019; Yang et al., 2021). How, for instance, changes in pest populations may further exacerbate climate issues or create new challenges for agricultural sustainability is an intriguing avenue for future exploration.
These gaps may be addressed by the research that could include a more thorough evaluation of existing forecasting models, examining their applicability to changing climate conditions (Balogun et al., 2020). Moreover, it could explore the development of new models that incorporate climate change variables, providing accurate predictions for sugarcane growers. This emphasis on forecasting would empower stakeholders with timely information, helping them anticipate and mitigate the impact of emerging pest and disease threats in the context of a changing climate (Rocklöv et al., 2023). By incorporating these considerations, the research could bridge critical gaps and offer a more holistic understanding of the relationship between climate change, sugarcane pests and diseases, and the adaptive measures necessary for sustaining the industry (Grandis et al., 2024).
3.4 Impact of climate change on plant growth-reducing factors of sugarcane
Sugarcane growth is significantly hindered by insect pests, diseases, and weeds, with climate change exacerbating these challenges. Elevated temperatures and altered precipitation patterns enhance pest populations and disease prevalence, disrupting plant health. Weeds thrive under these warmer conditions, competing aggressively with sugarcane and reducing yields. Climate change also disrupts the phenology and life cycles of pests, diseases, and weeds, making traditional management practices less effective. These shifts necessitate integrated management strategies, including cultural, biological, and chemical controls, alongside precision agriculture and adaptive breeding programs to develop resilient sugarcane varieties and ensure sustainable cultivation amidst evolving climatic conditions. The details of these factors are described in subsequent sections.
3.4.1 Influence of climate change on insect pests of sugarcane
Climate change profoundly influences insect pest dynamics in sugarcane cultivation, complicating efforts to maintain sustainable production. The relationship between shifting climatic conditions and pest behaviour necessitates a comprehensive understanding to develop long-term strategies for sustainability (Weier et al., 2024). A primary effect of climate change is the alteration of temperature patterns (Balasundram et al., 2023; Bibi and Rahman, 2023; Johnson, 2023; Singh et al., 2023). Pests such as white scales (Aulacaspis tegalensis) (Sarjan et al., 2021), white grubs (Cochliotis melolonthoides) (Kumar and Prasad, 2020), and yellow sugarcane aphids (Sipha flava) (Dumont et al., 2023) impact sugarcane productivity globally.
The yellow sugarcane aphid (YSA) is particularly sensitive to temperature variations (Skendžić et al., 2021; Bhattacharyya et al., 2023; Bouri et al., 2023; Prajapati et al., 2023). Climate change-induced temperature increases expand YSA’s suitable habitat range, leading to greater prevalence in sugarcane fields (Chen et al., 2023; Li et al., 2024; Nikpay et al., 2023). Higher temperatures also accelerate YSA’s reproductive capacity, exacerbating challenges for growers (Bhagarathi and Maharaj, 2023; Walia and Kaur, 2024). Changing rainfall patterns further contribute to insect pest proliferation in sugarcane fields (Valencia Arbeláez et al., 2021). Altered precipitation affects water availability, influencing pest abundance and distribution (Subedi et al., 2023). In regions with changing rainfall, pests like white scales, white grubs, and YSA may find new ecological niches, shifting population dynamics (See Table 3). This necessitates adaptive pest management strategies that consider evolving climatic conditions (Janousek et al., 2023; Waheed et al., 2023).
Climate change also alters the phenology and behaviour of insect pests (Subedi et al., 2023; Shafiq et al., 2024). Shifts in life stage timing, such as reproduction and larval development, are linked to climatic signals. Disruptions in these signals can increase pest pressure on sugarcane crops, impacting yield and quality (Tait et al., 2021; Zheng et al., 2024). For instance, the reproductive patterns of Heteronychus paolii Arrow (Dynastinae) and Schizonycha vastatrix Chiaramonte (Melolonthinae) are influenced by temperature and rainfall variability, causing population fluctuations (Cock and Allard, 2013). Extreme weather events associated with climate change, such as hurricanes and cyclones, complicate insect pest dynamics in sugarcane cultivation (Cilas and Bastide, 2020; Volk et al., 2023). These events disrupt habitats and pest behaviour, leading to altered infestation patterns (Volk et al., 2023). Post-hurricane conditions may favour rapid pest proliferation, threatening sugarcane production sustainability (Palanivel and Shah, 2021).
3.4.2 Influence of climate change on diseases of sugarcane
Climate change presents numerous challenges to sugarcane production, particularly through its influence on disease pathogens (Savary and Willocquet, 2020). Understanding the relationship between changing climatic conditions and sugarcane diseases is crucial for developing effective long-term sustainability strategies (See Table 4). One significant impact of climate change on sugarcane diseases is the alteration in temperature patterns (Linnenluecke et al., 2020). Rising global temperatures create favourable conditions for the proliferation of certain disease pathogens. For instance, smut disease, caused by the fungus Sporisorium scitamineum, thrives in warmer conditions (Bhuiyan et al., 2021; Rajput et al., 2021). Increased temperatures associated with climate change contribute to the incidence and severity of smut, posing a substantial threat to sugarcane yields. Similarly, leaf scald, caused by the bacterium Xanthomonas alibilineans, is influenced by temperature shifts, with high temperatures exacerbating its symptoms (Bini et al., 2023; Li et al., 2024).
Changing rainfall patterns also play a pivotal role in the spread and severity of sugarcane diseases (Kim et al., 2024). Climate change leads to shifts in rainfall timing, distribution, and intensity, affecting the water-dependent life cycles of disease-causing organisms. Gummosis, caused by the bacterium Xanthomonas axonopodis pv. vasculorum, is highly influenced by moisture conditions (Hussain et al., 2024; Li et al., 2024; Parthasarathy et al., 2024). Altered rainfall patterns can facilitate the spread of this bacterium or create conducive conditions for its development, increasing gummosis incidences in sugarcane fields. Leaf rust, caused by Puccinia melanocephala, is also sensitive to moisture levels, with deviations from normal rainfall patterns affecting its prevalence (Wójtowicz et al., 2020).
The geographical distribution and behaviour of disease vectors, such as insects and fungi, are influenced by climate change. These vectors are crucial in transmitting pathogens, and their altered patterns contribute to disease spread in sugarcane fields. Climate-induced shifts in the distribution and behaviour of pests like white scales, white grubs, and yellow sugarcane aphids can lead to variations in disease transmission patterns, further complicating disease dynamics in sugarcane cultivation (Dumont et al., 2023).
Extreme weather events associated with climate change, such as hurricanes and cyclones, also impact sugarcane disease dynamics (Badillo-Márquez et al., 2021; Christina et al., 2021). These events can cause physical damage to sugarcane plants, creating entry points for pathogens and facilitating disease spread. Hurricanes and cyclones can increase sugarcane crops’ vulnerability to diseases like leaf rust and smut (Ahmad, 2023). Additionally, the aftermath of extreme weather events can create conditions favourable for rapid pathogen proliferation, threatening sugarcane production sustainability (Singh et al., 2018; Noureen et al., 2022).
3.4.3 Influence of climate change on notorious weeds of sugarcane
Climate change has a multifaceted impact on the dynamics of notorious weeds affecting sugarcane cultivation, presenting challenges to the sustainability of this vital crop (Chaki et al., 2023; Vasileiou et al., 2024; Nath et al., 2024). The interplay between shifting climatic conditions and the behaviour of noxious weeds requires a comprehensive understanding to develop effective strategies for long-term sustainability.
One of the primary effects of climate change on weeds is the alteration in temperature and precipitation patterns (Sreekanth et al., 2023; Kachare et al., 2024). Rising global temperatures influence the germination, growth, and reproductive processes of weeds, with some species thriving in warmer conditions. Weeds such as Imperata cylindrica (cogon grass) and Sorghum halepense (Johnson grass), recognized as notorious competitors with sugarcane, may experience increased vigour and expansion of their ecological range in response to elevated temperatures (Shumail et al., 2022; Othman et al., 2023; Tan et al., 2024). Changes in precipitation patterns, including altered onset and distribution of rainfall, further contribute to the weed proliferation challenge. Weeds like Cyperus rotundus (purple nutsedge) and Echinochloa colona (jungle rice) are adapted to varying moisture conditions, and climate-induced shifts in precipitation can create favourable habitats for these aggressive competitors (Eslami and Arpanahi, 2023; Keerthi Sree et al., 2023; Mahgoub, 2023).
Climate change also influences the phenology and life cycles of notorious weeds in sugarcane fields (Pires da Silva et al., 2014; Giraldeli et al., 2021). The timing of seed germination, emergence, and flowering is intricately linked to environmental cues, including temperature and photoperiod. As these cues undergo alterations due to climate change, the phenological patterns of weeds may be disrupted, impacting their synchronization with sugarcane crops (Anwar et al., 2021; Deeksha et al., 2022; Santosh and Pavithran, 2024). This mismatch in timing can result in increased weed pressure on susceptible crops, affecting overall yields and hindering sustainable sugarcane production. The impact is particularly pronounced with weeds like I. cylindrica, known for its aggressive growth and ability to outcompete sugarcane in various agro-climatic conditions (Sivakumar et al., 2018).
Changing climatic conditions also influence the distribution and spread of notorious weeds, creating challenges for weed management practices (Anwar et al., 2021; Rao et al., 2023). Most notorious weeds often disperse their seeds through wind, water, or human activities (Balah, 2021; Khattak et al., 2024). Climate-induced alterations in wind patterns and increased frequency of extreme weather events can facilitate the long-distance dispersal of weed seeds, leading to the colonization of new areas (Table 5). This expansion of weed distribution zones poses a significant threat to the sustainability of sugarcane cultivation, as it increases the likelihood of weed infestations in previously unaffected regions (Allsopp et al., 2021; Baltazar and De Datta, 2023).
Extreme weather events associated with climate change, such as hurricanes, cyclones, and floods, further exacerbate the challenges posed by notorious weeds in sugarcane fields. These events can disrupt weed management practices and create conditions conducive to rapid weed proliferation. For example, flooding can displace weed seeds and rhizomes, redistributing them across fields and complicating efforts to control their spread (Smith et al., 2021; Haring, 2022). The aftermath of extreme weather events may necessitate intensive weed control measures to prevent the establishment of weed populations that could jeopardize the health and productivity of sugarcane crops.
3.5 Managing insect pests, diseases, and weeds in sugarcane fields amid climate change
Integrated management practices are vital for addressing the complex challenges posed by insect pests, diseases, and notorious weeds in sugarcane fields, especially in the context of climate change. These integrated approaches combine cultural, biological, and chemical control measures to mitigate impacts while minimizing environmental risks. Integrated Pest Management (IPM) strategies include crop rotation, the use of natural predators, and targeted pesticide applications (Koshariya et al., 2023; Lantero et al., 2023). These measures collectively help in reducing pest populations and protecting the environment (Baste and Watson, 2022; Morya and Kumar, 2023). Adaptive breeding programs are essential, focusing on developing pest-resistant sugarcane varieties that can adapt to evolving pest pressures, such as breeding for YSA resistance (Bakala et al., 2020; Naqvi et al., 2022; Shahid et al., 2024).
Integrated Disease Management (IDM) practices involve adjusting planting schedules, optimizing irrigation, and using biological controls such as natural predators or beneficial microorganisms (Bhuiyan et al., 2021; Ram et al., 2022). Chemical control, when carefully managed, remains a crucial tool against disease outbreaks (Grigorieva et al., 2023; Gyamfi et al., 2024). Breeding programs are also vital in developing disease-resistant varieties by incorporating traits that enhance resistance to specific pathogens influenced by climate change (Legros et al., 2021; Nunes et al., 2024; Parveen and Rashtrapal, 2024).
Integrated Weed Management (IWM) combines cultural, mechanical, biological, and chemical control measures to manage weed populations effectively (Scavo and Mauromicale, 2020; Kousta et al., 2024). Strategies such as crop rotation, cover cropping, and targeted herbicide applications play integral roles in IWM, enhancing sugarcane resilience against weed encroachment (Cherubin et al., 2021; Schrader et al., 2024). Adaptive breeding programs also focus on developing sugarcane varieties that can better compete with and resist notorious weeds, ensuring sustainable production amidst evolving climatic conditions (Gobu et al., 2020; van Antwerpen et al., 2022; Geng and Yufeng, 2023; Spelman, 2024).
