- 1Department of Neurology, The First Affiliated Hospital of Xi’an Jiaotong University, Xi’an, China
- 2Department of Neurology, Yulin Hospital of Traditional Chinese Medicine, Shaanxi, China
- 3Huyi Hospital of Traditional Chinese Medicine, Xi’an, China
Objectives: Amyloid-β (Aβ) deposition in the brain is the hallmark of Alzheimer’s disease (AD) pathology. Hypertension is a risk factor for AD, but the effects of hypertension on Aβ deposition are not fully determined. Considering peripheral Aβ closely relates to Aβ deposition in the brain, we investigated the relationships between blood pressure (BP) level and plasma Aβ concentrations.
Methods: One-thousand and sixty-nine participants (age above 45) from a village in the suburbs of Xi’an, China were enrolled. Questionnaires and validated Chinese versions of the Mini-Mental State Examination (MMSE) were used to collect information about vascular risk factors and assess cognition function. The apolipoprotein E (ApoE) genotype was detected using PCR and sequencing. Plasma Aβ levels were measured using ELISA. The associations between BP and plasma Aβ levels were analyzed by using multivariate linear regression.
Results: Plasma Aβ1–40 level was higher in high BP group than that in normal BP group (53.34 ± 8.50 pg/ml vs. 51.98 ± 8.96 pg/ml, P = 0.013), in high SBP group than that in normal SBP group (53.68 ± 8.69 pg/ml vs. 51.88 ± 8.80 pg/ml, P = 0.001) and in high MABP group than that in normal MABP group (54.05 ± 8.78 pg/ml vs. 52.04 ± 8.75 pg/ml, P = 0.001). After controlling for the confounding factors, SBP (b = 0.078, P < 0.001), DBP (b = 0.090, P = 0.008) and MABP (b = 0.104, P < 0.001) correlated with plasma Aβ1–40 level positively in ApoE ε4 non-carriers, but not ApoE ε4 carriers.
Conclusions: Elevated BP levels were associated with increased plasma Aβ1–40 levels in middle-aged and elderly ApoE ε4 non-carriers.
Introduction
Alzheimer’s disease (AD) is the most common cause of dementia, affecting more than 33.9 million people worldwide (Barnes and Yaffe, 2011). The deposition of amyloid-β (Aβ) in the brain is the main pathological characteristic of AD (Karran et al., 2011), and the amyloid cascade hypothesis is widely considered to underlie the pathogenesis of AD (Karran et al., 2011). Studies have found that elevated blood pressure (BP) levels in midlife may be related to the development and progression of AD in later life (Kivipelto et al., 2001; Qiu et al., 2005; Gottesman et al., 2017; Walker et al., 2019). According to recent studies, hypertension is associated with an increased Aβ burden in the brain (Ingmar and Deborah, 2013). Based on accumulating evidence, elevated BP may impair the clearance of Aβ and increase Aβ production in both the peripheral circulation and the central nervous system (Faraco and Iadecola, 2013).
Plasma Aβ, the source of which is mainly brain efflux via low-density lipoprotein receptor-related protein-1 (LRP1) through the blood-brain barrier (BBB) or glymphatic system (Roberts et al., 2014), is closely related to brain Aβ deposition (Vergallo et al., 2019). A complex equilibrium is believed to exist between the amyloid burden in the brain and plasma Aβ levels in both animal models and healthy individuals (DeMattos et al., 2002b; Giedraitis et al., 2007). The continuous translocation of Aβ from the brain parenchyma to the peripheral blood is essential for preventing Aβ accumulation and reducing the Aβ burden in the brain (DeMattos et al., 2001; Matsuoka et al., 2003). Plasma Aβ levels were recently reported to be associated with the incidence of AD (Ertekin-Taner et al., 2008; Lambert et al., 2009; Pérez-Grijalba et al., 2019). However, the relationship between the blood pressure (BP) level and plasma Aβ level is currently unclear. In the present study, we investigated the relationships between the parameters of BP and plasma Aβ levels in middle-aged and older individuals in the general population.
Materials and Methods
Study Population
This is an ongoing population-based study designed to determine the potential vascular factors for AD in the general population. We used the cluster sampling method from October 2014 to March 2015 to make a face-to-face questionnaire survey on all the permanent residents in Qubao village, Huyi District, Xi’an City, and conducted a household survey on the disabled. The lifestyle and population composition of the village are similar to other rural areas in Xi’an. This cluster sampling method is consistent with statistical rules and has been proved to be reliable in our previous publications (Wang et al., 2018). The inclusion criteria were the following: (1) age 45 years or older, (2) registered permanent resident living in Qubao Village for more than 3 years, and (3) consented to participate in the study. The exclusion criteria were the following: (1) individuals who suffered from severe kidney disease, cancer, chronic liver conditions or a severe heart, pulmonary, or hematological disease, (2) individuals taking anti-hypertensive medicine, and (3) had missing covariates, or at least one aberrant plasma Aβ1–40 or Aβ1–42 level. The flow chart of study is shown in Figure 1.
Among the 1, 699 residents living in the village and older than 45 years, 227 were taking anti-hypertension medicine, 329 had missing covariates, and 74 had at least one aberrant plasma Aβ1–40 or Aβ1–42 level (exceeding ± 3 SDs from the mean). After all the exclusions, a final count of 1, 069 subjects was included in the study. The protocols used were reviewed and approved by the Ethics Committee of the First Affiliated Hospital of Xi’an Jiaotong University (No: XJTU1AF2014LSK-111).
