- 1Department of Epidemiology and Health Statistics, School of Public Health, Guangdong Medical University, Dongguan, China
- 2Department of Neurology, Second Hospital Affiliated of Xinjiang Medical University, Xinjiang, China
- 3Maternal and Child Research Institute, Shunde Women and Children’s Hospital, Guangdong Medical University, Foshan, China
- 4Precision Laboratory, School of Public Health, Guangdong Medical University, Dongguan, China
Background: Linear associations between circulating insulin-like growth factor-1 (IGF-1) levels and Parkinson’s disease (PD) have been evidenced in observational studies. Yet, the causal relationship between IGF-1 levels and PD remains obscure. We conducted Mendelian randomization to examine the correlation between genetically predicted IGF-1 levels and PD.
Methods: By reviewing genome-wide association studies (GWAS) that are publicly accessible, we uncovered SNPs linked to both serum concentrations of IGF-1 and PD. A two-sample Mendelian randomization (MR) analysis was carried out to evaluate the individual effect of IGF-1 on PD.
Results: In a primary causal effects model in MR analysis, employing the inverse-variance weighted (IVW) method, IGF-1 levels exhibited a notable association with the risk of PD (OR, 1.020, 95% CI, 1.003–1.038, p = 0.0215). Multiple evaluations revealed that horizontal pleiotropy was improbable to distort the main results (MR-Egger: P PD intercept =0.719), and no bias was detected by leave-one-out analysis.
Conclusion: This study unearthed evidence indicating that heightened IGF-1 levels might be causally correlated with an increased risk of PD.
1 Introduction
Research into human aging has intensified, and the investigations into brain health have become more in-depth. Parkinson’s disease (PD) is predominantly an age-associated condition. Although PD presents in a minor percentage of younger adults, the majority of patients (over 75%) are over the age of 65 (Pringsheim et al., 2014; Deuschl et al., 2020). PD is a neurodegenerative disease attributed to progressive neuronal loss in the substantia nigra pars compacta, with typical clinical features including tremor, rigidity, bradykinesia/akinesia, and postural instability (Scorza et al., 2021). Moreover, PD, inflicting a considerable societal and economic burden worldwide, serves as a principal cause of disability and mortality among the elderly (Heemels, 2016; Berson et al., 2018). In clinical practice, there are no established curative methods for PD, and age stands as the sole universally accepted risk factor (Dorsey et al., 2007; Kalia and Lang, 2015; Lee and Gilbert, 2016; Scorza et al., 2018; Balestrino and Schapira, 2020).
In recent years, circulating insulin-like growth factor-1 (IGF-1), a neurotrophic hormone integral to systemic development, has gained increasing recognition for its pivotal role in brain health, influencing both central nervous system plasticity and the development of all major neural cells (O’Kusky and Ye, 2012; Dyer et al., 2016).
In a recent investigation, a linkage between IGF-1 and PD risk has been unveiled (Cao et al., 2023). It explored and fully characterized the dose–response correlation between IGF-1 and PD risk within a sizable cohort of the general populace. Nonetheless, owing to the propensity for observational inquiries to incur confounding biases, such as the potential impact of diverse underlying maladies on the varying levels of IGF-1, novel methodologies are imperative for scrutinizing causal relationships.
Mendelian randomization (MR) is a technique that is used to infer causality by employing one or more genetic variants that influence a risk factor. These genetic tools help discern the impact of the risk factor on the disease (Davey Smith and Hemani, 2014). We used an MR design to explore the causal relationship between IGF-1 and PD risk.
2 Materials and methods
2.1 Study design
MR analysis, harnessing genetic allocation variation, remains impervious to the influence of confounding factors, thereby serving as a surrogate for risk factors in instrumental variable analyses. Genetic variation is deemed effective only when closely correlated with relevant risk factors and influences outcomes solely through exposure, rather than directly affecting results (Smith and Ebrahim, 2003). Considering the susceptibility of observational studies to the impact of reverse causality and undiscovered confounding factors, this study employed a two-sample MR analysis to explore the causal relationship between circulating IGF-1 levels and PD. The SNP data for IGF-1 and PD were sourced from the Genome-Wide Association Studies (GWAS) database (Figure 1).
