- 1Department of Neurology, Xiangya Hospital, Central South University, Changsha, Hunan, China
- 2Clinical Research Center for Cerebrovascular Disease of Hunan Province, Central South University, Changsha, Hunan, China
- 3National Clinical Research Center for Geriatric Disorders, Xiangya Hospital, Central South University, Changsha, Hunan, China
- 4Department of Rehabilitation, Hunan Provincial People’s Hospital, The First Affiliated Hospital of Hunan Normal University, Changsha, Hunan, China
- 5Department of Geriatric Neurology, Xiangya Hospital, Central South University, Changsha, Hunan, China
Insulin-like growth factor-1 (IGF-1) is an active polypeptide protein that closely resembles the structural sequence of insulin and is involved in a variety of metabolic processes in the body. Decreased IGF-1 circulation levels are associated with an increased risk of stroke and a poorer prognosis, but the relationship with cerebral small vessel disease (cSVD) is unclear. Some studies found that the level of IGF-1 in patients with cSVD was significantly reduced, but the clinical significance and underlying mechanisms are unknown. This article reviews the correlation between IGF-1 and cerebrovascular disease and explores the potential relationship and mechanism between IGF-1 and cSVD.
1. Introduction
Cerebral small vessel disease (cSVD) is a common neurological disorder in elderly individuals that can lead to acute and chronic clinical syndromes, such as stroke and cognitive impairment, seriously affecting quality of life (Litak et al., 2020). Moreover, it has been shown that abnormal glucose metabolism may be an important risk factor for cSVD. Specifically, cSVD, particularly cerebral microhemorrhage, is more common in young people with type 1 diabetes than in healthy controls (Thorn et al., 2019). Furthermore, carotid-femoral pulse wave velocity and reactive hyperemia index values were higher and lower, respectively, in diabetic foot patients than in control diabetic patients without diabetic foot and healthy controls (Tuttolomondo et al., 2017), indicating endothelial dysfunction in diabetes.
Insulin-like growth factor-1 (IGF-1) is a bioactive peptide with high structural sequence similarity to insulin, involved in various metabolic processes in the body; furthermore, it is an important nutritional, growth, angiogenic and anti-apoptotic factor with neuroprotective effects on neuroinflammation and excitotoxicity (Wrigley et al., 2017; Hayes et al., 2021b). IGF-1 participates in various anti-inflammatory and pro-inflammatory processes in vivo, such as affecting the direction of polarization of microglia (Labandeira-Garcia et al., 2017). Moreover, neuroinflammation is an important link of stroke pathogenesis and affects the prognosis (Tuttolomondo et al., 2016). Studies have shown a negative correlation between age and circulating IGF-1 levels in humans and rodents, associated with a high risk of stroke and poor outcomes (Johnsen et al., 2005; Saber et al., 2017; Zhang et al., 2019; Lee et al., 2021). Additionally, altered IGF-1 levels are associated with an increased risk of multiple age-related neuropathies characterized by neurovascular dysfunction, excitotoxicity, and oxidative stress (Sonntag et al., 2013; Gubbi et al., 2018; Hayes et al., 2021a). However, the characteristics of clinically relevant changes in IGF-1 in cSVD remain unclear. Some studies have reported decreased IGF-1 levels in patients with cSVD and an association with cognitive impairment (Kang et al., 2021); however, the exact mechanism is not known. This article reviewed the potential relationship between IGF-1 and cSVD and its mechanisms.
2. Relationship between IGF-1 and cerebral vessel disease
2.1. IGF-1 and ischemic stroke
IGF-1 is associated with atherosclerosis and vascular disease development. For example, Framingham et al. found that circulating IGF-1 levels negatively correlate with ischemic stroke incidence, especially in patients with insulin resistance (Saber et al., 2017). Several clinical studies have found that patients with low circulating IGF-1 levels at admission have a higher mortality rate and are associated with increased stroke scale scores from the National Institute of Health several months after stroke (Bondanelli et al., 2006; De Smedt et al., 2011). A longitudinal study of chronic ischemic stroke survivors found that high IGF-1 levels 3 months post-stroke were associated with better Modified Rankin Scale scores (Åberg et al., 2011). Moreover, human IGF-1 levels are negatively correlated with the development of post-stroke depression (Zhang et al., 2018; Table 1).
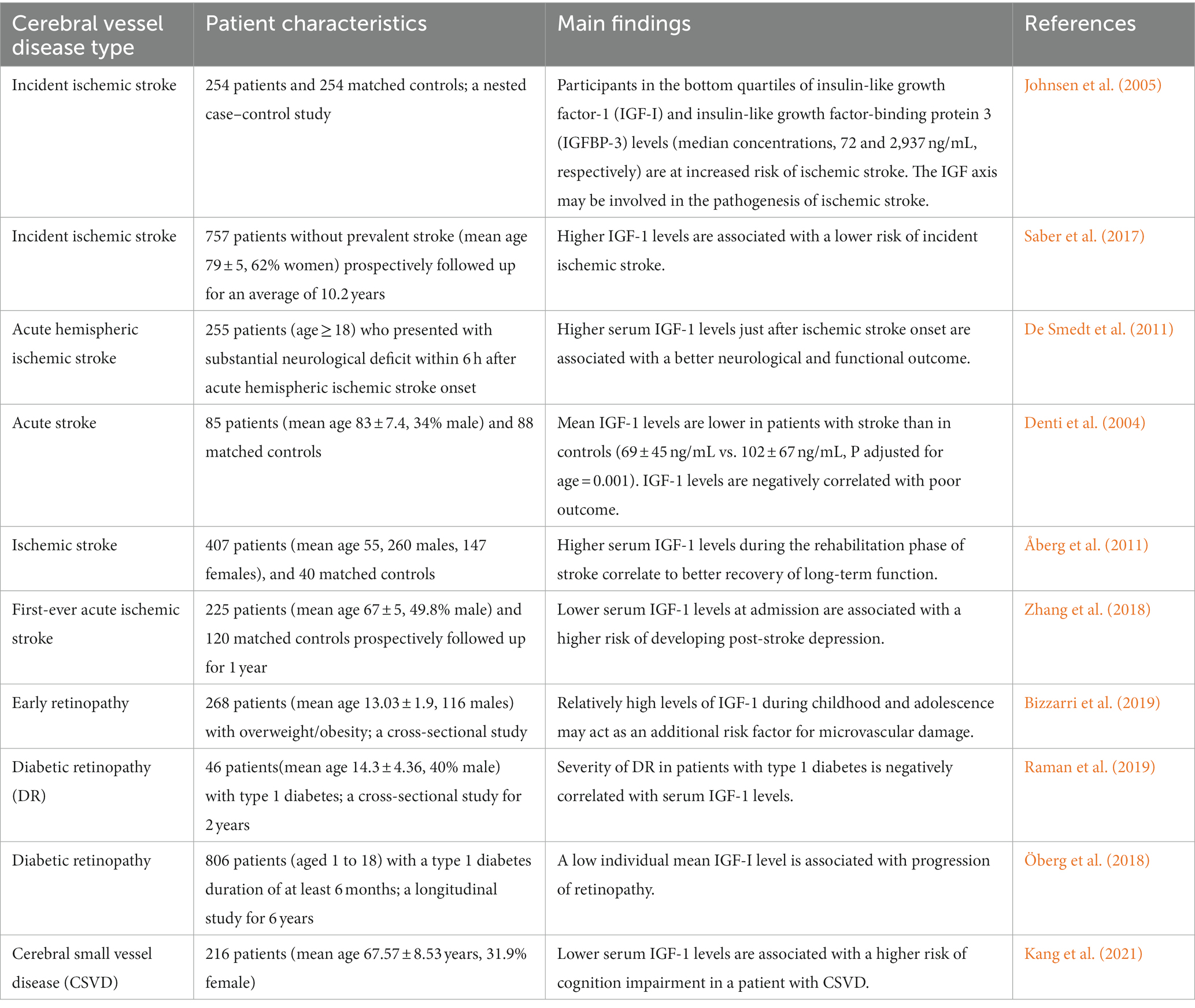
Table 1. The main findings of clinical studies on insulin-like growth factor-1 and cerebral vessel disease.
