- Department of Anesthesiology, McGovern Medical School, University of Texas Health Science Center at Houston, Houston, TX, United States
Chronic inflammation affects many diseases and conditions, including aging. Interferons are a part of the immune defense against viral infections. Paradoxically, various aging tissues and organs from mammalian hosts perpetually accumulate changes brought by interferon pathway activation. Herein, we connote the mechanisms behind this phenomenon and discuss its implications in age-related pathology.
Introduction
Chronic inflammation is associated with many known pathologies, ranging from cardiovascular disease to cancer. Mounting evidence now links chronic inflammation to aging, a phenomenon commonly referred to as inflammaging (Franceschi and Campisi 2014; Kennedy et al., 2014). Evolutionarily, inflammation is a cellular mechanism to clear invading pathogens or damaged tissue and coordinate repair and restoration. The protective inflammatory response is transient and tightly controlled by the coordinated actions from copious pro- and anti-inflammatory factors.
Although early studies revealed that aging mammalian hosts generally display increased systemic inflammation, the degree and nature of tissue- and organ-level inflammation remained unclear. Recently, profound insights on the core signature related to tissue aging arose from several large-scale unbiased surveys of key tissues, organs, and cells from different hosts at various intervals during their lifespan.
Interferon Activation Accompanies All Tissue Aging
Benayoun et al. collected heart, liver, olfactory bulb, and primary neural stem cells from mice at three different ages and simultaneously analyzed their epigenomes and transcriptomes (Benayoun et al., 2019). This comprehensive study identified recurrent age-related chromatin and transcriptional changes in the upregulation of immune system response pathways, particularly the interferon (IFN) response, in all mouse tissues. Intriguingly, the 16 genes upregulated with age across tissues are responsive to interferon, and the phenomenon of age-related innate immune activation is seemingly applicable to humans. Shavlakadze et al. compared liver, skeletal muscle, kidney, and the hippocampus at seven different age points of rats and, via gene expression profiling, discovered a progressive upregulation of innate immune responses, including IFN responses, in the tissues (Shavlakadze et al., 2019). Among the 11 annotated genes jointly upregulated in all four tissues, the majority are inducible by interferon. Angelidis et al. conducted concomitant transcriptome and proteome analysis and revealed a similar upregulation of immune activation, including the interferon response, focusing on cells isolated from young versus old mouse lungs (Angelidis et al., 2019). Moreover, Baruch et al. compared multiple aged tissues and identified the choroid plexus as a locus within the aging brain that expresses extraordinarily high IFN signature (Baruch et al., 2014). Despite pending confirmation in humans, these studies collectively highlight that progressive inflammation, in particular IFN response, constitutes a conserved core process related to tissue and organ aging, a term coined here as IFN-aging.
Forces Driving Age-Related Interferon Response
From these intriguing discoveries and other recent research development, a paradigm that blends the fundamental principles of molecular biology and immunology starts to emerge. The mammalian genome encodes three types of interferons–types I (primarily IFNα/β), II (IFNγ), and III (IFNλ), among which types I (IFN-I) and III IFN are induced via innate immune responses upon sensing of microbial nucleic acids by innate immune receptors, such as cGAS (Roers et al., 2016). In the absence of pathogen infection, host-derived nucleic acids may activate the IFN pathway aberrantly, a process now postulated to take place during aging.
