- 1School of Biological and Food Processing Engineering, Huanghuai University, Zhumadian, China
- 2College of Animal Husbandry and Veterinary Science, Henan Agricultural University, Zhengzhou, China
Here, we report the genetic features and evolutionary mechanisms of two tet (M)-bearing plasmids (pTA2 and pTA7) recovered from swine Escherichia coli isolates. The genetic profiles of pTA2 and pTA7 and corresponding transconjugants were accessed by S1 nuclease pulsed-field gel electrophoresis and Southern hybridization, followed by whole genome sequencing and bioinformatics analysis. The biological influences of pTA2 and pTA7 were determined by stability and direct competition assays. Both pTA7 and pTA2 had the IncR backbone sequences but differed in the multidrug resistance region (MDR). The MDR of pTA2 consisted of sul3, tet (M), qnrS1, bleO, oqxAB, floR, aadA1, cmlA1, aadA2, and tet (A)-tetR (A) in addition to 22 insertion sequences. Notably, pTA2 carried the novel complex Tn7124 (IS26-ctp-lp-tet (M)-hp-IS406tnp-IntI4-IS26) harboring tet (M). The fragment carrying tet (M) (IS26-ctp-lp-tet (M)-IS406 tnp-ctp-aadA1-cmlA1-aadA2-dfrA12-IntI1), named Tn6942-like, and the two resistance modules ISVsa3-VirD2-floR-lysR and tet (A)-tetR (A) were located in the MDR of pTA7. Both pTA2 and pTA7 were highly stable in E. coli DH5α cells with no fitness cost to the host or disadvantage in growth competition. These results indicate that transposons carrying tet (M) continuously integrate via mediation with an insertion sequence, which accelerates the transmission of tet (M) in E. coli isolates through integration of other drug-resistant genes, thereby posing a potential serious threat to the efficacy of clinical treatment.
1 Introduction
The tetracycline resistance gene tet (M), which is widely distributed among Gram-positive bacteria, imparts resistance to tetracyclines via a ribosomal protection mechanism. To date, tet (M) has been identified in 38 genera of Gram-positive bacteria and 39 genera of Gram-negative bacteria (1–3). In most bacteria, tet (M) is associated with other tetracycline resistance genes, likely involving conjugative transposons located on the chromosome and conjugative plasmids (4–6). In Gram-positive bacteria, tet (M) has been linked to 23 transposons, including Tn916/Tn1545-like in Enterococcus spp. (7), Tn5801-like in Staphylococcus aureus and Enterococcus faecalis (6, 8), and Tn5397-like in Clostridium difficile and Enterococcus faecium (9, 10). Among these, Tn916, which was the first conjugative transposon found to covey resistance to antibiotics (1), harbors 24 open reading frames that are organized into functional modules involved in conjugation transfer, recombination (excision and insertion responses), transcriptional regulation, and auxiliary functions (antibiotic resistance) (11, 12). Subsequently, Tn1545, which was identified in Streptococcus pneumoniae, is highly homologous to Tn916 (difference of only one nucleotide), especially between the ~2,000-bp integrase and cleavase genes that encode translocation functions. Also, Tn1545 harbors three resistant genes [tet (M), ermAM, and aphA-3] (13, 14), while Tn916 only harbors tet (M). The similarity in the tet (M) sequence between Tn1545 and Tn916 is reportedly 94.5% (15), demonstrating that tet (M) is evolutionarily conserved across different species of bacteria. Tn5801, a member of the Tn916 family, was initially isolated from Staphylococcus aureus in 2001 (16). Afterward, different types of Tn5801-like gene islands have been identified in Enterococcus, Lactobacillus, Lactococcus, Staphylococcus, Streptococcus, and Clostridium isolates from humans, animals, and food products in Europe, the United States, Asia, and Australia, suggesting that the element carrying tet (M) has spread among Gram-positive species worldwide (6, 17–21). Transmission of tet (M) is mediated by transposons and plasmids. However, the gene environment of tet (M) remains unclear.
Although various transposons carrying tet (M) have been extensively reported in Gram-positive bacteria, the mode of transmission in Gram-negative bacteria remains unclear. Our group previously reported that tet (M) was linked to Tn6539 in E. coli, Tn6709 and Tn6942 in Salmonella (22–24). Here, we report the novel tet (M)-bearing Tn7124 and a Tn6942-like fragment carried by pTA2 and pTA7, respectively, and clarify the molecular, genetic, and biological characteristics of pTA2 and pTA7 in E. coli DH5α cells.
2 Materials and methods
2.1 Bacterial strains
Two tet (M)-positive E. coli strains (A2 and A7) isolated from the feces of pigs in Henan province, China, in December 2017 were identified using the VITEK 2 automated bacterial identification and susceptibility testing system (bioMérieux, Marcy-l’Étoile, France). E. coli strains J53 (sodium azide resistant), DH5α, and ATCC 25922 were stored in our laboratory.
2.2 Transformation experiments
Competent E. coli DH5α cells were transformed with the plasmids of the donor strains A2 and A7 by electroporation. The transformants were screened on Luria–Bertani (LB) agar plates containing doxycycline (16 mg/L). The conjugation frequency was calculated as the ratio of the number of transconjugants per recipient. Both transformants were confirmed by S1 nuclease pulsed-field gel electrophoresis (S1-PFGE). The presence of tet (M) in the transformants was confirmed by polymerase chain reaction analysis and whole genome sequencing.
2.3 Antimicrobial susceptibility testing
The susceptibility of three tet (M)-positive isolates and corresponding transformants to 13 antibiotics (i.e., amoxicillin, ceftiofur, cefquinome, gentamicin, amikacin, oxytetracycline, doxycycline, florfenicol, colistin, trimethoprim-sulfoxazole, enrofloxacin, olaquindox, and mequindox) was determined by the broth micro dilution method in accordance with the guidelines of the Clinical and Laboratory Standards Institute (25, 26). E. coli ATCC 25922 was used for quality control.
2.4 S1-PFGE and southern hybridization
DNA from the donor strains and transformants were treated with S1 nuclease and then separated by PFGE. The location of tet (M) was determined by Southern hybridization.