2.2. IGF-1 and cSVD
2.2.1. Clinical correlation between IGF-1 and cSVD
As an extension of the central nervous system (CNS), the retina exhibits a feature similar to the brain in terms of anatomy and physiological function, including microvascular structures (London et al., 2013). Studies have shown that patients with cSVD often present retinal changes associated with cerebral changes, such as the foveal outer plexiform layer volume being positively correlated with the number and volume ratio index of white matter lesions, and the temporal peripapillary retinal nerve fiber layer thickness being negatively correlated with it (Langner et al., 2022). IGF-1 is involved in the pathological process of retinal degeneration and may be associated with the microglia-mediated inflammatory response and insulin resistance (Arroba et al., 2018). A single-center cross-sectional study of overweight children and adolescents reported that IGF-1 levels were significantly elevated in patients with early atherosclerotic retinopathy independent of age, sex, and family history of type 2 diabetes, obesity, cardiovascular disease, hypertension, and dyslipidemia (Bizzarri et al., 2019). In contrast, in a study of adolescent patients with type 1 diabetes, retinopathy severity was negatively correlated with serum IGF-1 levels (Öberg et al., 2018; Raman et al., 2019; Table 1). However, not every vascular pathological sign seen in the cerebral due to IGF-1 deficiency will be shown in the retina. Induction of chronic hypertension in an IGF-1-deficient mouse model exacerbated the development of cerebral microhemorrhage, with retinal vascular abnormalities and gliosis, but without signs of retinal hemorrhage and retinal degeneration (Miller et al., 2022). Thus, IGF-1 might not be a good biomarker for using retinal changes as cerebrovascular changes.
cSVD is an important cause of vascular cognitive impairment (VCI). In humans, IGF-1 levels are positively correlated with hippocampal volume and memory (Maass et al., 2016; Norling et al., 2020). A study of patients with cSVD showed a significant correlation between significantly low IGF-I levels and Montreal Cognitive Assessment scores in patients with cognitive impairment (Kang et al., 2021). Another study of patients with cognitive impairment showed that patients with low IGF-1 levels showed dramatically lowered Mini-Mental State Examination scores over time (Vidal et al., 2016). Additionally, IGF-1 gene therapy was found to improve cognitive function in a mouse model of ischemic stroke (Zhu et al., 2008; Hayes et al., 2021b), suggesting that IGF-1 may play a role in cognitive impairment due to cSVD.
2.2.2. Effect of IGF-1 on cSVD-related imaging
A few studies have investigated the effect of IGF-1 on cSVD-related imaging indicators. A study found that patients with brain white matter injury with high baseline serum IGF-1 had better recovery (Feeney et al., 2017). A fetal sheep ischemic model showed that exogenous IGF-1 may decrease the loss and demyelination of postischemic oligodendrocytes and increase gliosis by inhibiting postischemic programmed cell death, thus playing a role in white matter protection (Guan et al., 2001). There are many similarities between cerebral microbleeds (CMBs) observed in IGF-1-deficient mice and in elderly hypertensive patients, including the relative size of the hemorrhage, clinical signs, and progressive nature of the pathological process. IGF-1 deficiency primarily increases the probability of cortical or subcortical CMBs (Toth et al., 2015b; Tarantini et al., 2017). In a study on the relationship between IGF-1, cognitive function, and neuroimaging indicators in elderly hypertensive patients, IGF-1 deficiency was found to be associated with hippocampal atrophy (Angelini et al., 2009). Another study showed that reduced serum IGF-1 levels were associated with brain atrophy and a high risk of Alzheimer’s disease in a healthy elderly population (Westwood et al., 2014).
3. Possible mechanism of IGF-1 in cSVD
3.1. Neurovascular units
The NVU is the blood–brain barrier’s basic structural and functional unit and plays an important role in SVD pathogenesis, and it consists of neurons, astrocytes, vascular endothelial cells, pericytes, and vascular smooth muscle cells (Figure 1; Yang et al., 2022). Studies have shown that IGF-1 can affect various components of the NVU (Figure 2; Table 2).
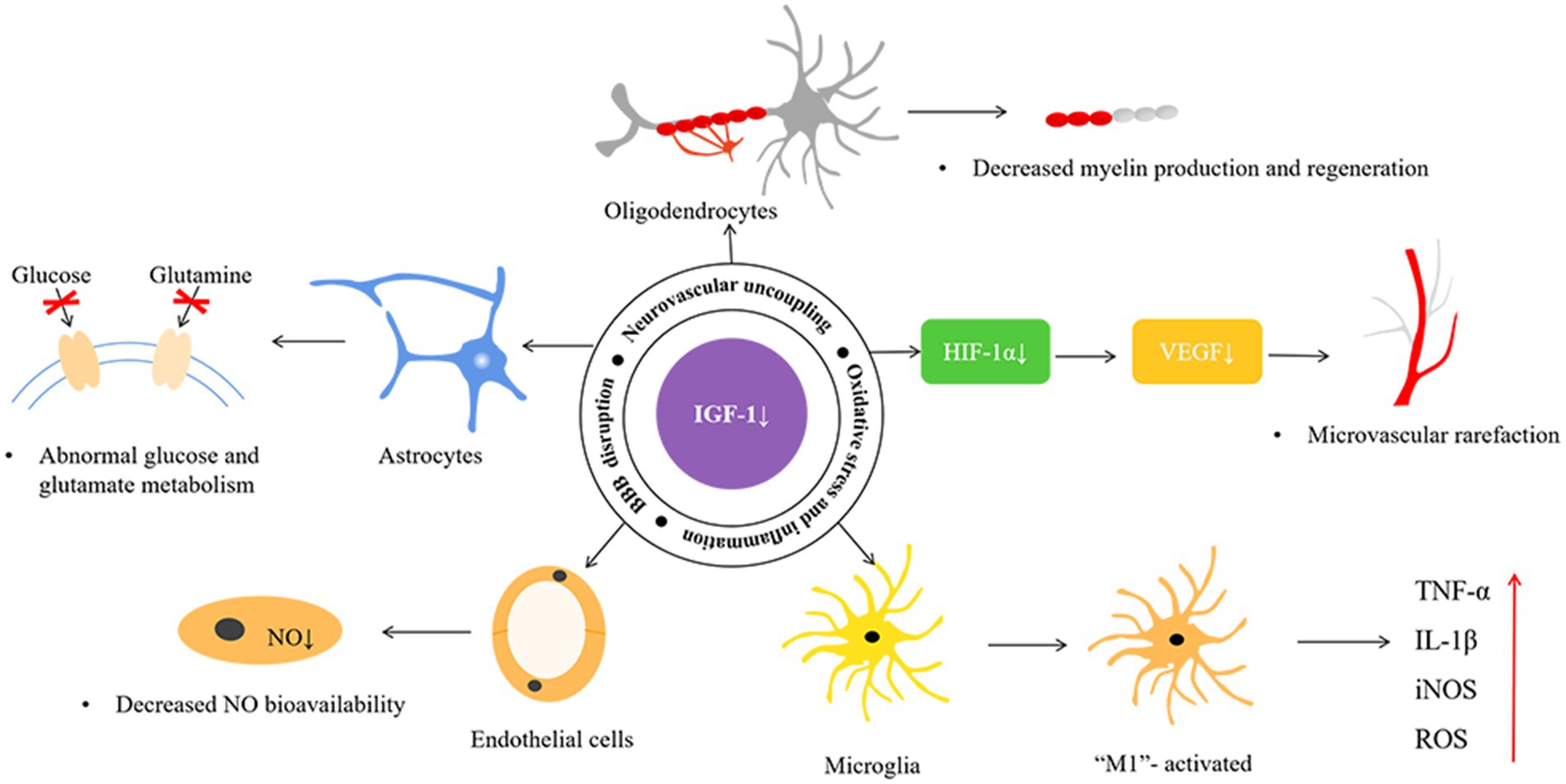
Figure 2. The possible mechanisms of insulin-like growth factor-1 and cerebral small vessel disease. HIF-1α, hypoxia-inducible factor-1α; VEGF, vascular endothelial growth factor; NO, nitric oxide; TNF-α, tumor necrosis factor-α; IL-1β, interleukin-1β; iNOS, inducible nitric oxide synthase; ROS, reactive oxygen species.