Constituting >40% of the human and rodent genomes, transposable elements (TE), with viral reminiscence, are routinely repressed in somatic cells to prevent their mobilization (Bourque et al., 2018; Makałowski et al., 2019). The “transposon theory of aging” posits that the cellular control mechanism that keeps TEs in check declines with age and contributes to age-related tissue dysfunction (Murray 1990; De Cecco et al., 2013; Li et al., 2013; Gorbunova et al., 2014; Wood et al., 2016; Gorbunova et al., 2021). In vitro, primary cells under replicative or other stress can enter a state of growth arrest named senescence. While undergoing other cellular and molecular changes, these cells secrete proinflammatory factors, a feature termed senescence-associated secretive phenotype (SASP) (Coppé et al., 2008). At a late stage, senescent cells accumulate cytosolic DNA from activated LINE-1, a family of TEs, which activates the cGAS-STING innate signaling pathway to initiate IFN-I response as part of full SASP (Franceschi and Campisi 2014; De Cecco et al., 2019). TE derepression and cGAS-STING-IFN innate signaling is predominant in a group of diseases called laminopathy, including several conditions of accelerated aging (Graziano et al., 2018). Mechanical stress from defective nuclear lamina can disrupt the heterochromatin structure that normally silences LINE-1, which leads to its activation and subsequent immune response (Kreienkamp et al., 2018; Earle et al., 2020). Sirtuins are a class of nicotinamide adenine dinucleotide-consuming enzymes implicated in diverse biological pathways. In particular, SIRT3 and SIRT6 critically stabilize the nuclear lamina and maintain TE quiescence as the cells or animals lacking these genes undergo senescence or premature aging, respectively (Simon et al., 2019; Diao et al., 2021).
Genomic instability and epigenetic alterations are key hallmarks of aging (López-Otín et al., 2013). DNA damage affects many aspects of the aging phenotype, and genetic mutations affecting genome maintenance and repair promote the premature onset of age-related pathologies (Rieckher et al., 2021; Schumacher et al., 2021). Double-stranded DNA damage is sufficient to induce IFNβ production, which stimulates the p53 pathway and promotes senescence (Yu et al., 2015). While age-associated epigenetic alterations weaken the genome surveillance mechanism and increase TE transposition, manipulating genes known to affect heterochromatin structure mitigate the expression of TEs and extend life span (Wood et al., 2016). Not coincidentally, laminopathy often manifests with DNA damage, epigenome alteration, and loss of genomic integrity (Shumaker et al., 2006; Kreienkamp et al., 2018; Earle et al., 2020). In keeping with these mechanisms, Benayoun et al. detected TE activation in conjunction with epigenetic reprogramming in the aging tissues, a finding consistent with the profound upturn of IFN response (Benayoun et al., 2019).
Besides nuclear defects, aging cells accumulate mutations and deletions in the mitochondrial genome. The “mitochondrial theory of aging” denotes that accumulation of damage to mitochondria and mitochondria DNA (mtDNA) leads to aging of humans and animals, in line with the accelerated aging of mtDNA mutator mice (Trifunovic et al., 2004; Kujoth et al., 2005; Jang et al., 2018). mtDNA evolutionarily resembles bacterial DNA, an agonist for innate IFN response. mtDNA stress stimulates the escape of mtDNA into the cytosol, where it engages with the cGAS-STING pathway to initiate IFN response (West et al., 2015). Intriguingly, genetic ablation of the IFN-I receptor not only attenuates the hyperinflammatory phenotypes but also extends the life span of the mtDNA mutator mice (Lei et al., 2021). Altogether, these studies reveal a causal relationship between age-associated nuclear or mitochondrial dysregulation and innate IFN response (Figure 1).