Data Collection
Subjects completed standardized questionnaires of general information to collect demographic data (age, sex, and education levels) and lifestyle habits (alcohol abuse, self-reported smoking history as current/former/never, and physical activity level) and underwent tests to determine the levels of multiple laboratory markers. We also recorded the medical history of cardiovascular disease, and transient ischaemic attack (TIA) or stroke. Additionally, we measured height, weight, BMI {which was calculated as [weight (kg)]/[height (m)2]}, and the pulse rate. The following vascular risk factors were measured: hypertension (defined as a mean systolic blood pressure measurement ≥140 mm Hg or diastolic blood pressure ≥ 90 mm Hg, a self-reported medical diagnosis, or use of antihypertensive drug therapy), diabetes (fasting serum glucose level ≥7.0 mmol/L, or use of diabetic medication or insulin.), and hyperlipidemia (fasting serum cholesterol concentration >5.18 mmol/L, serum triglyceride concentration >1.70 mmol/L, serum LDL cholesterol concentration >3.37 mmol/L, serum HDL cholesterol concentration <1.04 mmol/L, a self-reported medical diagnosis, or use of medication). Laboratory test parameters were measured in the clinical laboratory of The First Affiliated Hospital of Xi’an Jiaotong University.
Cognitive Evaluation
The Mini-Mental State Examination (MMSE) was used to assess global cognition (Katzman et al., 1988) in a quiet room. Examiners underwent standardized training prior to the study, and consistency between the examiners was evaluated in a pilot study (kappa: 0.76–1). We chose an MMSE score lower than the cut-off value set by Katzman et al. (1988) as the criterion for cognitive impairment; specifically, the cut-off value was ≤17 for the uneducated, ≤20 for individuals with primary school education, and ≤24 for individuals educated at the junior high school level or above.
BP Measurements
Blood pressure measurements were obtained during the inclusion interview for this study. Two brachial blood pressure measurements were recorded twice in a seated position after subjects had rested for at least 10 min. The instruments were a manual mercury sphygmomanometer with an appropriate-sized cuff (Shanghai Medical Instruments Co. Shanghai, China). Korotkoff phases 1 and 5 established the levels of systolic blood pressure (SBP) and diastolic blood pressure (DBP), respectively. The average of two measurements was used for analysis. The mean arterial pressure (MABP) was defined as [(SBP + DBP)/3].
Four variables, BP, SBP, DBP, and MABP, were used as indicators of the blood pressure level. A high BP was defined as a mean SBP ≥140 mm Hg or DBP ≥ 90 mm Hg. A high SBP was defined as a mean SBP ≥140 mm Hg. A high DBP was defined as a mean DBP ≥ 90 mm Hg. A high MABP was defined as a mean MABP ≥ 105 mm Hg.
Detection of Plasma β-Amyloid Levels
Fasting blood samples (8:00–9:00 AM) were collected into vacutainers containing EDTA, an anticoagulant, centrifuged at 3000 g for 10 min, and stored at -80°C until use. Plasma Aβ1–40 and Aβ1–42 levels were measured with sandwich enzyme-linked immunosorbent assay kits (ELISA, Yuanye Co., Shanghai, China) as previously described (Wang et al., 2018). Measurements were performed by professionals in an independent laboratory of neurology. All measures were conducted under standardized conditions. The Aβ1–42 assay does not cross-react with Aβ1–40, and the Aβ1–40 assay does not cross-react with Aβ1–42. The recovery of the Aβ1–42 assay ranges from 75% to 106%, with an average value of 92%. The recovery of the Aβ1–40 assay ranges from 78% to 105%, with an average value of 90%. Measurements by recording the absorbance at 450 nm on a RT-6000 analyzer (Rayto Co., Shenzhen, China), and then concentrations were calculated using the standard curve. The limit of detection for each assay was 1.0 pg/ml. All samples were measured in duplicate and the results were averaged. The intra-assay and inter-assay coefficients of variation were less than 7% and 9%, respectively.
Apolipoprotein E Genotyping
Polymerase chain reaction (PCR) was used to amplify the target gene fragment and the ApoE genotype was determined by one generation sequencing in 961 participants. ApoE genotype was defined based on the number of ε4 alleles. Based on the ApoE genotype, the participants were classified into the ApoE ε4 (−) group (E2/2, E2/3, and E3/3) and the ApoE ε4 (+) group (E2/4, E3/4, and E4/4).
Data Analysis
All of the data were analyzed with SPSS 22.0 software. All graphs were drawn using GraphPad Prism software version 5.0. Quantitative variables are reported as the means ± SD or medians (interquartile ranges), and qualitative variables are reported as numbers (percentages). Unpaired Student’s t-tests were used to analyses data with an approximately normal distribution, the Mann-Whitney U-test was used to compare data with skewed distributions, and the c2 or Fisher’s exact test was used for categorical data. However, plasma triglyceride and fasting blood glucose (FBG) levels were log transformed prior to analysis, as they displayed skewed distributions. Then, unpaired Student’s t-tests were used to compare the differences in plasma Aβ levels in the subgroups stratified by BP parameters. Partial correlation coefficients and multivariate linear regression models were used to evaluate the associations between BP levels and Aβ levels after adjusting for the confounding factors. Model 1 was adjusted for age and sex, and model 2 was additionally adjusted for the BMI, pulse rate, ApoEε4 carrier status, log-transformed fasting blood glucose level, log-transformed triglyceride level, total cholesterol level, high-density lipoprotein level, smoking status, drinking status, physical activity level, stroke, transient ischaemic attack, and heart disease. The linear correlation and regression analyses were performed to explore the potential effect of ApoE genotype on the relationships. Potential confounders identified in previous studies that might affect BP and plasma Aβ levels were considered. A P value of <0.05 (two-tailed) was considered statistically significant.
Results
Characteristics of the Study Population
The characteristics of the study population are presented in Table 1. The high BP group was older and included a higher proportion of individuals with diabetes, dyslipidemia, and a lack of physical activity. This group also presented higher values for the BMI, pulse rate, fasting blood glucose level, and serum cholesterol and triglyceride concentrations. A significantly lower MMSE score was recorded by the high BP group, but the ApoE ε4 allele carrier status was not different between the high BP group and the normal BP group.