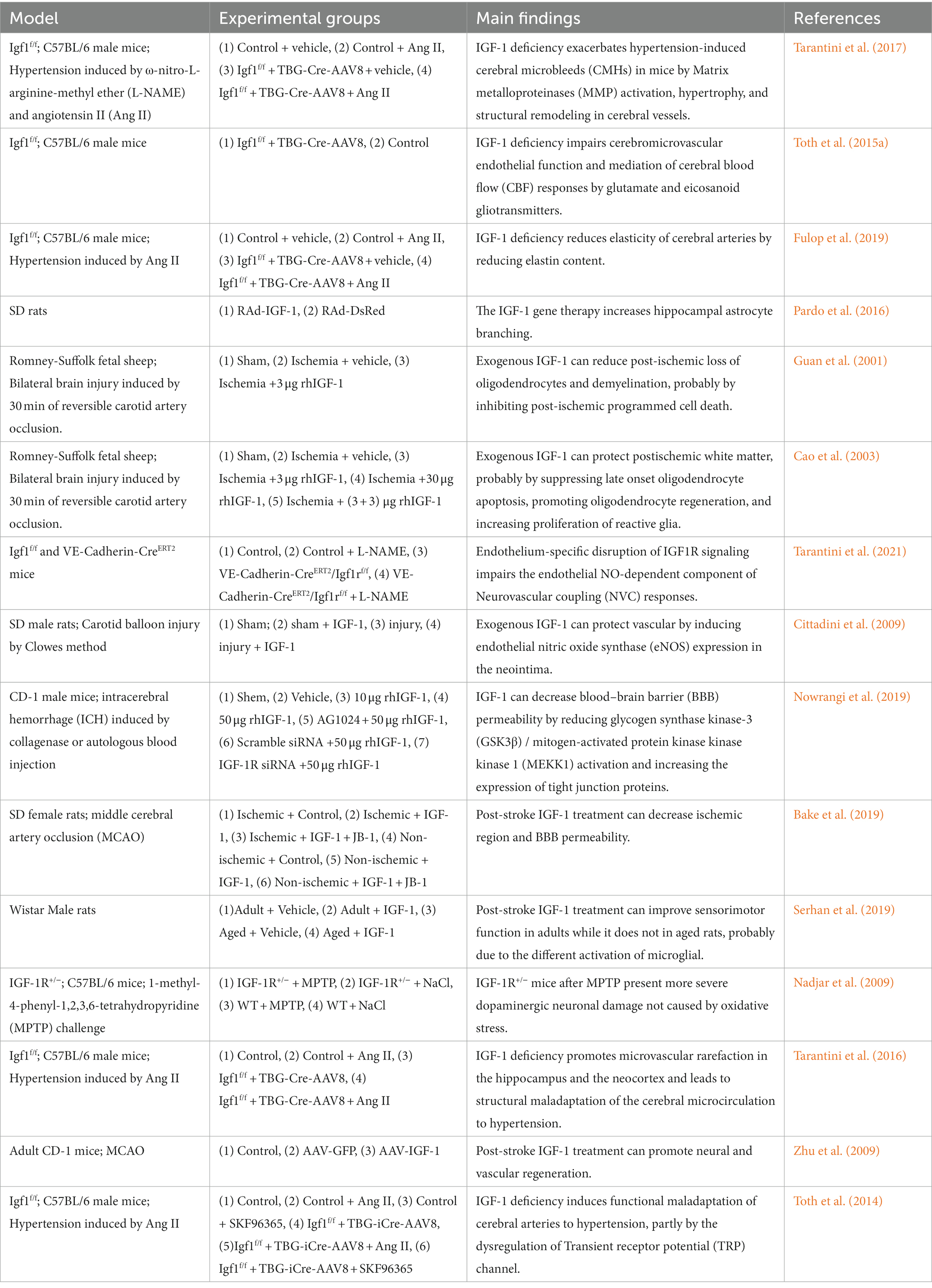
Table 2. The main findings of possible mechanism of insulin-like growth factor-1 and cerebral small vessel disease.
3.1.1. Astrocytes
Astrocytes play important roles in neurotransmitter metabolism, synaptic transmission, nutritional support, blood–brain barrier function, neuroimmunity, and neurovascular remodeling (Stanimirovic and Friedman, 2012; Hort et al., 2019). During neuronal activity, astrocytes can signal directly to the brain’s perivascular endothelial cells through the endfeet, which in turn regulate cerebral blood flow (CBF) and ensure energy supply (Price et al., 2018).
Deficient IGF-1 signaling in astrocytes has been reported to decrease glutamate metabolism in vitro and in vivo by reducing the expression and availability of cell surface glutamate transporter proteins. Glutamate excitotoxicity is a major driver of neurodegeneration after ischemic stroke. Age-associated IGF-1 deficiency may also indirectly impair neurological function by altering the function of the supporting glial cells and vasculature (Prabhu et al., 2019; Hayes et al., 2021a). IGF-1 deficiency also impairs the production and release of vasomediator eicosanoids from astrocytes, alters endothelial nitric oxide (NO) production, and increases susceptibility to hypertension-induced microhemorrhage (Toth et al., 2015a; Tarantini et al., 2017; Fulop et al., 2019). Furthermore, IGF-1 affects glucose metabolism in astrocytes (Hernandez-Garzón et al., 2016) and, during the inflammatory response, degeneration of astrocytes and endfeet may occur due to matrix metalloproteinase (MMP) release degrading the dystrophin-dystroglycan complex that anchors the endfeet to the basement membrane of the vasculature (Weekman and Wilcock, 2016; Price et al., 2018; Hort et al., 2019). Moreover, IGF-1 has been shown to increase the number of astrocytes, connexins, and gap junctions (Åberg et al., 2003), and gene therapy has shown that IGF-1 increases astrocyte branching (Pardo et al., 2016).
