- 1Department of Pathobiology Pharmacology and Zoological Medicine, Faculty of Veterinary Medicine, Ghent University, Merelbeke, Belgium
- 2YUN NV, Niel, Belgium
- 3Department of Bioscience Engineering, University of Antwerp, Antwerp, Belgium
- 4Department of Small Animal Medicine and Clinical Biology, Faculty of Veterinary Medicine, Ghent University, Merelbeke, Belgium
The external ear canal, characterized by species-specific structural and physiological differences, maintains a hostile environment that prevents microbial overgrowth and foreign body entry, supported by factors such as temperature, pH, humidity, and cerumen with antimicrobial properties. This review combines several studies on the healthy ear canal’s structure and physiology with a critical approach to the potential existence of an ear microbiome. We use a comparative multi-species approach to explore how allergic conditions alter the ear canal microenvironment and cerumen in different mammalian species, promoting pathogen colonization. We propose a pathogenetic model in which allergic conditions disrupt the antimicrobial environment of the EEC, creating circumstances favorable for facultative pathogenic micro-organisms like Staphylococcus and Malassezia species, leading to otitis externa (OE). A better understanding of the underpinning mechanisms may lead to innovative approaches to disease mitigation.
1 Introduction
Inflammation of the external ear canal (EEC), otitis externa (OE), is a common dermatological disorder, diagnosed in approximately 1% of human patients in primary care (1). Changes in parameters such as the pH, temperature, or composition of earwax—a unique waxy substance derived from desquamated epithelial cells, sebaceous and ceruminous glands—can create an ideal environment for bacteria and/or yeasts to colonize and infect the ear canal. The OE-patient may present with pain, erythema and pruritis of the EEC and when microbial overgrowth is confirmed by cytology, topical antimicrobial treatment is required (2, 3). Complications include otitis media, otitis interna and even osteomyelitis of the temporal bone (2, 4, 5). Importantly, as long as the predisposing factor for OE—such as regular swimming, local trauma or allergic disease (6–8)—is not removed, these patients are at high risk of recurrence, resulting in chronic OE which often requires repeated antimicrobial treatment.
Antimicrobial resistance in ear pathogens is increasing (9, 10), which necessitates the development of alternatives to treat and prevent OE. As has been proposed in other skin disorders such as acne vulgaris and allergic dermatitis (11–13), it may be relevant to study new strategies that—instead of limiting the growth of ear pathogens by using bacteriostatic and bacteriocidic drugs—help to restore the balance between commensal microorganisms in the EEC, referred to as the ear microbiome. However—until now—little evidence exists on the presence, complexity, and protective role of a potential microbial community within the EEC. Moreover, it appears that the EEC is provided with many features that limit the growth of microorganisms, as will be discussed in this review.
We here aimed at investigating the presence and potential role of an ear microbiome by identifying the different challenges encountered by microorganisms entering the EEC, such as the different components of cerumen and the acidic pH. We focus on the disturbing impact of allergic dermatitis (AD)—a common underlying factor for OE—on different components of the microenvironment of the EEC to identify potential mechanisms favoring pathogen overgrowth. We use a cross-species comparative approach to identify common drivers of OE by including humans, dogs (prevalence of allergic OE comparable to humans) and horses (low allergic OE prevalence). By identifying the species-specific factors that contribute to maintaining a hostile environment within the ear canal, we aim to investigate the presence of an ear microbiome through a rigorous and critical analysis. Considering the impact of allergic dermatitis on the EEC and cerumen, we tried to identify new leads for future research into the prevention and treatment of allergy-induced OE.
2 The EEC in health and allergic dermatitis
An overview of the features of OE and the pathogenesis of allergic dermatitis related to OE in humans, dogs and horses is given in Table 1 and Supplementary Table 1. AD refers to different forms of AD, including a local hypersensitivity inflammatory response after exposure such as allergic contact dermatitis (14), and atopic dermatitis, a generalized chronic immune-mediated inflammatory skin disorder associated with IgE-antibodies (15–17). As shown in Table 1, OE in humans is associated with a local inflammatory reaction that occurs after direct contact of the EEC with hearing aids or chemicals in cosmetic products, while OE in dogs is associated with a generalized inflammatory reaction to environmental allergens (atopic dermatitis). The pathogenesis of atopic dermatitis in humans and dogs shows many similarities (18), however—as opposed to dogs with atopy (19)—OE is not a typical symptom of atopic dermatitis in humans (19). The question arises why this form of AD seems to have less impact on the human EEC compared with the canine EEC.