Precision agriculture utilizes cutting-edge technologies such as drones, sensors, and data analytics to offer transformative real-time monitoring and management of pests, diseases, and weeds (Ayoub-Shaikh et al., 2022; Karunathilake et al., 2023). This approach enhances resource efficiency and reduces environmental impacts through optimized interventions (Ahmad, 2023; Nikpay et al., 2023). Precision agriculture aligns with the broader goal of sustainable and efficient farming practices, ensuring the resilience of sugarcane cultivation amid changing climatic conditions (Grandis et al., 2024; Pachiappan et al., 2024; Shaktawat and Swaymprava, 2024).
Early detection of pest, disease, and weed infestations allows for targeted interventions, optimizing resource use and minimizing impacts on sugarcane (Mylonas et al., 2020; He et al., 2024). Research into innovative resistant varieties and novel control methods is essential for staying ahead of emerging challenges in sugarcane cultivation (Storkey et al., 2021; Gautam and Hens, 2022; Roy et al., 2023; Kamath et al., 2024). Continued research and innovation are crucial for sustainable sugarcane cultivation in a changing climate, as they inform adaptive management strategies and develop resistant varieties and novel control methods (Hill et al., 2011; Bordonal et al., 2018; Singh et al., 2019; Ali et al., 2023). Integrating IPM, IDM, and IWM strategies with precision agriculture and early detection ensures sustainable and productive sugarcane cultivation worldwide (Maxwell et al., 2019; Mercer, 2020; Walsh et al., 2020; Srinivasa Rao et al., 2022). These approaches help the sugarcane industry navigate the complexities of climate change and ensure long-term resilience (Chen et al., 2023; Harvey et al., 2023; Ranganathan et al., 2023).
3.6 Climate-resilient strategies for disease and pest management in sugarcane
Adaptive strategies play a pivotal role in mitigating the impact of climate change on sugarcane diseases and insect pests, offering prospects for the long-term sustainability of sugarcane cultivation (Shahzad et al., 2021; Bhatt et al., 2023; Kurmi et al., 2024). These strategies encompass a holistic approach that addresses the dynamic interactions between shifting climatic conditions and the complex ecosystems harbouring pathogens and pests. By integrating adaptive measures, the sugarcane industry can enhance resilience, minimize losses, and secure the sustainability of this crucial crop. One key adaptive strategy involves the development and implementation of climate-resilient sugarcane varieties (Taylor et al., 2023). Understanding the evolving dynamics of diseases and insect pests influenced by climate change allows breeders to incorporate traits that confer resistance or tolerance. For instance, breeding for resistance against yellow sugarcane aphids can lead to the creation of varieties capable of withstanding the changing pest pressures (Ademe et al., 2024). These climate-resilient varieties act as a frontline defence, reducing the susceptibility of sugarcane crops to diseases and pests, thereby contributing to sustainability in the face of climatic uncertainties.
Integrated disease management (IDM) practices represent a comprehensive adaptive strategy that combines cultural, biological, and chemical control measures to manage diseases effectively (Taylor et al., 2023). Cultural practices, such as adjusting planting schedules and optimizing irrigation, are tailored to mitigate disease risks influenced by climate change (Mishra and Mishra, 2023; Ademe et al., 2024). Biological control methods involve the introduction of natural predators or beneficial microorganisms to regulate disease vectors (Muhammad et al., 2024; Barathi et al., 2024). Chemical control, while being judiciously managed to minimize environmental impact, remains an essential tool in the arsenal against disease outbreaks (Collett et al., 2020; Dolatabadian et al., 2022). The IDM allows for a dynamic and adaptable approach, ensuring the sustainability of sugarcane production amidst the changing disease landscape. The use of drones, sensors, and data analytics in precision agriculture practices provide valuable insights into the status of crops, allowing early detection of outbreaks and targeted interventions (Shahi et al., 2023; Chaudhari et al., 2024). By staying ahead of disease and pest dynamics through real-time monitoring, precision agriculture contributes to the resilience of sugarcane cultivation in the face of evolving climatic conditions.
Climate-resilient agronomic practices play a crucial role in adapting sugarcane cultivation to the changing climate (del Pozo et al., 2019; Röling, 2019). Optimizing planting schedules, irrigation practices, and crop rotations based on climatic conditions helps mitigate the risks associated with diseases and insect pests (Richard et al., 2022; Kowalska et al., 2023). These adaptive practices ensure that sugarcane crops are strategically positioned to withstand varying environmental stresses. Farmers can proactively address challenges posed by diseases and pests, promoting the long-term health and sustainability of sugarcane crops by aligning agronomic activities with the evolving climatic conditions (Usman et al., 2020; Gonçalves et al., 2024).
On-going innovation ensures that the sugarcane industry remains dynamic and resilient in the face of climate-induced uncertainties (Aggarwal et al., 2022; Anekwe et al., 2023). Economic thresholds, another vital aspect of adaptive strategies, offer a systematic approach to making treatment decisions for diseases and insect pests. Determining the pest density at which management action should be taken to prevent economic losses guides intervention strategies (Hendrichs et al., 2021; Wolff, 2023). Economic thresholds are dynamic and can vary based on factors such as crop variety, growth stage, market value, and growing conditions. Ensuring effective control while minimizing unnecessary interventions by establishing and regularly updating economic thresholds, sugarcane growers can optimize pest management practices (Nikpay et al., 2023; Mangena et al., 2024; Reddy et al., 2024).
Adaptive strategies for sugarcane sustainability involve monitoring and predicting climate trends to anticipate future challenges (Linnenluecke et al., 2018; Grigorieva et al., 2023). Studying trends in rainfall and temperature over an extended period in sugarcane cultivation areas allows for the identification of potential shifts in disease and pest prevalence (Caron et al., 2018; Khatri et al., 2023). Growers can proactively plan interventions and implement adaptive measures by understanding how climate variables influence the dynamics of pathogens and pests (Heeb et al., 2019; Taylor et al., 2021). This forward-looking approach enables the development of climate adaptation and mitigation actions necessary to contain crop pests and diseases, fostering sustainability in the sugarcane industry (Altieri, 2002; Grigorieva et al., 2023; Mrabet, 2023). Adaptive strategies play a critical role in mitigating the impact of climate change on sugarcane diseases and insect pests, offering prospects for sustainability in the face of evolving climatic conditions (Shahzad et al., 2021). Climate-resilient agronomic practices form a comprehensive toolkit for the sugarcane industry (Tan et al., 2022; Alotaibi, 2023; Lopes, 2023).
4 Conclusions
Climate change exerts a profound impact on sugarcane production, affecting insect pests, diseases, and notorious weeds. Variations in temperature, altered rainfall patterns, and extreme weather events significantly reshape pest and disease dynamics, posing severe challenges to sustainable sugarcane cultivation. Integrated pest and disease management practices are essential to adapt to these changing environmental conditions. Implementing comprehensive control methods can help mitigate the effects of climate change on pest and disease proliferation. Adaptive breeding is another critical strategy, focusing on developing climate-resilient sugarcane varieties. These varieties are better equipped to withstand shifting climates and maintain productivity. Precision agriculture utilizes advanced technologies to monitor and manage crop health and environmental conditions more effectively. This approach can significantly enhance the ability to respond to climate-induced changes in pest and disease behaviour. Early detection and monitoring systems are crucial for the timely identification and control of pest and weed outbreaks. Establishing these systems can help manage infestations before they cause significant damage to sugarcane crops. Ongoing research and innovation are vital to continuously advancing knowledge and techniques to combat the effects of climate change on sugarcane cultivation. By staying at the forefront of agricultural science, the industry can better adapt to evolving climatic conditions. The sugarcane industry can enhance its resilience, minimize potential losses, and ensure the sustainability of this vital crop amidst the challenges posed by climate change.
Data availability statement
The original contributions presented in the study are included in the article/supplementary material. Further inquiries can be directed to the corresponding authors.
Author contributions
BM: Conceptualization, Data curation, Formal analysis, Funding acquisition, Investigation, Methodology, Project administration, Resources, Software, Supervision, Validation, Visualization, Writing – original draft, Writing – review & editing. PN: Supervision, Validation, Visualization, Writing – review & editing. CJ: Supervision, Validation, Visualization, Writing – review & editing.
Funding
The author(s) declare that no financial support was received for the research, authorship, and/or publication of this article.
Conflict of interest
The authors declare that the research was conducted in the absence of any commercial or financial relationships that could be construed as a potential conflict of interest.
Publisher’s note
All claims expressed in this article are solely those of the authors and do not necessarily represent those of their affiliated organizations, or those of the publisher, the editors and the reviewers. Any product that may be evaluated in this article, or claim that may be made by its manufacturer, is not guaranteed or endorsed by the publisher.
References
Abbass K., Qasim M. Z., Song H., Murshed M., Mahmood H., Younis I. (2022). A review of the global climate change impacts, adaptation, and sustainable mitigation measures. Environ. Sci. pollut. Res. 29, 42539–42559. doi: 10.1007/s11356-022-19718-6
Ademe D., Tesfaye K., Simane B., Zaitchik B. F., Alemayehu G., Adgo E. (2024). Optimizing agronomic practices to harness climate change impacts on potato production in tropical highland regions. Eur. J. Agron. 152, 127021. doi: 10.1016/j.eja.2023.127021
Adesipo A., Fadeyi O., Kuca K., Krejcar O., Maresova P., Selamat A., et al. (2019). Smart and climate-smart agricultural trends as core aspects of smart village functions. Sensors 20, 5977. doi: 10.3390/s20215977
Aggarwal P., Roy J., Pathak H., Naresh Kumar S., Venkateswarlu B., Ghosh A., et al. (2022). “Managing climatic risks in agriculture,” in Indian Agriculture Towards 2030: Pathways for Enhancing Farmers’ Income, Nutritional Security and Sustainable Food and Farm Systems (Springer Nature Singapore, Singapore), 83–108. Available at: https://link.springer.com/bookseries/11234.
Ahmad H. H. (2023). Effects of urbanization and green house gases on sugarcane growth. Int. J. Agric. Sustain. Dev. 5, 32–40.
Akhter R., Sofi S. A. (2022). Precision agriculture using IoT data analytics and machine learning. J. King Saud Univ. - Comput. Inf. Sci. 34, 5602–5618. doi: 10.1016/j.jksuci.2021.05.013
Alengebawy A., Abdelkhalek S. T., Qureshi S. R., Wang M. (2021). Heavy metals and pesticides toxicity in agricultural soil and plants: Ecological risks and human health implications. Toxics 9, 42. doi: 10.3390/toxics9030042
Alexander L. V. (2016). Global observed long-term changes in temperature and precipitation extremes: A review of progress and limitations in IPCC assessments and beyond. Weather Climate Extremes 11, 4–16. doi: 10.1016/j.wace.2015.10.007
Ali M. A., Abdellah I. M., Eletmany M. R. (2023). Towards sustainable management of insect pests: Protecting food security through ecological intensification. Int. J. Chem. Biochem. Sci. 24, 386–394.
Allsopp P. G., Croft B., Fillols E. (2021). Situation analysis and opportunities for pest, disease and weed RD&A (including biosecurity) in Australian sugarcane: final report 2019/015. Available online at: https://hdl.handle.net/11079/18130.