2.5 Plasmid sequencing and annotation
Plasmids pTA2 and pTA7 were extracted from the transformants ZA2 and ZA7 using the QIAGEN® Plasmid Midi Kit (Qiagen GmbH, Hilden, Germany). Whole genome sequencing of A2 and A7 were conducted a HiSeq™ Sequencing System (Illumina, Inc., San Diego, CA, United States) and Oxford Nanopore Technologies (ONT) MinION platforms (Oxford Nanopore Technologies Ltd., Oxford) with 400 bp paired-end reads. The obtained sequences were assembled using unicycler 0.5.0 and Flye 2.9.11 software with the hybrid strategy. The pTA2 and pTA7 sequences were initially predicted and annotated using the RAST (Rapid Annotation using Subsystem Technology) server (v2.0),2 and corrected manually using the Basic Local Alignment Search Tool (BLAST) algorithm.3 The plasmids replicon genotype were identified using PlasmidFinder 2.1.4 Insertion sequence elements were recovered using ISfinder.5 Contigs containing resistance genes were ascertained using the comprehensive antibiotic resistance database (CARD).6 Comparative analysis was conducted and plasmid maps were generated using the Easyfig application7 and the BLAST Ring Image Generator.8
2.6 Biological characteristics of pTA2 and pTA7
The stability of pTA2 and pTA7 in E. coli DH5α cells cultured in antibiotic-free LB broth were determined as previously described (27). Briefly, a 100-μL aliquot of suspended bacterial cells was diluted in LB broth and plated on LB agar. Then, 20 colonies were randomly chosen and replica plated onto LB agar plates with 16 μg/mL doxycycline, and the presence of the tet(M) gene were confirmed by PCR.
The bacterial growth kinetics of E. coli ZA2 (ZA7) and DH5α cells were observed by culturing overnight in LB medium with and without doxycycline. Then, 107 colony-forming units (CFUs) were added independently into 20 mL of fresh LB medium with and without doxycycline and cultured at 37°C for 12 h. Absorbance at 600 nm was measured every hour.
The fitness costs of pTA2 and pTA7 were determined by competition assays of E. coli ZA2 (ZA7) and DH5α cells as previously described. Briefly, E. coli ZA2 (ZA7) and DH5α cells were cultured in 20 mL of LB broth at 37°C for 16 h. Then, 2 × 106 CFUs of E. coli ZA2 (ZA7) and DH5α cells were cultured in 20 mL of antibiotic-free LB broth at 37°C with continuous shaking (120 rpm). Finally, 4 × 106 CFUs were transferred to 20 mL of fresh LB broth every 24 h. Samples were collected every hour during the first 12 h and then every 24 h for 7 days. The CFUs of each sample on LB agar plates with and without doxycycline were quantified.
2.7 Nucleotide sequence accession number
The complete sequences of pTA2 and pTA7 in addition to chromosome A2 and A7 were submitted to the GenBank database9 under accession numbers CP069710, CP069708, CP069711, and CP069709, respectively.
3 Results and discussion
3.1 Characterization of Escherichia coli strains A2 and A7
Although transformation of E. coli strains A2 and A7 by conjugation was not possible, E. coli DH5α cells were successfully transformed by electroporation at the same frequency of 1 × 10−6. Strains A2 and A7, and the corresponding transconjugants ZA2 and ZA7, were resistant to tetracycline, oxytetracycline, florfenicol, and amoxicillin, while A2 and ZA2 were resistant to sulfamethoxazole-trimethoprim (Table 1). S1-PFGE showed that a single plasmid was obtained from the transconjugants ZA2 and ZA7 (designated as pTA2 and pTA7, respectively). As shown in Supplementary Figures S1, S2, Southern hybridization indicated that tet(M) was located on pTA2 (~110 kb) and pTA7 (~70 kb).
3.2 Sequence analysis of plasmids in Escherichia coli strains A2 and A7
Whole genome sequencing showed that the chromosome of strain A2 comprised 4,761,946 bp (4,593 protein-coding regions) and harbored one copy of pTA2 (120,379 bp), while the chromosome of strain A7 comprised 4,620,143 bp (4,423 protein-coding regions) and harbored one copy of pTA7 (77,243 bp). The genome of A2 had more than 30 mobile elements, which included IS1A, ISEc17, IS609, IS150, and IS1H, while the genome of A7 had more than 90 mobile elements, which mainly included ISKpn26 and IS1R (Figure 1).