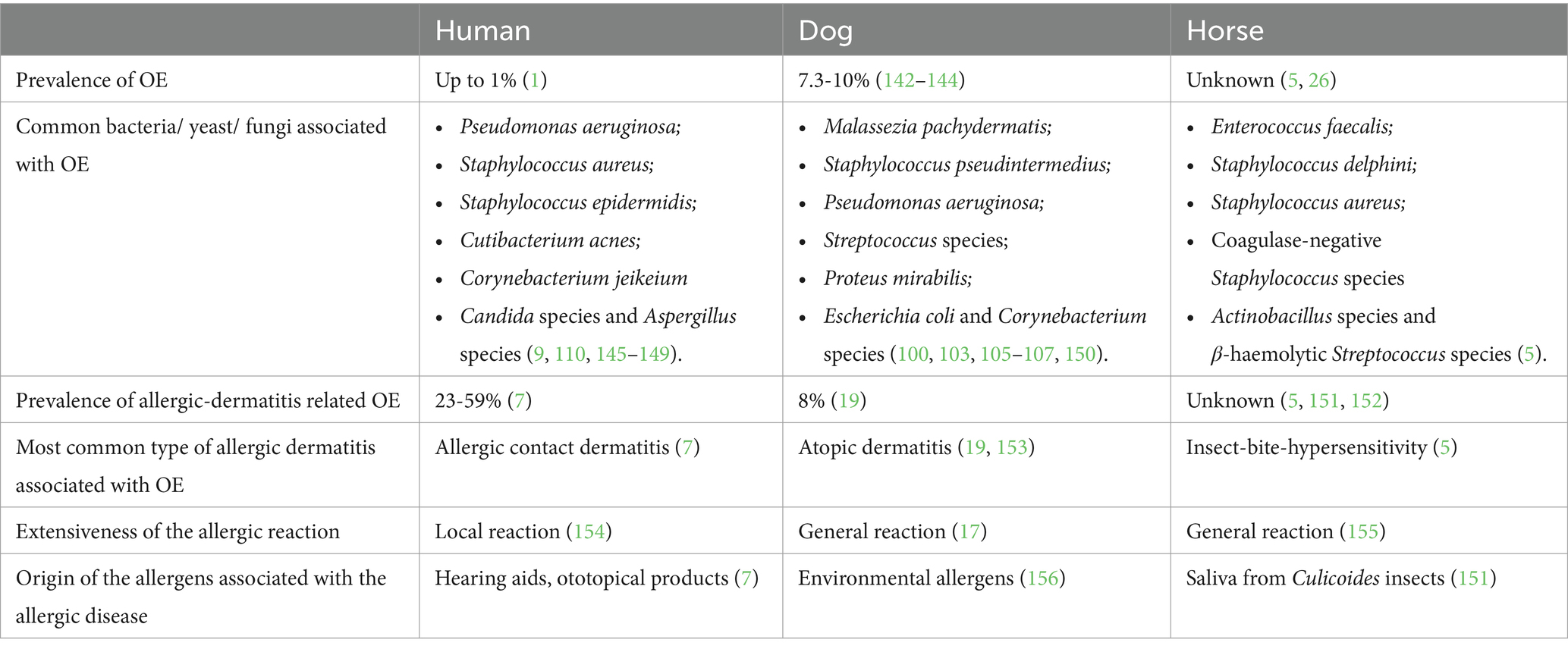
Table 1. Prevalence of (allergic-dermatitis-related) OE in humans, dogs and horses and features of the allergic disease most associated with OE.
2.1 Structure related to disease
The anatomy of the EEC differs greatly in humans, dogs and horses; both in length, shape and, most interesting, the ratio between the cartilaginous—containing sebaceous and ceruminous glands—and the non-glandular osseous parts of the ear canal. The comparison of the species-specific differences in the anatomic features and physiologic parameters of the EEC is shown in Table 2. The canine EEC is almost exclusively supported by cartilage and as such, entirely provided with sebaceous and ceruminous glands. Furthermore, the canine ear canal is defined by a site-dependent distribution of sebaceous and ceruminous glands, with an increasing ceruminous/sebaceous gland ratio toward the tympanum (20–23). In contrast, cartilage supports only 1/3rd to 1/4th of the human EEC and 3/4th of the equine EEC (24–28). It is possible that these anatomic varieties have a different impact on both the volume and composition of cerumen between these species. Structure-related predisposing factors for OE include both physical and histologic alterations. Narrowing of the EEC lumen—due to anatomical varieties, chronic changes as well as accumulation of cerumen—is associated with OE and ceruminoliths in humans and dogs (19, 29, 30). Reduced ear canal permeability might affect the antimicrobial microenvironment in the external ear, providing better conditions for pathogens to survive and colonize.