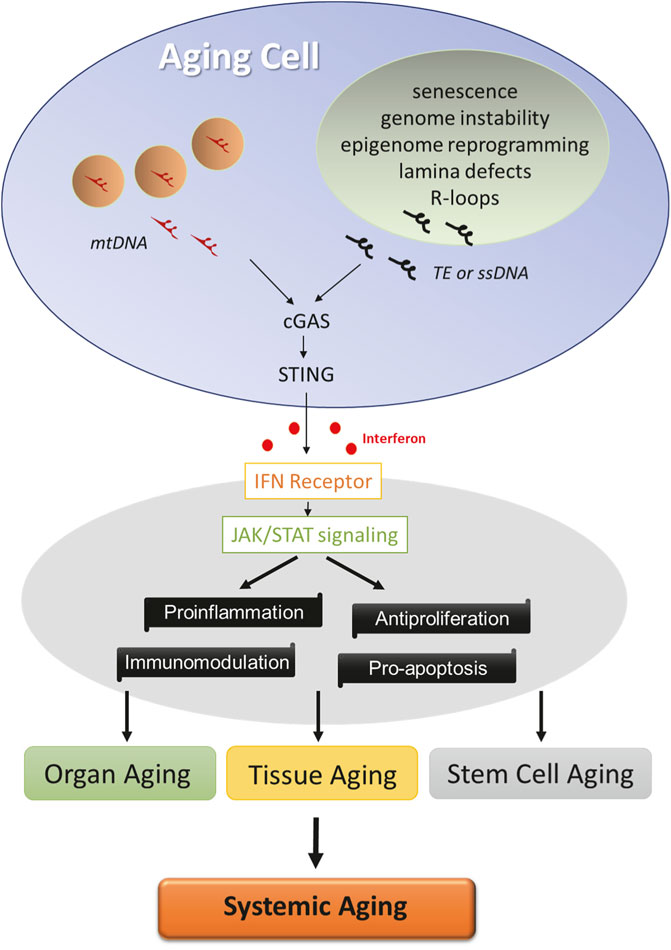
FIGURE 1. Factors driving age-related IFN response. Cells undergo multiple molecular changes in the nuclei (green) and mitochondria (pink) during aging. Cytosolic sensing of TE, ssDNA, or mitochondrial DNA by cGAS activates STING and initiates innate interferon response in aging cells. Interferon signaling via JAK/STAT pathway exerts a profound influence on cellular functions, and its activation constitutes a core program of tissue, organ, and stem cell aging, which likely contributes to the systemic aging process.
Concluding Remarks
The recent discovery of IFN-aging has important implications not only in basic research but also in translational perspectives. Nucleoside reverse-transcriptase inhibitors (NRTi) are a class of FDA-approved drugs that suppress TE by interfering with the reverse transcription step of retroelement replication (Jones et al., 2008; Dai et al., 2011). Treatment with NRTi effectively decreased TE transposition, dampened IFN response, and extended the life span of aging mice and Drosophila (Wood et al., 2016; Simon et al., 2019), consistent with the driver role of TE derepression in aging. Separately, calorie restriction extends the life span of Drosophila in conjunction with decreased TE transposition (Wood et al., 2016). One of the exciting developments of the aging field is the success of senolytic compounds in eliminating senescent cells from tissues and rejuvenating the aging host in vivo (Baker et al., 2011; Xu et al., 2018), albeit its specific impact on tissue IFN response remains to be established.
The conceptual breakthrough of the IFN-aging phenomenon undoubtedly invites studies to illuminate several key questions to shed more light on the core aging process:
1) What are the cell types in each tissue or organ most vulnerable to IFN activation? Are they all senescent cells? Besides stromal and other cell types, tissue macrophages can accumulate age-dependent DNA damage and polarize towards a proinflammatory state (Horn and Triantafyllopoulou 2018; Guimarães et al., 2021). With technical improvement, omic approaches should help generate a refined tissue aging map at a single-cell level in the future (He et al., 2020).
2) In addition to TEs and mtDNA, aberrant R-loop structures containing RNA/DNA hybrids are linked to chronic inflammation and human diseases (Richard and Manley 2017). Interestingly, R-loops in aged pancreatic cells trigger the release of cytoplasmic single-stranded DNA (ssDNA), leading to IFN activation in a STING-dependent manner (Chatzidoukaki et al., 2021). How central is the cGAS-STING axis in tissue versus organismic aging? Do other nucleic acid-sensing immune pathways participate in IFN-aging?
3) With aging, white adipose tissue cells are highly susceptible to DNA damage and senescence (Smith et al., 2021). These adipocytes elicit a chronic autoinflammatory response, which is significantly amplified by obesity-driven IFN activation (Karakasilioti et al., 2013; Chan et al., 2020). How does IFN contribute to the aging of specific tissue? Is there any functional divergence among the IFN subtypes? This is an important point to clarify as IFN can exert proinflammatory, antiproliferative, proapoptotic, and immunomodulatory functions in a contact-dependent manner (Ng et al., 2016; Burke and Young 2019).