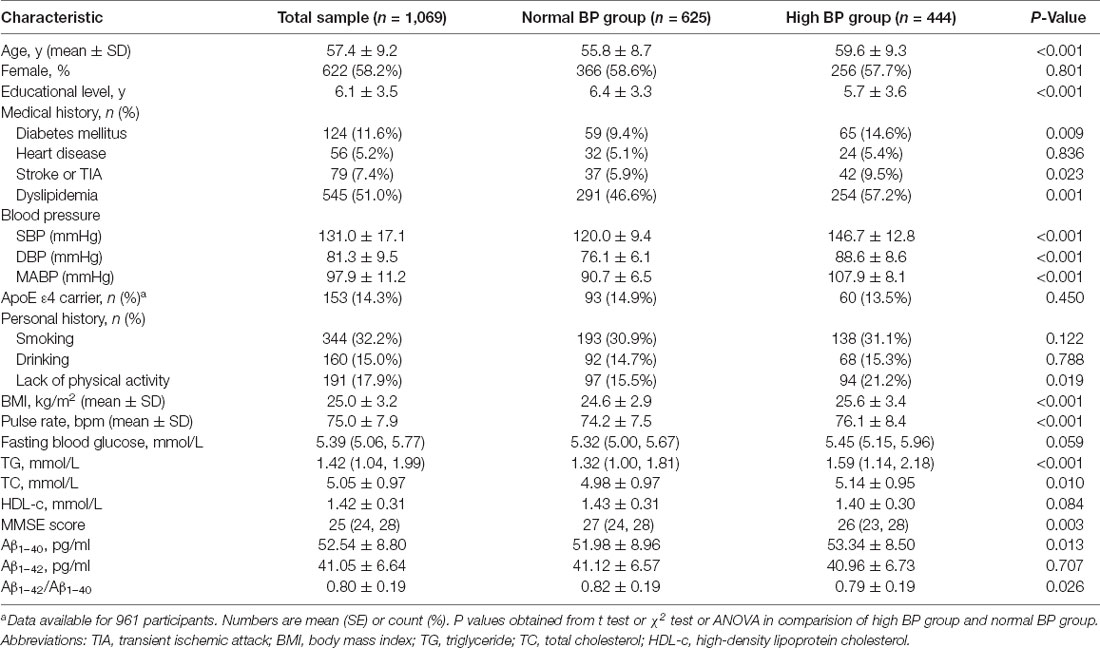
Table 1. Characteristics of the total study population and the population stratified by blood pressure.
Comparison of Plasma Aβ Levels Between the High BP Group and Normal BP Group
As shown in Table 1 and Figure 2; plasma Aβ1–40 level was higher in the high BP group than that in the normal BP group (53.34 ± 8.50 pg/ml vs. 51.98 ± 8.96 pg/ml, P = 0.013), while the Aβ1–42/Aβ1–40 ratio was significantly lower (0.79 ± 0.19 vs. 0.82 ± 0.19, P = 0.026), but the Aβ1–42 level had no significant difference between the high BP group and normal BP group (P = 0.707). Furthermore, plasma Aβ1–40 level was higher in the high SBP group (53.68 ± 8.69 pmol/L vs. 51.88 ± 8.80 pmol/L, P = 0.001) and high MABP group (54.05 ± 8.78 pmol/L vs. 52.04 ± 8.75 pmol/L, P = 0.001), but Aβ1–42/Aβ1–40 ratio was lower in the high SBP (0.79 ± 0.19 vs. 0.81 ± 0.19, P = 0.038), high DBP (0.78 ± 0.19 vs. 0.81 ± 0.19, P = 0.023), and high MABP (0.78 ± 0.20 vs. 0.81 ± 0.19, P = 0.009) groups.
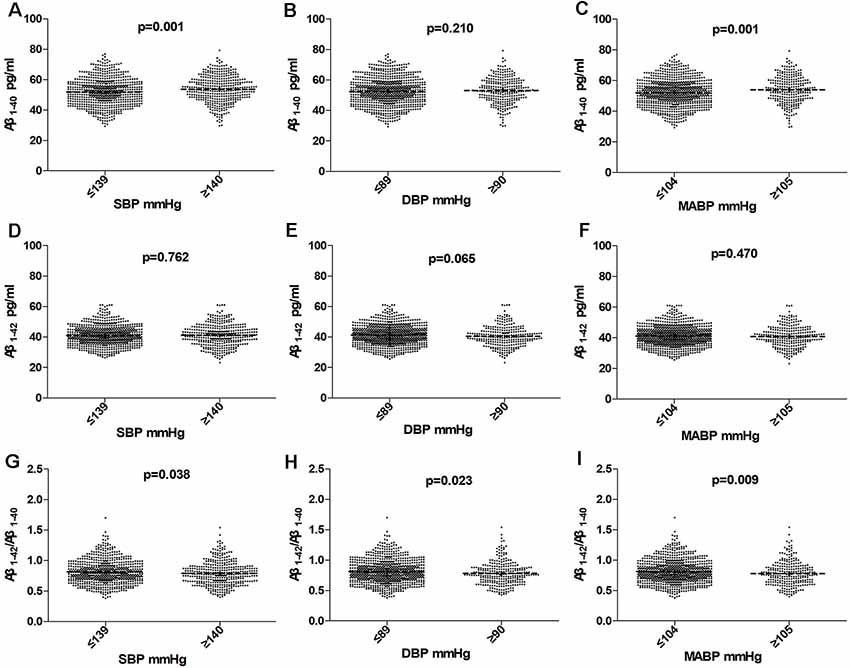
Figure 2. Comparision of Aβ1–40, Aβ1–42, and Aβ1–42/Aβ1–40 in subjects according to SBP (A,D,G), DBP (B,E,H), MABP (C,F,I) in the total population. Horizontal lines represent the mean and 95% CI. Abbreviations: BP, blood pressure; SBP, systolic blood pressure; DBP, diastolic blood pressure; MABP, mean arterial blood pressure.