Alotaibi M. (2023). Climate change, its impact on crop production, challenges, and possible solutions. Notulae Botanicae Horti Agrobotanici Cluj-Napoca 51, 13020–13020. doi: 10.15835/nbha51113020
Altieri M. A. (2002). Agroecology: the science of natural resource management for poor farmers in marginal environments. Agriculture Ecosyst. Environ. 93, 1–24. doi: 10.1016/S0167-8809(02)00085-3
Andrieu N., Howland F., Le Coq J., Milena A., Loboguerrero A. M., Chia E. (2019). Co-designing climate-smart farming systems with local stakeholders: A methodological framework for achieving large-scale change. Front. Sustain. Food Syst. 3. doi: 10.3389/fsufs.2019.00037
Anekwe I. M. S., Zhou H., Mkhize M. M., Akpasi S.O. (2023). Addressing Climate Change Challenges in South Africa: A Study in KwaZulu Natal Province. In: Chatterjee U., Shaw R., Kumar S., Raj A. D., Das S. (eds) Climate Crisis: Adaptive Approaches and Sustainability. Sustainable Development Goals Series. (Springer, Cham). doi: 10.1007/978-3-031-44397-8_24
Anwar M. P., Islam A. K., Yeasmin S., Rashid M. H., Juraimi A. S., Ahmed S., et al. (2021). Weeds and their responses to management efforts in a changing climate. Agronomy 11, 1921. doi: 10.3390/agronomy11101921
Araújo S. O., Peres R. S., Ramalho J. C., Lidon F., Barata J. (2023). Machine learning applications in agriculture: Current trends, challenges, and future perspectives. Agronomy 13, 2976. doi: 10.3390/agronomy13122976
Archana T. S., Kumar D., Ghosh P., Kumar V. (2024). Plant pathogen detection: exploring modern techniques and future directions. Indian Phytopathol., 1–13. doi: 10.1007/s42360-024-00762-w
Askarianzadeh A., Minaeimoghadam M. (2018). Biology, natural enemies and damage of the sugarcane whitefly (Neomaskellia andropogonis) (Homoptera: Aleyrodidae) in Iran. Int. J. Trop. Insect Sci. 38, 381–386. doi: 10.1017/S1742758418000164
Awasthi L. P., Singh S. P., Verma H. N. (2015). “Role of defensive antiviral proteins from higher plants in the management of viral diseases,” in Recent Advances in the Diagnosis and Management of Plant Diseases. Ed. Awasthi L. P. (Springer, New Delhi). doi: 10.1007/978-81-322-2571-3
Ayoub-Shaikh T., Rasool T., Rasheed Lone F. (2022). Towards leveraging the role of machine learning and artificial intelligence in precision agriculture and smart farming. Comput. Electron. Agric. 198, 107119. doi: 10.1016/j.compag.2022.107119
Aysu T. (2016). A study on pyrolysis of Canada thistle (Cirsium arvense) with titania based catalysts for bio-fuel production. Bioresource Technol. 219, 175–184. doi: 10.1016/j.biortech.2016.07.132
Badillo-Márquez A. E., Aguilar-Lasserre A. A., Miranda-Ackerman M. A., Sandoval-González O. O., Villanueva-Vásquez D., Posada-Gómez R. (2021). An agent-based model-driven decision support system for assessment of agricultural vulnerability of sugarcane facing climatic change. Mathematics 9, 3061. doi: 10.3390/math9233061
Bakala H. S., Singh G., Srivastava P. (2020). “Smart breeding for climate resilient agriculture,” in Plant breeding-current and future views (London, United Kingdom: IntechOpen). doi: 10.5772/intechopen.94847
Balah M. A. (2021). “Ecological management of weeds in desert regions,” in Management and Development of Agricultural and Natural Resources in Egypt’s Desert (Springer International Publishing, Cham), 291–315. doi: 10.1007/978–3-030–73161-8_11
Balasundram S. K., Shamshiri R. R., Sridhara S., Rizan N. (2023). The role of digital agriculture in mitigating climate change and ensuring food security: An overview. Sustainability 15, 5325. doi: 10.3390/su15065325
Balogun A. L., Marks D., Sharma R., Shekhar H., Balmes C., Maheng D., et al. (2020). Assessing the potentials of digitalization as a tool for climate change adaptation and sustainable development in urban centres. Sustain. Cities Soc. 53, 101888. doi: 10.1016/j.scs.2019.101888
Baltazar A. M., De Datta S. K. (2023). Weed Science and Weed Management in Rice and Cereal-Based Cropping Systems, 2 Volumes (United States: John Wiley & Sons). doi: 10.1002/9781119737582
Bam A., Addison P., Conlong D. (2020). Acridid ecology in the sugarcane agro-ecosystem in the Zululand region of KwaZulu-Natal, South Africa. J. Orthoptera Res. 29, 9–16. doi: 10.3897/jor.29.34626
Barathi S., Sabapathi N., Kandasamy S., Lee J. (2024). Present status of insecticide impacts and eco-friendly approaches for remediation-a review. Environ. Res. 240, 117432. doi: 10.1016/j.envres.2023.117432
Bartels W. L., Furman C. A., Diehl D. C., Royce F. S., Dourte D. R., Ortiz B. V., et al. (2013). Warming up to climate change: A participatory approach to engaging with agricultural stakeholders in the Southeast US. Regional Environ. Change 13, 45–55. doi: 10.1007/s10113-012-0371-9
Barton M. G., Terblanch J. S., Sinclair B. J. (2019). Incorporating temperature and precipitation extremes into process-based models of African Lepidoptera changes the predicted distribution under climate change. Ecol. Model. 394, 53–65. doi: 10.1016/j.ecolmodel.2018.12.017
Baste I. A., Watson R. T. (2022). Tackling the climate, biodiversity and pollution emergencies by making peace with nature 50 years after the stockholm conference. Global Environ. Change 73, 102466. doi: 10.1016/j.gloenvcha.2022.102466
Behnam-Oskuyee S., Ziaee M., Shishehbor P. (2020). Evaluation of different insecticides for the control of sugarcane whitefly, Neomaskellia andropogonis Corbett (Homoptera: Aleyrodidae). J. Saudi Soc. Agric. Sci. 19, 255–260. doi: 10.1016/j.jssas.2018.11.004
Bhagarathi L. K., Maharaj G. (2023). Impact of climate change on insect biology, ecology, population dynamics, and pest management: A critical review. World J. Adv. Res. Rev. 19 (3), 541–568. doi: 10.30574/wjarr.2023.19.3.1843
Bhatt R., Kumar R., Kashyap L., Alataway A., Dewidar A. Z., Mattar M. A. (2022). Growth, yield, quality and insect-pests in sugarcane (Saccharum officinarum) as affected by differential regimes of irrigation and potash under stressed conditions. Agronomy 12, 1942. doi: 10.3390/agronomy12081942
Bhatt C. M., Patel P., Ghetia T., Mazzeo P. L. (2023). Effective heart disease prediction using machine learning techniques. Algorithms 16 (2), 88. doi: 10.3390/a16020088
Bhattacharyya B., Gogoi I., Das P. P. G., Kalita B. (2023). “Management of agricultural insect pests for sustainable agriculture and environment,” in Sustainable Agriculture and the Environment (Amsterdam, The Netherlands: Academic Press), 161–193. doi: 10.1016/B978-0-323-90500-8.00001-4
Bhuiyan S. A., Magarey R. C., McNeil M. D., Aitken K. S. (2021). Sugarcane smut, caused by Sporisorium scitamineum, a major disease of sugarcane: A contemporary review. Phytopathology 111, 1905–1917. doi: 10.1094/PHYTO-05-21-0221-RVW
Bibi F., Rahman A. (2023). An overview of climate change impacts on agriculture and their mitigation strategies. Agriculture 13, 1508. doi: 10.3390/agriculture13081508
Bini A. P., Rossi G. D., Poeschl Y., Serra M. C. D., Camargo L. E. A., Monteiro-Vitorello C. B., et al. (2023). Molecular, biochemical and metabolomics analyses reveal constitutive and pathogen-induced defense responses of two sugarcane contrasting genotypes against leaf scald disease. Plant Physiol. Biochem. 203, 108033. doi: 10.1016/j.plaphy.2023.108033
Bordonal R. D. O., Carvalho J. L. N., Lal R., de Figueiredo E. B., de Oliveira B. G., La Scala N. (2018). Sustainability of sugarcane production in Brazil. A review. Agron. Sustain. Dev. 38, 1–23. doi: 10.1007/s13593-018-0490-x
Boudh S., Singh J. S. (2019). “Pesticide contamination: environmental problems and remediation strategies,” in Emerging and eco-friendly approaches for waste management 2018, 245–269. doi: 10.1007/978–981-10–8669-4_12
Bouri M., Arslan K. S., Şahin F. (2023). Climate-smart pest management in sustainable agriculture: promises and challenges. Sustainability 15, 4592. doi: 10.3390/su15054592
Bowling R. D., Brewer M. J., Kerns D. L., Gordy J., Seiter N., Elliott N. E., et al. (2016). Sugarcane aphid (Hemiptera: Aphididae): A new pest on sorghum in north america. J. Integr. Pest Manage. 7 (1). doi: 10.1093/jipm/pmw011
Brewer M. J., Gordy J. W., Kerns D. L., Woolley J. B., Rooney W. L., Bowling R. D. (2017). Sugarcane aphid population growth, plant injury, and natural enemies on selected grain sorghum hybrids in texas and louisiana. J. Economic Entomol 110 (5), 2109–2118. doi: 10.1093/jee/tox204
Budeguer F., Enrique R., Perera M. F., Racedo J., Castagnaro A. P., Noguera A. S., et al. (2021). Genetic transformation of sugarcane, current status and future prospects. Front. Plant Sci. 12. doi: 10.3389/fpls.2021.768609
Calvin W., Beuzelin J. M., Liburd O. E., Branham M. A., Jean Simon L. (2021). Effects of biological insecticides on the sugarcane aphid, Melanaphis sacchari (Zehntner) (Hemiptera: Aphididae), in sorghum. Crop protection 142, 105528. doi: 10.1016/j.cropro.2020.105528
Caron P., Ferrero y de Loma-Osorio G., Nabarro D., Hainzelin E., Guillou M., Andersen I., et al. (2018). Food systems for sustainable development: proposals for a profound four-part transformation. Agron. Sustain. Dev. 38, 1–12. doi: 10.1007/s13593-018-0519-1
Cervantes-Romero B., Pérez-Rodríguez P., Rott P., Valdez-Balero A., Osnaya-González M., Robledo-Paz A., et al. (2021). Distribution, phylogeny, and pathogenicity of Xanthomonas albilineans causing sugarcane leaf scald in Mexico. Crop Prot. 150, 105799. doi: 10.1016/j.cropro.2021.105799
Chaiyana A., Khiripet N., Ninsawat S., Siriwan W., Shanmugam M. S., Virdis S. G. (2024). Mapping and predicting cassava mosaic disease outbreaks using earth observation and meteorological data-driven approaches. Remote Sens. Applications: Soc. Environ. 35, 101231. doi: 10.1016/j.rsase.2024.101231
Chaki A. K., Zahan T., Hossain M. S., Ferdous Z., Islam M. A., Islam M. T., et al. (2023). Climate-Smart Agriculture Technologies and Practices in Bangladesh (Dhaka, Bangladesh: SAARC Agriculture Centre, SAARC), 111p. Available at: https://www.researchgate.net/publication/376812477.
Chaudhari P., Patil R. V., Mahalle P. N. (2024). Machine learning-based detection and extraction of crop diseases: A comprehensive study on disease patterns for precision agriculture. Int. J. Intelligent Syst. Appl. Eng. 12, 394–406.