4) Aging of the immune system impacts the morbidity and mortality of the elderly. DNA damage in bone marrow stem cells can induce IFNβ production, which leads to immune stem cell senescence and decline (Yu et al., 2015). Strikingly, the senescent immune cells are sufficient to trigger systemic aging, as non-lymphoid organs also show increased senescence and damage in mice, despite restricted DNA damage in hematopoietic cells (Yousefzadeh et al., 2021). How important is IFN in organismic aging is yet to be fully elucidated.
Answers to all these questions would greatly facilitate the eventual development of targeted therapeutics to modify age-related processes and pathologies.
Author Contributions
WC formulated the concepts, wrote the manuscript, and approved the submitted version.
Funding
The study was funded by grants of NIH AG057587, AG074283, BrightFocus ADR, and Brown Foundation 2020 Healthy Aging Initiative.
Conflict of Interest
WC received honorarium as an invited speaker in a symposium sponsored by AstraZeneca.
Publisher’s Note
All claims expressed in this article are solely those of the authors and do not necessarily represent those of their affiliated organizations, or those of the publisher, the editors and the reviewers. Any product that may be evaluated in this article, or claim that may be made by its manufacturer, is not guaranteed or endorsed by the publisher.
References
Angelidis, I., Simon, L. M., Fernandez, I. E., Strunz, M., Mayr, C. H., Greiffo, F. R., et al. (2019). An Atlas of the Aging Lung Mapped by Single Cell Transcriptomics and Deep Tissue Proteomics. Nat. Commun. 10, 963. doi:10.1038/s41467-019-08831-9
Baker, D. J., Wijshake, T., Tchkonia, T., LeBrasseur, N. K., Childs, B. G., van de Sluis, B., et al. (2011). Clearance of p16Ink4a-Positive Senescent Cells Delays Ageing-Associated Disorders. Nature 479, 232–236. doi:10.1038/nature10600
Baruch, K., Deczkowska, A., David, E., Castellano, J. M., Miller, O., Kertser, A., et al. (2014). Aging-induced Type I Interferon Response at the Choroid Plexus Negatively Affects Brain Function. Science 346, 89–93. doi:10.1126/science.1252945
Benayoun, B. A., Pollina, E. A., Singh, P. P., Mahmoudi, S., Harel, I., Casey, K. M., et al. (2019). Remodeling of Epigenome and Transcriptome Landscapes with Aging in Mice Reveals Widespread Induction of Inflammatory Responses. Genome Res. 29, 697–709. doi:10.1101/gr.240093.118
Bourque, G., Burns, K. H., Gehring, M., Gorbunova, V., Seluanov, A., Hammell, M., et al. (2018). Ten Things You Should Know about Transposable Elements. Genome Biol. 19, 199. doi:10.1186/s13059-018-1577-z
Burke, J. D., and Young, H. A. (2019). IFN-γ: A Cytokine at the Right Time, Is in the Right Place. Semin. Immunol. 43, 101280. doi:10.1016/j.smim.2019.05.002
Chan, C. C., Damen, M. S. M. A., Moreno-Fernandez, M. E., Stankiewicz, T. E., Cappelletti, M., Alarcon, P. C., et al. (2020). Type I Interferon Sensing Unlocks Dormant Adipocyte Inflammatory Potential. Nat. Commun. 11, 2745. doi:10.1038/s41467-020-16571-4