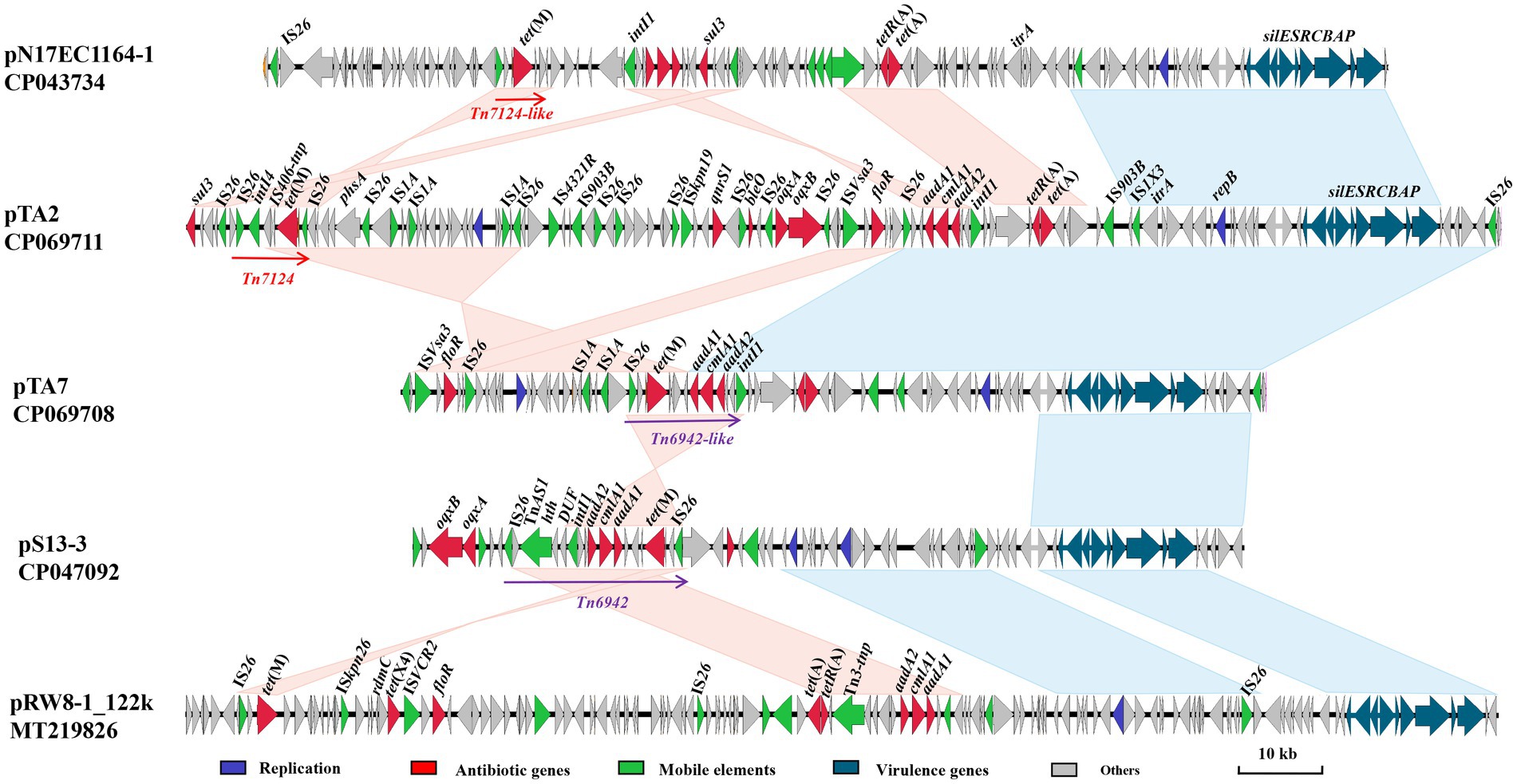
Figure 1. Comparative analysis of pTA2, pTA7 with other plasmids including pN17EC1163–1, pS13-3, pRW8_122k. Replicon genes are in blue; resistance genes in red; mobile elements in green; the loci of virulence-associated genes (silESRCBAP) in teal; and hypothetical proteins in gray.
BLASTn analysis indicated that pTA2 was 99.75% sequence similarity with pTA7 at 72% coverage, 99.95% at 73% coverage with E. coli p2_BE2-5 (CP032988) isolated from an egret in Chengdu, China, 99.76% at 72% coverage with E. coli pN17EC1163–1 (IncR-X1, CP043734) isolated from swine in the United States, and 99.20% at 56% coverage with E. coli p14EC007b (CP024133) isolated from clinical patients in Guangdong, China (Figure 2). In addition to the three plasmids mentioned above, only 12 strains exhibited higher sequence similarity with pTA2 (99.2% at 51–66% coverage), which included 11 E. coli strains and 1 Salmonella isolate from swine, and there were only three plasmids isolated from E. coli strains obtained from animals, including plasmid pYUXJMC1-2, (CP125352, chicken meat), plasmid unnamed, (CP038858, feces of pig) and plasmid unnamed11 (CP122915, feces of Sus scrofa). Compared to plasmids pTA2 and pTA7, they just shared backbone sequences of IncR plasmid. While pTA2 shared lower sequence similarity with other plasmids in the NCBI database. Although reports of pTA2 and pTA7-like plasmids are limited, the majority of 17 plasmids isolated in China exhibited regional characteristics among various hosts, indicating wide circulation.
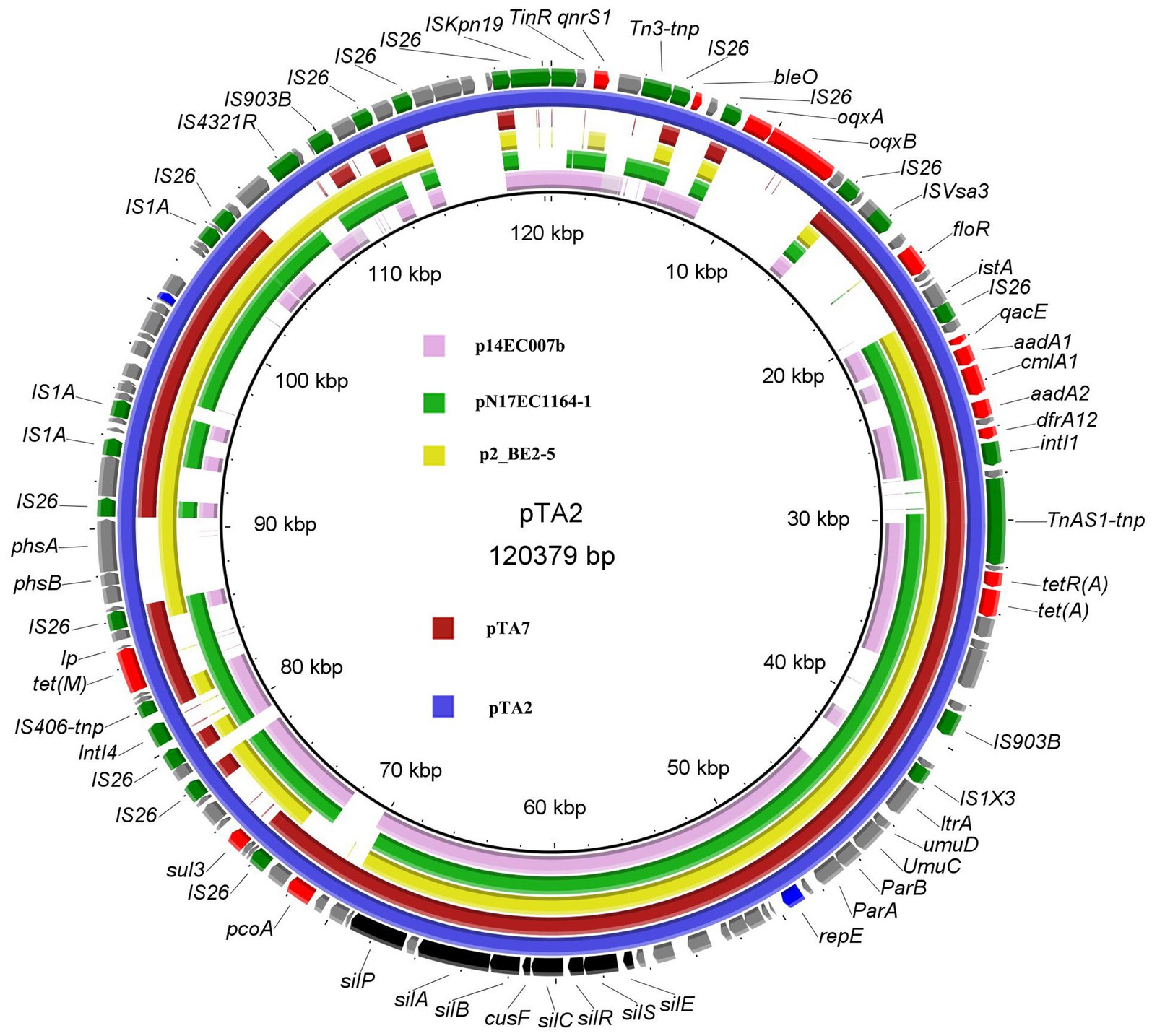
Figure 2. Structural comparisons of pTA2, pTA7 and the similar plasmids. The outer ring represents the CDSs of the reference sequence pTA2. The similar plasmids including the E.coli plasmids pN17EC1163-1 (IncR-X1, CP043734), p14EC007b (CP024133) and p2_BE2-5 (CP032988).