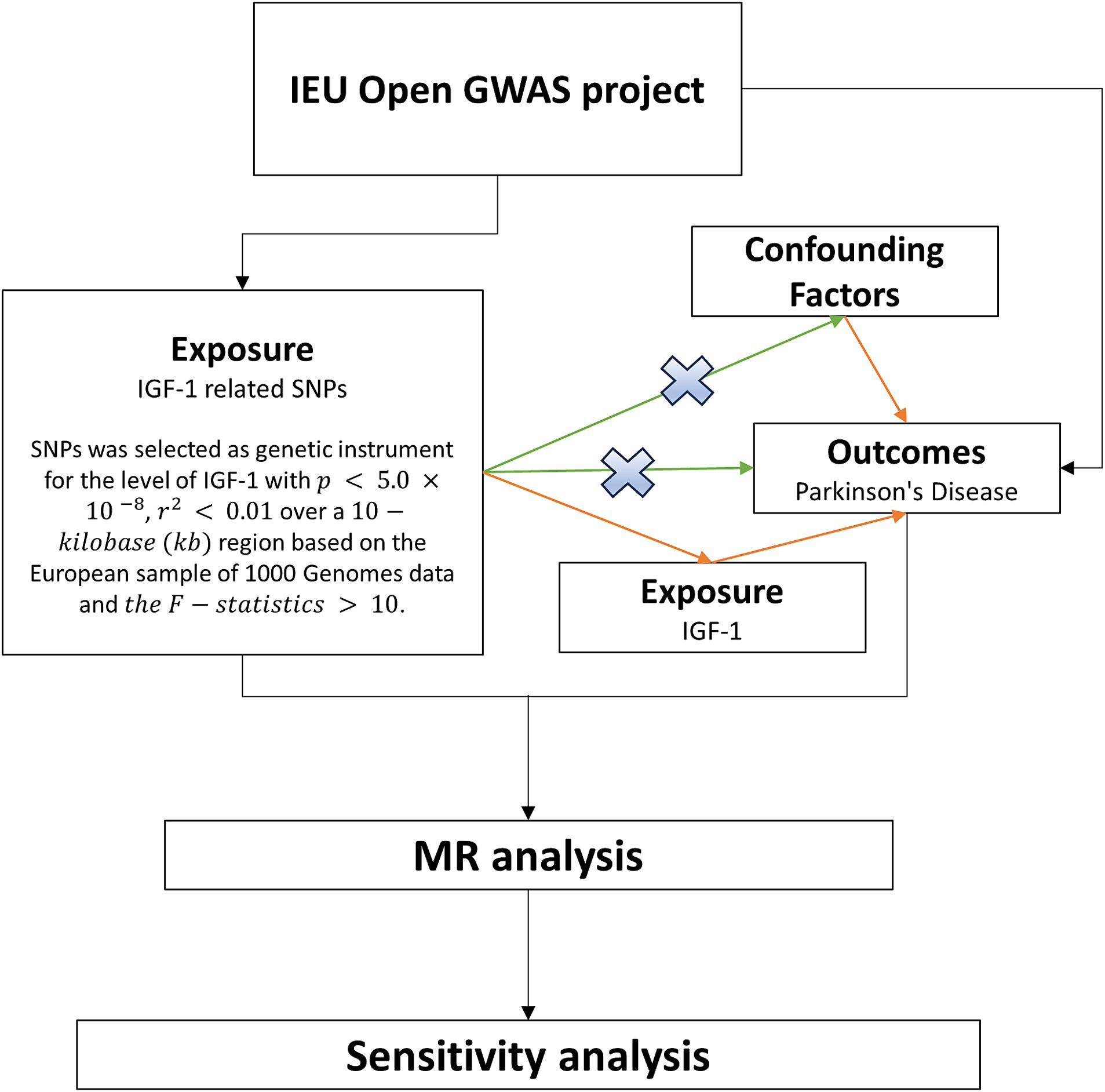
Figure 1. Flowchart of MR in this study. The study data were from the IEU Open GWAS project, where SNPs for exposure and outcome were first extracted, and instrumental variables were screened based on the SNPs for exposure. The causal effect was further explored using two-sample Mendelian randomization, and sensitivity analyses were used to test the stability of the results. MR, Mendelian randomization; SNP, Single Nucleotide Polymorphisms; IEU, Integrative Epidemiology Unit; GWAS, Genome-Wide Association Studies; IGF-1, Insulin-like growth factor-1.
2.2 Genetic instrument selection
The data for IGF-1 were obtained from 358,072 European-descent participants in the UK Biobank, with a sample size of 342,439 for IGF-1. We satisfied the conditions for SNPs to serve as effective instrumental variables (IVs): (i) strong correlation with exposure ( ); (ii) SNP independence ensured by over a 10-kilobase (kb) region based on European samples from the 1,000 Genomes data; (iii) avoidance of pathways where SNPs could directly influence outcomes (horizontal pleiotropy). Weak IV bias was mitigated using an F-statistic >10 (Huang et al., 2021). As a result, 574 SNPs were selected from 13,586,000 available SNPs as IVs for circulating IGF-1 levels. Data were downloaded from Integrative Epidemiology Unit (IEU) GWAS database (see Table 1).
2.3 Data origin of PD
Summary-level data for PD (GWAS ID: ieu-b-7) were sourced from the International Parkinson’s Disease Genomics Consortium, comprising 33,674 cases among a sample size of 482,730. The aforementioned data can be retrieved through the OpenGWAS project (mrcieu.ac.uk) and are summarized in Table 1.
2.4 Statistical analysis
In models devoid of horizontal pleiotropy, IVW yields results of maximal efficiency, rendering it as the reference in this study employing a random-effects model (Burgess et al., 2016; Burgess and Thompson, 2017; Davies et al., 2019). Several other approaches were utilized to ascertain the consistency of results. These include the simple median method, which provides reliable estimates even in the presence of at least 50% non-effective IVs; the weighted median method, which, building upon this premise, accounts for differences in estimation accuracy; and the maximum likelihood method, characterized by its smaller standard errors, used to obtain parameters of the probability density function for the database (Milligan, 2003; Burgess et al., 2017).