Chawade A., Van Ham J., Blomquist H., Bagge O., Alexandersson E., Ortiz R. (2019). High-throughput field-phenotyping tools for plant breeding and precision agriculture. Agronomy 9, 258. doi: 10.3390/agronomy9050258
Chen Y., Yang J., Wang Y., Qi J., Luan Q. (2023). The impact on climate change perception and adaptation of smallholder concepts: evidence from a sugarcane community, China. Environ. Impact Assess. Rev. 102, 107213. doi: 10.1016/j.eiar.2023.107213
Cherubin M. R., Carvalho J. L. N., Cerri C. E. P., Nogueira L. A. H., Souza G. M., Cantarella H. (2021). Land use and management effects on sustainable sugarcane-derived bioenergy. Land 10, 72. doi: 10.3390/land10010072
Christina M., Jones M. R., Versini A., Mézino M., Le Mezo L., Auzoux S., et al. (2021). Impact of climate variability and extreme rainfall events on sugarcane yield gap in a tropical Island. Field Crops Res. 274, 108326. doi: 10.1016/j.fcr.2021.108326
Cilas C., Bastide P. (2020). Challenges to cocoa production in the face of climate change and the spread of pests and diseases. Agronomy 10, 1232. doi: 10.3390/agronomy10091232
Cock M. J., Allard G. B. (2013). Observations on white grubs affecting sugar cane at the Juba Sugar Project, South-Western Somalia, in the 1980s, and implications for their management. Insects 4, 241–272. doi: 10.3390/insects4020241
Collett S. R., Smith J. A., Boulianne M., Owen R. L., Gingerich E., Singer R. S., et al. (2020). Principles of disease prevention, diagnosis, and control. Dis. Poultry, 1–78. doi: 10.1002/9781119371199.ch1
Daphal S. D., Koli S. M. (2023). Enhancing sugarcane disease classification with ensemble deep learning: A comparative study with transfer learning techniques. Heliyon 9, e18261. doi: 10.1016/j.heliyon.2023.e18261
Das U., Ansari M. A. (2021). The nexus of climate change, sustainable agriculture and farm livelihood: contextualizing climate smart agriculture. Climate Res. 84, 23–40. doi: 10.3354/cr01648
Deeksha M. G., Khan M. S., Kumaranag K. M. (2022). Plant-pollinator interaction network among the scrubland weed flora from foothills of north-western Indian Himalaya. Int. J. Trop. Insect Sci. 42, 1593–1603. doi: 10.1007/s42690-021-00681-7
Delcour I., Spanoghe P., Uyttendaele M. (2015). Literature review: Impact of climate change on pesticide use. Food Res. Int. 68, 7–15. doi: 10.1016/j.foodres.2014.09.030
del Pozo A., Brunel-Saldias N., Engler A., Ortega-Farias S., Acevedo-Opazo C., Lobos G. A., et al. (2019). Climate change impacts and adaptation strategies of agriculture in Mediterranean-climate regions (MCRs). Sustainability 11, 2769. doi: 10.3390/su11102769
Dessoky E. S., Ismail R. M., Elarabi N. I., Abdelhadi A. A., Abdallah N. A. (2021). Improvement of sugarcane for borer resistance using Agrobacterium mediated transformation of cry1Ac gene. GM Crops Food 12, 47–56. doi: 10.1080/21645698.2020.1809318
Dhanaraju M., Chenniappan P., Ramalingam K., Pazhanivelan S., Kaliaperumal R. (2022). Smart farming: Internet of Things (IoT)-based sustainable agriculture. Agriculture 12, 1745. doi: 10.3390/agriculture12101745
Dixon J. L., Stringer L. C., Challinor A. J. (2014). Farming system evolution and adaptive capacity: Insights for adaptation support. Resources 3, 182–214. doi: 10.3390/resources3010182
Dolatabadian A. R. I. A., Cornelsen J., Huang S. H. U. A. N. G. L. O. N. G., Zou Z., Fernando W. D. (2022). Sustainability on the farm: breeding for resistance and management of major canola diseases in Canada contributing towards an IPM approach. Can. J. Plant Pathol. 44, 157–190. doi: 10.1080/07060661.2021.1991480
Donatelli M., Magarey R. D., Bregaglio S., Willocquet L., Whish P. M., Savary S. (2017). Modelling the impacts of pests and diseases on agricultural systems. Agric. Syst. 155, 213–224. doi: 10.1016/j.agsy.2017.01.019
Dumont T., Marodon J., Nibouche S., Hoarau J. Y., Barau L. (2023). “Genetic variability of sugarcane varieties for resistance to Sipha flava,” in Proceedings of the International Society of Sugar Cane Technologists, vol. 31. Hyderabad: ISSCT, 628–636.
Durán-Díaz P. (2023). Sustainable land governance for water–energy–food systems: A framework for rural and peri-urban revitalisation. Land 12, 1828. doi: 10.3390/land12101828
Eslami S. V., Arpanahi N. K. (2023). Water stress may decelerate purple nutsedge (Cyperus rotundus) invasion in arid environments. PREPRINT. doi: 10.21203/rs.3.rs-2828533/v1
Esquivel I. L., Faris A. M., Brewer M. J. (2021). Sugarcane aphid, Melanaphis sacchari (Hemiptera: Aphididae), abundance on sorghum and johnsongrass in a laboratory and field setting. Crop Prot. 148, 105715. doi: 10.1016/j.cropro.2021.105715
Evers G. W., Burson B. L. (2004). Dallisgrass and other Paspalum species. Warm-season (C4) grasses. Agron. Monogr. 45, 681–713. doi: 10.2134/agronmonogr45.c20
Faris A. M., Elliott N. C., Brewer M. J. (2022). Suppression of the sugarcane aphid, Melanaphis sacchari (Hemiptera: Aphididae), by resident natural enemies on susceptible and resistant sorghum hybrids. Environ. Entomology 51, 332–339. doi: 10.1093/ee/nvab147
Fassnacht F. E., White J. C., Wulder M. A., Næsset E. (2024). Remote sensing in forestry: Current challenges, considerations and directions. Forestry: Int. J. For. Res. 97, 11–37. doi: 10.1093/forestry/cpad024
Finch D. M., Butler J. L., Runyon J. B., Fettig C. J., Kilkenny F. F., Jose S., et al. (2021). “Effects of climate change on invasive species,” in Poland T. M., Patel-Weynand T., Finch D. M., Miniat C. F., Hayes D. C., Lopez V. M. eds. Invasive Species in Forests and Rangelands of the United States. Cham: Springer, 57–83. doi: 10.1007/978–3-030–45367-1_4
Fogliata S. V., Vera A., Gastaminza G., Cuenya M. I., Zucchi M. I., Willink E., et al. (2016). Reproductive isolation between two populations of Diatraea saccharalis (F.) (Lepidoptera: Crambidae) from different host plant species and regions in Argentina. Bull. Entomological Res. 106, 591–597. doi: 10.1017/S0007485316000249
Fresneda-Quintana C., Martínez-Rodríguez A., Laffita-Leyva A., Zamora-Díaz O. (2024). Multispectral image processing to assess sugarcane nitrogen needs. Rev. Cienc. Técnicas Agropecuarias 33 (1), e08.
Gaertner M., Wilson J. R., Cadotte M. W., MacIvor J. S., Zenni R. D., Richardson D. M. (2017). Non-native species in urban environments: patterns, processes, impacts and challenges. Biol. Invasions 19, 3461–3469. doi: 10.1007/s10530-017-1598-7
Gautam S., Hens L. (2022). Omikron: where do we go in a sustainability context? Environ. Dev. Sustainability 24 (4), 4491–4492. doi: 10.1007/s10668-022-02207-8
Geng B. A. I., Yufeng G. E. (2023). Crop stress sensing and plant phenotyping systems: A review. Smart Agric. 5, 66. doi: 10.12133/j.smartag.SA202211001
Ghirardello G. A., Araujo L. D. S., Silva G. S. D., Silva A. F. M., Campos L. H. F. D., Victoria Filho R. (2022). Efficacy of the herbicides indaziflam and clomazone on problematic weeds of family Poaceae to sugarcane crop. Bioscience J. 38, 1981–3163. doi: 10.14393/BJ-v38n0a2022-56358
Giliba R. A., Mpinga I. H., Ndimuligo S. A., Mpanda M. M. (2020). Changing climate patterns risk the spread of Varroa destructor infestation of African honey bees in Tanzania. Ecol. Processes 9, 1–11. doi: 10.1186/s13717-020-00247-4
Giraldeli A. L., Silva A. F. M., Baccin L. C., da Silva Araújo L., da Silva G. S., Pagenotto A. C. V., et al. (2021). Chemical management of Cyperus rotundus L. and other weeds at sugarcane in PSS system. Bioscience J. 37, 1981–3163. doi: 10.14393/BJ-v37n0a2021-51527
Girolamo-Neto C. D., Sanches I. D. A., Neves A. K., Prudente V. H. R., Körting T. S., Picoli M. C. A., et al. (2019). Assessment of texture features for Bermudagrass (Cynodon dactylon) detection in sugarcane plantations. Drones 3, 36. doi: 10.3390/drones3020036
Gobu R., Shiv A., Kumar C. A., Basavaraj P. S., Harish D., Adhikari S., et al. (2020). “Accelerated crop breeding towards development of climate resilient varieties,” in Climate change and Indian Agriculture: Challenges and Adaptation Strategies, eds. Rao C.S., Srinivas T., Rao R. V. S., Rao N. S., Vinayagam S. S., Krishnan P., et al (Hyderabad: ICAR-National Academy of Agricultural Research Management), 1–22.
Gonçalves M. C., Pinto L. R., Pimenta R. J. G., da Silva M. F. (2024). “Sugarcane,” in Viral Diseases of Field and Horticultural Crops (Amsterdam, The Netherlands: Academic Press), 193–205. doi: 10.1016/B978–0-323–90899-3.00056–2
Govindaraju M., Li Y., Zhang M. (2019). Emerging bacterial disease (leaf scald) of sugarcane in China: pathogenesis, diagnosis, and management. Plant diseases-current threats Manage. trends. doi: 10.5772/intechopen.88333
Grandis A., Fortirer J. S., Navarro B. V., de Oliveira L. P., Buckeridge M. S. (2024). Biotechnologies to improve sugarcane productivity in a climate change scenario. Bioenergy Res. 17, 1–26. doi: 10.1007/s12155-023-10649-9
Grigorieva E., Livenets A., Stelmakh E. (2023). Adaptation of agriculture to climate change: A scoping review. Climate 11, 202. doi: 10.3390/cli11100202
Gyamfi E. K., ElSayed Z., Kropczynski J., Yakubu M. A., Elsayed N. (2024). Agricultural 4.0 leveraging on technological solutions: study for smart farming sector. (United States: University of Cincinnati Ohio). doi: 10.48550/arXiv.2401.00814
Hamdi A., Shaban K., Erradi A., Mohamed A., Rumi S. K., Salim F. D. (2022). Spatiotemporal data mining: A survey on challenges and open problems. Artif. Intell. Rev. 55, 1441–1488. doi: 10.1007/s10462-021-09994-y
Haring S. C. (2022). Adapting Integrated Pest Management for Weeds in California Orchards (Davis: University of California).
Harvey J. A., Tougeron K., Gols R., Heinen R., Abarca M., Abram P. K., et al. (2023). Scientists’ warning on climate change and insects. Ecol. Monogr. 93, e1553. doi: 10.1002/ecm.1553
Hauser N., Conlon K. C., Desai A., Kobziar L. N. (2021). Climate change and infections on the move in North America. Infection Drug Resistance 14, 5711–5723. doi: 10.2147/IDR.S305077
He Y. A. N., Shuang C. H. E. N., Zhao J. K., Zhang Z. B., Chen L. L., Huang R. M., et al. (2024). Dynamic changes in weed abundance and biodiversity following different green manure establishment. J. Integr. Agric. doi: 10.1016/j.jia.2024.01.007
Heeb L., Jenner E., Cock M. J. (2019). Climate-smart pest management: building resilience of farms and landscapes to changing pest threats. J. Pest Sci. 92, 951–969. doi: 10.1007/s10340-019-01083-y
Hendrichs J., Vreysen M. J. B., Enkerlin W. R., Cayol J. P. (2021). “Strategic options in using sterile insects for area-wide integrated pest management,” in Sterile insect technique (Vienna, Austria: CRC Press), 841–884.