The Association Between BP and Plasma Aβ Levels in the Multivariate Analysis
In the total population, the relationship between BP and plasma Aβ levels was estimated using partial correlation analyses and multivariate regression analyses. As shown in Figure 3 and Table 2; plasma Aβ1–40 levels correlated with SBP (r = 0.106, β = 0.056, P = 0.001) and MABP (r = 0.082, β = 0.065, P = 0.008) positively after controlling for age and sex. After additionally controlling for all the confounding factors listed in Table 2, plasma Aβ1–40 levels were positively correlated with SBP (r = 0.122, β = 0.068, P < 0.001), DBP (r = 0.072, β = 0.071, P = 0.019) and MABP (r = 0.104, β=0.087, P = 0.001). The Aβ1–42/Aβ1–40 ratio was negatively correlated with SBP (r = −0.068, β = −0.001, P = 0.027), DBP (r = −0.063, β = −0.001, P = 0.040), and MABP (r = −0.071, β = −0.001, P = 0.021).
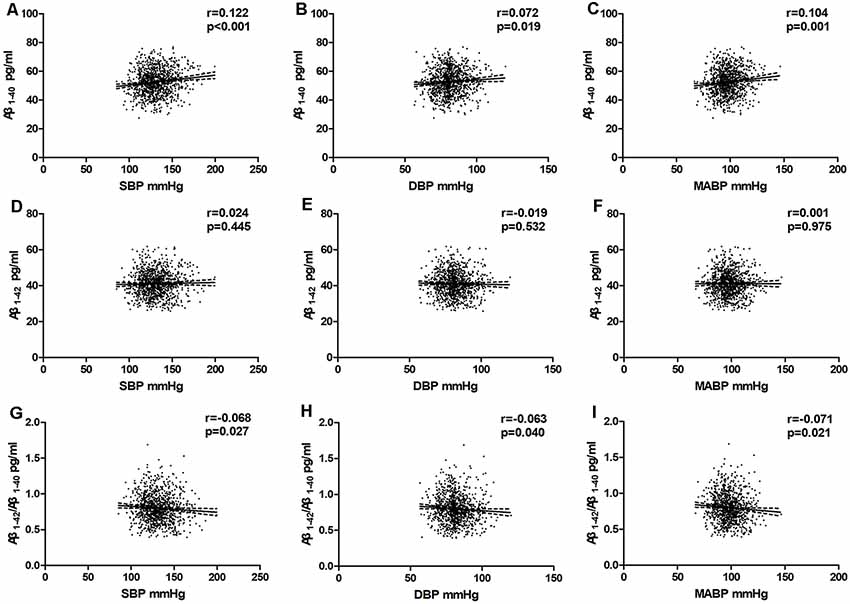
Figure 3. Partial linear correlations between plasma Aβ and SBP, DBP, MABP in total participants. Partial linear correlations of blood pressure (BP) with plasma Aβ1–40 levels (A–C) and plasma Aβ1–42 levels (D–F) and Aβ1–42/Aβ1–40 (G–I) are shown in the figure. Partial correlation coefficients and P values were obtained after adjustment for age, sex, BMI, pulse rate, log-transformed fasting blood glucose, log-transformed TG, TC, HDL-C, smoking, drinking, physical activity level, stroke, transient ischaemic attack (TIA), and heart disease.
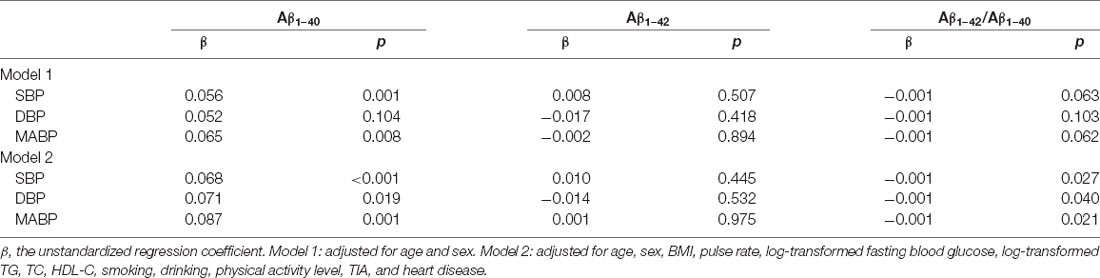
Table 2. Multiple linear regression models analysis of blood pressure components and plasma Aβ1–40, Aβ1–42, and Aβ1–42/Aβ1–40 ratio in total study subjects.
The Effects of the ApoE ε4 Allele on Plasma Aβ Levels
As ApoE ε4 is the strongest genetic risk factor for AD, we investigated the effects of the ApoE ε4 allele on plasma Aβ levels. In the univariate analysis, ApoE ε4 non-carriers had a lower proportion of females than ApoE ε4 carriers, however other covariates (age, gender, MMSE score, education level, smoking status, drinking status, intensity of physical activity, hypertension, diabetes mellitus, coronary heart disease, MABP, and BMI) had no significant difference between the two groups. The plasma Aβ1–40 and Aβ1–42 concentrations and the Aβ1–42/Aβ1–40 ratio had no significant difference between ApoE ε4 non-carriers and ApoE ε4 carriers (Table 3).
Multivariate Analysis of the Relationship Between BP and Plasma Aβ Levels in Individuals Stratified According to the ApoE ε4 Status
The multivariate linear regression analyses and partial correlation analyses were performed in subgroups stratified according to ApoE ε4 status. In ApoE ε4 non-carriers, after controlling for confounders, plasma Aβ1–40 levels correlated with SBP, DBP and MABP positively (rSBP = 0.143, βSBP = 0.078, P < 0.001; rDBP = 0.093, βDBP = 0.090, P = 0.008; and rMABP = 0.126 βMABP = 0.104, P < 0.001, respectively); the Aβ1–42/Aβ1–40 ratio correlated with SBP, DBP, and MABP negatively (rSBP = −0.072, βSBP = −0.001, P = 0.043; rDBP = −0.066, βDBP = −0.001, P = 0.063; and rMABP = −0.074, βMABP = −0.001, P = 0.037, respectively). However, among ApoE ε4 carriers, these relationships disappeared. These suggested that the association between BP and plasma Aβ levels may depend on the ApoE ε4 status (Table 4).