Chatzidoukaki, O., Stratigi, K., Goulielmaki, E., Niotis, G., Akalestou-Clocher, A., Gkirtzimanaki, K., et al. (2021). R-loops Trigger the Release of Cytoplasmic ssDNAs Leading to Chronic Inflammation upon DNA Damage. Sci. Adv. 7, eabj5769. doi:10.1126/sciadv.abj5769
Coppé, J. P., Patil, C. K., Rodier, F., Sun, Y., Muñoz, D. P., Goldstein, J., et al. (2008). Senescence-associated Secretory Phenotypes Reveal Cell-Nonautonomous Functions of Oncogenic RAS and the P53 Tumor Suppressor. Plos Biol. 6, 2853–2868. doi:10.1371/journal.pbio.0060301
Dai, L., Huang, Q., and Boeke, J. D. (2011). Effect of Reverse Transcriptase Inhibitors on LINE-1 and Ty1 Reverse Transcriptase Activities and on LINE-1 Retrotransposition. BMC Biochem. 12, 18. doi:10.1186/1471-2091-12-18
De Cecco, M., Criscione, S. W., Peterson, A. L., Neretti, N., Sedivy, J. M., and Kreiling, J. A. (2013). Transposable Elements Become Active and mobile in the Genomes of Aging Mammalian Somatic Tissues. Aging 5, 867–883. doi:10.18632/aging.100621
De Cecco, M., Ito, T., Petrashen, A. P., Elias, A. E., Skvir, N. J., Criscione, S. W., et al. (2019). L1 Drives IFN in Senescent Cells and Promotes Age-Associated Inflammation. Nature 566, 73–78. doi:10.1038/s41586-018-0784-9
Diao, Z., Ji, Q., Wu, Z., Zhang, W., Cai, Y., Wang, Z., et al. (2021). SIRT3 Consolidates Heterochromatin and Counteracts Senescence. Nucleic Acids Res. 49, 4203–4219. doi:10.1093/nar/gkab161
Earle, A. J., Kirby, T. J., Fedorchak, G. R., Isermann, P., Patel, J., Iruvanti, S., et al. (2020). Mutant Lamins Cause Nuclear Envelope Rupture and DNA Damage in Skeletal Muscle Cells. Nat. Mater. 19, 464–473. doi:10.1038/s41563-019-0563-5
Franceschi, C., and Campisi, J. (2014). Chronic Inflammation (Inflammaging) and its Potential Contribution to Age-Associated Diseases. J. Gerontol. A. Biol. Sci. Med. Sci. 69 (Suppl. 1), S4–S9. doi:10.1093/gerona/glu057
Gorbunova, V., Boeke, J. D., Helfand, S. L., and Sedivy, J. M. (2014). Sleeping Dogs of the Genome. Science 346, 1187–1188. doi:10.1126/science.aaa3177
Gorbunova, V., Seluanov, A., Mita, P., McKerrow, W., Fenyö, D., Boeke, J. D., et al. (2021). The Role of Retrotransposable Elements in Ageing and Age-Associated Diseases. Nature 596, 43–53. doi:10.1038/s41586-021-03542-y
Graziano, S., Kreienkamp, R., Coll-Bonfill, N., and Gonzalo, S. (2018). Causes and Consequences of Genomic Instability in Laminopathies: Replication Stress and Interferon Response. Nucleus 9, 258–275. doi:10.1080/19491034.2018.1454168
Guimarães, G. R., Almeida, P. P., de Oliveira Santos, L., Rodrigues, L. P., de Carvalho, J. L., and Boroni, M. (2021). Hallmarks of Aging in Macrophages: Consequences to Skin Inflammaging. Cells 10, 1323. doi:10.3390/cells10061323
He, X., Memczak, S., Qu, J., Belmonte, J. C. I., and Liu, G.-H. (2020). Single-cell Omics in Ageing: a Young and Growing Field. Nat. Metab. 2, 293–302. doi:10.1038/s42255-020-0196-7
Horn, V., and Triantafyllopoulou, A. (2018). DNA Damage Signaling and Polyploid Macrophages in Chronic Inflammation. Curr. Opin. Immunol. 50, 55–63. doi:10.1016/j.coi.2017.11.002
Jang, J. Y., Blum, A., Liu, J., and Finkel, T. (2018). The Role of Mitochondria in Aging. J. Clin. Invest. 128, 3662–3670. doi:10.1172/jci120842