Hill J. K., Griffiths H. M., Thomas C. D. (2011). Climate change and evolutionary adaptations at species’ range margins. Annu. Rev. Entomology 56, 143–159. doi: 10.1146/annurev-ento-120709-144746
Hossain M. I., Ahmad K., Siddiqui Y., Saad N., Rahman Z., Haruna A. O., et al. (2020). Current and prospective strategies on detecting and managing Colletotrichum falcatum causing red rot of sugarcane. Agronomy 10, 1253. doi: 10.3390/agronomy10091253
Hoy J. W., Hollier C. A. (2009). Effect of brown rust on yield of sugarcane in Louisiana. Plant Dis. 93, 1171–1174. doi: 10.1094/PDIS-93-11-1171
Hume J. B., Almeida P. R., Buckley C. M., Criger L. A., Madenjian C. P., Robinson K. F., et al. (2021). Managing native and non-native sea lamprey (Petromyzon marinus) through anthropogenic change: A prospective assessment of key threats and uncertainties. J. Great Lakes Res. 47, S704–S722. doi: 10.1016/j.jglr.2020.08.015
Hussain T., Khan F. A., Sikander R. A. (2024). Assessment of mycotic diseases attack on indigenous old mango plants grown in Vatala park, Tehsil Bernala District Bhimber, Azad Kashmir. Natural Resour. Conserv. Res. 6, 2985. doi: 10.24294/nrcr.v6i2.2985
Janousek W. M., Douglas M. R., Cannings S., Clément M. A., Delphia C. M., Everett J. G., et al. (2023). Recent and future declines of a historically widespread pollinator linked to climate, land cover, and pesticides. Proc. Natl. Acad. Sci. 120, e2211223120. doi: 10.1073/pnas.2211223120
January B., Yusufu A., Urassa F. (2020). First report of yellow sugarcane aphid Sipha flava Forbes (Homoptera: Aphididae) in Tanzania. J. Plant Prot. Res. 60, 320–322. doi: 10.24425/jppr.2020.133316
Jogi Q., Hajano G. A., Kandharo M. N., Shah A. N., Soomro A. A., Abbasi Z. A., et al. (2019). 17. Examine different weed management techniques in sugarcane (Saccharum officinarum L.). Pure Appl. Biol. (PAB) 8, 151–159. doi: 10.19045/bspab.2018.700173
Johnson N. (2023). Eco-Evolutionary Ramifications of Herbicide-Driven Plant Modifications on Plant-Herbivore Interactions in Modern Agricultural Landscapes. Doctoral dissertation. (University of Michigan: United States). doi: 10.7302/8349
Johnson R. M., Orgeron A. J., Spaunhorst D. J., Huang I.-S., Zimba P. V. (2023). Discrimination of weeds from sugarcane in Louisiana using hyperspectral leaf reflectance data and pigment analysis. Weed Technol. 37, 123–131. doi: 10.1017/wet.2023.14
Jorgenson A. K., Fiske S., Hubacek K., Li J., McGovern T., Rick T., et al. (2019). Social science perspectives on drivers of and responses to global climate change. Wiley Interdiscip. Reviews: Climate Change 10, e554. doi: 10.1002/wcc.554
Joyce A. L., White W. H., Nuessly G. S., Solis M. A., Scheffer S. J., Lewis M. L., et al. (2014). Geographic population structure of the sugarcane borer, Diatraea saccharalis (F.) (Lepidoptera: Crambidae), in the Southern United States. PloS One 9, e110036. doi: 10.1371/journal.pone.0110036
Kachare N. V., Khan A. I., Suryawanshi M. V. (2024). Impact of climate change on sugarcane production and its mitigation a review. Int. J. Prog. Res. Eng. Manag. Sci. 03 (12), 389–396. doi: 10.58257/IJPREMS32389
Kadam R. L., Ahire R. D., Tadavi F. R., Behera R. K. (2023). Constraints & suggestions perceived by the sugarcane growers towards sustainable cultivation practices. The Pharma Innov. J. 12 (8), 1550–1553. doi: 10.22271/tpi
Kalimba U. B., Culas R. J. (2020). “Climate change and farmers’ adaptation: Extension and capacity building of smallholder farmers in Sub-Saharan Africa,” in Global Climate Change and Environmental Policy. Eds. Venkatramanan V., Shah S., Prasad R. (Springer, Singapore). doi: 10.1007/978–981-13–9570-3_13
Kamath S. V., Attokkaran J. R., Maraddi A. S., Samage A., D’Souza G. B., Yoon H., et al. (2024). Cello-MOF filters for multifaceted emerging pollutant abatement and their value addition. Chem. Eng. J. 479, 147805. doi: 10.1016/j.cej.2023.147805
Kamga S. F., Ndjomatchoua F. T., Guimapi R. A., Klingen I., Tchawoua C., Hjelkrem G. R., et al. (2022). The effect of climate variability in the efficacy of the entomopathogenic fungus Metarhizium acridum against the desert locust Schistocerca gregaria. Sci. Rep. 12, 7535. doi: 10.1038/s41598-022-11424-0
Karounos M., Cherry R., McCray M., Ji S. (2020). Survival and behavior of melanotus communis (Coleoptera: elateridae) in Florida sugarcane soils. J. Entomological Sci. 55, 499–506. doi: 10.18474/0749-8004-55.4.499
Karounos M., Cherry R., Odero D., Sandhu H., Beuzelin J. (2019). Host plant preference of Melanotus communis (Coleoptera: Elateridae) among weeds and sugar cane varieties found in Florida sugar cane fields. Florida Entomologist 102, 403–407. doi: 10.1653/024.102.0213
Karunathilake E. M., Le A. T., Heo S., Chung Y. S., Mansoor S. (2023). The Path to Smart Farming: Innovations and opportunities in precision agriculture. Agriculture 13, 1593. doi: 10.3390/agriculture13081593
Keerthi Sree Y., Lakra N., Manorama K., Ahlawat Y., Zaid A., Elansary H., et al. (2023). Drought-induced morpho-physiological, biochemical, metabolite responses and protein profiling of chickpea (Cicer arietinum l.). Agronomy 13 (7). doi: 10.3390/agronomy13071814
Khan M. A., Akhtar M. S. (2015). “Agricultural adaptation and climate change policy for crop production in Africa,” in Crop Production and Global Environmental Issues. Ed. Hakeem K. (Springer, Cham). doi: 10.1007/978–3-319–23162-4_18
Khatri P., Kumar P., Shakya K. S., Kirlas M. C., Tiwari K. K. (2023). “Understanding the intertwined nature of rising multiple risks in modern agriculture and food system,” in Environment, Development and Sustainability. Springer Nature B.V, 1–44. doi: 10.1007/s10668-023-03638-7
Khatri-Chhetri A., Pant A., Aggarwal P. K., Vasireddy V. V., Yadav A. (2019). Stakeholders prioritization of climate-smart agriculture interventions: Evaluation of a framework. Agric. Syst. 174, 23–31. doi: 10.1016/j.agsy.2019.03.002
Khattak W. A., Sun J., Hameed R., Zaman F., Abbas A., Khan K. A., et al. (2024). Unveiling the resistance of native weed communities: insights for managing invasive weed species in disturbed environments. Biol. Rev. 99 (3), 753–777. doi: 10.1111/brv.13043
Kim Y. O., Johnson J. D., Lee E. J. (2005). Phytotoxicity of Phytolacca americana leaf extracts on the growth, and physiological response of Cassia mimosoides. J. Chem. Ecol. 31, 2963–2974. doi: 10.1007/s10886-005-8407-y
Kim Y. U., Webber H., Adiku S. G., Júnior R. D. S. N., Deswarte J. C., Asseng S., et al. (2024). Mechanisms and modelling approaches for excessive rainfall stress on cereals: Waterlogging, submergence, lodging, pests and diseases. Agric. For. Meteorology 344, 109819. doi: 10.1016/j.agrformet.2023.109819
Knutson T. R., Zhang R., Horowitz L. W. (2016). Prospects for a prolonged slowdown in global warming in the early 21st century. Nat. Commun. 7 (1), 1–12. doi: 10.1038/ncomms13676
Koralewski T. E., Wang H., Grant W. E., Brewer M. J., Elliott N. C. (2022). Evaluation of areawide forecasts of wind-borne crop pests: sugarcane aphid (Hemiptera: aphididae) infestations of sorghum in the great plains of North America. J. Economic Entomology 115, 863–868. doi: 10.1093/jee/toac035
Koshariya A. K., Sharma N., Satapathy S. N., Thilagam P., Laxman T., Rai S. (2023). Safeguarding Agriculture: A comprehensive review of plant protection strategies. Int. J. Environ. Climate Change 13, 272–281. doi: 10.9734/ijecc/2023/v13i113168
Kousta A., Katsis C., Tsekoura A., Chachalis D. (2024). Effectiveness and selectivity of pre-and post-emergence herbicides for weed control in grain legumes. Plants 13, 211. doi: 10.3390/plants13020211
Kowalska H., Masiarz E., Ignaczak A., Marzec A., Hać-Szymańczuk E., Salamon A., et al. (2023). Advances in multigrain snack bar technology and consumer expectations: A review. Food Rev. Int. 39 (1), 93–118. doi: 10.1080/87559129.2022.2094402
Kumar S., Prasad C. S. (2020). Biology and isolation of bio-agents from sugarcane white grub in western plain zone of Uttar Pradesh, India. J. Pharmacognosy Phytochem. 9, 2798–2801.
Lantero E., Matallanas B., Callejas C. (2023). Current status of the main olive pests: useful integrated pest management strategies and genetic tools. Appl. Sci. 13, 12078. doi: 10.3390/app132112078
Lee B. W., Oeller L. C., Crowder D. W. (2022). Integrating community ecology into models of vector-borne virus transmission. Plants 12, 2335. doi: 10.3390/plants12122335
Lee S., Yun C. M. (2023). A deep learning model for predicting risks of crop pests and diseases from sequential environmental data. Plant Methods 19, 145. doi: 10.1186/s13007-023-01122-x
Legros M., Marshall J. M., Macfadyen S., Hayes K. R., Sheppard A., Barrett L. G. (2021). Gene drive strategies of pest control in agricultural systems: Challenges and opportunities. Evolutionary Appl. 14, 2162–2178. doi: 10.1111/eva.13285
Li A. (2024). Technological innovation in disease detection and management in sugarcane planting. Bioscience Methods 15 (1), 58–65. doi: 10.5376/bm.2024.15.0007
Li A., Wang M., Chen Z., Qin C., Liao F., Wu Z., et al. (2021). Integrated Transcriptome and Metabolome Analysis to Identify Sugarcane Gene Defense against Fall Armyworm (Spodoptera frugiperda) Herbivory. Int. J. Mol. Sci. 23, 13712. doi: 10.3390/ijms232213712
Li J., Zhang Z., Lei Q., Lu B., Jin C., Liu X., et al. (2022). Multiple herbicide resistance in Eleusine indica from sugarcane fields in China. Pesticide Biochem. Physiol. 182, 105040. doi: 10.1016/j.pestbp.2022.105040
Li H., Peng Y., Wu C., Li Z., Zou L., Mao K., et al. (2024). Assessing genome-wide adaptations associated with range expansion in the pink rice borer, Sesamia inferens. Insect Sci. doi: 10.1111/1744-7917.13320
Li P., Liang C., Jiao J., Ruan Z., Sun M., Fu X., et al. (2024). Exogenous priming of chitosan induces resistance in Chinese prickly ash against stem canker caused by Fusarium zanthoxyli. Int. J. Biol. Macromolecules, 129119. doi: 10.1016/j.ijbiomac.2023.129119
Linnenluecke M. K., Nucifora N., Thompson N. (2018). Implications of climate change for the sugarcane industry. Wiley Interdiscip. Reviews: Climate Change 9, e498. doi: 10.1002/wcc.498
Linnenluecke M. K., Zhou C., Smith T., Thompson N., Nucifora N. (2020). The impact of climate change on the Australian sugarcane industry. J. Cleaner Production 246, 118974. doi: 10.1016/j.jclepro.2019.118974
Lopes M. A. (2023). Rethinking plant breeding and seed systems. Available online at: http://www.alice.cnptia.embrapa.br/alice/handle/doc/1158393.