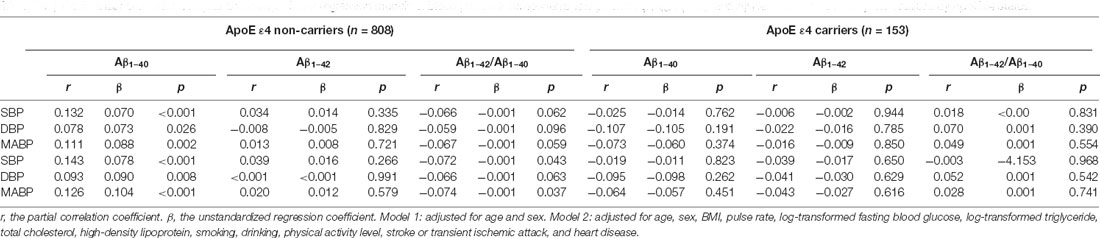
Table 4. Partial linear correlation analysis and multiple linear regression models of blood pressure components and plasma Aβ1–40, Aβ1–42, and Aβ1–42/Aβ1–40 ratio in subjects stratified by ApoE ε4 status.
Discussion
In this cross-sectional study, we investigated the relationships between multiple BP components and plasma Aβ levels and found that elevated BP levels were associated with increased plasma Aβ1–40 levels and decreased Aβ1–42/Aβ1–40 ratio in middle-aged and older villagers, even after controlling for other confounding factors. However, the association was only observed in ApoE ε4 non-carriers, but not ApoE ε4 carriers.
Several publications have suggested an association between plasma Aβ and BP levels (Fujiwaraa et al., 2003; Abdullah et al., 2009; Lambert et al., 2012; Ruiz et al., 2013; Wang et al., 2018). However, a consistent conclusion has not been drawn. A positive correlation between SBP and plasma Aβ1–40 levels (Abdullah et al., 2009; Lambert et al., 2012) or a negative correlation between SBP and plasma Aβ1–40 levels (Abdullah et al., 2009), as well as a positive correlation between DBP and plasma Aβ1–42 levels (Fujiwaraa et al., 2003) have been reported. These inconsistencies are likely due to the use of different inclusion criteria, exclusion criteria, and test methods. In those cross-sectional studies, investigators either used a case-control study design with a small sample size (Lambert et al., 2012; Ruiz et al., 2013) or only explored plasma Aβ1–42 and BP levels (Fujiwaraa et al., 2003).
Unlike the previous studies, our present study used a random cluster sampling method with a large sample size consisting of middle-aged and elderly individuals in the general population. All enrolled residents lived in the selected village for over 3 years with permanent residency. Plasma Aβ levels were detected using ELISA, which has been demonstrated as an accurate and dependable method (Katzman et al., 1988). We did a multiple analysis to adjusted for almost all identified potential confounder factors, including the ApoE genotype (Rodrigue et al., 2013; Giau et al., 2015), BMI (Qiu et al., 2005), MMSE score, and serum TC, TG and HDL-c levels (Matsuzaki et al., 2011). The relationships between plasma Aβ level and BP levels did not change. These results were similar to the three-city study by Lambert et al. (2012) which showed that elevated BP levels were associated with decreased plasma Aβ1–42/Aβ1–40 ratio.
The mechanism underlying the association between plasma Aβ and BP levels is not well understood. One possible mechanism is that BP may affect the deposition of Aβ in the brain. It has been reported that hypertension is associated with Aβ deposition in the brain in individuals with normal cognition. Animal experiments also observed a direct effect of hypertension on the deposition of Aβ in the brain (Cifuentes et al., 2015; Faraco et al., 2016). In PET imaging studies of middle-aged to old adults with normal cognition, Langbaum et al. revealed an association between elevated SBP and fibrillary Aβ levels in the brain (Langbaum et al., 2012). In addition, Rodrigue et al. (2013) observed a significant correlation between controlled hypertension and lower brain Aβ burden compared with un-medicated hypertension (Rodrigue et al., 2013). Aβ is generated primarily in the brain and cleared mainly through the blood-brain barrier from the central to the peripheral. Aβ levels are in dynamic equilibrium between CSF and plasma (DeMattos et al., 2002b). An increased efflux from the brain to plasma may result in higher plasma Aβ levels (DeMattos et al., 2002a). Thus, an elevated BP associated with higher plasma Aβ levels might relate to increased Aβ generation in the brains of individuals with normal cognition. And our previous studies showed that plasma Aβ40 levels positively correlated with sRAGE and sLRP1 (Gao et al., 2018), while BP positively correlated with Aβ40 and negatively correlated with sRAGE (Jiang et al., 2017). Therefore we speculate that BP changes the balance between peripheral and central Aβ affecting the levels of plasma Aβ40 and peripheral transporters. Further, it might have affected the peripheral clearance pathway of Aβ, increasing the deposition of Aβ in the brain and participating in the development of Aβ pathological process. These conjectures have not been confirmed and should be verified in experiments performed in appropriate animal models.
Another possible mechanism is that elevated BP may have affected the integrity of the BBB, increasing the Aβ transport. Research shows that hypertension damages the integrity of vascular endothelial cells and affects the vascular wall (Faraco and Iadecola, 2013). It has been hypothesized (Shah et al., 2012) that the vasoactivity of Aβ in combination with hypertension could hardly destroy the integrity of the vascular wall and reduce the clearance of Aβ in the brain which would lead to an inflammatory response and cell death. Despite all of this, the authors suggested that vascular integrity was an important part of the early trajectory. Importantly, blood pressure and plasma Aβ levels were measured 10–20 years before the diagnosis of AD, which indicates that early intervention on elevated BP might be very important for reducing AD caused by hypertension. This conclusion is also suggested by our results. Moreover, the elevated BP might impair the vascular clearance of Aβ and increase its cleavage from APP in both peripheral and the central nervous system to further facilitate the onset of AD (Ingmar and Deborah, 2013).
We did not find an association between BP and plasma Aβ1–42 levels, which might be due to the different physiological roles of Aβ1–40 and Aβ1–42. Aβ1–42 is insoluble and prone to fibrillate and deposit in senile plaques with greater neurotoxicity (Verbeek et al., 1997). While, Aβ1–40 is soluble, and has direct physiological or toxic effects on the blood vessel wall (Niwa et al., 2000). The levels of Aβ1–40, but not Aβ1–42, are markedly increased in patients with ischemic stroke (Lee et al., 2005) and diffuse SVD (Gomis et al., 2009). These indicated that Aβ1–40 is more closely relate to vascular disease than Aβ1–42.