Furthermore, to ascertain potential impacts on MR outcomes and eliminate violations of MR assumptions due to heterogeneity and pleiotropy within the utilized IVs, a comprehensive assessment was conducted. This included heterogeneity, pleiotropy, and sensitivity, corresponding to Egger regression combined with MR-PRESSO global tests, Cochran’s Q statistic, and single SNP analysis combined with leave-one-out sensitivity analysis, respectively. Egger regression analysis and MR-PRESSO global tests were deemed to evaluate potential directional pleiotropy (Verbanck et al., 2018; Ong and MacGregor, 2019). If directional pleiotropy was detected with a p-value <0.05 based on the intercept from MR-Egger, MR-PRESSO was utilized for secondary assessment, followed by outlier removal and subsequent recalculation of causal effects. Cochran’s Q statistic from IVW and MR-Egger was utilized to examine the magnitude of heterogeneity (Storey and Tibshirani, 2003). MR-PRESSO was also utilized to test for it. Single SNP analysis and leave-one-out sensitivity analysis were utilized to determine if individual SNPs were sufficient to impact overall results. In leave-one-out plots, the harmonious distribution of all lines around 0 indicated that results were unaffected by which SNP was removed.
When p < 0.05 with two-tailed testing, the results are considered significant. All primary outcomes were obtained using the “TwoSampleMR” package within the R (version 4.1.2) environment.
3 Results
On the basis of a primary causal effects model with MR analyses through the IVW method, IGF-1 was significantly associated with the risk of PD (OR, 1.020; 95% CI, 1.003–1.038, p = 0.0215, Figure 2). The maximum likelihood results were consistent with IVW (OR, 1.020, 95% CI, 1.006–1.035, p = 0.0047). The directions of MR-Egger, weighted median, and simple median results were exactly in line with the estimates of IVW revealing significant robustness of the main analysis.
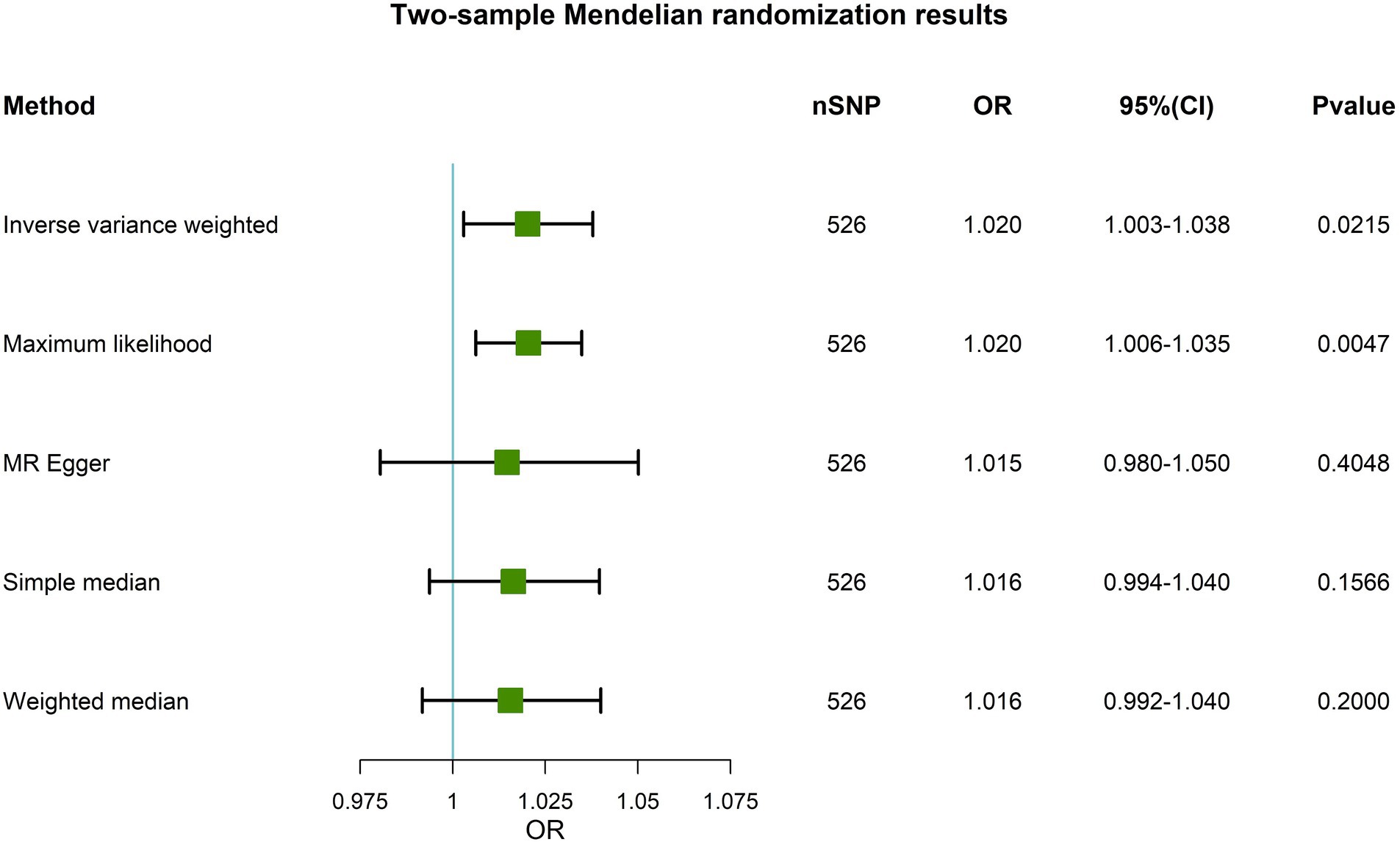
Figure 2. A forest plot showing the association of genetically predicted circulating IGF-1 with PD. Five Mendelian randomization methods are used. nSNP is the number of instrumental variables selected for MR. OR is the PD risk ratio corresponding to each unit increase in circulating IGF-1 levels. When the OR (95% CI) is greater than 1, it means that the PD risk increases. On the contrary, the risk of PD is reduced. A p value less than 0.05 indicates statistical significance between circulating IGF-1 levels and PD risk. MR, Mendelian randomization; OR, Odds Ratio; CI, Confidence Interval; SNP, Single Nucleotide Polymorphisms; PD, Parkinson’s disease; IGF-1, Insulin-like growth factor-1.