Allergic dermatitis is associated with a disruption and alteration of the skin barrier, which lines the EEC. Both in humans and dogs, atopic skin is characterized by a higher abundance of short chain ceramides compared to long chain ceramides, which impedes the integrity of the skin barrier and causes an increase in transepidermal water loss (31–34). Moreover, lesional skin from atopic dogs is defined by a decreased level of sphingomyelins and cholesteryl esters, essential compounds of the epidermal barrier (34). The expression of filaggrin, a crucial protein in the epidermal barrier; and claudin-1, an essential component of tight junctions, is decreased in human patients with atopic dermatitis, thereby further impairing the skin barrier (35, 36). The contribution of filaggrin mutations on the pathogenesis of CAD remains to be elucidated (18).
Considering the anatomic variations between dogs and humans, it seems possible that the inflammatory parameters associated with the pathogenesis of atopic dermatitis have a different impact on the canine EEC than on the human EEC, which could explain the difference in predisposition for OE in atopic patients of both species. The canine EEC is relatively longer than the human ear canal and in contrast to the simple, short horizontal EEC of humans, the canine EEC consists of a vertical and horizontal canal, with a sharp junction in between. Moreover, the canine EEC is almost completely covered by cerumen, which is in great contrast to humans, where sebaceous and ceruminous glands are only present in a small part of the EEC. Therefore, the induction of a general cutaneous inflammatory response in atopic subjects could hypothetically have a greater impact on the microenvironment and microbiome—containing facultative pathogens—in a longer, more enclosed, perhaps less aerobic canine EEC than on the shorter, more open human EEC.
Regarding the length of the EEC and the distribution of the sebaceous and ceruminous glands, the equine EEC can be considered as intermediate between the human and canine EEC. Interestingly, horses with Insect-bite-hypersensitivity (IBH), an allergic condition which resembles atopic dermatitis as discussed above, are also at risk for OE. This strengthens the assumption that the variations in length and distribution of the different glands in the EEC may, at least partly, be responsible for the different effect of allergies on the EEC in humans, dogs and horses.
2.2 (Patho) physiology of the EEC
An overview of the physiologic parameters of the EEC is shown in Table 2.
The temperature within the EEC resembles the average body temperature, both in dogs and humans. Yet, ear temperature was found to be significantly lower in men and elderly people, compared to women and adults, respectively (37). Also in dogs, ear temperature differed depending on age. Huang et al. demonstrated that dogs younger than 6 years and dogs weighing less than 6 kg showed significantly higher ear temperatures than dogs over 6 years old and over 6 kg bodyweight, respectively (38). So far, ear temperature has not been identified as a contributing factor to ear disease. Considering ‘calor’ as a key element in an inflammatory reaction, one could expect an increase in ear temperature during inflammation, which could potentially alter the growth and survival of certain microorganisms and stimulate an immunologic response. However, Yoshida et al. measured the EEC temperature in both OE and healthy dogs and did not find significant differences between these 2 groups of dogs (39).
As shown in Table 2, the pH of the human EEC is moderate to strong acidic, and as such, comparable to the skin pH. This low pH is caused by exocytosis of lysosomes and hydrolyzation of phospholipids, helps in creating an antimicrobial environment, enhances the epidermal barrier and has an influence on the epidermal lipid synthesis (40). Interestingly, the pH within the canine EEC appears to be less acidic, and also the skin pH in dogs, and in other mammals, is on average higher than the pH of human skin, which is possibly due to the presence of the hairy coat in mammals (e.g., skin pH in dogs: 6-7, skin pH in cats: 6.4–6.9, skin pH in horses: 7-8) (40). An acidic skin pH ensures better adhesion of the resident skin microbiota on the skin surface (41). Therefore, it is possible that the composition and diversity of the ear microbiome is affected differently by the acidic pH in the human ear canal and the higher pH in the canine ear canal.
The effect of allergic dermatitis on the otic pH, temperature and humidity has not been studied so far. In dogs, lesional atopic skin is determined by an elevation in skin pH (42). These changes in pH, however small, can have a significant influence on the microbiome, favoring the colonization of certain pathogens such as Staphylococcus aureus (43).
3 Cerumen as a defense mechanism toward OE-pathogens
Cerumen is a crucial biofluid in preventing microbial growth and infection of the EEC. To fulfill this function, cerumen contains several fundamental compounds, derived from desquamated epithelial cells and secretions of the sebaceous and ceruminous glands. In this section, the different compounds in cerumen with a defined antimicrobial action will be discussed.