In the subgroup analysis stratified according to the ApoE ε4 status, the association between plasma Aβ and BP levels were only observed in ApoE ε4 non-carriers, but not in ApoE ε4 carriers. The underlying mechanism is not clear. As the strongest genetic risk factor for AD, the ApoE ε4 allele was closely associated with the decrease of cerebral spinal flow Aβ and the increase of aggregation and deposition of cerebral Aβ in the brain (Liu et al., 2013; Giau et al., 2015). ApoE ε4 might compromise the effects of BP on plasma Aβ levels. Recent articles reported that the association between plasma Aβ levels and Aβ deposition in the brain was exactly observed in ApoE ε4 non-carriers (Swaminathan et al., 2014; Tateno et al., 2017). Additionally, Katsuya et al. reported that the prevalence of hypertension is lower in ApoE ε4 carriers (Katsuya et al., 2002). These indicated that the relationship between BP and plasma Aβ levels is dependent on ApoE ε4 states.
In this study, we measured plasma Aβ levels using an ELISA kit marketed by Yuanye Co (China). Compared to Aβ levels mentioned in other publications, the Aβ42 levels we determined are higher. We speculate this discrepancy might be due to the heterogeneity of the different populations studied and the measurement methods used and standards of Aβ that have not been validated to each other. Compared to the INNO-BIA assay, the ELISA Aβ40 levels measures are slightly lower, while the Aβ42 levels are slightly higher (Barnes and Yaffe, 2011). Our previous studies using the same ELISA kit produced credible results (Jiang et al., 2017; Gao et al., 2018; Wang et al., 2018); however, we acknowledge more rigorous data are needed to validate the comparisons.
Conclusion
In summary, in this population-based cross-sectional study, we found that elevated BP levels were associated with increased plasma Aβ1–40 levels and decreased Aβ1–42/Aβ1–40 ratio in middle-aged and elderly villagers, particularly in ApoE ε4 non-carriers. Considering peripheral Aβ closely related to the deposition of Aβ in the brain, these results indicated that hypertension contribution to AD may be associated with peripheral Aβ transport dysfunction. The potential mechanism requires further validation.
Limitations
First, in this cross-sectional study, BP and plasma Aβ levels were measured only once at a single time point, which is susceptible to physiological bias. Second, the cross-sectional design makes it difficult to determine causal relationships between plasma Aβ and BP levels. It is thus essential to conduct prospective cohort studies to identify the effects of BP on plasma Aβ levels. Third, we did not measure the levels of Aβ in the CSF and the deposition of Aβ in the brain simultaneously. Therefore, the increase in plasma Aβ levels may not indicate an increase of Aβ burden in the brain.
Data Availability Statement
The raw data supporting the conclusions of this article will be made available by the authors, without undue reservation.
Ethics Statement
The studies involving human participants were reviewed and approved by the Ethics Committee of the First Affiliated Hospital of Xi’an Jiaotong University (No: XJTU1AF2014LSK-111). The patients/participants provided their written informed consent to participate in this study.
Author Contributions
MS and SS conceived the study, participated in its design, and wrote the manuscript. NH, CC, LD, LG, SW, JinW, KH, and JingW analyzed the data. QQ designed the study and revised the manuscript. All authors contributed to the article and approved the submitted version.
Funding
This study was supported by the Natural Science Foundation of China (No. 81771168), by the Key Research & Development Programs of Shaanxi Province (No. 2018ZDXM-SF-052), and by the clinical research award of the First Affiliated Hospital of Xi’an Jiaotong University (No. XJTU1AF-CRF-2018-004).
Conflict of Interest
The authors declare that the research was conducted in the absence of any commercial or financial relationships that could be construed as a potential conflict of interest.
Acknowledgments
We thank all study participants for their cooperation.
Abbreviations
BP, blood pressure; SBP, systolic blood pressure; DBP, diastolic blood pressure; MABP, mean arterial blood pressure.
References
Abdullah, L., Luis, C., Paris, D., Mouzon, B., Ait-Ghezala, G., Allen, E., et al. (2009). High serum Abeta and vascular risk factors in first-degree relatives of Alzheimer’s disease patients. Mol. Med. 15, 95–100. doi: 10.2119/molmed.2008.00118
Barnes, D. E., and Yaffe, K. (2011). The projected effect of risk factor reduction on Alzheimer’s disease prevalence. The Lancet Neurolol. 10, 819–828. doi: 10.1016/S1474-4422(11)70072-2
Cifuentes, D., Poittevin, M., Dere, E., Broquères-You, D., Bonnin, P., Benessiano, J., et al. (2015). Hypertension accelerates the progression of Alzheimer-like pathology in a mouse model of the disease. Hypertension 65, 218–224. doi: 10.1161/HYPERTENSIONAHA.114.04139
DeMattos, R. B., Bales, K. R., Cummins, D. J., Dodart, J. C., Paul, S. M., Holtzman, D. M., et al. (2001). Peripheral anti-A beta antibody alters CNS and plasma A beta clearance and decreases brain A beta burden in a mouse model of Alzheimer’s disease. Proc. Natl. Acad. Sci. U S A 98, 8850–8855. doi: 10.1073/pnas.151261398