There might be heterogeneity ( , Table 2). Regardless, the IVW estimate remained unbiased, affirming the conclusion’s reliability and acceptability. In addition, despite some contradiction noted in the horizontal pleiotropy assessment between MR results (p = 0.719) and MR-PRESSO, the uniformity in results (OR, 1.020; 95% CI, 1.004–1.036, p = 0.0140, Table 3) persisted across outlier-corrected analysis in MR-PRESSO.
The leave-one-out analysis guarantees the resilience, as no individual SNP exhibits a significant impact (Supplementary Figure S1). Furthermore, the symmetry observed in the funnel plot (Figure 3) indicates the satisfactory fulfillment of the INSIDE assumption (Bowden et al., 2015). A scatter plot is presented in Figure 4, which shows consistency in the results.
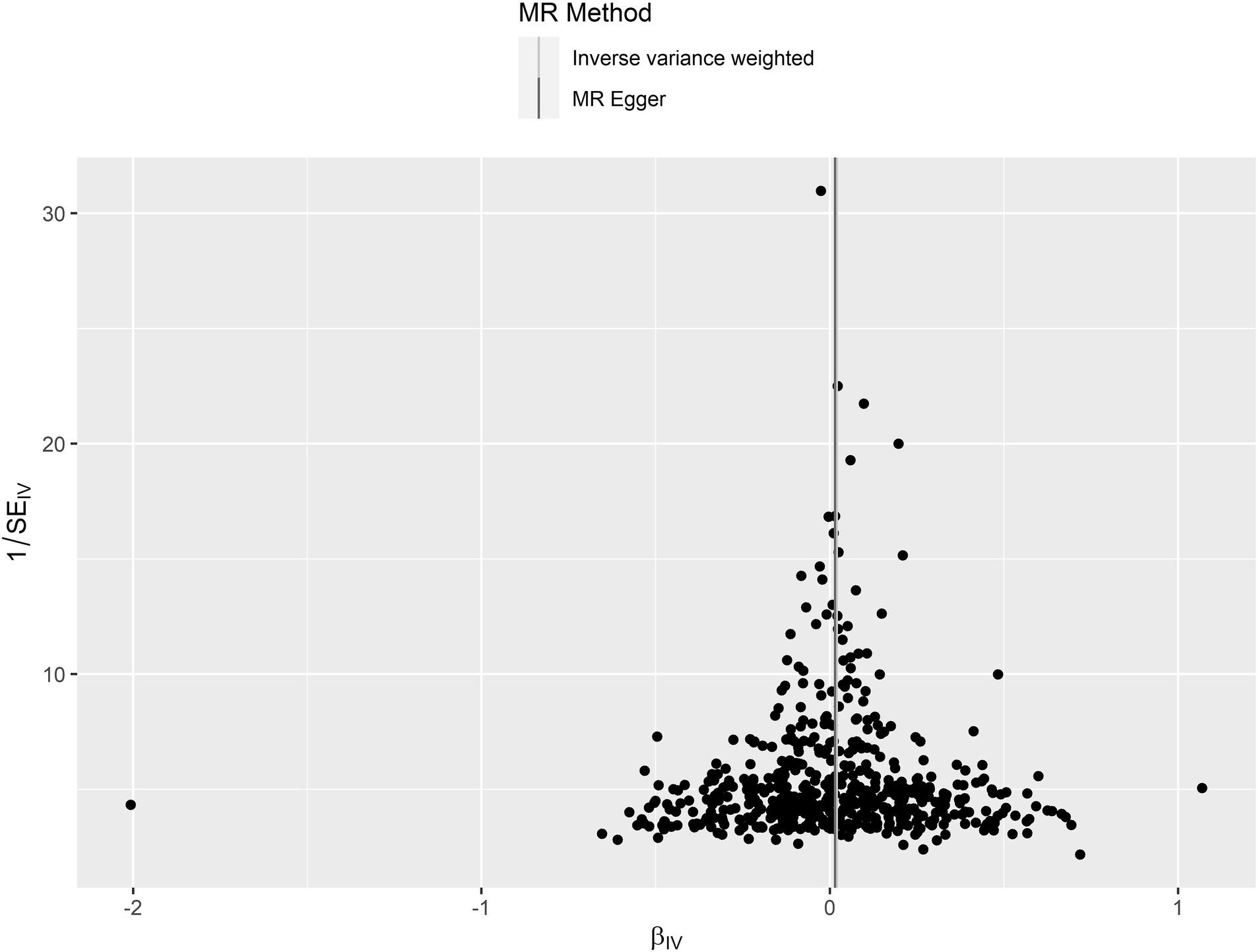
Figure 3. A funnel plot for circulating IGF-1 on PD. The figure shows that the scatter distribution exhibits a symmetrical shape with essentially no deviation from the overall. SE, Standard Error; MR, Mendelian randomization.