Jones, R. B., Garrison, K. E., Wong, J. C., Duan, E. H., Nixon, D. F., and Ostrowski, M. A. (2008). Nucleoside Analogue Reverse Transcriptase Inhibitors Differentially Inhibit Human LINE-1 Retrotransposition. PLoS One 3, e1547. doi:10.1371/journal.pone.0001547
Karakasilioti, I., Kamileri, I., Chatzinikolaou, G., Kosteas, T., Vergadi, E., Robinson, A. R., et al. (2013). DNA Damage Triggers a Chronic Autoinflammatory Response, Leading to Fat Depletion in NER Progeria. Cel Metab. 18, 403–415. doi:10.1016/j.cmet.2013.08.011
Kennedy, B. K., Berger, S. L., Brunet, A., Campisi, J., Cuervo, A. M., Epel, E. S., et al. (2014). Geroscience: Linking Aging to Chronic Disease. Cell 159, 709–713. doi:10.1016/j.cell.2014.10.039
Kreienkamp, R., Graziano, S., Coll-Bonfill, N., Bedia-Diaz, G., Cybulla, E., Vindigni, A., et al. (2018). A Cell-Intrinsic Interferon-like Response Links Replication Stress to Cellular Aging Caused by Progerin. Cel Rep. 22, 2006–2015. doi:10.1016/j.celrep.2018.01.090
Kujoth, G. C., Hiona, A., Pugh, T. D., Someya, S., Panzer, K., Wohlgemuth, S. E., et al. (2005). Mitochondrial DNA Mutations, Oxidative Stress, and Apoptosis in Mammalian Aging. Science 309, 481–484. doi:10.1126/science.1112125
Lei, Y., Guerra Martinez, C., Torres-Odio, S., Bell, S. L., Birdwell, C. E., Bryant, J. D., et al. (2021). Elevated Type I Interferon Responses Potentiate Metabolic Dysfunction, Inflammation, and Accelerated Aging in mtDNA Mutator Mice. Sci. Adv. 7, eabe7548. doi:10.1126/sciadv.abe7548
Li, W., Prazak, L., Chatterjee, N., Grüninger, S., Krug, L., Theodorou, D., et al. (2013). Activation of Transposable Elements during Aging and Neuronal Decline in Drosophila. Nat. Neurosci. 16, 529–531. doi:10.1038/nn.3368
López-Otín, C., Blasco, M. A., Partridge, L., Serrano, M., and Kroemer, G. (2013). The Hallmarks of Aging. Cell 153, 1194–1217. doi:10.1016/j.cell.2013.05.039
Makałowski, W., Gotea, V., Pande, A., and Makałowska, I. (2019). “Transposable Elements: Classification, Identification, and Their Use as a Tool for Comparative Genomics,” in Evolutionary Genomics: Statistical and Computational Methods. Editor M. Anisimova (New York, NYNew York: Springer), 177–207.
Murray, V. (1990). Are Transposons a Cause of Ageing? Mutat. Research/DNAging 237, 59–63. doi:10.1016/0921-8734(90)90011-f
Ng, C. T., Mendoza, J. L., Garcia, K. C., and Oldstone, M. B. A. (2016). Alpha and Beta Type 1 Interferon Signaling: Passage for Diverse Biologic Outcomes. Cell 164, 349–352. doi:10.1016/j.cell.2015.12.027
Richard, P., and Manley, J. L. (2017). R Loops and Links to Human Disease. J. Mol. Biol. 429, 3168–3180. doi:10.1016/j.jmb.2016.08.031
Rieckher, M., Garinis, G. A., and Schumacher, B. (2021). Molecular Pathology of Rare Progeroid Diseases. Trends Mol. Med. 27, 907–922. doi:10.1016/j.molmed.2021.06.011
Roers, A., Hiller, B., and Hornung, V. (2016). Recognition of Endogenous Nucleic Acids by the Innate Immune System. Immunity 44, 739–754. doi:10.1016/j.immuni.2016.04.002
Schumacher, B., Pothof, J., Vijg, J., and Hoeijmakers, J. H. J. (2021). The central Role of DNA Damage in the Ageing Process. Nature 592, 695–703. doi:10.1038/s41586-021-03307-7