Comparative sequence analysis demonstrated that pTA2 and pTA7 were the IncR type, with GC contents of 51%, and the same replication initiator repB, which shared 100% amino acid identity to the corresponding region of pK245 of Klebsiella pneumoniae strain DQ449578 isolated from a patient in Taiwan, China, in 2002. Both pTA2 and pTA7 had typical IncR plasmid backbone genes, including repB, parAB, and umuCD, but not resD, and the downstream virulence gene silESRCBAP (Figure 1).
The most notable difference between pTA2 and pTA7 was the MDR region. The MDR region of pTA7 consisted of three resistance modules, ISVsa3-VirD2-floR-lysR, insertion sequence (IS)26-ctp-lp-tet(M)-IS406tnp-ctp-aadA1-cmlA1-aadA2-dfrA12-IntI1, and tet(A)-tetR(A), and several insertion sequences. The fragment carrying tet(M) was 2,300 kb in length. The tet(M)-bearing structure Tn6942 was similar to pS13-3 (CP047092) of Salmonella enterica, but lacked the helix-inverse-helix domain, Tn3 family transposase, and IS26, which were located downstream of insI1 in pTA7. Therefore, the fragment was named Tn6942-like. The MDR region of pTA2 consists of 11 resistance genes, including sul3, tet(M), qnrS1, bleO, oqxAB, floR, aadA1, cmlA1, aadA2, and tet(A)-tetR(A), and 22 insertion sequences, which included IS26, IS1A, IS903B, ISVsa3, ISKpn19, IS4321R, and IS1X. Notably, pTA2 contained sul3, qnrS1, bleO, and an oqxAB-bearing resistance module along with several insertion sequences upstream of ISVsa3, while the other sequences were almost consistent with pTA7 (Figure 1).
In addition, the fragment containing tet(M) slightly differed between pTA7 and pTA2. The tet(M) sequence was located at the composite Tn7124 between two IS26-bracked composites, as determined in reference to the Transposon Nomenclature Database.10 Tn7124 is 7.2 kb in length and organized as IS26, conjugative transfer protein ctp, tet(M) leader peptide gene, tet(M), hypothetical protein (hp), integrase (Int)I4, ctp, and IS26 (Figure 1). Comparative sequence analysis showed that fragment IS26-ctp-lp-tet(M) was relatively conserved and shared 100% sequence identity with the sequences of pN17EC1163–1 and pRW8-1_122k (Figure 1), while the downstream sequence of tet(M) was hp-IS406 tnp-IntI3-hp, which is same sequence as Tn7124-like of pN17EC1163–1.
Although usually coded by the bacterial chromosome, tet(M) was also located on the conjugative plasmids IncHI2, IncFII, IncFI, IncN1-IncHI2, and IncX1-FI isolated from Campylobacter jejuni, Neisseria meningitidis, Kingella denitrificans, Aikenella erosus, Clostridium percapsulatus, Salmonella, and E. coli (28, 29). Hence, conjugation plasmids can facilitate horizontal transfer of tet(M) genes between species. IncR plasmids were initially identified as a novel group of incompatible plasmids in 2009 (30). Subsequent studies have reported that conjugation plasmids are broadly distributed in clinically relevant strains of Enterobacteriaceae and Klebsiella pneumoniae, which intensified the fitness of the host cell by conferring resistance to fluoroquinolones (qnrS1 and qnrB4), aminoglycosides (armA), and beta-lactams (blaKPC-2, blaDHA-1, blaNDM-1, and blaVIM-1) and carbapenems (31–34). In addition, IncR plasmids often coexist with other replicants, such as IncC, IncN, IncHI, and IncFII. Thus, the resistance reservoir carried by IncR plasmids may spread to transferable plasmids through translocation or plasmid recombination events, thereby contributing to the high plasticity of multiple replicant plasmids (35). The complex class 1 integrons and ISs located on pTA2 and pTA7 are formidable gene-capturing tools that can mobilize extremely large sections of DNA encoding a variety of antibiotic resistance genes (36). Therefore, tet(M)-bearing pTA2 and pTA7 may have spread between different species of bacteria and conferred resistance to tetracycline.