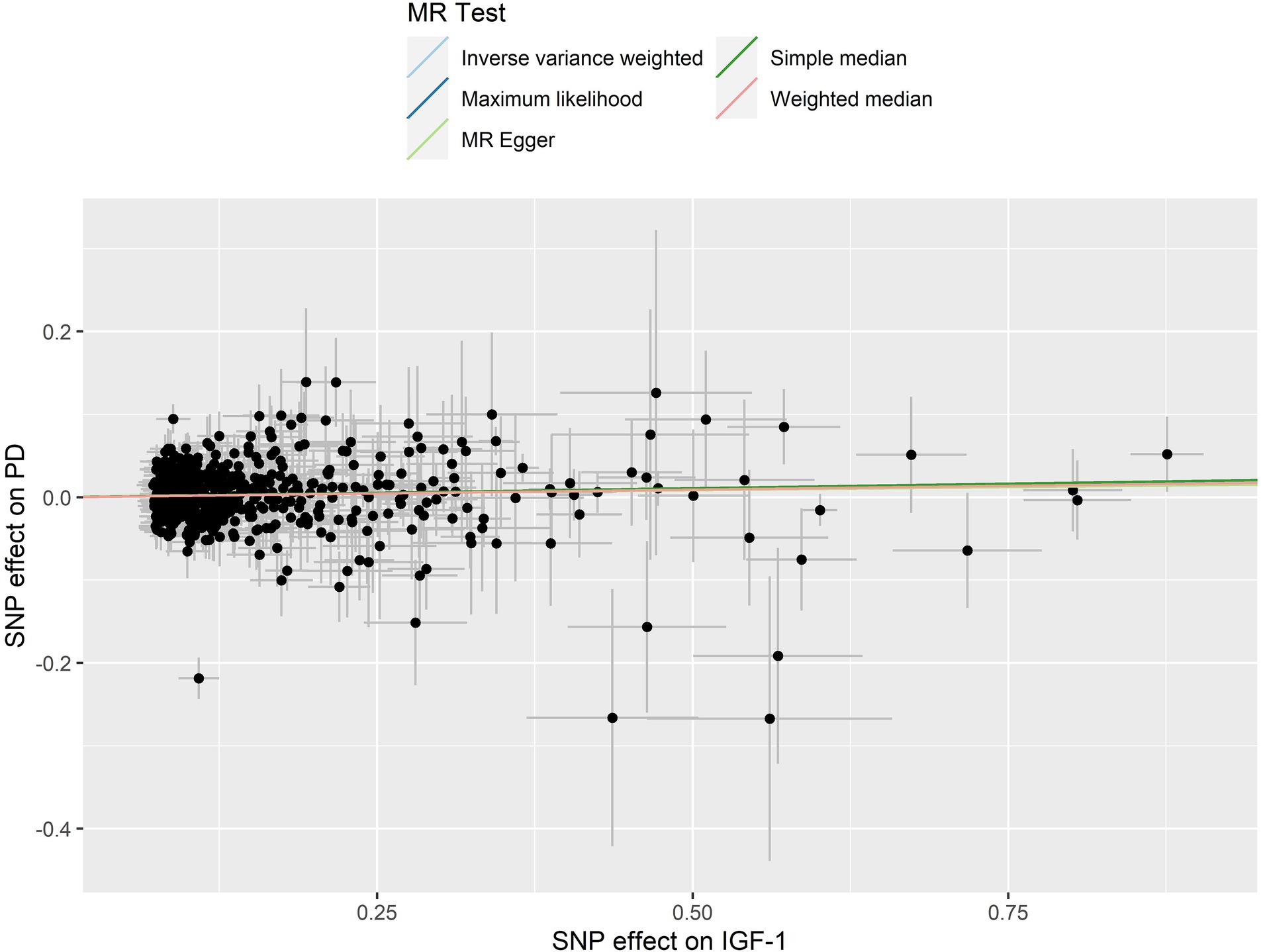
Figure 4. A scatter plot for circulating IGF-1 on PD. The figure showing the genetic correlation between circulating IGF-1 levels and PD risk based on different MR methods. The slopes of the lines represent the causal effect of each method. The slope of the straight line is greater than 0, indicating a positive overall effect of circulating IGF-1 levels on PD risk. SNP, Single Nucleotide Polymorphisms; IGF-1, Insulin-like growth factor-1; PD, Parkinson’s disease.