Lovelli S., Perniola M., Ferrara A., Amato M., Di Tommaso T. (2010). Photosynthetic response to water stress of pigweed (Amaranthus retroflexus) in a Southern-Mediterranean area. Weed Sci. 58, 126–131. doi: 10.1614/WS-09-039.1
Lu G., Wang Z., Xu F., Pan Y., Grisham M. P., Xu L. (2021). Sugarcane mosaic disease: characteristics, identification and control. Microorganisms 9, 1984. doi: 10.3390/microorganisms9091984
Maduka C. P., Adegoke A. A., Okongwu C. C., Enahoro A., Osunlaja O., Ajogwu A. E. (2023). Review of laboratory diagnostics evolution in Nigeria’s response to COVID-19. Int. Med. Sci. Res. J. 3, 1–23. doi: 10.51594/imsrj.v3i1.581
Mahesh P., Srikanth J., Salin K., Singaravelu B., Chandran K., Mahendran B. (2019). Phenology of sugarcane leaf hopper Pyrilla perpusilla (Walker) (Homoptera: Lophopidae) and its natural enemies in a crop island scenario. Crop Prot. 120, 151–162. doi: 10.1016/j.cropro.2019.02.020
Mahgoub A. M. (2023). Ecological importance and sociological characters of fourteen widespread weeds and their contribution to the observed (dis) similarity between weed communities. Heliyon 9 (4), e13638. doi: 10.1016/j.heliyon.2023.e13638
Majhi P. K., Samal I., Bhoi T. K., Pattnaik P., Pradhan C., Gupta A., et al. (2023). “Climate-smart agriculture: an integrated approach to address climate change and food security,” in Climate Change and Insect Biodiversity (United States: CRC Press), 221–245. doi: 10.1201/9781003382089–14
Makgoba M. C., Tshikhudo P. P., Nnzeru L. R., Makhado R. A. (2021). Impact of fall armyworm (Spodoptera frugiperda) (J.E. Smith) on small-scale maize farmers and its control strategies in the Limpopo province, South Africa. Jàmbá: J. Disaster Risk Stud. 13 (1), 1016. doi: 10.4102/jamba.v13i1.1016
Mandal A., Sarkar B., Mandal S., Vithanage M., Patra A. K., Manna M. C. (2019). “Impact of agrochemicals on soil health,” in Agrochemicals detection, treatment and remediation. Butterworth-Heinemann, 161–187. doi: 10.1016/B978–0-08–103017–2.00007–6
Matthieson L. (2007). Climate change and the Australian Sugarcane Industry: impacts, adaptation and R&D opportunities. SRDC Technical Report (Brisbane, Australia: Sugar Research and Development).
Maxwell S. L., Butt N., Maron M., McAlpine C. A., Chapman S., Ullmann A., et al. (2019). Conservation implications of ecological responses to extreme weather and climate events. Diversity Distributions 25, 613–625. doi: 10.1111/ddi.12878
Mercer N. H. (2020). Management of sugarcane aphid (Melanaphis sacchari) using biological and cultural tactics in Kentucky. Theses and Dissertations—Entomology. (University of Kentucky: United States), 51. doi: 10.13023/etd.2020.094
Mishra H., Mishra D. (2023). Artificial intelligence and machine learning in agriculture: Transforming farming systems. Res. Trends Agric. Sci. 1, 1–16.
Montalvo-Navarrete J. M., Lasso-Palacios A. P. (2024). Energy access sustainability criteria definition for Colombian rural areas. Renewable Sustain. Energy Rev. 189, 113922. doi: 10.1016/j.rser.2023.113922
Morya G. P., Kumar R. (2023). Sustainable pest management-A review in the Indian context. Curr. Res. Agric. 4 (1), 1–7. doi: 10.18782/2582–7146.169
Mrabet R. (2023). “Sustainable agriculture for food and nutritional security,” in Sustainable Agriculture and the Environment (Amsterdam, The Netherlands: Academic Press), 25–90. doi: 10.1016/B978-0-323-90500-8.00013-0
Msomba B. H., Ndaki P. M., Nassary E. K. (2021). Assessing impact of climate change and extreme weather events on the sustainability of sugarcane production. J. Global Agric. Ecol. 11 (4), 33–45.
Muhammad M., Ahmad M. W., Basit A., Ullah S., Mohamed H. I., Nisar N., et al. (2024). “Plant growth-promoting rhizobacteria and their applications and role in the management of soilborne diseases,” in Bacterial Secondary Metabolites (Amsterdam, The Netherlands: Elsevier), 59–82. doi: 10.1016/B978-0-323-95251-4.00001-6
Muhammad F., Sarwar J., Amin F., Safi M. H., Khurshid A., Shah M., et al. (2023). Effect of granular insecticides against sugarcane stem borer (Chilo infuscatellus) and sugarcane root borer (Emalocera depresella) at district Mardan. Pure Appl. Biol. 12, 1194–1200. doi: 10.19045/bspab.2023.120122
Mulianga B., Bégué A., Simoes M., Todoroff P. (2013). Forecasting regional sugarcane yield based on time integral and spatial aggregation of MODIS NDVI. Remote Sens. 5, 2184–2199. doi: 10.3390/rs5052184
Mwangi R. W., Mustafa M., Charles K., Wagara I. W., Kappel N. (2023). Selected emerging and reemerging plant pathogens affecting the food basket: A threat to food security. J. Agric. Food Res. 14, 100827. doi: 10.1016/j.jafr.2023.100827
Mylonas I., Stavrakoudis D., Katsantonis D., Korpetis E. (2020). “Better farming practices to combat climate change,” in Climate change and food security with emphasis on wheat (Amsterdam, The Netherlands: Academic Press), 1–29. doi: 10.1016/B978-0-12-819527-7.00001-7
Naqvi R. Z., Siddiqui H. A., Mahmood M. A., Najeebullah S., Ehsan A., Azhar M., et al. (2022). Smart breeding approaches in post-genomics era for developing climate-resilient food crops. Front. Plant Sci. 13. doi: 10.3389/fpls.2022.972164
Narayana T. L., Venkatesh C., Kiran A., Kumar A., Khan S. B., Almusharraf A., et al. (2024). Advances in real time smart monitoring of environmental parameters using IoT and sensors. Heliyon 10, e28195. doi: 10.1016/j.heliyon.2024.e28195
Nath C. P., Singh R. G., Choudhary V. K., Datta D., Nandan R., Singh S. S. (2024). Challenges and alternatives of herbicide-based weed management. Agronomy 14, 126. doi: 10.3390/agronomy14010126
Ncoyini Z., Savage M. J., Strydom S. (2022). Limited access and use of climate information by small-scale sugarcane farmers in South Africa: A case study. Climate Serv. 26, 100285. doi: 10.1016/j.cliser.2022.100285
Ngcobo S., Hill T., Jewitt G., Archer E. (2023). A yield gap analysis to assess vulnerability of commercial sugarcane to climatic extremes in southern Africa. J. Agric. Food Res. 14, 100734. doi: 10.1016/j.jafr.2023.100734
Nguru W., Mwongera C. (2023). Predicting the future climate-related prevalence and distribution of crop pests and diseases affecting major food crops in Zambia. PloS Climate 2, e0000064. doi: 10.1371/journal.pclm.0000064
Nikpay A., Tiwari A. K., Vejar-Cota G., Ziaee M., Wilson B., Srivastava S., et al. (2023). “Biotic stresses in sugarcane plants and its management,” in Agro-industrial perspectives on sugarcane production under environmental stress (Springer Nature Singapore, Singapore), 301–359. doi: 10.1007/978–981-19–3955-6_15
Noureen A., Aziz R., Ismail A., Trzcinski A. P. (2022). The impact of climate change on waterborne diseases in Pakistan. Sustainability Climate Change 15, 138–152. doi: 10.1089/scc.2021.0070
Nunes P. S., Junior G. V. L., Mascarin G. M., Guimarães R. A., Medeiros F. H., Arthurs S., et al. (2024). Microbial consortium of biological products: do they have a future? Biol. Control 188, 105439. doi: 10.1016/j.biocontrol.2024.105439
Odero D. C., Rice R., Baucum L. (2013). Biology and control of goosegrass in sugarcane: SSAGR367/SC096, 1/2013. EDIS 2013. doi: 10.32473/edis-sc096-2013
Othman S. H., Lazim Z. S., Al-Wakaa A. H. A. (2023). “Allelopathic potential of aqueous plant extracts against seed germination and seedling growth of weeds,” in IOP Conference Series: Earth and Environmental Science. IOP Publishing Ltd, England and Wales, vol. 1262, 032041. doi: 10.1088/1755-1315/1262/3/032041
Pachiappan K., Anitha K., Pitchai R., Sangeetha S., Satyanarayana T. V. V., Boopathi S. (2024). “Intelligent machines, ioT, and AI in revolutionizing agriculture for water processing,” in Handbook of Research on AI and ML for Intelligent Machines and Systems. Pennsylvania, USA, 374–399. doi: 10.4018/978–1-6684–9999-3.ch015
Page M. J., McKenzie J. E., Bossuyt P. M., Boutron I., Hoffmann T. C., Mulrow C. D., et al. (2021). The PRISMA 2020 statement: an updated guideline for reporting systematic reviews. BMJ 372, n71. doi: 10.1136/bmj.n71
Palanivel H., Shah S. (2021). Unlocking the inherent potential of plant genetic resources: food security and climate adaptation strategy in Fiji and the Pacific. Environment Dev. Sustainability 23, 14264–14323. doi: 10.1007/s10668-021-01273-8
Parthasarathy S., Lakshmidevi P., Satya V. K., Gopalakrishnan C. (2024). Plant Pathology and Disease Management: Principles and Practices (United States: CRC Press). doi: 10.1201/9781032711973
Parveen S., Rashtrapal P. S. (2024). Integrated pest management strategies using endophytic entomopathogenic fungi. Plant Sci. Today 11 (1), 568–574. doi: 10.14719/pst.2740
Pavinato C. V. A., Michel A. P., Omoto C., Zucchi M. I. (2017). Influence of historical land use and modern agricultural expansion on the spatial and ecological divergence of sugarcane borer, Diatraea saccharalis (Lepidoptera: Crambidae) in Brazil. Heredity 120, 25–37. doi: 10.1038/s41437-017-0018-1
Pekarcik A. J., Jacobson A. L. (2021). Evaluating sugarcane aphid, Melanaphis sacchari (Hemiptera: Aphididae), population dynamics, feeding injury, and grain yield among commercial sorghum varieties in Alabama. J. Economic Entomology 114, 757–768. doi: 10.1093/jee/toab013
Peng B., Guan K., Tang J., Ainsworth E. A., Asseng S., Bernacchi C. J., et al. (2020). Towards a multiscale crop modelling framework for climate change adaptation assessment. Nat. Plants 6, 338–348. doi: 10.1038/s41477-020-0625-3
Petropoulos F., Apiletti D., Assimakopoulos V., Babai M. Z., Barrow D. K., Ben Taieb S., et al. (2022). Forecasting: theory and practice. Int. J. Forecasting 38, 705–871. doi: 10.1016/j.ijforecast.2021.11.001
Pires da Silva B., Oliveira de Almeida R., Salgado T. P., Pedro Luis da C. A. A. (2014). Efficacy of imazapic, halosulfuron and sulfentrazone for Cyperus rotundus L. control in response to weed tuber density. Afr. J. Agric. Res. 9, 3458–3464. doi: 10.5897/AJAR2013.8411
Prado G. S., Rocha D. C., Santos L. N., Contiliani D. F., Nobile P. M., Camargo J., et al. (2024). CRISPR technology towards genome editing of the perennial and semi-perennial crops citrus, coffee and sugarcane. Front. Plant Sci. 14, 1331258. doi: 10.3389/fpls.2023.1331258
Preti M., Verheggen F., Angeli S. (2021). Insect pest monitoring with camera-equipped traps: Strengths and limitations. J. Pest Sci. 94, 203–217. doi: 10.1007/s10340-020-01309-4
Qin Z. Q., Luo Y. W., Li D. W., Song X. P., Wei J. J., Wei C. Y., et al. (2020). Field evaluation of infestation levels of the sugarcane mealybug Saccharicoccus sacchari (Cockerell) on different sugarcane varieties in Guangxi Province, China: Preliminary results. Intl. Sugar J. 122 (1461), 648–653.
Raid R. N., Comstock J. C., Sood S. (2013). Sugarcane orange rust in Florida: its history, current status, and management. J. Am. Soc. Sugar Cane Technol. 33, 57.