In this study, the tet(M)-bearing complex Tn7124 had two IS26 elements in opposite directions at boundaries. IS26, a member of the IS6 insertion sequence family, transmits antibiotic resistance genes in Gram-negative bacteria primarily through the formation of complex transposons, of which most contain a central region containing the resistance gene and two IS26 elements at the boundaries in the same or opposite directions (37). In Gram-negative bacteria, IS26 elements are usually found at the boundary of the transposon, but there is no marker of IS26 integration on either side of the 8-bp target point repeat (38, 39). Therefore, complex transposons are not formed by metastasis, but rather homologous recombination. In 2014, Harmer et al. proposed a new resistance gene mechanism model mediated by IS26, which considered IS26 and resistance genes as mobile units (TU), a new family of mobile genetic (40). The TU released from the gene sequence can recognize another adjacent IS26 as a target site in the same direction, which resulted in tandem arrangement of IS26 elements and the formation of complex transposons.
The frequency of TU-mediated co-integration formation was 60 times higher than that of single IS26-mediated co-integration formation, indicating that intact IS26 elements in the gene sequence can recruit IS26-mediated resistance genes. TU integration can occur through reactions catalyzed by Tnp (transposase of IS26) or by recA-dependent homologous recombination. However, the reaction catalyzed by tnp is 100 times more efficient than recA-dependent homologous recombination. In addition, analysis of different patterns of the flanking sequences of 70 IS26 copies in 8 genomes of carbapenemase-producing Enterobacteriaceae found that IS26 promoted rearrangement of drug-resistant plasmids through inter-and intramolecular replication translocations (41). Despite repeated attempts, the closed circular intermediate IS26-ctp-lp-tet(M)-hp-IS406 tnp-IntI3-hp of Tn7124 was not detected in the A2 strain and no direct repeats were found flanking the IS26 element in Tn7124, suggesting that Tn7124 may have occurred by recombination, rather than transposition, which was likely controlled by the IS26 element.
3.3 Biological characteristics of pTA2 and pTA7
pTA2 and pTA7 were highly stable (100%) for 14 days in E. coli DH5α cells cultured in antibiotic-free medium, indicating that both carried the resistance genes tet(M), tet(A), floR, aadA1, cmlA1, aadA2, and sul2. As shown in Figure 3A, ZA2 and ZA7 exhibited similar growth as E. coli strain DH5α in LB broth without doxycycline, while the growth rates of ZA2 and ZA7 were slightly increased in LB broth without doxycycline than with doxycycline. E. coli strain DH5α failed to grow in the presence of doxycycline. The growth kinetics obtained over 12 h showed no evidence of any fitness cost exacted by pTA2 and pTA7.
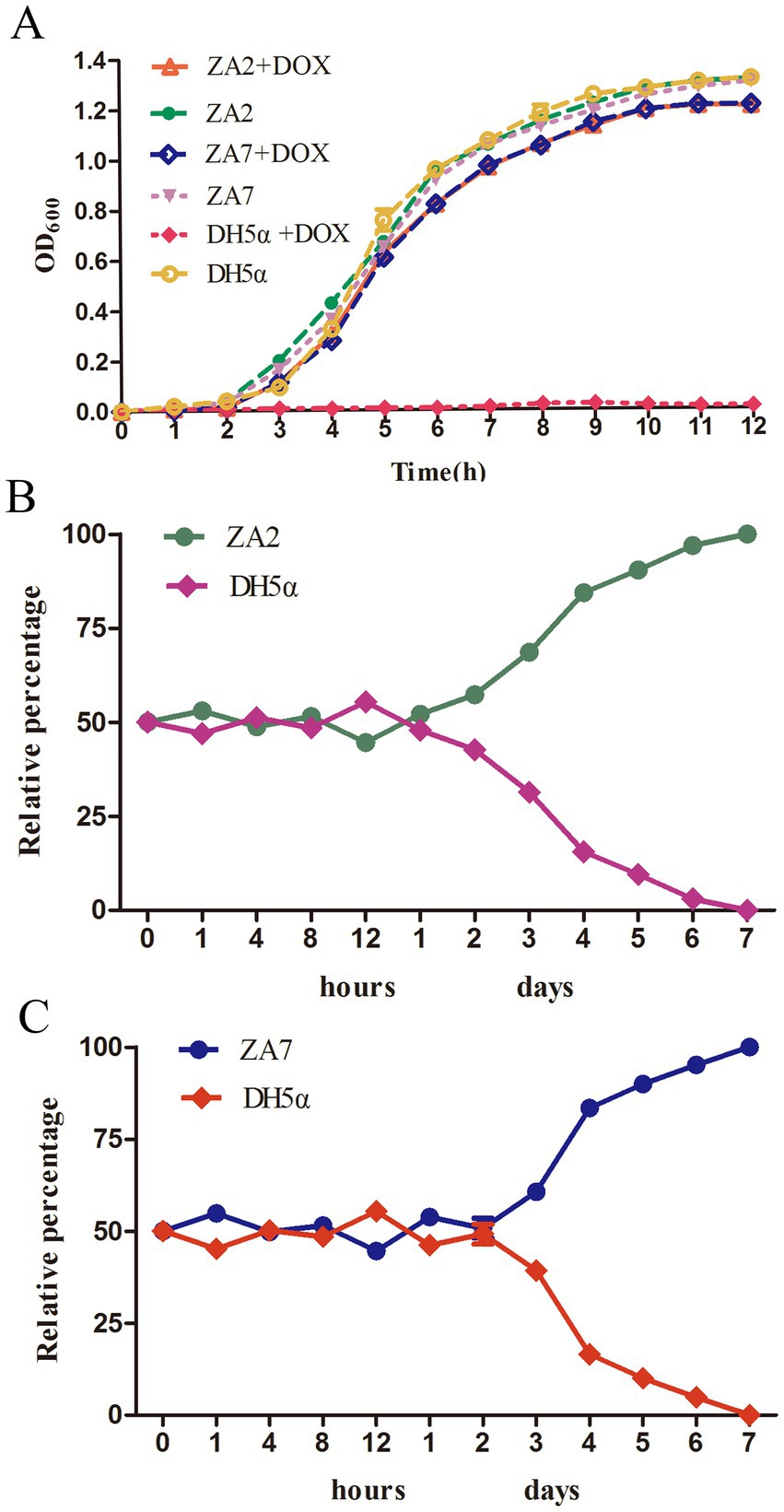
Figure 3. (A) The growth kinetics of strains ZA2, ZA7and DH5α in LB broth in the presence and absence of 16 ug/ml doxycycline. The standard deviations of the mean of three independent experiments represented by error bars. (B) The competition profiles of strains ZA2 and DH5α, the initial ratio was equal. The relative percentage of each strain at different time points is shown. The competition curves were constructed with the mean of three independent experiments that the standard deviation represented by error bars. (C) The competition profiles of strains ZA7 and DH5α, the initial ratio was equal. The relative percentage of each strain at different time points is shown. The competition curves were constructed with the mean of three independent experiments that the standard deviation represented by error bars.