4 Discussion
Within our investigation, leveraging GWAS data from those of European ancestry, two independent MR analyses unveil a causal association between IGF-1 and PD risk to a degree. The robust findings and thorough sensitivity analyses adeptly validate the fulfilment of the three fundamental assumptions: relevance, independence, and exclusion restriction assumption.
Substantial evidence emphasizes the notable association between IGF-1 and brain health, particularly in conditions such as major depressive disorder (MDD), anxiety, and cognitive impairments (Mosiołek et al., 2021; Shi et al., 2023). Degenerative neurological conditions with greater impacts on populations have been linked to IGF-1 alterations (Watanabe et al., 2005; Gasperi and Castellano, 2010; Ghazi Sherbaf et al., 2018; Gubbi et al., 2018). However, the findings remain inconclusive when it comes to PD development. While both basic and translational research consistently assert that IGF-1 provides direct protection to all neuronal cells and can inhibit inflammatory cascade reactions at the cellular level (Fernandez et al., 2012; Labandeira-Garcia et al., 2017), there is currently a dearth of scholarly discourse addressing whether this anti-inflammatory effect holds protective implications in animal experiments. Furthermore, compelling evidence has linked IGF-1 to the initiation and progression of PD (Castilla-Cortázar et al., 2020).
In a study using the UK Biobank, the male sex was identified as a primary risk factor for PD, with elevated levels of IGF-1 ranking closely behind. This underscored its significant role in the patho-mechanisms of PD and its potential as a predictive biomarker (Allwright et al., 2023).
Elevated serum IGF-1 levels in PD patients have been negatively correlated with nonmotor symptoms, such as anxiety, depression, and cognitive dysfunction, and combining IGF-1 with EGF enhances the diagnostic value for PD (Shi et al., 2023).
A prospective study confirmed a positive association between IGF-1 concentration and the risk of PD (Cao et al., 2023), which is consistent with the direction of effect results obtained from our MR analysis. This association may be due to the involvement of IGF-1 in nerve growth and formation (Rabinovsky, 2004). IGF-1 serum concentrations might become an important biomarker for assessing the risk of PD, providing new perspectives on the prevention of PD.
The study had several strengths and limitations. The main strength was that Mendelian randomization analysis enhanced the comprehensive assessment of the association between circulating IGF-1 and PD by reducing bias from residual confounding and reverse causation. Moreover, the study used a large amount of PD case–control data, which was sufficient to detect even small effects. In addition, the genetic tools for IGF-1 had good validity, which ensured the robustness of our results. Despite the strengths of this study, there were some weaknesses. Firstly, the implementation of sex-specific MR analysis serves to address the inquiry stemming from the sex correlation of growth hormone-stimulated IGF-1 (Brabant and Wallaschofski, 2007). Nevertheless, despite exhaustive online searches, suitable data sources for analysis is elusive. Hence, presently, the initiation of hormone-related gender-stratified GWAS and subsequent core MR analysis were warranted. Secondly, we encounter a conspicuous limitation whereby the population is exclusively of European descent, hence precluding generalizability to other ethnicities, thereby rendering the applicability of our findings uncertain when extrapolated to different populations. Finally, the potential for a non-linear relationship between them cannot be disregarded, even though prevailing research indicates a linear relationship, necessitating analysis within the context of clinical practice.
5 Conclusion
This MR analysis report corroborates a causal nexus between serum IGF-1 levels and PD, establishing a pivotal groundwork for the forthcoming clinical diagnosis and management of PD.
Data availability statement
The datasets presented in this study can be found in online repositories. The names of the repository/repositories and accession number(s) can be found in the article/Supplementary material.
Ethics statement
Ethical approval was not required for the study involving humans in accordance with the local legislation and institutional requirements. Written informed consent to participate in this study was not required from the participants or the participants’ legal guardians/next of kin in accordance with the national legislation and the institutional requirements.
Author contributions
JX: Writing – original draft, Writing – review & editing. PF: Conceptualization, Methodology, Writing – review & editing. JY: Writing – original draft. MY: Validation, Writing – review & editing. JW: Conceptualization, Writing – review & editing. CC: Methodology, Writing – review & editing. JN: Conceptualization, Writing – review & editing.
Funding
The author(s) declare financial support was received for the research, authorship, and/or publication of this article. Our study was supported by National Natural Science Foundation of China (grant number 82273709), the Natural Science Foundation of Guangdong Province, China (grant number 2021A1515011038), Discipline construction project of Guangdong Medical University (grant number 4SG23001G), Non-funded Scientific and Technological Research Project in Zhanjiang (grant number 2023B01047).
Acknowledgments
We want to acknowledge all the GWASs for making the summary data publicly available, and we appreciate all the investigators and participants who contributed to those studies.
Conflict of interest
The authors declare that the research was conducted in the absence of any commercial or financial relationships that could be construed as a potential conflict of interest.
Publisher’s note
All claims expressed in this article are solely those of the authors and do not necessarily represent those of their affiliated organizations, or those of the publisher, the editors and the reviewers. Any product that may be evaluated in this article, or claim that may be made by its manufacturer, is not guaranteed or endorsed by the publisher.