3.1.2. Oligodendrocytes
Oligodendrocytes play a key role in regulating neuronal excitability, maintaining and protecting normal neuronal function by wrapping around axons to form insulating myelin structures (Janowska et al., 2020).
Increasing evidence states an important role of IGF-1 in controlling oligodendrocyte function and the process of myelin production and regeneration (Mason et al., 2003; Zeger et al., 2007). In studies of ischemia–reperfusion injury, oligodendrocyte survival and myelin density were increased, and tissue swelling of white matter bundles was decreased in the sagittal parabasal gyrus within 90 min after IGF-1 administration, which may be related to apoptosis inhibition and increased oligodendrocyte proliferation (Guan et al., 2001; Cao et al., 2003). Although the protective effects of IGF-1 on oligodendrocytes have been demonstrated, the specific mechanisms involved remain to be investigated further.
3.1.3. Endothelial cells
Endothelial cells form the lining of blood vessels and play an important role in the exchange of plasma and tissue fluid. In addition, they can control vasodilation and vasoconstriction by releasing vasoactive substances and balancing coagulation and anticoagulation. Endothelial dysfunction can result in several vascular pathologies, such as vascular stiffness, demyelination, and blood–brain barrier disruption (Maiuolo et al., 2018).
Evidence suggests that IGF-1 deficiency significantly impairs NO-dependent components of endothelial cells (Tarantini et al., 2021). Reduced endothelium-derived NO was observed in VCI and in a model of chronic cerebral hypoperfusion in hypertensive rats (Ren et al., 2018). In an animal model of IGF-1 deficiency, which usually exhibited increased reactive oxygen species (ROS) production and decreased NO bioavailability (Csiszar et al., 2008), IGF-1 treatment up-regulates endothelial nitric oxide synthase (eNOS) and increases NO bioavailability (Cittadini et al., 2009; Sonntag et al., 2013).
3.1.4. Blood–brain barrier
Activation of the phosphatidylinositol 3-kinase-protein kinase B (PI3K-Akt) pathway has been shown to be activated by IGF-1 receptor stimulation, thereby attenuating the activation of glycogen synthase kinase-3β and mitogen-activated protein kinase kinase kinase 1 and increasing the expression of tight junction proteins (occludin and claudin-5), thus protecting the blood–brain barrier (Nowrangi et al., 2019). Animal models of deficient circulating IGF-1 usually exhibit impaired blood–brain barrier integrity (Hayes et al., 2021a), and in a stroke mouse model, IGF-1 infusion reduced blood–brain barrier permeability and decreased infarct volume caused by middle cerebral artery occlusion in middle-aged female rats (Bake et al., 2019). Recombinant IGF-1 injection improved blood–brain barrier permeability in a mouse model of intracerebral hemorrhage (Nowrangi et al., 2019).
3.2. Oxidative stress and inflammation
Many lines of evidence suggest that neuroinflammation is one of the primary pathophysiological mechanisms in cSVD, and microglia are the initiating factor in this process (Terasaki et al., 2014; Wardlaw et al., 2019). In the activated state, microglia have one of two cell phenotypes: M1 or M2. The M1 microglia have strong phagocytic capacity and secrete various pro-inflammatory factors, such as tumor necrosis factor-α (TNF-α), interleukin (IL)-6, inducible nitric oxide synthase (iNOS) and IL-1β, which enhance the inflammatory response and neurological injury, while the M2 microglia secrete anti-inflammatory mediators, such as IL-10 and transforming growth factor-β, which reduce inflammatory injury (Boche et al., 2013).
Numerous studies have shown that IGF-1 directly regulates microglial structure and function. Exogenous IGF-1 promotes M2 polarization of microglia, inhibits the M1 phenotype, alters the status of microglia phenotype activation, and reduces the production and release of microglia-associated TNF-α, IL-1β, iNOS, and ROS (Figure 2; Grinberg et al., 2013). Administration of IGF-1 after ischemic and/or hemorrhagic stroke attenuates microglial activation, inflammatory response, and ROS (Sohrabji and Williams, 2013; Serhan et al., 2019). A negative correlation between serum IL-6 and IGF-1 levels has been reported, and IGF/IGF binding protein-3 (IGFBP-3) administration to patients with severe burns induced anti-inflammatory effects and reduced IL-6 and TNF-α levels (Jeschke et al., 2000; Spies et al., 2002; Sukhanov et al., 2007; Higashi et al., 2012). IGF-1 deficiency has also been reported to exacerbate hypertension-induced oxidative stress, promote MMP activation, and lead to increased cerebral artery fragility, while antioxidant treatment attenuates age-associated hypertension-induced MMP activation (Tarantini et al., 2017). Moreover, IGF-1 can suppress the astrocyte response to inflammatory stimuli and protect neurons against neurotoxins (Labandeira-Garcia et al., 2017). The application of 1-methyl-4-phenyl-1,2,3,6-tetrahydropyridine in heterozygous IGF-1R mice performed more severe dopaminergic neuronal damage in the substantia nigra than it did in wild-type animals (Nadjar et al., 2009). Furthermore, exogenous IGF-I and IGF-I gene therapy inhibited the expression of toll-like receptor 4 and reduced the inflammatory response of astrocytes (Bellini et al., 2011).
3.3. Hemodynamics
3.3.1. Microvascular rarefaction
Vascular density is closely associated with local blood perfusion. MVR reduces blood flow, which can lead to cerebral ischemic disease. Reduced availability of certain hormones and growth factors, including growth hormone, IGF-1, and vascular endothelial growth factor (VEGF), may contribute to MVR (Norling et al., 2020).
VEGF regulates microvascular density in the CNS, and its release is primarily induced by hypoxia-inducible factor-1α stimulated by IGF-1 (Figure 2; Lopez-Lopez et al., 2004; Sonntag et al., 2013). Animal and human studies suggest chronic VEGF inhibition leads to decreased endothelial cell survival, reduced blood flow, and vascular loss. Using IGF-1R-targeted antibodies to treat cancer patients can indirectly inhibit angiogenesis by suppressing VEGF production (Bid et al., 2012). Another study found that endocrine IGF-1 deficiency in IGF-1 knockout mice promoted hypertension-induced loss of hippocampal and neocortical microvessel density, which was reversed by IGF-1 infusion (Tarantini et al., 2016). Post-ischemic treatment with IGF-1 effectively promoted neural and vascular regeneration in the chronic stage of cerebral infarction. IGF-1 gene transfer significantly enhanced neovascularization and vascular density in the peri-infarct and injection needle tract area (Zhu et al., 2009).