Further studies on the fitness cost of plasmids carrying tet(M) will be of great interest to determine the importance of these plasmids in tetracycline resistance of E. coli. As shown in Figures 3B,C, equal numbers of bacteria with and without plasmids were mixed and subcultured every 24 h for 7 days, and the proportion of bacteria carrying plasmids was monitored every hour for the first 12 h. During this period, the ratio was almost 1:1 at every time point, whereas ZA2 outnumbered E. coli DH5α after co-culture for 2 days and ZA7 after 3 days. Afterward, the number of E. coli DH5α cells in the mixed culture continuously decreased. At 7 days, the proportions of ZA2 and ZA7 decreased to less than 1%. The transconjugants ZA2 and ZA7 showed competitive advantages relative to E. coli DH5α throughout the entire experiment.
4 Conclusion
The novel tet(M)-bearing resistance modules Tn7124 and Tn6942-like were found in pTA2 and pTA7 isolated from E. coli strains A2 and A7. Transfer of tet(M) occurred with the resistant genes sul3, qnrS1, bleO, oqxAB, floR, aadA1, cmlA1, aadA2, and tet(A)-tetR(A) in the novel self-transmissible pTA2, and with floR, aadA1, cmlA1, aadA2, and tet(A)-tetR(A) in pTA7, suggesting that pTA2 and pTA7 may act as reservoirs for these genes. Hence, these IncR plasmids likely facilitate the dissemination of tet(M) and other resistance genes. Therefore, there is an urgent need to strengthen surveillance and efforts to limit the spread of this MDR plasmid among Enterobacteriaceae, as well as to enhance surveillance of antibiotic resistance outwards the hospital settings, because studying resistance into the hospital setting had no result on fighting resistance, all these years. Typing of clones, plasmids and transposons derived from food producing animals could reveal MDR origins and their circulation between humans and animals.
Data availability statement
The datasets presented in this study can be found in online repositories. The names of the repository/repositories and accession number(s) can be found in the article/Supplementary material.
Ethics statement
For convenient sampling, the samples were collected for bacterial isolation after the farmer’s verbal consent. In accordance with the protocol approved by the Institutional Animal Care and Use Committee of Huanghuai University, China (Permit Number: 16–0085 date: 15 June 2013), the sample collection procedures were carried out with strict adherence to ethical guidelines to ensure animal welfare and minimize suffering.
Author contributions
YL: Formal analysis, Funding acquisition, Software, Writing – original draft, Writing – review & editing. ZQ: Data curation, Writing – original draft. YM: Writing – original draft. MW: Supervision, Writing – review & editing. GH: Conceptualization, Formal analysis, Writing – review & editing. EL: Data curation, Formal analysis, Supervision, Writing – review & editing.
Funding
The author(s) declare that financial support was received for the research, authorship, and/or publication of this article. This work was supported in part by the Natural Science Foundation of Henan Province (232300420217).
Conflict of interest
The authors declare that the research was conducted in the absence of any commercial or financial relationships that could be construed as a potential conflict of interest.
Publisher’s note
All claims expressed in this article are solely those of the authors and do not necessarily represent those of their affiliated organizations, or those of the publisher, the editors and the reviewers. Any product that may be evaluated in this article, or claim that may be made by its manufacturer, is not guaranteed or endorsed by the publisher.
Supplementary material
The Supplementary material for this article can be found online at: https://www.frontiersin.org/articles/10.3389/fvets.2024.1430398/full#supplementary-material
Supplementary Figure S1 | (a) S1-PFGE of E. coli A2 and its transconjugant ZA2. (b) Southern hybridization of E. coli A2 and ZA2 with the tet(M) gene as the probe. Marker, Salmonella Braenderup H9812.
Supplementary Figure S2 | (a) S1-PFGE of E. coli A7 and its transconjugant ZA7. (b) Southern hybridization of E. coli A7 and ZA7 with the tet(M) gene as the probe. Marker, Salmonella Braenderup H9812.