Supplementary material
The Supplementary material for this article can be found online at: https://www.frontiersin.org/articles/10.3389/fnagi.2024.1333289/full#supplementary-material
References
Allwright, M., Mundell, H., Sutherland, G., Austin, P., and Guennewig, B. (2023). Machine learning analysis of the UK biobank reveals IGF-1 and inflammatory biomarkers predict Parkinson's disease risk. PLoS One 18:e0285416. doi: 10.1371/journal.pone.0285416
Balestrino, R., and Schapira, A. H. V. (2020). Parkinson disease. Eur. J. Neurol. 27, 27–42. doi: 10.1111/ene.14108
Berson, A., Nativio, R., Berger, S. L., and Bonini, N. M. (2018). Epigenetic regulation in neurodegenerative diseases. Trends Neurosci. 41, 587–598. doi: 10.1016/j.tins.2018.05.005
Bowden, J., Davey Smith, G., and Burgess, S. (2015). Mendelian randomization with invalid instruments: effect estimation and bias detection through egger regression. Int. J. Epidemiol. 44, 512–525. doi: 10.1093/ije/dyv080
Brabant, G., and Wallaschofski, H. (2007). Normal levels of serum IGF-I: determinants and validity of current reference ranges. Pituitary 10, 129–133. doi: 10.1007/s11102-007-0035-9
Burgess, S., Bowden, J., Fall, T., Ingelsson, E., and Thompson, S. G. (2017). Sensitivity analyses for robust causal inference from Mendelian randomization analyses with multiple genetic variants. Epidemiology 28, 30–42. doi: 10.1097/ede.0000000000000559
Burgess, S., Dudbridge, F., and Thompson, S. G. (2016). Combining information on multiple instrumental variables in Mendelian randomization: comparison of allele score and summarized data methods. Stat. Med. 35, 1880–1906. doi: 10.1002/sim.6835
Burgess, S., and Thompson, S. G. (2017). Interpreting findings from Mendelian randomization using the MR-egger method. Eur. J. Epidemiol. 32, 377–389. doi: 10.1007/s10654-017-0255-x
Cao, Z., Min, J., Tan, Q., Si, K., Yang, H., and Xu, C. (2023). Circulating insulin-like growth factor-1 and brain health: evidence from 369,711 participants in the UK biobank. Alzheimers Res. Ther. 15:140. doi: 10.1186/s13195-023-01288-5
Castilla-Cortázar, I., Aguirre, G. A., Femat-Roldán, G., Martín-Estal, I., and Espinosa, L. (2020). Is insulin-like growth factor-1 involved in Parkinson's disease development? J. Transl. Med. 18:70. doi: 10.1186/s12967-020-02223-0
Davey Smith, G., and Hemani, G. (2014). Mendelian randomization: genetic anchors for causal inference in epidemiological studies. Hum. Mol. Genet. 23, R89–R98. doi: 10.1093/hmg/ddu328
Davies, N. M., Hill, W. D., Anderson, E. L., Sanderson, E., Deary, I. J., and Davey, S. G. (2019). Multivariable two-sample Mendelian randomization estimates of the effects of intelligence and education on health. eLife 8:8. doi: 10.7554/eLife.43990
Deuschl, G., Beghi, E., Fazekas, F., Varga, T., Christoforidi, K. A., Sipido, E., et al. (2020). The burden of neurological diseases in Europe: an analysis for the global burden of disease study 2017. Lancet Public Health 5, e551–e567. doi: 10.1016/s2468-2667(20)30190-0
Dorsey, E. R., Constantinescu, R., Thompson, J. P., Biglan, K. M., Holloway, R. G., Kieburtz, K., et al. (2007). Projected number of people with Parkinson disease in the most populous nations, 2005 through 2030. Neurology 68, 384–386. doi: 10.1212/01.wnl.0000247740.47667.03
Dyer, A. H., Vahdatpour, C., Sanfeliu, A., and Tropea, D. (2016). The role of insulin-like growth factor 1 (IGF-1) in brain development, maturation and neuroplasticity. Neuroscience 325, 89–99. doi: 10.1016/j.neuroscience.2016.03.056
Fernandez, A. M., Jimenez, S., Mecha, M., Dávila, D., Guaza, C., Vitorica, J., et al. (2012). Regulation of the phosphatase calcineurin by insulin-like growth factor I unveils a key role of astrocytes in Alzheimer's pathology. Mol. Psychiatry 17, 705–718. doi: 10.1038/mp.2011.128
Gasperi, M., and Castellano, A. E. (2010). Growth hormone/insulin-like growth factor I axis in neurodegenerative diseases. J. Endocrinol. Investig. 33, 587–591. doi: 10.1007/bf03346653
Ghazi Sherbaf, F., Mohajer, B., Ashraf-Ganjouei, A., Mojtahed Zadeh, M., Javinani, A., Sanjari Moghaddam, H., et al. (2018). Serum insulin-like growth Factor-1 in Parkinson's disease; study of cerebrospinal fluid biomarkers and white matter microstructure. Front. Endocrinol. (Lausanne) 9:608. doi: 10.3389/fendo.2018.00608