3.3.2. Regulation of CBF
Age-associated IGF-1 deficiency plays an important role in cerebral artery maladaptation to changes in the hemodynamic environment (Ungvari and Csiszar, 2012). Circulating IGF-1 levels decrease significantly with age and are associated with decreased basal CBF, and an age-related decrease in IGF-1 level may predict the magnitude of age-related decrease in neurovascular coupling response (Toth et al., 2022). Another study showed that hypertension in IGF-1-deficient mice is associated with impaired adaptive changes in myogenic constriction of cerebral arteries, mimicking the aging phenotype (Toth et al., 2014). Hypertension induction in IGF-1 knockout mice revealed impaired cerebrovascular autoregulation (Tarantini et al., 2017; Fulop et al., 2019). This functional maladaptation and increased microhemorrhage risk in hypertension may be related to the fact that IGF-1 deficiency disrupts the vascular remodeling process by impairing hypertension-induced adaptive media hypertrophy and dysregulating extracellular matrix remodeling, resulting in weakened cerebral arteries and increased circumferential stress (Tarantini et al., 2017; Norling et al., 2020).
4. Conclusion and outlook
In conclusion, serum IGF-1 levels might reflect the severity of cSVD and be associated with cognitive decline. Although IGF-1 may influence the formation and progression of cSVD in terms of NVU, CBF regulation, and neuroinflammation, evidence from clinical studies is lacking. The correlation between cSVD and IGF-1 is still being investigated, and it remains to be confirmed whether IGF-1 can be a predictor of cSVD by numerous basic and clinical studies. At the same time, these mechanisms are interrelated, and determining their specific order of occurrence is difficult. Therefore, the specific mechanisms by which IGF-1 affects cSVD remain to be elucidated.
A study found that stroke patients and high IGF1/IGFBP-3 ratios had better outcomes (Denti et al., 2004). Another study of the relationship between cognitive impairment and IGF-1 and IGFBP-3 found that plasma IGF-1 concentrations were not associated with dementia incidence, while doubling of plasma IGFBP-3 concentration reduced the risk ratio of dementia by 23% (Almeida et al., 2018). Therefore, future studies should probably not be limited to IGF-1 alone; the role of IGFBPs in regulating IGF-1 utilization may be a direction for further research.
Author contributions
HD: investigation, data curation, and writing-original draft. JX, LH, and LZ: investigation and data curation. WG and FY: conceptualization, supervision, and writing-review and editing. All authors contributed to the article and approved the submitted version.
Acknowledgments
We would like to thank Editage (www.editage.cn) for English language editing.
Conflict of interest
The authors declare that the research was conducted in the absence of any commercial or financial relationships that could be construed as a potential conflict of interest.
Publisher’s note
All claims expressed in this article are solely those of the authors and do not necessarily represent those of their affiliated organizations, or those of the publisher, the editors and the reviewers. Any product that may be evaluated in this article, or claim that may be made by its manufacturer, is not guaranteed or endorsed by the publisher.
References
Åberg, N. D., Blomstrand, F., Åberg, M. A. I., Björklund, U., Carlsson, B., Carlsson-Skwirut, C., et al. (2003). Insulin-like growth factor-I increases astrocyte intercellular gap junctional communication and connexin43 expression in vitro. J. Neurosci. Res. 74, 12–22. doi: 10.1002/jnr.10734
Åberg, D., Jood, K., Blomstrand, C., Jern, C., Nilsson, M., Isgaard, J., et al. (2011). Serum IGF-I levels correlate to improvement of functional outcome after ischemic stroke. J. Clin. Endocrinol. Metab. 96, E1055–E1064. doi: 10.1210/jc.2010-2802
Almeida, O. P., Hankey, G. J., Yeap, B. B., Paul Chubb, S. A., Gollege, J., and Flicker, L. (2018). Risk of prevalent and incident dementia associated with insulin-like growth factor and insulin-like growth factor-binding protein 3. Mol. Psychiatry 23, 1825–1829. doi: 10.1038/mp.2017.152
Angelini, A., Bendini, C., Neviani, F., Bergamini, L., Manni, B., Trenti, T., et al. (2009). Insulin-like growth factor-1 (IGF-1): relation with cognitive functioning and neuroimaging marker of brain damage in a sample of hypertensive elderly subjects. Arch. Gerontol. Geriatr. 49, 5–12. doi: 10.1016/j.archger.2009.09.006
Arroba, A. I., Campos-Caro, A., Aguilar-Diosdado, M., and Valverde, Á. M. (2018). IGF-1, inflammation and retinal degeneration: a close network. Front. Aging Neurosci. 10:203. doi: 10.3389/fnagi.2018.00203
Bake, S., Okoreeh, A., Khosravian, H., and Sohrabji, F. (2019). Insulin-like growth factor (IGF)-1 treatment stabilizes the microvascular cytoskeleton under ischemic conditions. Exp. Neurol. 311, 162–172. doi: 10.1016/j.expneurol.2018.09.016
Bellini, M. J., Hereñú, C. B., Goya, R. G., and Garcia-Segura, L. M. (2011). Insulin-like growth factor-I gene delivery to astrocytes reduces their inflammatory response to lipopolysaccharide. J. Neuroinflammation 8:21. doi: 10.1186/1742-2094-8-21
Bid, H. K., Zhan, J., Phelps, D. A., Kurmasheva, R. T., and Houghton, P. J. (2012). Potent inhibition of angiogenesis by the IGF-1 receptor-targeting antibody SCH717454 is reversed by IGF-2. Mol. Cancer Ther. 11, 649–659. doi: 10.1158/1535-7163.Mct-11-0575
Bizzarri, C., Pedicelli, S., Romanzo, A., Bocchini, S., Bottaro, G., Cianfarani, S., et al. (2019). The impact of IGF-I, puberty and obesity on early retinopathy in children: a cross-sectional study. Ital. J. Pediatr. 45:52. doi: 10.1186/s13052-019-0650-x
Boche, D., Perry, V. H., and Nicoll, J. A. (2013). Review: activation patterns of microglia and their identification in the human brain. Neuropathol. Appl. Neurobiol. 39, 3–18. doi: 10.1111/nan.12011
Bondanelli, M., Ambrosio, M. R., Onofri, A., Bergonzoni, A., Lavezzi, S., Zatelli, M. C., et al. (2006). Predictive value of circulating insulin-like growth factor I levels in ischemic stroke outcome. J. Clin. Endocrinol. Metab. 91, 3928–3934. doi: 10.1210/jc.2006-1040