3.1 The physical trapping function of cerumen: the contribution of lipids and mucins
3.1.1 Lipids
The high lipid concentration of cerumen acts as a physical capture mechanism for pathogens, foreign objects, and debris. Furthermore, a hydrophobic environment impedes the growth and survival of most microorganisms. Cerumen makes the epithelium of the EEC hard to reach and potential receptors for pathogens are covered, thereby preventing the adhesion of these microorganisms. Most research in humans has focused on the characterization and quantification of the lipid content, due to the high hydrophobic feature of cerumen. After lipid extraction of 19 cerumen samples, Bortz et al. reported an average lipid fraction of 52% +/-3% of the dry weight of human cerumen (44). The most recent study on the composition of lipids within human cerumen was performed by Stransky et al. (45), with emphasis on the nonpolar lipid fraction. The results are summarized in Figure 1. Huang et al. used lipid extraction to measure the lipid content and applied thin layer chromatography for the identification of the lipids present in 36 healthy cerumen samples from 20 dogs (46, 47). The total lipid content was on average 49.58% and as such, resembles the lipid content of human cerumen. The following lipids were detected: cholesterol, cholesterol esters, free fatty acids, fatty aldehydes, waxes, triglycerides, lecithin and sphingomyelin. Studies on the general lipid content of equine cerumen were not found.
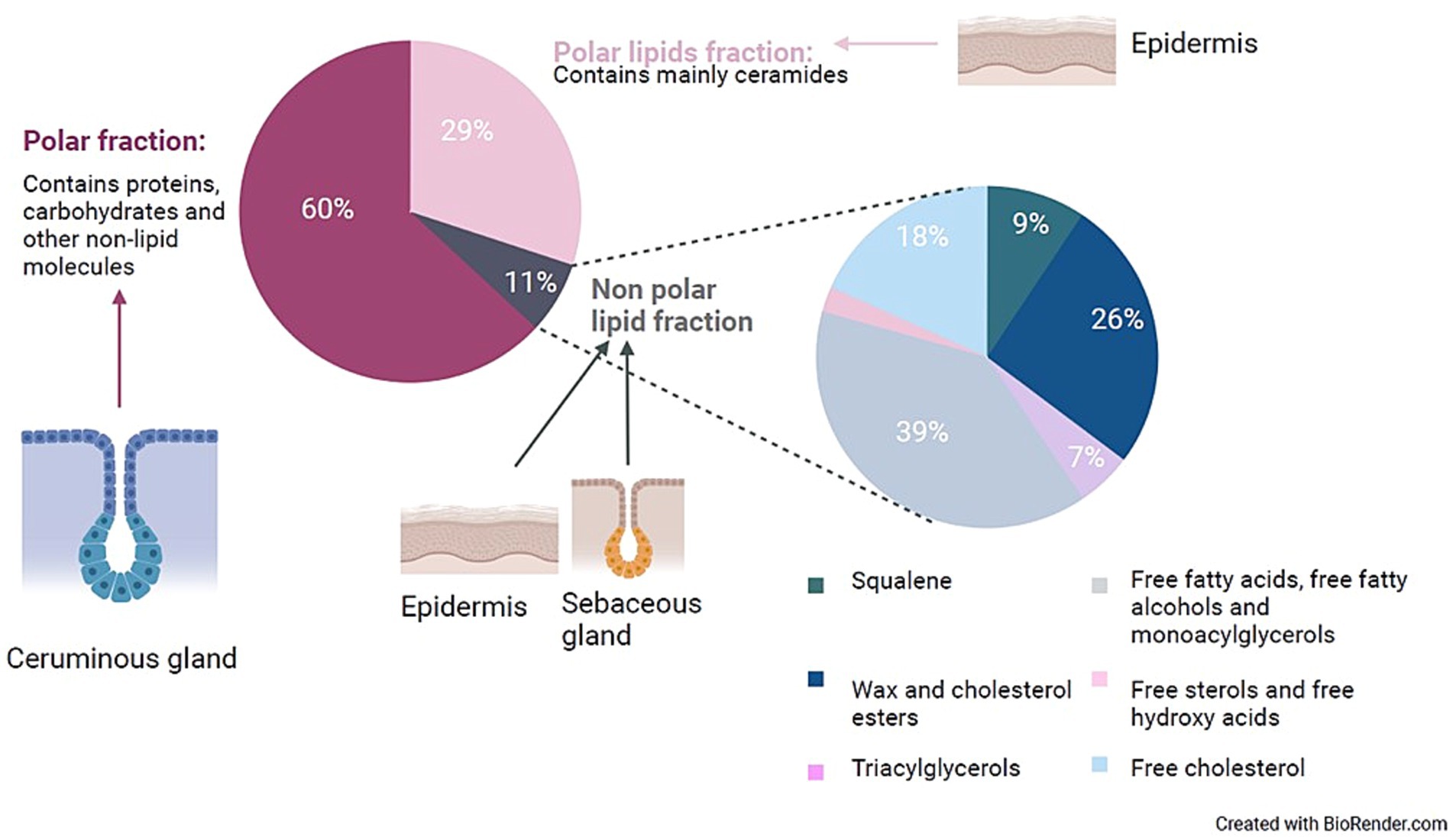
Figure 1. Presentation of the distribution and probable origin of the components of human cerumen. This figure was made by the authors of this review, based on the rough data available in the study by Stransky et al. (77) and the suggested origin of these lipids was based on literature research. During the study of Stransky et al., 200 doses of cerumen from the ears of a healthy individual were collected and separated by column chromatography. The non-polar lipid fraction was further characterized by gas chromatography—mass spectrometry.