Rajput M. A., Rajput N. A., Syed R. N., Lodhi A. M., Que Y. (2021). Sugarcane smut: Current knowledge and the way forward for management. J. Fungi 7, 1095. doi: 10.3390/jof7121095
Ram B., Karuppaiyan R., Hemaprabha G. (2022). “Sugarcane Breeding,” in Fundamentals of Field Crop Breeding (Springer Nature Singapore, Singapore), 499–570. doi: 10.1007/978–981-16–9257-4_9
Ramanujam B., Hosamani A., Poornesha B., Sowmya E. (2021). Biological control of white grubs, Holotrichia serrata (Fabricius) in sugarcane by two species of entomopathogenic fungi, Metarhizium anisopliae and Beauveria bassiana. Int. J. Trop. Insect Sci. 41, 671–680. doi: 10.1007/s42690-020-00256-y
Ranganathan K., Krishnan M., Kuppusamy A., Azrag A. G., Jandhyala S., Arjunan N. (2023). Spatio-temporal distribution of sugarcane shoot borer, Chilo infuscatellus (Lepidoptera: Crambidae) and its associated natural enemy Sturmiopsis inferens (Diptera: Tachinidae) in India. Int. J. Trop. Insect Sci. 43, 277–287. doi: 10.1007/s42690-022-00940-1
Rao A. Q., Bajwa K. S., Ali M. A., Bakhsh A., Iqbal A., Latif A., et al. (2023). Evaluation of weed control efficiency of herbicide resistant transgenic cotton. J. Anim. Plant Sci. 33 (1), 75–84. doi: 10.36899/JAPS.2023.1.0596
Reddy A. V., Thiruvengatanadhan R., Srinivas M., Dhanalakshmi P. (2024). Region based segmentation with enhanced adaptive histogram equalization model with definite feature set for sugarcane leaf disease classification. Int. J. Intelligent Syst. Appl. Eng. 12, 428–442.
Rice J. L., Hoy J. W., Grisham M. P. (2019). Sugarcane mosaic distribution, incidence, increase, and spatial pattern in Louisiana. Plant Dis. 103, 2051–2056. doi: 10.1094/PDIS-01-19-0099-RE
Richard B., Qi A., Fitt B. D. (2022). Control of crop diseases through Integrated Crop Management to deliver climate-smart farming systems for low-and high-input crop production. Plant Pathol. 71, 187–206. doi: 10.1111/ppa.13493
Rocklöv J., Semenza J. C., Dasgupta S., Robinson E. J., Abd El Wahed A., Alcayna T., et al. (2023). Decision-support tools to build climate resilience against emerging infectious diseases in Europe and beyond. Lancet Regional Health–Europe 32, 100701. doi: 10.1016/j.lanepe.2023.100701
Röling N. (2019). “The agricultural research-technology transfer interface: a knowledge systems perspective,” in Making the link (United States: CRC Press), 1–42.
Roy D., Ghosh S., Datta D., Sreekanth D., Pawar D., Mukherjee P. K., et al. (2023). “Climate resilient weed management for crop production,” in Climate-Resilient Agriculture, Vol 2: Agro-Biotechnological Advancement for Crop Production (Springer International Publishing, Cham), 125–156. doi: 10.1007/978–3-031–37428-9_7
Saha N., Mazumdar P. C., Basak J., Raha A., Majumder A., Chandra K. (2016). Subterranian termite genus Odontotermes (Blattaria: Isoptera: Termitidae) from Chhattisgarh, India with its annotated checklist and revised key. J. Threatened Taxa 8, 8602–8610. doi: 10.11609/jott.2016.8.3.8541-8640
Sakdapolrak P., Sterly H., Borderon M., Bunchuay-Peth S., Naruchaikusol S., Ober K., et al. (2024). Translocal social resilience dimensions of migration as adaptation to environmental change. Proc. Natl. Acad. Sci. 121, e2206185120. doi: 10.1073/pnas.2206185120
Santosh S., Pavithran M. (2024). Iron-based smart alloys for critical applications: A review on processing, properties, phase transformations, and current trends. J. Mater. Sci.: Mater. Engin 19 (1), 1–17. doi: 10.1186/s40712-024-00150-2
Sarjan M., Muchlis M., Muthahanas I. (2021). The diversity of major insect pests at sugarcane development center in Dompu District, West Nusa Tenggara. J. Sci. Sci. Educ. 2, 38–46. doi: 10.29303/jossed.v2i1.712
Sarkar M., Gorai P., Modak B. K. (2024). “Indigenous strategies and adaptive approaches to scrabble recent climate crisis in two districts (Bankura and purulia) of West Bengal, India,” in Climate Crisis: Adaptive Approaches and Sustainability (Springer Nature Switzerland, Cham), 75–101. doi: 10.1007/978–3-031–44397-8_5
Savary S., Willocquet L. (2020). Modeling the impact of crop diseases on global food security. Annu. Rev. Phytopathol. 58, 313–341. doi: 10.1146/annurev-phyto-010820-012856
Scavo A., Mauromicale G. (2020). Integrated weed management in herbaceous field crops. Agronomy 10, 466. doi: 10.3390/agronomy10040466
Schrader S., Graham S., Campbell R., Height K., Hawkes G. (2024). Grower attitudes and practices toward area-wide management of cropping weeds in Australia. Land Use Policy 137, 107001. doi: 10.1016/j.landusepol.2023.107001
Shafiq S., Haq M. Z. U., Shahbaz A., Shafique S., Riaz M., Hanif M. T., et al. (2024). “Agrochemicals and climate change,” in Water-Soil-Plant-Animal Nexus in the Era of Climate Change. Pennsylvania, USA, 49–77. doi: 10.4018/978–1-6684–9838-5.ch003
Shahi T. B., Xu C. Y., Neupane A., Guo W. (2023). Recent advances in crop disease detection using UAV and deep learning techniques. Remote Sens. 15, 2450. doi: 10.3390/rs15092450
Shahid M. S., Akram A., Imtiaz U., Saba I., Kabir R., Hayat U., et al. (2024). Review of recent advances in chemical fertilizers and their impact on crop productivity and sustainability. Eur. Chem. Bull. 13 (1), 62–71. doi: 10.53555/ecb/2024.13.01.10
Shahzad A., Ullah S., Dar A. A., Sardar M. F., Mehmood T., Tufail M. A., et al. (2021). Nexus on climate change: Agriculture and possible solution to cope future climate change stresses. Environ. Sci. pollut. Res. 28, 14211–14232. doi: 10.1007/s11356-021-12649-8
Shaktawat P., Swaymprava S. (2024). “Digital agriculture: exploring the role of information and communication technology for sustainable development,”. In: Eds. Mallick B., Anshuman J. Navigating Agricultural Extension: A Comprehensive Guide. (PMW, New Delhi), 31.
Sharma R. P., Dharavath R., Edla D. R. (2023). IoFT-FIS: Internet of farm things based prediction for crop pest infestation using optimized fuzzy inference system. Internet Things 21, 100658. doi: 10.1016/j.iot.2022.100658
Sharma T., Shera P. S. (2021). Biological anomalies in the sugarcane leafhopper, Pyrilla perpusilla (Walker) due to parasitism by Fulgoraecia melanoleuca (Fletcher) (Lepidoptera: Epipyropidae). Egyptian J. Biol. Pest Control 31, 1–8. doi: 10.1186/s41938-021-00486-3
Shawky S., Rundle-Thiele S., David P. (2023). What enables and prevents sugarcane growing practice change? A review of publicly available sources for practice change projects. Austr. J. Environ. Manag. 30 (3–4), 327–347. doi: 10.1080/14486563.2023.2238662
Shivanna K. R. (2022). Climate change and its impact on biodiversity and human welfare. Proc. Indian Natl. Sci. Academy. Part A Phys. Sci. 88, 160–171. doi: 10.1007/s43538-022-00073-6
Shrestha R. B., Thapa Y. B. eds. (2019). Policy and program priorities in agricultural research & Development in South Asia. Bangkok, Thailand: SAARC Agriculture Center, Dhaka, Bangladesh, and Asia-Pacific Association of Agricultural Research Institutions (APAARI). p. 376.
Shumail H., Khalid S., Ghaffar N., Akhtar N., Haq S. I. U. (2022). A study of some weeds of Pakistan and their response to abiotic stress-a review. J. Pure Appl. Agric. 7 (1), 51–58.
Simões R. A., Feliciano J. R., Solter L. F., Delalibera I. (2015). Impacts of Nosema sp. (Microsporidia: Nosematidae) on the sugarcane borer, Diatraea saccharalis (Lepidoptera: Crambidae). J. Invertebrate Pathol. 129, 7–12. doi: 10.1016/j.jip.2015.05.006
Simões S., Oliveira T., Nunes C. (2022). Influence of computers in students’ academic achievement. Heliyon 8 (3), e09004. doi: 10.1016/j.heliyon.2022.e09004
Singh A., Chauhan S. S., Singh A., Singh S. B. (2006). Deterioration in sugarcane due to pokkah boeng disease. Sugar Technol. 8, 187–190. doi: 10.1007/BF02943659
Singh B. K., Delgado-Baquerizo M., Egidi E., Guirado E., Leach J. E., Liu H., et al. (2023). Climate change impacts on plant pathogens, food security and paths forward. Nat. Rev. Microbiol. 21 (10), 640–656. doi: 10.1038/s41579-023-00900-7
Singh P., Hussain T., Patel S., Akhtar N. (2018). Impact of climate change on root–pathogen interactions. In: Giri B., Prasad R., Varma A. eds. Root Biology. Soil Biology vol. 52. Springer, Cham. doi: 10.1007/978–3-319–75910-4_16
Singh P., Singh S. N., Tiwari A. K., Pathak S. K., Singh A. K., Srivastava S., et al. (2019). Integration of sugarcane production technologies for enhanced cane and sugar productivity targeting to increase farmers’ income: strategies and prospects. 3 Biotechnol. 9, 1–15. doi: 10.1007/s13205-019-1568-0
Sivakumar K., Rawat G. S., Badola R., Adhikari B. S., Kamalakannan B. (2018). Study on ecological & socio-economic impact of invasive species, Prosopis juliflora and Lantana camara, and their removal from forest, common and fallow land of Tamil Nadu. Technical Report (Dehradun, India: Wildlife Institute of India).
Skendžić S., Zovko M., Živković I. P., Lešić V., Lemić D. (2021). The impact of climate change on agricultural insect pests. Insects 12, 440. doi: 10.3390/insects12050440
Smith P., Greenwell A., Baldvins T. (2021). Noxious weed mapping and monitoring at Francis E. Warren Air Force Base 2018–2020. Colorado: United States: Colorado State University. Libraries. Doctoral dissertation.
Sorvali J., Kaseva J., Peltonen-Sainio P. (2021). Farmer views on climate change–a longitudinal study of threats, opportunities and action. Climatic Change 164, 1–19. doi: 10.1007/s10584-021-03020-4
Soumia P. S., Shirsat D. V., Chitra N., Karuppaiah V., Gadge A. S., Thangasamy A., et al. (2023). Invasion of fall armyworm, (Spodoptera frugiperda, J E Smith) (Lepidoptera, Noctuidae) on onion in the maize–onion crop sequence from Maharashtra, India. Front. Ecol. Evol. 11. doi: 10.3389/fevo.2023.1279640
Spaunhorst D. J. (2021). Spring-applied triclopyr mixtures for Bermudagrass (Cynodon dactylon) suppression in Louisiana sugarcane. Weed Technol. 35, 589–597. doi: 10.1017/wet.2021.20
Spelman R. (2024). Determining the Effect of Cover Cropping Legacy on Mycotoxin Accumulation and Fusarium Disease in Maize. Pennsylvania USA: Pennsylvania State University. https://projects.sare.org/sare_project/gne21-270/.