Cao, Y., Gunn, A. J., Bennet, L., Wu, D., George, S., Gluckman, P. D., et al. (2003). Insulin-like growth factor (IGF)-1 suppresses oligodendrocyte caspase-3 activation and increases glial proliferation after ischemia in near-term fetal sheep. J. Cereb. Blood Flow Metab. 23, 739–747. doi: 10.1097/01.Wcb.0000067720.12805.6f
CITTADINI, A., MONTI, M. G., CASTIELLO, M. C., D’ARCO, E., GALASSO, G., SORRIENTO, D., et al. (2009). Insulin-like growth factor-1 protects from vascular stenosis and accelerates re-endothelialization in a rat model of carotid artery injury. J. Thromb. Haemost. 7, 1920–1928. doi: 10.1111/j.1538-7836.2009.03607.x
Csiszar, A., Labinskyy, N., Perez, V., Recchia, F. A., Podlutsky, A., Mukhopadhyay, P., et al. (2008). Endothelial function and vascular oxidative stress in long-lived GH/IGF-deficient Ames dwarf mice. Am. J. Physiol. Heart Circ. Physiol. 295, H1882–H1894. doi: 10.1152/ajpheart.412.2008
de Smedt, A., Brouns, R., Uyttenboogaart, M., de Raedt, S., Moens, M., Wilczak, N., et al. (2011). Insulin-like growth factor I serum levels influence ischemic stroke outcome. Stroke 42, 2180–2185. doi: 10.1161/strokeaha.110.600783
Denti, L., Annoni, V., Cattadori, E., Angela Salvagnini, M., Visioli, S., Francesca Merli, M., et al. (2004). Insulin-like growth factor 1 as a predictor of ischemic stroke outcome in the elderly. Am. J. Med. 117, 312–317. doi: 10.1016/j.amjmed.2004.02.049
Feeney, C., Sharp, D. J., Hellyer, P. J., Jolly, A. E., Cole, J. H., Scott, G., et al. (2017). Serum insulin-like growth factor-I levels are associated with improved white matter recovery after traumatic brain injury. Ann. Neurol. 82, 30–43. doi: 10.1002/ana.24971
Fulop, G. A., Ramirez-Perez, F. I., Kiss, T., Tarantini, S., Valcarcel Ares, M. N., Toth, P., et al. (2019). IGF-1 deficiency promotes pathological remodeling of cerebral arteries: a potential mechanism contributing to the pathogenesis of intracerebral hemorrhages in aging. J. Gerontol. A Biol. Sci. Med. Sci. 74, 446–454. doi: 10.1093/gerona/gly144
Grinberg, Y. Y., Dibbern, M. E., Levasseur, V. A., and Kraig, R. P. (2013). Insulin-like growth factor-1 abrogates microglial oxidative stress and TNF-α responses to spreading depression. J. Neurochem. 126, 662–672. doi: 10.1111/jnc.12267
Guan, J., Bennet, L., George, S., Wu, D., Waldvogel, H. J., Gluckman, P. D., et al. (2001). Insulin-like growth factor-1 reduces postischemic white matter injury in fetal sheep. J. Cereb. Blood Flow Metab. 21, 493–502. doi: 10.1097/00004647-200105000-00003
Gubbi, S., Quipildor, G. F., Barzilai, N., Huffman, D. M., and Milman, S. (2018). 40 YEARS of IGF1: IGF1: the Jekyll and Hyde of the aging brain. J. Mol. Endocrinol. 61, T171–t185. doi: 10.1530/jme-18-0093
Hayes, C. A., Ashmore, B. G., Vijayasankar, A., Marshall, J. P., and Ashpole, N. M. (2021a). Insulin-like growth Factor-1 differentially modulates glutamate-induced toxicity and stress in cells of the Neurogliovascular unit. Front. Aging Neurosci. 13:751304. doi: 10.3389/fnagi.2021.751304
Hayes, C. A., Valcarcel-Ares, M. N., and Ashpole, N. M. (2021b). Preclinical and clinical evidence of IGF-1 as a prognostic marker and acute intervention with ischemic stroke. J. Cereb. Blood Flow Metab. 41, 2475–2491. doi: 10.1177/0271678x211000894
Hernandez-Garzón, E., Fernandez, A. M., Perez-Alvarez, A., Genis, L., Bascuñana, P., Fernandez de la Rosa, R., et al. (2016). The insulin-like growth factor I receptor regulates glucose transport by astrocytes. Glia 64, 1962–1971. doi: 10.1002/glia.23035
Higashi, Y., Sukhanov, S., Anwar, A., Shai, S. Y., and Delafontaine, P. (2012). Aging, atherosclerosis, and IGF-1. J. Gerontol. A Biol. Sci. Med. Sci. 67, 626–639. doi: 10.1093/gerona/gls102
Hort, J., Vališ, M., Kuča, K., and Angelucci, F. (2019). Vascular cognitive impairment: information from animal models on the pathogenic mechanisms of cognitive deficits. Int. J. Mol. Sci. 20:2405. doi: 10.3390/ijms20102405
Janowska, J., Gargas, J., Ziemka-Nalecz, M., Zalewska, T., and Sypecka, J. (2020). Oligodendrocyte response to pathophysiological conditions triggered by episode of perinatal hypoxia-ischemia: role of IGF-1 secretion by glial cells. Mol. Neurobiol. 57, 4250–4268. doi: 10.1007/s12035-020-02015-z
Jeschke, M. G., Barrow, R. E., and Herndon, D. N. (2000). Insulinlike growth factor I plus insulinlike growth factor binding protein 3 attenuates the proinflammatory acute phase response in severely burned children. Ann. Surg. 231, 246–252. doi: 10.1097/00000658-200002000-00014
Johnsen, S. P., Hundborg, H. H., Sørensen, H. T., Ørskov, H., Tjønneland, A., Overvad, K., et al. (2005). Insulin-like growth factor (IGF) I, -II, and IGF binding protein-3 and risk of ischemic stroke. J. Clin. Endocrinol. Metab. 90, 5937–5941. doi: 10.1210/jc.2004-2088
Kang, J., Luo, W., Zhang, C., Ren, Y., Cao, L., Wu, J., et al. (2021). Positive association between serum insulin-like growth Factor-1 and cognition in patients with cerebral small vessel disease. J. Stroke Cerebrovasc. Dis. 30:105790. doi: 10.1016/j.jstrokecerebrovasdis.2021.105790
Labandeira-Garcia, J. L., Costa-Besada, M. A., Labandeira, C. M., Villar-Cheda, B., and Rodríguez-Perez, A. I. (2017). Insulin-like growth Factor-1 and Neuroinflammation. Front. Aging Neurosci. 9:365. doi: 10.3389/fnagi.2017.00365
Langner, S. M., Terheyden, J. H., Geerling, C. F., Kindler, C., Keil, V. C. W., Turski, C. A., et al. (2022). Structural retinal changes in cerebral small vessel disease. Sci. Rep. 12:9315. doi: 10.1038/s41598-022-13312-z
Lee, J., Lee, J., Lee, M., Lim, J. S., Kim, J. H., Yu, K. H., et al. (2021). Association between serum insulin-like growth Factor-1 and neurological severity in acute ischemic stroke. J. Clin. Neurol. 17, 206–212. doi: 10.3988/jcn.2021.17.2.206
Litak, J., Mazurek, M., Kulesza, B., Szmygin, P., Litak, J., Kamieniak, P., et al. (2020). Cerebral Small Vessel Disease. Int. J. Mol. Sci. 21:49729. doi: 10.3390/ijms21249729
London, A., Benhar, I., and Schwartz, M. (2013). The retina as a window to the brain-from eye research to CNS disorders. Nat. Rev. Neurol. 9, 44–53. doi: 10.1038/nrneurol.2012.227