DeMattos, R. B., Bales, K. R., Cummins, D. J., Paul, S. M., and Holtzman, D. M. (2002a). Brain to plasma amyloid-beta efflux: a measure of brain amyloid burden in a mouse model of Alzheimer’s disease. Science 295, 2264–2267. doi: 10.1126/science.1067568
DeMattos, R. B., Bales, K. R., Parsadanian, M., O’Dell, M. A., Foss, E. M., Paul, S. M., et al. (2002b). Plaque-associated disruption of CSF and plasma amyloid-beta (Abeta) equilibrium in a mouse model of Alzheimer’s disease. J. Neurochem. 81, 229–236. doi: 10.1046/j.1471-4159.2002.00889.x
Ertekin-Taner, N., Younkin, L. H., Yager, D. M., Parfitt, F., Baker, M. C., Asthana, S., et al. (2008). Plasma amyloid beta protein is elevated in late-onset Alzheimer disease families. Neurology 70, 596–606. doi: 10.1212/01.WNL.0000278386.00035.21
Faraco, G., and Iadecola, C. (2013). Hypertension: a harbinger of stroke and dementia. Hypertension 62, 810–817. doi: 10.1161/HYPERTENSIONAHA.113.01063
Faraco, G., Park, L., Zhou, P., Luo, W., Paul, S. M., Anrather, J., et al. (2016). Hypertension enhances Aβ-induced neurovascular dysfunction, promotes β-secretase activity and leads to amyloidogenic processing of APP. J. Cereb. Blood Flow Metab. 36, 241–252. doi: 10.1038/jcbfm.2015.79
Fujiwaraa, Y., Takahashib, M., Tanakac, M., Hoshid, T., Someyab, T., Shinkai, S., et al. (2003). Relationships between plasma β-amyloid peptide 1–42 and atherosclerotic risk factors in community-based older populations. Gerontology 49, 374–379. doi: 10.1159/000073765
Gao, L., Jiang, Y., Wei, S., Shang, S., Li, P., Chen, C., et al. (2018). The level of plasma amyloid-40 is correlated with peripheral transport proteins in cognitively normal adults: a population-based cross-sectional study. J. Alzheimers Dis. 65, 951–961. doi: 10.3233/JAD-180399
Giau, V. V., BagyinszkY, E., An, S. S., and Kim, S. Y. (2015). Role of apolipoprotein E in neurodegenerative diseases. Neuropsychiatr. Dis. Treat. 11, 1723–1737. doi: 10.2147/NDT.S84266
Giedraitis, V., Sundelof, J., Irizarry, M. C., Garevik, N., Hyman, B. T., Wahlund, L. O., et al. (2007). The normal equilibrium between CSF and plasma amyloid beta levels is disrupted in Alzheimer’s disease. Neurosci. Lett. 427, 127–131. doi: 10.1016/j.neulet.2007.09.023
Gomis, M., Sobrino, T., Ois, A., Milla’n, M., Rodríguez-Campello, A., Rodríguez-Gonza’lez, R., et al. (2009). Plasma beta-amyloid 1–40 is associated with the diffuse small vessel disease subtype. Stroke 40, 3197–3201. doi: 10.1161/STROKEAHA.109.559641
Gottesman, R. F., Schneider, A. L. C., Zhou, Y., Coresh, J., Green, E., Gupta, N., et al. (2017). Association between midlife vascular risk factors and estimated brain amyloid deposition. JAMA 317, 1443–1450. doi: 10.1001/jama.2017.3090
Ingmar, S., and Deborah, G. (2013). Update on hypertension and Alzheimer’s disease. Neurol. Res. 28, 605–611. doi: 10.1179/016164106X130506
Jiang, Y., Shang, S., Li, P., Chen, C., Dang, L., Wang, J., et al. (2017). Pulse pressure is associated with plasma amyloid-β transport dysfunction. J. Hypertension 36, 569–579. doi: 10.1097/HJH.0000000000001565
Karran, E., Mercken, M., and De Strooper, B. (2011). The amyloid cascade hypothesis for Alzheimer’s disease: an appraisal for the development of therapeutics. Nat. Rev. Drug Discov. 10, 698–712. doi: 10.1038/nrd3505
Katsuya, T., Baba, S., Ishikawa, K., Mannami, T., Fu, Y., Inamoto, N., et al. (2002). Epsilon 4 allele of apolipoprotein E gene associates with lower blood pressure in young Japanese subjects: the Suita Study. J. Hyperten. 20, 2017–2021. doi: 10.1097/00004872-200210000-00021
Katzman, R., Zhang, M., Ouang, Y., Liu, W., and Yu, E. (1988). A Chinese version of the MINI-mental state examination; impact of illiteracy in a Shanghai dementia survey. J. Clin. Epidemiol. 41, 971–978. doi: 10.1016/0895-4356(88)90034-0
Kivipelto, M., Helkala, E. L., Laakso, M. P., Hanninen, T., Hallikainen, M., Alhainen, K., et al. (2001). Midlife vascular risk factors and Alzheimer’s disease in later life: longitudinal, population based study. BMJ 322, 1447–1451. doi: 10.1136/bmj.322.7300.1447
Lambert, J. C., Dallongeville, J., Ellis, K. A., Schraen-Maschke, S., Lui, J., Laws, S., et al. (2012). Association of plasma Aß peptides with blood pressure in the elderly. PLoS One 6:e18536. doi: 10.1371/journal.pone.0018536
Lambert, J. C., Schraen-Maschke, S., Richard, F., Fievet, N., Rouaud, O., Berr, C., et al. (2009). Association of plasma amyloid β with risk of dementia: the prospective three-city study. Neurology 73, 847–853. doi: 10.1212/WNL.0b013e3181b78448
Langbaum, J. B., Chen, K., Launer, L. J., Fleisher, A. S., Lee, W., Liu, X., et al. (2012). Blood pressure is associated with higher brain amyloid burden and lower glucose metabolism in healthy late middle-age persons. Neurobiol. Aging 33:827. doi: 10.1016/j.neurobiolaging.2011.06.020
Lee, P. H., Bang, O. Y., Hwang, E. M., Lee, J. S., Joo, U. S., Mook-Jung, I., et al. (2005). Circulating beta amyloid protein is elevated in patients with acute ischemic stroke. J. Neural Transm. 112, 1371–1379. doi: 10.1007/s00702-004-0274-0