Sreekanth D., Pawar D. V., Mishra J. S., Naidu V. S. G. R. (2023). Climate change impacts on crop–weed interaction and herbicide efficacy. Curr. Sci. 124 (6), 686–692. doi: 10.18520/cs/v124/i6/686–692
Srinivasan G., Agarwal A., Bandara U. (2024). “Climate change impacts on water resources and agriculture in Southeast Asia with a focus on Thailand, Myanmar, and Cambodia,” in The Role of Tropics in Climate Change (Amsterdam, The Netherlands: Elsevier), 17–32. doi: 10.1016/B978-0-323-99519-1.02002-0
Srinivasa Rao M., Mani M., Prasad Y. G., Prabhakar M., Sridhar V., Vennila S., et al. (2022). Climate change and pest management strategies in horticultural and agricultural ecosystems. In: Mani M. eds. Trends in Horticultural Entomology. Springer, Singapore, 81–122. doi: 10.1007/978–981-19–0343-4_3
Storkey J., Mead A., Addy J., MacDonald A. J. (2021). Agricultural intensification and climate change have increased the threat from weeds. Global Change Biol. 27, 2416–2425. doi: 10.1111/gcb.15585
Subedi B., Poudel A., Aryal S. (2023). The impact of climate change on insect pest biology and ecology: Implications for pest management strategies, crop production, and food security. J. Agric. Food Res. 14, 100733. doi: 10.1016/j.jafr.2023.100733
Tait G., Mermer S., Stockton D., Lee J., Avosani S., Abrieux A., et al. (2021). Drosophila suzukii (Diptera: Drosophilidae): a decade of research towards a sustainable integrated pest management program. J. Economic Entomology 114, 1950–1974. doi: 10.1093/jee/toab158
Tamiru L., Fekadu H. (2019). Effects of climate change variability on agricultural productivity. Int. J. Environ. Sci. Natural Resour. 17, 555953. doi: 10.19080/IJESNR.2019.17.555953
Tan M., Ding Y., Bourdôt G. W., Qiang S. (2024). Evaluation of Bipolaris yamadae as a bioherbicidal agent against grass weeds in arable crops. Pest Manage. Sci. 80, 166–175. doi: 10.1002/ps.7630
Tan C., Kalhoro M. T., Faqir Y., Ma J., Osei M. D., Khaliq G. (2022). Climate-resilient microbial biotechnology: A perspective on sustainable agriculture. Sustainability 14, 5574. doi: 10.3390/su14095574
Taylor B., Casey J., Annamalai S., Finch E. A., Beale T., Holland W., et al. (2021). Minimizing pest and disease risks in uncertain climates: CABI initiatives developing new technologies and tools for outreaching early warning to farmers. AgriRxiv 2021, 20210271298. doi: 10.31220/agriRxiv.2021.000
Taylor B., Tonnang H. E. Z., Beale T., Holland W., Oronje M., Abdel-Rahman E. M., et al. (2023). Leveraging data, models & farming innovation to prevent, prepare for & manage pest incursions: delivering a pest risk service for low-income countries. Sci. Innov. Food Syst. Transformation 439–453. doi: 10.1007/978–3-031–15703-5_23
Tlatlaa J. S., Tryphone G. M., Nassary E. K. (2023). Unexplored agronomic, socioeconomic and policy domains for sustainable cotton production on small landholdings: A systematic review. Front. Agron. 5. doi: 10.3389/fagro.2023.1281043
Tripathi H. G., Kunin W. E., Smith H. E., Sallu S. M., Maurice S., Machera S. D., et al. (2022). Climate-smart agriculture and trade-offs with biodiversity and crop yield. Front. Sustain. Food Syst. 6. doi: 10.3389/fsufs.2022.868870
Tu Y., Wang Y., Jiang H., Ren H., Wang X., Lv W. (2024). A loop-mediated isothermal amplification assay for the rapid detection of Didymella segeticola causing tea leaf spot. J. Fungi 10, 467. doi: 10.3390/jof10070467
Usman M., Akram N., Imitaz S., Haider S. M. (2020). Sugarcane: Diseases due to pests, pest management strategies and factors influencing the production of sugarcane. Am. Sci. Res. J. Eng. Technol. Sci. (ASRJETS) 65, 126–139.
Valencia Arbeláez J. A., Giraldo A. S., Castaño Villa G. J., Vallejo Espinosa L. F., Salazar Gutierrez M. R., Vargas G. (2021). Population dynamics of sugarcane borers, Diatraea spp., under different climatic scenarios in Colombia. PloS One 16 (1), e0244694. doi: 10.1371/journal.pone.0244694
van Antwerpen R., van Heerden P. D. R., Keeping M. G., Titshall L. W., Jumman A., Tweddle P. B., et al. (2022). A review of field management practices impacting root health in sugarcane. Adv. Agron. 173, 79–162. doi: 10.1016/bs.agron.2022.02.002
van Zonneveld M., Turmel M., Hellin J. (2020). Decision-making to diversify farm systems for climate change adaptation. Front. Sustain. Food Syst. 4. doi: 10.3389/fsufs.2020.00032
Vasileiou M., Kyriakos L. S., Kleisiari C., Kleftodimos G., Vlontzos G., Belhouchette H., et al. (2024). Transforming weed management in sustainable agriculture with artificial intelligence: a systematic literature review towards weed identification and deep learning. Crop Prot. 176, 106522. doi: 10.1016/j.cropro.2023.106522
Vermeulen S. J., Dinesh D., Howden S. M., Cramer L., Thornton P. K. (2018). Transformation in practice: A review of empirical cases of transformational adaptation in agriculture under climate change. Front. Sustain. Food Syst. 2. doi: 10.3389/fsufs.2018.00065
Vinayaka, Prasad P. R. C. (2024). AI-enhanced remote sensing applications in Indian sugarcane research: A Comprehensive Review. Sugar Tech. 26, 609–628. doi: 10.1007/s12355-024-01409-w
Virtudazo E. V., Nojima H., Kakishima M. (2001). Taxonomy of Puccinia species causing rust diseases on sugarcane. Mycoscience 42, 167–175. doi: 10.1007/BF02464133
Viswanathan R. (2022). Sugarcane diseases: Diagnostics and management. RASSA J. Sci. Soc. 4, 36–44. doi: 10.5958/2583-3715.2022.00005.3
Viswanathan R., Selvakumar R., Geetha N., Balaji C. G., Annadurai A., Pazhani A. S., et al. (2022). Epidemiology of sugarcane wilt: predisposition by root borer Polyocha depresella a myth or reality. Indian Phytopathol. 75, 3–14. doi: 10.1007/s42360-021-00398-0
Volk G. M., Carver D., Irish B. M., Marek L., Frances A., Greene S., et al. (2023). Safeguarding plant genetic resources in the United States during global climate change. Crop Sci. 63, 2274–2296. doi: 10.1002/csc2.21003
Waheed A., Haxim Y., Islam W., Ahmad M., Muhammad M., Alqahtani F. M., et al. (2023). Climate change reshaping plant-fungal interaction. Environ. Res. 238, 117282. doi: 10.1016/j.envres.2023.117282
Walia S. S., Kaur T. (2024). Earthworms and Vermicomposting: Species, Procedures and Crop Application (United Kingdom: Springer Nature). doi: 10.1007/978-981-99-8953-9
Walsh J. E., Ballinger T. J., Euskirchen E. S., Hanna E., Mård J., Overland J. E., et al. (2020). Extreme weather and climate events in northern areas: A review. Earth-Science Rev. 209, 103324. doi: 10.1016/j.earscirev.2020.103324
Wang Y. H., Ma Y. L., Feng G. J., Li H. H. (2018). Abiotic factors affecting seed germination and early seedling emergence of large crabgrass (Digitaria sanguinalis). Planta Daninha 36, e018166895. doi: 10.1590/s0100-83582018360100068
Ward G. M., Kambey C. S., Faisan J. P. Jr., Tan P. L., Daumich C. C., Matoju I., et al. (2022). Ice-Ice disease: an environmentally and microbiologically driven syndrome in tropical seaweed aquaculture. Rev. Aquaculture 14, 414–439. doi: 10.1111/raq.12606
Waters E. K., Chen C. C. M., Azghadi M. R. (2024). Sugarcane health monitoring with satellite spectroscopy and machine learning: A Review. arXiv preprint arXiv:2404.16844. doi: 10.48550/arXiv.2404.16844
Way M. J., Conlong D. E., Martin L. A., McFarlane S. A., Stranack R., Keeping M. G., et al. (2015). First record of yellow sugarcane aphid, Siphaflava (Homoptera: Aphididae), in the South African sugarcane industry. Proceeding South Afr. Sugar Technol. Assoc. 87, 53–57.
Weier S. M., Bringhenti T., Anders M., Abdulai I., Foord S., Grass I., et al. (2024). “Management options for macadamia orchards with special focus on water management and ecosystem services,” in Sustainability of Southern African Ecosystems under Global Change: Science for Management and Policy Interventions (Springer International Publishing, Cham), 625–652. doi: 10.1007/978–3-031–10948-5_22
Williams L., Cherry R., Shapiro-Ilan D. (2022). Effect of host size on susceptibility of Melanotus Communis (Coleoptera: Elateridae) Wireworms to Entomopathogens. J. Nematol. 54 (1), e2022–1. doi: 10.2478/jofnem-2022-0033
Wójtowicz A., Wójtowicz M., Sigvald R., Czernecki B., Ratajkiewicz H., Łacka A., et al. (2020). Assessment of the impact of climate change on the latency period of leaf rust on triticale in Poland. Acta Agriculturae Scandinavica Section B—Soil Plant Sci. 70, 195–207. doi: 10.1080/09064710.2019.1696394
Wolff L. (2023). Regulating pests—material politics and calculation in integrated pest management. Environ. Plann. E: Nat. Space 6, 455–472. doi: 10.1177/25148486221076138
Xiao J., Chen Z., Liang T., Yang S., Tan H. (2024). Analysis of metabolome and microbiome revealed the resistance mechanisms in sugarcane cultivars with high resistance to pokkah boeng disease. Chem. Biol. Technol. Agric. 11, 1–16. doi: 10.1186/s40538-024-00570-4
Yanagi M. (2024). Climate change impacts on wheat production: Reviewing challenges and adaptation strategies. Adv. Resour. Res. 4, 89–107. doi: 10.50908/arr.4.1_89
Yang L. H., Postema E. G., Hayes T. E., Lippey M. K., MacArthur-Waltz D. J. (2021). The complexity of global change and its effects on insects. Curr. Opin. Insect Sci. 47, 90–102. doi: 10.1016/j.cois.2021.05.001
Yang Q., Wang Q. J., Hakala K. (2022). Calibrating anomalies improves forecasting of daily reference crop evapotranspiration. J. Hydrology 610, 128009. doi: 10.1016/j.jhydrol.2022.128009
Zaman M., Khan I. A., Schmidt S., Murphy R., Poulsen M. (2022). Morphometrics, distribution, and DNA barcoding: An integrative identification approach to the genus Odontotermes (Termitidae: Blattodea) of Khyber Pakhtunkhwa, Pakistan. Forests 13, 674. doi: 10.3390/f13050674
Zhao Y., Chen J., Shi Y., Fu Y., Huang T., Rott P. C., et al. (2022). Sugarcane responses to two strains of Xanthomonas albilineans differing in pathogenicity through a differential modulation of salicylic acid and reactive oxygen species. Frontier Plant Sci. 13. doi: 10.3389/fpls.2022.1087525
Keywords: agroecology, biotic stressors, climate adaptation, crop resilience, environmental impact, integrated pest management
Citation: Msomba BH, Ndaki PM and Joseph CO (2024) Sugarcane sustainability in a changing climate: a systematic review on pests, diseases, and adaptive strategies. Front. Agron. 6:1423233. doi: 10.3389/fagro.2024.1423233
Received: 25 April 2024; Accepted: 23 July 2024;
Published: 12 August 2024.
Edited by:
Nasim Ahmad Yasin, University of the Punjab, PakistanReviewed by:
Muhammad Rizwan Tariq, University of the Punjab, PakistanSaber Hussain, University of the Punjab, Pakistan
Zain Mushtaq, University of the Punjab, Pakistan
Samia Faiz, University of Sargodha, Pakistan
Copyright © 2024 Msomba, Ndaki and Joseph. This is an open-access article distributed under the terms of the Creative Commons Attribution License (CC BY). The use, distribution or reproduction in other forums is permitted, provided the original author(s) and the copyright owner(s) are credited and that the original publication in this journal is cited, in accordance with accepted academic practice. No use, distribution or reproduction is permitted which does not comply with these terms.
*Correspondence: Bahati Hakimu Msomba, aGFraW11YmFoYXRpQGdtYWlsLmNvbQ==