Footnotes
1. ^https://github.com/fenderglass/Flye
3. ^http://blast.ncbi.nlm.nih.gov/blast
4. ^https://cge.food.dtu.dk/services/PlasmidFinder/
5. ^https://isfinder.biotoul.fr/
7. ^https://mjsull.github.io/Easyfig/
8. ^https://sourceforge.net/projects/brig/
References
1. Franke, AE, and Clewell, DB. Evidence for a chromosome-borne resistance transposon (Tn916) in Streptococcus faecalis that is capable of "conjugal" transfer in the absence of a conjugative plasmid. J Bacteriol. (1981) 145:494–502. doi: 10.1128/jb.145.1.494-502.1981
2. Doenhoefer, A, Franckenberg, S, Wickles, S, Berninghausen, O, Beckmann, R, and Wilson, DN. Structural basis for Tetm-mediated tetracycline resistance. Proc Natl Acad Sci U S A. (2012) 109:16900–5. doi: 10.1073/pnas.1208037109
3. Roberts, MC, and Schwarz, S. Tetracycline and Phenicol resistance genes and mechanisms: importance for agriculture, the environment, and humans. J Environ Qual. (2016) 45:576–92. doi: 10.2134/jeq2015.04.0207
4. Jones, CH, Tuckman, M, Murphy, E, and Bradford, PA. Identification and sequence of a tet(M) tetracycline resistance determinant homologue in clinical isolates of Escherichia coli. J Bacteriol. (2006) 188:7151–64. doi: 10.1128/JB.00705-06
5. Tuckman, M, Petersen, PJ, Howe, AYM, Orlowski, M, Mullen, S, and Chan, K. Occurrence of tetracycline resistance genes among Escherichia coli isolates from the phase 3 clinical trials for tigecycline. Antimicrob Agents Chemother. (2007) 51:3205–11. doi: 10.1128/AAC.00625-07
6. de Vries, LE, Christensen, H, Skov, RL, Aarestrup, FM, and Agersø, Y. Diversity of the tetracycline resistance gene tet(M) and identification of Tn916-and Tn5801-like (Tn6014) transposons in Staphylococcus aureus from humans and animals. J Antimicrob Chemother. (2009) 64:490–500. doi: 10.1093/jac/dkp214
7. Cauwerts, K, Decostere, A, De Graef, EM, Haesebrouck, F, and Pasmans, F. High prevalence of tetracycline resistance in Enterococcus isolates from broilers carrying the erm(B) gene. Avian Pathol. (2007) 36:395–9. doi: 10.1080/03079450701589167
8. León-Sampedro, R, Fernández-de-Bobadilla, MD, San, MÁ, Baquero, F, and Coque, TM. Transfer dynamics of Tn6648, a composite integrative conjugative element generated by tandem accretion of Tn5801 and Tn6647 in Enterococcus faecalis. J Antimicrob Chemother. (2019) 74:2517–23. doi: 10.1093/jac/dkz239
9. Agersø, Y, Pedersen, AG, and Aarestrup, FM. Identification of Tn5397-like and Tn916-like transposons and diversity of the tetracycline resistance gene tet(M) in enterococci from humans, pigs and poultry. J Antimicrob Chemother. (2006) 57:832–9. doi: 10.1093/jac/dkl069
10. Roberts, AP, Johanesen, PA, Lyras, D, Mullany, P, and Rood, JI. Comparison of Tn5397 from Clostridium difficile, Tn916 from Enterococcus faecalis and the CW459 tet(M) element from Clostridium perfringens shows that they have similar conjugation regions but different insertion and excision modules. Microbiology. (2001) 147:1243–51. doi: 10.1099/00221287-147-5-1243
11. Roberts, AP, and Mullany, P. A modular master on the move: the Tn916 family of mobile genetic elements. Trends Microbiol. (2009) 17:251–8. doi: 10.1016/j.tim.2009.03.002
12. Roberts, AP, and Mullany, P. Tn916-like genetic elements: a diverse group of modular mobile elements conferring antibiotic resistance. FEMS Microbiol Rev. (2011) 35:856–71. doi: 10.1111/j.1574-6976.2011.00283.x
13. Flannagan, SE, Zitzow, LA, Su, YA, and Clewell, DB. Nucleotide sequence of the 18-kb conjugative transposon Tn916 from Enterococcus faecalis. Plasmid. (1994) 32:350–4. doi: 10.1006/plas.1994.1077
14. Poyart-Salmeron, C, Trieu-Cuot, P, Carlier, C, and Courvalin, P. The integration-excision system of the conjugative transposon Tn1545 is structurally and functionally related to those of lambdoid phages. Mol Microbiol. (1990) 4:1513–21. doi: 10.1111/j.1365-2958.1990.tb02062.x
15. Oggioni, MR, Dowson, CG, Smith, JM, Provvedi, R, and Pozzi, G. The tetracycline resistance gene tet(M) exhibits mosaic structure. Plasmid. (1996) 35:156–63. doi: 10.1006/plas.1996.0018
16. Kuroda, M, Ohta, T, Uchiyama, I, Baba, T, Yuzawa, H, Kobayashi, I, et al. Whole genome sequencing of meticillin-resistant Staphylococcus aureus. Lancet. (2001) 357:1225–40. doi: 10.1016/S0140-6736(00)04403-2
17. Denapaite, D, Brückner, R, Nuhn, M, Reichmann, P, Henrich, B, and Maurer, P. The genome of Streptococcus mitis B6--what is a commensal? PLoS One. (2010) 5:e9426. doi: 10.1371/journal.pone.0009426
18. Holden, MT, Lindsay, JA, Corton, C, Quail, MA, Cockfield, JD, Pathak, S, et al. Genome sequence of a recently emerged, highly transmissible, multi-antibiotic-and antiseptic-resistant variant of methicillin-resistant Staphylococcus aureus, sequence type 239 (TW). J Bacteriol. (2010) 192:888–92. doi: 10.1128/JB.01255-09
19. Brenciani, A, Tiberi, E, Tili, E, Mingoia, M, Palmieri, C, Varaldo, PE, et al. Genetic determinants and elements associated with antibiotic resistance in viridans group streptococci. J Antimicrob Chemother. (2014) 69:1197–204. doi: 10.1093/jac/dkt495
20. León-Sampedro, R, Novais, C, Peixe, L, Baquero, F, and Coque, TM. Diversity and evolution of the Tn5801-tet(M)-like integrative and conjugative elements among Enterococcus, Streptococcus, and Staphylococcus. Antimicrob Agents Chemother. (2016) 60:1736–46. doi: 10.1128/AAC.01864-15
21. Li, H, Zhao, C, Chen, H, Zhang, F, He, W, Wang, X, et al. Identification of gene clusters associated with host adaptation and antibiotic resistance in Chinese Staphylococcus aureus isolates by microarray-based comparative genomics. PLoS One. (2013) 8:e53341. doi: 10.1371/journal.pone.0053341