AD influences the skin and sebum lipid composition. The total skin lipid percentage is significantly lower in human and canine AD patients which includes a decrease in ceramides, important contributors to the skin barrier (48, 49). Gene expression analysis of lesional skin of horses with IBH suggested also an alteration of the ceramide production compared to healthy skin (50). Lesional skin from atopic dogs is defined by a decreased level of sphingomyelins and cholesteryl esters, essential compounds of the epidermal barrier (34). Studies on the lipid composition of the skin and/or sebum in patients with contact dermatitis were not found.
The observed effects of allergic dermatitis on the skin and sebum lipid profile may theoretically have an impact on the cerumen lipid profile and could impair its protective function. Hypothetically, the higher abundance of short chain ceramides in cerumen might result in a more aqueous cerumen, allowing certain microorganisms (such as Staphylococcus species and Malassezia pachydermatis) to grow and colonize more easily than in normal cerumen and compared to other species present in the ear microbiome, leading to dysbiosis and OE.
The changes of the EEC after insertion of water might resemble the effects of a more aqueous cerumen on certain pathogens. In both dogs and humans, swimming has been associated with a higher risk for developing acute OE (19, 51). The insertion of water in the EEC results in a more humid EEC and an elevation of the pH and leads to maceration of the epidermis. In our opinion, the presence of water within the EEC may impair the function of cerumen thereby creating ideal circumstances for pathogen colonization and, consequently, OE.
3.1.2 Mucins
Mucins are large glycoproteins and the main component of mucus, a viscous secretion lining diverse epithelia. Mucins can be actively secreted into the extracellular space or can be membrane-bound, the latter being released after enzymatic cleavage from the plasma membrane (52, 53). Feig et al. demonstrated the presence of several mucins in human cerumen [secreted mucins: Mucin-5B (MUC5B), Mucin-6 (MUC6), Mucin-7 (MUC7); and membrane-bound mucins: Mucin-1 (MUC1), Mucin-16 (MUC16) and Mucin-20 (MUC20)] (54). Among many other functions, mucins serve as a protective layer against pathogens (54, 55).
Histologic evaluation of the intestines of AD-induced mice showed a lowered content of mucins and was the only attempt made so far on the effect of atopic dermatitis on the expression of mucins (56). Additionally, it was shown that oral Pediococcus acidilactici supplementation increased mucin production, but decreased the clinical severity of atopic dermatitis (56). Studying the differences in concentration and structure of certain mucins in the cerumen of allergic patients compared to healthy patients could uncover a new piece of the complex pathogenesis of allergic dermatitis linked to OE and generate possible new insights to disease management.
3.2 Antimicrobial molecules present in cerumen
3.2.1 Antimicrobial lipids
Triglycerides are the primary source of free fatty acids after being hydrolyzed by the action of both bacterial lipases and acid lipases of the host’s skin, and possibly earwax too (57). A smaller part is provided by wax esters and cholesterol esters. Within the group of fatty acids (FA) in human cerumen—determined by Stransky et al. as described above—lauric acid (C12:0), myristic acid (C14:0), linoleic acid (C18:2 n-6), sapienic acid (C16:1 n-1) and palmitoleic acid (C16:1 n-7) have been identified. Several studies confirmed the antibacterial activity of these FA to mainly Gram-positive microorganisms, of which an overview is shown in Table 3. Unfortunately, based on the results described by Stransky et al., it was not possible to calculate the exact concentration of the different FA in cerumen. Therefore, it remains unclear whether the different FA in cerumen, as described by Stransky et al., are present at relevant concentrations to inhibit the growth of certain microorganisms.