Lopez-Lopez, C., LeRoith, D., and Torres-Aleman, I. (2004). Insulin-like growth factor I is required for vessel remodeling in the adult brain. Proc. Natl. Acad. Sci. U. S. A. 101, 9833–9838. doi: 10.1073/pnas.0400337101
Maass, A., Düzel, S., Brigadski, T., Goerke, M., Becke, A., Sobieray, U., et al. (2016). Relationships of peripheral IGF-1, VEGF and BDNF levels to exercise-related changes in memory, hippocampal perfusion and volumes in older adults. NeuroImage 131, 142–154. doi: 10.1016/j.neuroimage.2015.10.084
Maiuolo, J., Gliozzi, M., Musolino, V., Scicchitano, M., Carresi, C., Scarano, F., et al. (2018). The “frail” brain blood barrier in neurodegenerative diseases: role of early disruption of endothelial cell-to-cell connections. Int. J. Mol. Sci. 19:92693. doi: 10.3390/ijms19092693
Mason, J. L., Xuan, S., Dragatsis, I., Efstratiadis, A., and Goldman, J. E. (2003). Insulin-like growth factor (IGF) signaling through type 1 IGF receptor plays an important role in remyelination. J. Neurosci. 23, 7710–7718. doi: 10.1523/jneurosci.23-20-07710.2003
Miller, L. R., Tarantini, S., Nyúl-Tóth, Á., Johnston, M. P., Martin, T., Bullen, E. C., et al. (2022). Increased susceptibility to cerebral microhemorrhages is associated with imaging signs of microvascular degeneration in the retina in an insulin-like growth factor 1 deficient mouse model of accelerated aging. Front. Aging Neurosci. 14:788296. doi: 10.3389/fnagi.2022.788296
Nadjar, A., Berton, O., Guo, S., Leneuve, P., Dovero, S., Diguet, E., et al. (2009). IGF-1 signaling reduces neuro-inflammatory response and sensitivity of neurons to MPTP. Neurobiol. Aging 30, 2021–2030. doi: 10.1016/j.neurobiolaging.2008.02.009
Norling, A. M., Gerstenecker, A. T., Buford, T. W., Khan, B., Oparil, S., and Lazar, R. M. (2020). The role of exercise in the reversal of IGF-1 deficiencies in microvascular rarefaction and hypertension. Geroscience 42, 141–158. doi: 10.1007/s11357-019-00139-2
Nowrangi, D. S., McBride, D., Manaenko, A., Dixon, B., Tang, J., and Zhang, J. H. (2019). rhIGF-1 reduces the permeability of the blood-brain barrier following intracerebral hemorrhage in mice. Exp. Neurol. 312, 72–81. doi: 10.1016/j.expneurol.2018.11.009
Öberg, D., Salemyr, J., Örtqvist, E., Juul, A., and Bang, P. (2018). A longitudinal study of serum insulin-like growth factor-I levels over 6 years in a large cohort of children and adolescents with type 1 diabetes mellitus: a marker reflecting diabetic retinopathy. Pediatr. Diabetes 19, 972–978. doi: 10.1111/pedi.12681
Pardo, J., Uriarte, M., Cónsole, G. M., Reggiani, P. C., Outeiro, T. F., Morel, G. R., et al. (2016). Insulin-like growth factor-I gene therapy increases hippocampal neurogenesis, astrocyte branching and improves spatial memory in female aging rats. Eur. J. Neurosci. 44, 2120–2128. doi: 10.1111/ejn.13278
Prabhu, D., Khan, S. M., Blackburn, K., Marshall, J. P., and Ashpole, N. M. (2019). Loss of insulin-like growth factor-1 signaling in astrocytes disrupts glutamate handling. J. Neurochem. 151, 689–702. doi: 10.1111/jnc.14879
Price, B. R., Norris, C. M., Sompol, P., and Wilcock, D. M. (2018). An emerging role of astrocytes in vascular contributions to cognitive impairment and dementia. J. Neurochem. 144, 644–650. doi: 10.1111/jnc.14273
Raman, P., Singal, A. K., and Behl, A. (2019). Effect of insulin-like growth Factor-1 on diabetic retinopathy in pubertal age patients with type 1 diabetes. Asia Pac J Ophthalmol (Phila) 8, 319–323. doi: 10.1097/apo.0000000000000250
Ren, C., Li, N., Li, S., Han, R., Huang, Q., Hu, J., et al. (2018). Limb ischemic conditioning improved cognitive deficits via eNOS-dependent augmentation of angiogenesis after chronic cerebral Hypoperfusion in rats. Aging Dis. 9, 869–879. doi: 10.14336/ad.2017.1106
Saber, H., Himali, J. J., Beiser, A. S., Shoamanesh, A., Pikula, A., Roubenoff, R., et al. (2017). Serum insulin-like growth factor 1 and the risk of ischemic stroke: the Framingham study. Stroke 48, 1760–1765. doi: 10.1161/strokeaha.116.016563
Serhan, A., Boddeke, E., and Kooijman, R. (2019). Insulin-like growth Factor-1 is neuroprotective in aged rats with ischemic stroke. Front. Aging Neurosci. 11:349. doi: 10.3389/fnagi.2019.00349
Sohrabji, F., and Williams, M. (2013). Stroke neuroprotection: oestrogen and insulin-like growth factor-1 interactions and the role of microglia. J. Neuroendocrinol. 25, 1173–1181. doi: 10.1111/jne.12059
Sonntag, W. E., Deak, F., Ashpole, N., Toth, P., Csiszar, A., Freeman, W., et al. (2013). Insulin-like growth factor-1 in CNS and cerebrovascular aging. Front. Aging Neurosci. 5:27. doi: 10.3389/fnagi.2013.00027
Spies, M., Wolf, S. E., Barrow, R. E., Jeschke, M. G., and Herndon, D. N. (2002). Modulation of types I and II acute phase reactants with insulin-like growth factor-1/binding protein-3 complex in severely burned children. Crit. Care Med. 30, 83–88. doi: 10.1097/00003246-200201000-00013
Stanimirovic, D. B., and Friedman, A. (2012). Pathophysiology of the neurovascular unit: disease cause or consequence? J. Cereb. Blood Flow Metab. 32, 1207–1221. doi: 10.1038/jcbfm.2012.25
Sukhanov, S., Higashi, Y., Shai, S. Y., Vaughn, C., Mohler, J., Li, Y., et al. (2007). IGF-1 reduces inflammatory responses, suppresses oxidative stress, and decreases atherosclerosis progression in ApoE-deficient mice. Arterioscler. Thromb. Vasc. Biol. 27, 2684–2690. doi: 10.1161/atvbaha.107.156257
Tarantini, S., Nyúl-Tóth, Á., Yabluchanskiy, A., Csipo, T., Mukli, P., Balasubramanian, P., et al. (2021). Endothelial deficiency of insulin-like growth factor-1 receptor (IGF1R) impairs neurovascular coupling responses in mice, mimicking aspects of the brain aging phenotype. Geroscience 43, 2387–2394. doi: 10.1007/s11357-021-00405-2
Tarantini, S., Tucsek, Z., Valcarcel-Ares, M. N., Toth, P., Gautam, T., Giles, C. B., et al. (2016). Circulating IGF-1 deficiency exacerbates hypertension-induced microvascular rarefaction in the mouse hippocampus and retrosplenial cortex: implications for cerebromicrovascular and brain aging. Age (Dordr.) 38, 273–289. doi: 10.1007/s11357-016-9931-0