Liu, C. C., Liu, C. C., Kanekiyo, T., Xu, H., and Bu, G. (2013). Apolipoprotein E and Alzheimer disease: risk, mechanisms and therapy. Nat. Rev. Neurol. 9, 106–118. doi: 10.1038/nrneurol.2012.263
Matsuoka, Y., Saito, M., Lafrancois, J., Saito, M., Gaynor, K., Olm, V., et al. (2003). Novel therapeutic approach for the treatment of Alzheimer’s disease by peripheral administration of agents with an affinity to beta amyloid. J. Neurosci. 23, 29–33 doi: 10.1523/JNEUROSCI.23-01-00029.2003
Matsuzaki, T., Sasaki, K., and Hata, J. (2011). Association of Alzheimer disease pathology with abnormal lipid metabolism: the Hisayama study. Neurology 77, 1068–1075. doi: 10.1212/WNL.0b013e31822e145d
Niwa, K., Younkin, L., Ebeling, C., Turner, S. K., Westaway, D., Younkin, S., et al. (2000). Abeta 1–40-related reduction in functional hyperaemia in mouse neocortex during somatosensory activation. Proc. Natl. Acad. Sci. U S A 97, 9735–9740. doi: 10.1073/pnas.97.17.9735
Pérez-Grijalba, V., Arbizu, J., Romero, J., Prieto, E., Pesini, P., Sarasa, L., et al. (2019). Plasma Aβ42/40 ratio alone or combined with FDG-PET can accurately predict amyloid-PET positivity: a cross-sectional analysis from the AB255 Study. Alzheimers Res. Ther. 11:96. doi: 10.1186/s13195-019-0549-1
Qiu, C., Winblad, B., and Fratiglioni, L. (2005). The age-dependent relation of blood pressure to cognitive function and dementia. The Lancet Neurolol. 4, 487–499. doi: 10.1016/S1474-4422(05)70141-1
Roberts, K. F., Elbert, D. L., Kasten, T. P., Patterson, B. W., Sigurdson, W. C., Connors, R. E., et al. (2014). Amyloid-beta efflux from the central nervous system into the plasma. Ann. Neurol. 76, 837–844. doi: 10.1002/ana.24270
Rodrigue, K. M., Rieck, J. R., Kennedy, K. M., Devous, M. D., Diaz-Arrastia, R., Park, D. C., et al. (2013). Risk factors for β-amyloid deposition in healthy aging: vascular and genetic effects. JAMA Neurol. 70, 600–7606. doi: 10.1001/jamaneurol.2013.1342
Ruiz, A., Pesini, P., Espinosa, A., Pérez-Grijalba, V., Valero, S., Sotolongo-Grau, O., et al. (2013). Blood amyloid beta levels in healthy, mild cognitive impairment and Alzheimer’s disease individuals: replication of diastolic blood pressure correlations and analysis of critical covariates. PLoS One 8:e81334. doi: 10.1371/journal.pone.0081334
Shah, N. S., Vidal, J. S., Masaki, K., Petrovitch, H., Ross, G. W., Tilley, C., et al. (2012). Midlife blood pressure, plasma-amyloid and the risk for Alzheimer disease the honolulu asia aging study. Hypertension 59, 780–786. doi: 10.1161/HYPERTENSIONAHA.111.178962
Swaminathan, S., Risacher, S. L., Yoder, K. K., West, J. D., Shen, L., Kim, S., et al. (2014). Association of plasma and cortical amyloid beta is modulated by APOE epsilon4 status. Alzheimers Dement. 10, e9–e18. doi: 10.1016/j.jalz.2013.01.007
Tateno, A., Sakayori, T., Kim, W. C., Koeda, M., Kumita, S., Suzuki, H., et al. (2017). Effect of apolipoprotein E phenotype on the association of plasma amyloid beta and amyloid positron emission tomography imaging in Japan. Alzheimers Dement. 9, 51–56. doi: 10.1016/j.dadm.2017.08.002
Verbeek, M. M., Eikelenboom, P., and de Waal, R. M. (1997). Differences between the pathogenesis of senile plaques and congophilic angiopathy in Alzheimer disease. J. Neuropathol. Exp. Neurol. 56, 751–761. doi: 10.1097/00005072-199756070-00001
Vergallo, A., Mégret, L., Lista, S., Cavedo, E., Zetterberg, H., Blennow, K., et al. (2019). Plasma amyloid β 40/42 ratio predicts cerebral amyloidosis in cognitively normal individuals at risk for Alzheimer’s disease. Alzheimers Dement. 15, 764–775. doi: 10.1016/j.jalz.2019.03.009
Walker, K. A., Sharrett, A. R., Wu, A., Schneider, A. L. C., Albert, M., Lutsey, P. L., et al. (2019). Association of midlife to late-life blood pressure patterns with incident dementia. JAMA 322, 535–545. doi: 10.1001/jama.2019.10575
Keywords: Alzheimer’s disease, plasma β-amyloid level, blood pressure, apolipoprotein E, hypertension
Citation: She M, Shang S, Hu N, Chen C, Dang L, Gao L, Wei S, Huo K, Wang J, Wang J and Qu Q (2021) Blood Pressure Level Is Associated With Changes in Plasma Aβ1 –40 and Aβ1–42 Levels: A Cross-sectional Study Conducted in the Suburbs of Xi’an, China. Front. Aging Neurosci. 13:650679. doi: 10.3389/fnagi.2021.650679
Received: 07 January 2021; Accepted: 06 May 2021;
Published: 04 June 2021.
Edited by:
Nibaldo C. Inestrosa, Pontificia Universidad Católica de Chile, ChileReviewed by:
Colin Masters, University of Melbourne, AustraliaFernando Goni, New York University, United States
Copyright © 2021 She, Shang, Hu, Chen, Dang, Gao, Wei, Huo, Wang, Wang and Qu. This is an open-access article distributed under the terms of the Creative Commons Attribution License (CC BY). The use, distribution or reproduction in other forums is permitted, provided the original author(s) and the copyright owner(s) are credited and that the original publication in this journal is cited, in accordance with accepted academic practice. No use, distribution or reproduction is permitted which does not comply with these terms.
*Correspondence: Jin Wang, drwangjin@163.com; Qiumin Qu, quqiumin@xjtufh.edu.cn
† These authors have contributed equally to this work