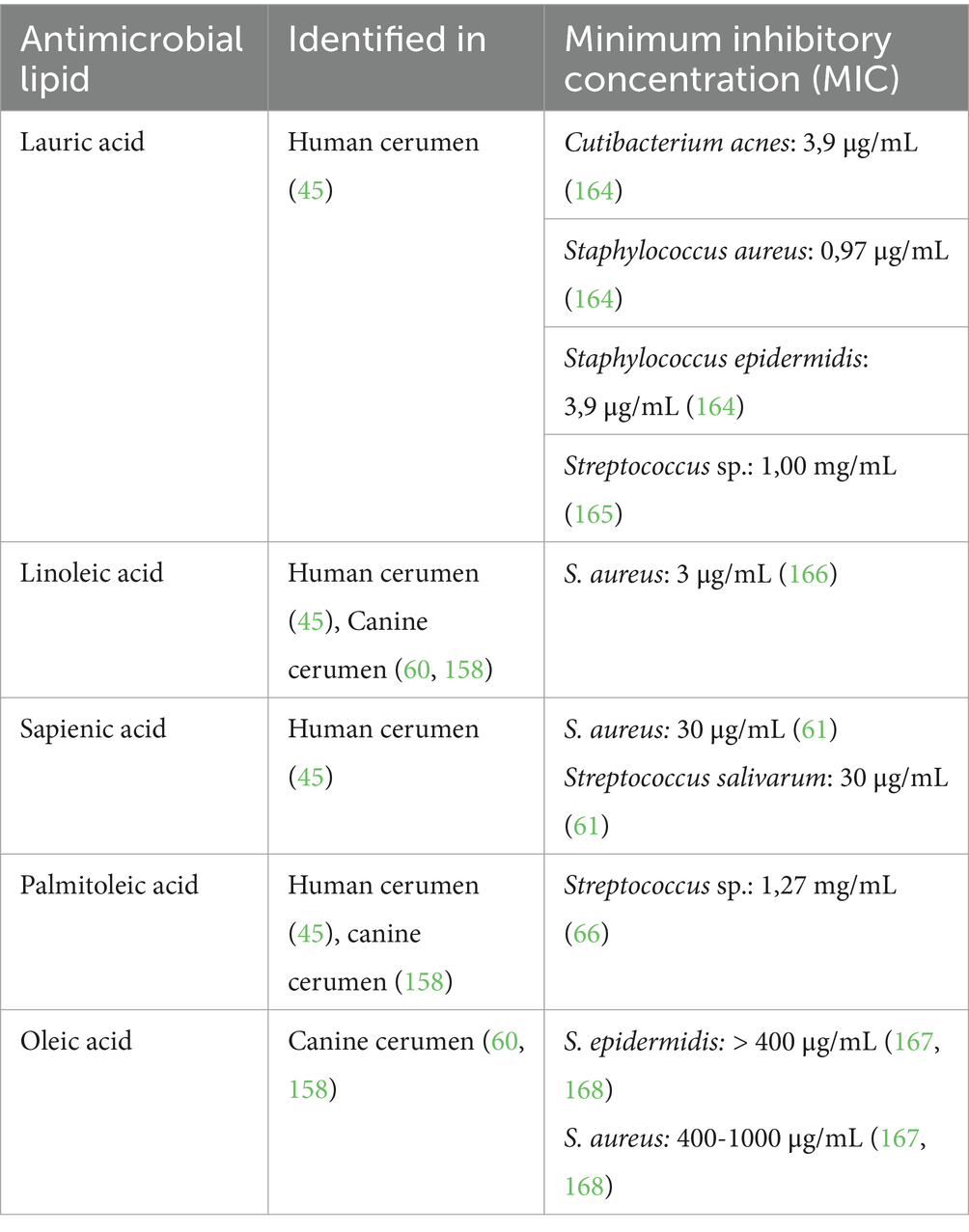
Table 3. Antimicrobial lipids present in cerumen and the minimum inhibitory concentration for skin and/or ear pathogens.
In addition to FA, the stratum corneum of the skin provides another group of lipids with antimicrobial properties: the sphingosine bases. These are formed enzymatically from the ceramides, present in the intercellular spaces of the stratum corneum. Sphingosine bases have a broad antimicrobial activity against various Gram-positive bacteria and fungi (including S. aureus, Streptococcus pyogenes, Micrococcus luteus, Cutibacterium acnes, Brevibacterium epidermidis and Candida albicans) (57, 58).
Using gas liquid chromatography and mass spectrometry, the different FA in 36 healthy canine cerumen samples were further characterized, revealing the presence of antimicrobial FA such as myristic acid (C14:0), palmitic acid (C16:0), palmitoleic acid (C16:1), oleic acid (C18:1) and linoleic acid (C18:2) (59). Due to differences in data reporting, it was not possible to compare the fatty acid profile of human and canine cerumen quantitatively.
Another study used gas chromatography to identify the level of total FA within samples of canine cerumen, but these samples were derived from dogs diagnosed with OE (60). Palmitic acid (C16:0), oleic acid (C18:1) and linoleic acid (C18:2) were present in almost all samples (n = 95).
Sapienic acid (C16:1n10) was the most abundant FA in human cerumen (45) and is also the major FA present in human sebum (57, 61). In canine cerumen, palmitoleic acid (C16:1n6)—an isomer of sapienic acid—is more commonly identified (46, 62). Both isomers have the ability to inhibit the growth of S. aureus (61) but due to the protective effect of staphylococcal oleate hydrolases, S. aureus can counter the inhibitory effect of palmitoleic acid, but not of sapienic acid (62). Based on these observations, human sebum and cerumen may be more antimicrobial toward S. aureus than canine sebum and cerumen. However, in dogs, the most abundant Staphylococcus species on the skin and in the ears is Staphylococcus pseudintermedius, and not S. aureus (42, 63). Whether palmitoleic acid can inhibit the growth of S. pseudintermedius is currently unknown.