Tarantini, S., Valcarcel-Ares, N. M., Yabluchanskiy, A., Springo, Z., Fulop, G. A., Ashpole, N., et al. (2017). Insulin-like growth factor 1 deficiency exacerbates hypertension-induced cerebral microhemorrhages in mice, mimicking the aging phenotype. Aging Cell 16, 469–479. doi: 10.1111/acel.12583
Terasaki, Y., Liu, Y., Hayakawa, K., Pham, L. D., Lo, E. H., Ji, X., et al. (2014). Mechanisms of neurovascular dysfunction in acute ischemic brain. Curr. Med. Chem. 21, 2035–2042. doi: 10.2174/0929867321666131228223400
Thorn, L. M., Shams, S., Gordin, D., Liebkind, R., Forsblom, C., Summanen, P., et al. (2019). Clinical and MRI features of cerebral small-vessel disease in type 1 diabetes. Diabetes Care 42, 327–330. doi: 10.2337/dc18-1302
Toth, L., Czigler, A., Hegedus, E., Komaromy, H., Amrein, K., Czeiter, E., et al. (2022). Age-related decline in circulating IGF-1 associates with impaired neurovascular coupling responses in older adults. Geroscience 44, 2771–2783. doi: 10.1007/s11357-022-00623-2
Toth, P., Tarantini, S., Ashpole, N. M., Tucsek, Z., Milne, G. L., Valcarcel-Ares, N. M., et al. (2015a). IGF-1 deficiency impairs neurovascular coupling in mice: implications for cerebromicrovascular aging. Aging Cell 14, 1034–1044. doi: 10.1111/acel.12372
Toth, P., Tarantini, S., Springo, Z., Tucsek, Z., Gautam, T., Giles, C. B., et al. (2015b). Aging exacerbates hypertension-induced cerebral microhemorrhages in mice: role of resveratrol treatment in vasoprotection. Aging Cell 14, 400–408. doi: 10.1111/acel.12315
Toth, P., Tucsek, Z., Tarantini, S., Sosnowska, D., Gautam, T., Mitschelen, M., et al. (2014). IGF-1 deficiency impairs cerebral myogenic autoregulation in hypertensive mice. J. Cereb. Blood Flow Metab. 34, 1887–1897. doi: 10.1038/jcbfm.2014.156
Tuttolomondo, A., Casuccio, A., Guercio, G., Maida, C., del Cuore, A., di Raimondo, D., et al. (2017). Arterial stiffness, endothelial and cognitive function in subjects with type 2 diabetes in accordance with absence or presence of diabetic foot syndrome. Cardiovasc. Diabetol. 16:2. doi: 10.1186/s12933-016-0483-5
Tuttolomondo, A., di Raimondo, D., Pecoraro, R., Maida, C., Arnao, V., Corte, V. D., et al. (2016). Early high-dosage atorvastatin treatment improved serum immune-inflammatory markers and functional outcome in acute ischemic strokes classified as large artery atherosclerotic stroke. Medicine (Baltimore) 95:e3186. doi: 10.1097/md.0000000000003186
Ungvari, Z., and Csiszar, A. (2012). The emerging role of IGF-1 deficiency in cardiovascular aging: recent advances. J. Gerontol. A Biol. Sci. Med. Sci. 67, 599–610. doi: 10.1093/gerona/gls072
Vidal, J. S., Hanon, O., Funalot, B., Brunel, N., Viollet, C., Rigaud, A. S., et al. (2016). Low serum insulin-like growth factor-I predicts cognitive decline in Alzheimer’s disease. J. Alzheimers Dis. 52, 641–649. doi: 10.3233/jad-151162
Wardlaw, J. M., Smith, C., and Dichgans, M. (2019). Small vessel disease: mechanisms and clinical implications. Lancet Neurol. 18, 684–696. doi: 10.1016/s1474-4422(19)30079-1
Weekman, E. M., and Wilcock, D. M. (2016). Matrix metalloproteinase in blood-brain barrier breakdown in dementia. J. Alzheimers Dis. 49, 893–903. doi: 10.3233/jad-150759
Westwood, A. J., Beiser, A., DeCarli, C., Harris, T. B., Chen, T. C., He, X. M., et al. (2014). Insulin-like growth factor-1 and risk of Alzheimer dementia and brain atrophy. Neurology 82, 1613–1619. doi: 10.1212/wnl.0000000000000382
Wrigley, S., Arafa, D., and Tropea, D. (2017). Insulin-like growth factor 1: at the crossroads of brain development and aging. Front. Cell. Neurosci. 11:14. doi: 10.3389/fncel.2017.00014
Yang, Q., Wei, X., Deng, B., Chang, Z., Jin, D., Huang, Y., et al. (2022). Cerebral small vessel disease alters neurovascular unit regulation of microcirculation integrity involved in vascular cognitive impairment. Neurobiol. Dis. 170:105750. doi: 10.1016/j.nbd.2022.105750
Zeger, M., Popken, G., Zhang, J., Xuan, S., Lu, Q. R., Schwab, M. H., et al. (2007). Insulin-like growth factor type 1 receptor signaling in the cells of oligodendrocyte lineage is required for normal in vivo oligodendrocyte development and myelination. Glia 55, 400–411. doi: 10.1002/glia.20469
Zhang, J., Liu, M., Huang, M., Chen, M., Zhang, D., Luo, L., et al. (2019). Ginsenoside F1 promotes angiogenesis by activating the IGF-1/IGF1R pathway. Pharmacol. Res. 144, 292–305. doi: 10.1016/j.phrs.2019.04.021
Zhang, W., Wang, W., and Kuang, L. (2018). The relation between insulin-like growth factor 1 levels and risk of depression in ischemic stroke. Int. J. Geriatr. Psychiatry 33, e228–e233. doi: 10.1002/gps.4774
Zhu, W., Fan, Y., Frenzel, T., Gasmi, M., Bartus, R. T., Young, W. L., et al. (2008). Insulin growth factor-1 gene transfer enhances neurovascular remodeling and improves long-term stroke outcome in mice. Stroke 39, 1254–1261. doi: 10.1161/strokeaha.107.500801
Keywords: insulin-like growth factor-1, cerebral small vessel disease, neurovascular unit, blood–brain barrier, microvascular rarefaction
Citation: Du H, Xia J, Huang L, Zheng L, Gu W and Yi F (2023) Relationship between insulin-like growth factor-1 and cerebral small vessel disease and its mechanisms: advances in the field. Front. Aging Neurosci. 15:1190869. doi: 10.3389/fnagi.2023.1190869
Edited by:
Luigi Puglielli, University of Wisconsin-Madison, United StatesReviewed by:
Antonino Tuttolomondo, Università degli Studi di Palermo, ItalyAri Dienel, University of Texas Health Science Center at Houston, United States
Krisztina Valter, Australian National University, Australia
Copyright © 2023 Du, Xia, Huang, Zheng, Gu and Yi. This is an open-access article distributed under the terms of the Creative Commons Attribution License (CC BY). The use, distribution or reproduction in other forums is permitted, provided the original author(s) and the copyright owner(s) are credited and that the original publication in this journal is cited, in accordance with accepted academic practice. No use, distribution or reproduction is permitted which does not comply with these terms.
*Correspondence: Wenping Gu, GWping393@csu.edu.cn; Fang Yi, yifang_xy@126.com