Shavlakadze, T., Morris, M., Fang, J., Wang, S. X., Zhu, J., Zhou, W., et al. (2019). Age-Related Gene Expression Signature in Rats Demonstrate Early, Late, and Linear Transcriptional Changes from Multiple Tissues. Cel Rep. 28, 3263–3273. e3. doi:10.1016/j.celrep.2019.08.043
Shumaker, D. K., Dechat, T., Kohlmaier, A., Adam, S. A., Bozovsky, M. R., Erdos, M. R., et al. (2006). Mutant Nuclear Lamin A Leads to Progressive Alterations of Epigenetic Control in Premature Aging. Proc. Natl. Acad. Sci. U.S.A. 103, 8703–8708. doi:10.1073/pnas.0602569103
Simon, M., Van Meter, M., Ablaeva, J., Ke, Z., Gonzalez, R. S., Taguchi, T., et al. (2019). LINE1 Derepression in Aged Wild-type and SIRT6-Deficient Mice Drives Inflammation. Cel Metab. 29, 871–885. e5. doi:10.1016/j.cmet.2019.02.014
Smith, U., Li, Q., Rydén, M., and Spalding, K. L. (2021). Cellular Senescence and its Role in white Adipose Tissue. Int. J. Obes. 45, 934–943. doi:10.1038/s41366-021-00757-x
Trifunovic, A., Wredenberg, A., Falkenberg, M., Spelbrink, J. N., Rovio, A. T., Bruder, C. E., et al. (2004). Premature Ageing in Mice Expressing Defective Mitochondrial DNA Polymerase. Nature 429, 417–423. doi:10.1038/nature02517
West, A. P., Khoury-Hanold, W., Staron, M., Tal, M. C., Pineda, C. M., Lang, S. M., et al. (2015). Mitochondrial DNA Stress Primes the Antiviral Innate Immune Response. Nature 520, 553–557. doi:10.1038/nature14156
Wood, J. G., Jones, B. C., Jiang, N., Chang, C., Hosier, S., Wickremesinghe, P., et al. (2016). Chromatin-modifying Genetic Interventions Suppress Age-Associated Transposable Element Activation and Extend Life Span in Drosophila. Proc. Natl. Acad. Sci. U.S.A. 113, 11277–11282. doi:10.1073/pnas.1604621113
Xu, M., Pirtskhalava, T., Farr, J. N., Weigand, B. M., Palmer, A. K., Weivoda, M. M., et al. (2018). Senolytics Improve Physical Function and Increase Lifespan in Old Age. Nat. Med. 24, 1246–1256. doi:10.1038/s41591-018-0092-9
Yousefzadeh, M. J., Flores, R. R., Zhu, Y., Schmiechen, Z. C., Brooks, R. W., Trussoni, C. E., et al. (2021). An Aged Immune System Drives Senescence and Ageing of Solid Organs. Nature 594, 100–105. doi:10.1038/s41586-021-03547-7
Keywords: aging, interferon, senescence, inflammaging, transposable elements, laminopathy, mitochondria
Citation: Cao W (2022) IFN-Aging: Coupling Aging With Interferon Response. Front. Aging 3:870489. doi: 10.3389/fragi.2022.870489
Received: 06 February 2022; Accepted: 08 March 2022;
Published: 02 May 2022.
Edited by:
Niharika Arora Duggal, University of Birmingham, United KingdomReviewed by:
George A. Garinis, University of Crete, GreeceCopyright © 2022 Cao. This is an open-access article distributed under the terms of the Creative Commons Attribution License (CC BY). The use, distribution or reproduction in other forums is permitted, provided the original author(s) and the copyright owner(s) are credited and that the original publication in this journal is cited, in accordance with accepted academic practice. No use, distribution or reproduction is permitted which does not comply with these terms.
*Correspondence: Wei Cao, d2VpLmNhb0B1dGgudG1jLmVkdQ==