Interestingly, oleic acid, identified in canine cerumen in 2 studies as described above (46, 60) was not detected in human earwax in the study by Stranksy et al., even though it was present in human skin sebum as studied by Raghaillaigh et al. (64). Besides its modulatory effect on the immune system, oleic acid is able to increase membrane permeability of S. aureus and subsequently induce bacterial death (65, 66). The reason why this FA has been found in canine cerumen samples and not yet in human samples remains unclear, however, more studies with more samples of both species are needed to confirm or invalidate this difference.
Studies describing the FA composition of equine cerumen were not found.
Several studies have already reported alterations in the skin and sebum lipid composition of human Atopic dermatitis patients compared to healthy individuals. By studying the sebum lipid profile of healthy persons and atopic dermatitis patients, a decrease in C18 and C18:1 ceramides and C18 dihydroceramides was observed (67). Furthermore, compared to healthy skin, a decreased concentration of long chain FA and sphingolipids was observed, caused by the effects of IL-4 and IL-13, involved in the inflammatory reaction in atopic dermatitis patients (68).
3.2.2 Antimicrobial proteins
Several researchers investigated the presence of antimicrobial peptides (AMPs) in human, canine and equine cerumen. Until now, the presence of human beta-defensin (hBD)-1, 2, 3; Human Neutrophil Protein (HNP)-1,2,3; LL-37; Secretory leukocyte protease inhibitor (hSLPI); human bactericidal permeability increasing protein (BPI) and two types of immunoglobulins (IgG and IgA) has been confirmed in cerumen. An overview of the different AMPs identified in human, canine and equine cerumen is shown in Table 4. More details on the function, expression and type of these different AMPs can be found in Supplementary Table 2.
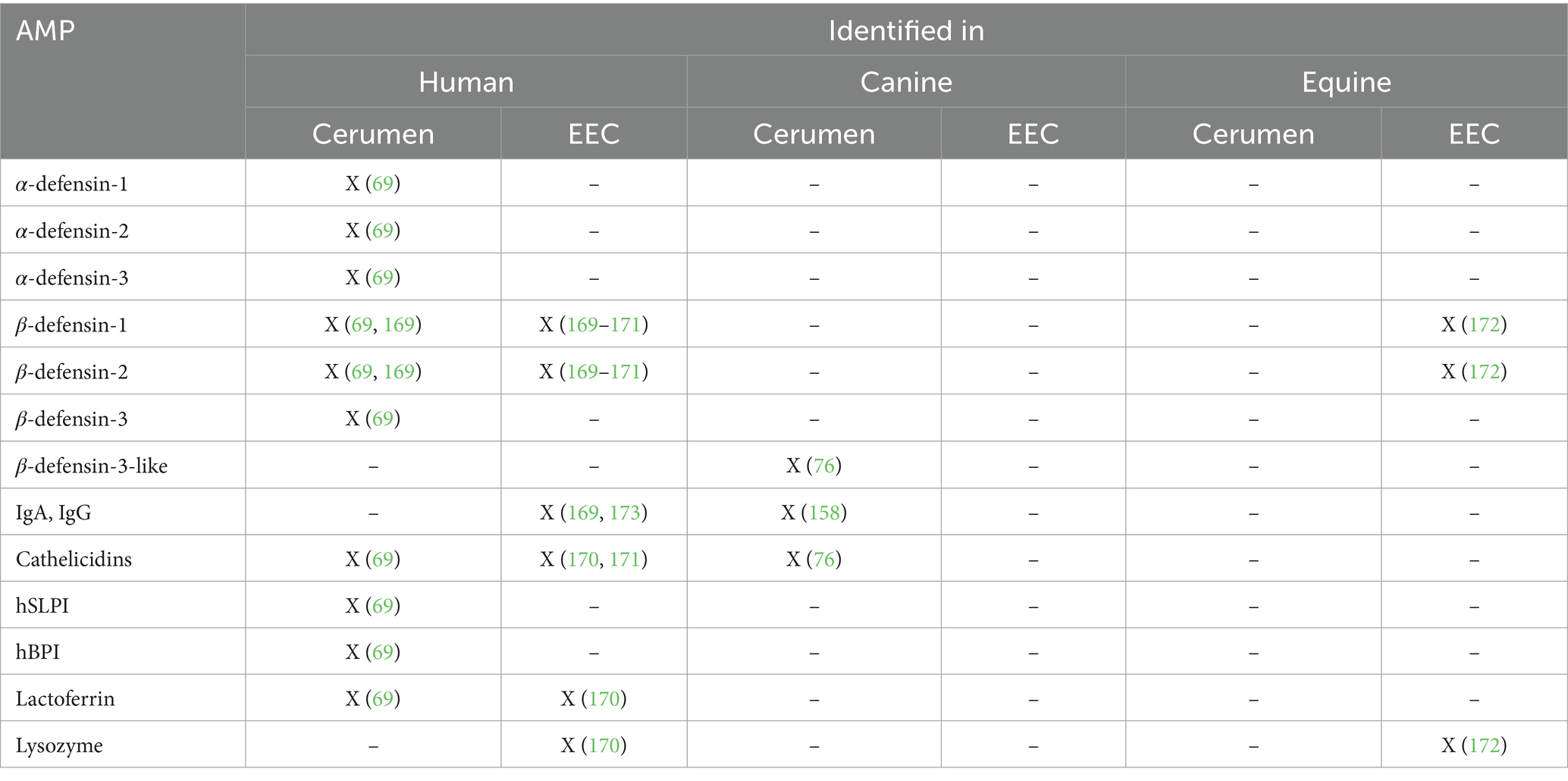
Table 4. Types of proteins with antimicrobial properties (Antimicrobial Proteins, AMP) identified in the cerumen and EEC of humans, dogs and horses.
One could question whether the AMPs described above are actively secreted in earwax or whether their presence is just a result of the presence of exfoliated keratinocytes. Schwaab et al. tried to resolve this question by comparing the cell-bound and non-cell-bound fraction of β-defensin 1,2,3, hSLPI, hBPI and α-defensin-1,2,3. They found that the total concentration of α-defensin-1,2,3 before PBS washing of cerumen was significantly higher than the concentration after PBS washing, confirming that the presence of these proteins in earwax is not exclusively due to the presence of keratinocytes (69). Moreover, when looking at the presence of antimicrobial proteins at the skin surface, α-defensins are usually not expressed by keratinocytes (70). Therefore, further investigation is needed to verify whether the presence of α-defensins in earwax is due to active secretion by the ceruminous glands. β-defensins, LL-37, hSPLI and hBPI are known to be expressed by keratinocytes, so at this point, it is still unclear whether they originate exclusively from the presence of exfoliated keratinocytes in earwax or whether they are actively secreted by ceruminous glands.