22. Liu, YY, Liu, XK, Cui, XD, Chen, M, Hu, GZ, and Pan, YS. Characterization of pTS14, an IncF2:A1:B1 plasmid carrying tet(M) in a Salmonella enterica isolate. Front Microbiol. (2020) 11:1523. doi: 10.3389/fmicb.2020.01523
23. Liu, YY, Sun, YW, Sun, HR, Luo, XW, He, DD, Wu, H, et al. Characterization of the IncHI2 plasmid pTW4 harboring tet(M) from an isolate of Escherichia coli ST162. J Antibiot. (2020) 73:876–80. doi: 10.1038/s41429-020-0337-y
24. Liu, YY, He, DD, Zhang, MK, Pan, YS, Wu, H, Yuan, L, et al. The formation of two hybrid plasmids mediated by IS26 and Tn6952 in Salmonella enterica serotype enteritidis. Front Microbiol. (2021) 12:676574. doi: 10.3389/fmicb.2021.676574
25. CLSI (2023). Performance Standards for Antimicrobial Disk and Dilution Susceptibility Tests for Bacteria lsolated From. CLSI VET01-Ed5. Available at: https://clsi.org/media/jaxdiuzp/vet01ed6e_sample.pdf
26. CLSI (2023). Performance Standards for Antimicrobial Susceptibility Testing. 33rd ed. CLSI supplement M100. Clinical. Available at: https://clsi.org/standards/products/free-resources/access-our-free-resources
27. Wu, R, Yi, LX, Yu, LF, Wang, J, Liu, Y, Chen, X, et al. Fitness advantage of mcr-1-bearing IncI2 and IncX4 plasmids in vitro. Front Microbiol. (2018) 9:331. doi: 10.3389/fmicb.2018.00331
28. Taylor, DE, Hiratsuka, K, Ray, H, and Manavathu, EK. Characterization and expression of a cloned tetracycline resistance determinant from Campylobacter jejuni plasmid pUA466. J Bacteriol. (1987) 169:2984–9. doi: 10.1128/jb.169.7.2984-2989.1987
29. Knapp, JS, Johnson, SR, Zenilman, JM, Roberts, MC, and Morse, SA. High-level tetracycline resistance resulting from TetM in strains of Neisseria spp., Kingella denitrificans, and Eikenella corrodens. Antimicrob Agents Chemother. (1988) 32:765–7. doi: 10.1128/AAC.32.5.765
30. García-Fernández, A, Fortini, D, Veldman, K, Mevius, D, and Carattoli, A. Characterization of plasmids harbouring qnrS1, qnrB2 and qnrB19 genes in Salmonella. J Antimicrob Chemother. (2009) 63:274–81. doi: 10.1093/jac/dkn470
31. Compain, F, Frangeul, L, Drieux, L, Verdet, C, Brisse, S, Arlet, G, et al. Complete nucleotide sequence of two multidrug-resistant IncR plasmids from Klebsiella pneumoniae. Antimicrob Agents Chemother. (2014) 58:4207–10. doi: 10.1128/AAC.02773-13
32. Guo, Q, Spychala, CN, McElheny, CL, and Doi, Y. Comparative analysis of an IncR plasmid carrying armA, blaDHA-1 and qnrB4 from Klebsiella pneumoniae ST37 isolates. J Antimicrob Chemother. (2016) 71:882–6. doi: 10.1093/jac/dkv444
33. Kocsis, E, Gužvinec, M, Butić, I, Krešić, S, Crnek, SŠ, Tambić, A, et al. blaNDM-1 carriage on IncR plasmid in Enterobacteriaceae strains. Microb Drug Resist. (2016) 22:123–8. doi: 10.1089/mdr.2015.0083
34. Qu, D, Shen, Y, Hu, L, Jiang, X, Yin, Z, Gao, B, et al. Comparative analysis of KPC-2-encoding chimera plasmids with multi-replicon IncR:IncpA1763-KPC:IncN1 or IncFIIpHN7A8:IncpA1763-KPC:IncN1. Infect Drug Resist. (2019) 12:285–96. doi: 10.2147/IDR.S189168
35. Qian, C, Zhu, X, Lu, J, Shen, K, Chen, Q, Zhou, W, et al. Characterization of an IncR plasmid with two copies of ISCR-linked qnrB6 from ST968 Klebsiella pneumoniae. Int J Genom. (2020) 2020:1–8. doi: 10.1155/2020/3484328
36. Toleman, MA, and Walsh, TR. Combinatorial events of insertion sequences and ICE in gram-negative bacteria. FEMS Microbiol Rev. (2011) 35:912–35. doi: 10.1111/j.1574-6976.2011.00294.x
37. Waters, V, Zlosnik, JE, Yau, YC, Speert, DP, Aaron, SD, and Guttman, DS. Comparison of three typing methods for Pseudomonas aeruginosa isolates from patients with cystic fibrosis. Eur J Clin Microbiol Infect Dis. (2012) 31:3341–50. doi: 10.1007/s10096-012-1701-z
38. Curiao, T, Cantón, R, Garcillán-Barcia, MP, Cruz, F, Baquero, F, and Coque, TM. Association of composite IS26-sul3 elements with highly transmissible IncI1 plasmids in extended-Spectrum-β-lactamase-producing Escherichia coli clones from humans. Antimicrob Agents Chemother. (2011) 55:2451–7. doi: 10.1128/AAC.01448-10
39. Zienkiewicz, M, Kern-Zdanowicz, I, Carattoli, A, Gniadkowski, M, and Cegłowski, P. Tandem multiplication of the IS26-flanked amplicon with the blaSHV-5 gene within plasmid p1658/97. FEMS Microb Let. (2013) 341:27–36. doi: 10.1111/1574-6968.12084
40. Harmer, CJ, Moran, RA, and Hall, RM. Movement of IS26-associated antibiotic resistance genes occurs via a translocatable unit that includes a single IS26 and preferentially inserts adjacent to another IS26. MBio. (2014) 5:e0180-14. doi: 10.1128/mBio.01801-14
Keywords: IncR-type plasmid, tet (M), insertion sequence 26, transposon 7124, Escherichia coli
Citation: Liu Y, Qiao Z, Ma Y, Wang M, Hu G and Li E (2024) Molecular characterization of the tet (M)-carrying transposon Tn7124 and plasmids in Escherichia coli isolates recovered from swine. Front. Vet. Sci. 11:1430398. doi: 10.3389/fvets.2024.1430398
Edited by:
Nora Mestorino, National University of La Plata, ArgentinaReviewed by:
Kristina Kadlec, Independent Researcher, Wunstorf, GermanyPanagiota Giakkoupi, University of West Attica, Greece
Javier Eduardo Fernandez, University of Bern, Switzerland
Copyright © 2024 Liu, Qiao, Ma, Wang, Hu and Li. This is an open-access article distributed under the terms of the Creative Commons Attribution License (CC BY). The use, distribution or reproduction in other forums is permitted, provided the original author(s) and the copyright owner(s) are credited and that the original publication in this journal is cited, in accordance with accepted academic practice. No use, distribution or reproduction is permitted which does not comply with these terms.
*Correspondence: Enzhong Li, enzhongli@163.com