Gubbi, S., Quipildor, G. F., Barzilai, N., Huffman, D. M., and Milman, S. (2018). 40 YEARS of IGF1: IGF1: the Jekyll and Hyde of the aging brain. J. Mol. Endocrinol. 61, T171–t185. doi: 10.1530/jme-18-0093
Huang, W., Xiao, J., Ji, J., and Chen, L. (2021). Association of lipid-lowering drugs with COVID-19 outcomes from a Mendelian randomization study. eLife 10:10. doi: 10.7554/eLife.73873
Kalia, L. V., and Lang, A. E. (2015). Parkinson's disease. Lancet 386, 896–912. doi: 10.1016/s0140-6736(14)61393-3
Labandeira-Garcia, J. L., Costa-Besada, M. A., Labandeira, C. M., Villar-Cheda, B., and Rodríguez-Perez, A. I. (2017). Insulin-like growth Factor-1 and Neuroinflammation. Front. Aging Neurosci. 9:365. doi: 10.3389/fnagi.2017.00365
Lee, A., and Gilbert, R. M. (2016). Epidemiology of Parkinson disease. Neurol. Clin. 34, 955–965. doi: 10.1016/j.ncl.2016.06.012
Milligan, B. G. (2003). Maximum-likelihood estimation of relatedness. Genetics 163, 1153–1167. doi: 10.1093/genetics/163.3.1153
Mosiołek, A., Mosiołek, J., Jakima, S., Pięta, A., and Szulc, A. (2021). Effects of antidepressant treatment on neurotrophic factors (BDNF and IGF-1) in patients with major depressive disorder (MDD). J. Clin. Med. 10:3377. doi: 10.3390/jcm10153377
O’Kusky, J., and Ye, P. (2012). Neurodevelopmental effects of insulin-like growth factor signaling. Front. Neuroendocrinol. 33, 230–251. doi: 10.1016/j.yfrne.2012.06.002
Ong, J. S., and MacGregor, S. (2019). Implementing MR-PRESSO and GCTA-GSMR for pleiotropy assessment in Mendelian randomization studies from a practitioner's perspective. Genet. Epidemiol. 43, 609–616. doi: 10.1002/gepi.22207
Pringsheim, T., Jette, N., Frolkis, A., and Steeves, T. D. (2014). The prevalence of Parkinson's disease: a systematic review and meta-analysis. Mov. Disord. 29, 1583–1590. doi: 10.1002/mds.25945
Rabinovsky, E. D. (2004). The multifunctional role of IGF-1 in peripheral nerve regeneration. Neurol. Res. 26, 204–210. doi: 10.1179/016164104225013851
Scorza, F. A., Almeida, A. C. G., Scorza, C. A., and Finsterer, J. (2021). Prevention of Parkinson’s disease-related sudden death. Clinics (São Paulo) 76:e3266. doi: 10.6061/clinics/2021/e3266
Scorza, F. A., Fiorini, A. C., Scorza, C. A., and Finsterer, J. (2018). Cardiac abnormalities in Parkinson's disease and parkinsonism. J. Clin. Neurosci. 53, 1–5. doi: 10.1016/j.jocn.2018.04.031
Shi, X., Zheng, J., Ma, J., Li, D., Gu, Q., Chen, S., et al. (2023). Correlation between serum IGF-1 and EGF levels and neuropsychiatric and cognitive in Parkinson's disease patients. Neurol. Sci. 44, 881–887. doi: 10.1007/s10072-022-06490-1
Smith, G. D., and Ebrahim, S. (2003). 'Mendelian randomization': can genetic epidemiology contribute to understanding environmental determinants of disease? Int. J. Epidemiol. 32, 1–22. doi: 10.1093/ije/dyg070
Storey, J. D., and Tibshirani, R. (2003). Statistical significance for genomewide studies. Proc. Natl. Acad. Sci. USA 100, 9440–9445. doi: 10.1073/pnas.1530509100
Verbanck, M., Chen, C. Y., Neale, B., and Do, R. (2018). Detection of widespread horizontal pleiotropy in causal relationships inferred from Mendelian randomization between complex traits and diseases. Nat. Genet. 50, 693–698. doi: 10.1038/s41588-018-0099-7
Keywords: Parkinson’s disease, causality, genetics, circulating insulin-like growth factor-1, Mendelian randomization
Citation: Xu J, Fan P, Yang J, Yin M, Wu J, Chen C and Ni J (2024) Causal relationship between circulating insulin-like growth factor-1 and Parkinson’s disease: a two-sample Mendelian randomization study. Front. Aging Neurosci. 16:1333289. doi: 10.3389/fnagi.2024.1333289
Edited by:
Manabu Funayama, Juntendo University, JapanReviewed by:
Vittorio Emanuele Bianchi, University of the Republic of San Marino, San MarinoKevin R. Duque, University of Cincinnati, United States
Copyright © 2024 Xu, Fan, Yang, Yin, Wu, Chen and Ni. This is an open-access article distributed under the terms of the Creative Commons Attribution License (CC BY). The use, distribution or reproduction in other forums is permitted, provided the original author(s) and the copyright owner(s) are credited and that the original publication in this journal is cited, in accordance with accepted academic practice. No use, distribution or reproduction is permitted which does not comply with these terms.
*Correspondence: Jindong Ni, nijd-gw@gdmu.edu.cn