The role of AMPs in atopic skin disease was studied frequently in both humans and dogs, retrieving inconsistent results. Depending on the used method, a decreased as well as an increased level of α-defensins, β-defensins and cathelicidin has been observed in lesional and non-lesional atopic skin (71–76). In normal skin, the expression of β-defensin-2, β-defensin-3 and cathelicidin is upregulated during infection and inflammation. Besides the current ambiguous effect of AD on the expression of AMPs, one could question why these AMPs—despite their potential impact on the onset of infection—ultimately fail to prevent infection in AD patients, leading to OE.
First, the antipathogenic effects of the AMPs in the EEC could be negligible relative to the change in lipid concentration and composition of cerumen, the impaired skin barrier and the inflammatory parameters involved in allergic skin disease—as discussed above. Moreover, most treatments for CAD will result in a suppression of the AMP-expression, which could theoretically contribute to the relapse of OE in atopic dogs, treated with systemic anti-inflammatory drugs, such as oclacitinib (77), ciclosporin or lokivetmab (78). Furthermore, Santoro et al. reported a defective secretion of β-defensin-3 and cathelicidin in non-lesional atopic skin after exposure to pathogens, indicating a loss of function due to an increased adhesion of these AMPs to corneocytes (72). Lastly, an induction of AMP-secretion can hypothetically lead to dysbiosis, as the effect of these host defense peptides can be different for each microorganism present in the EEC. To demonstrate, non-bacterial commensals belonging to the genus of Malassezia frequently colonize the EEC of atopic dogs, however, the antipathogenic effect of defensins and cathelicidin on the growth and survival of Malassezia species is currently not reported. Moreover, it seems that Malassezia furfur and M. pachydermatis are able to encourage the secretion of AMPs such as β-defensin-2 (79, 80), thereby strengthening the dysbiosis, possibly leading to colonization and infection of the EEC. However, only M. pachydermatis is currently associated with OE in atopic dogs, indicating that probably additional factors are needed for colonization.
In conclusion, more studies are necessary to evaluate the effect of allergic dermatitis on the cerumen profile; more specifically on how the changes in different (antimicrobial) compounds can contribute to the development of a more optimal environment for certain pathogens such as Staphylococcus species and Malassezia species.
4 Microorganisms associated with the EEC
4.1 Cerumen: barrier or nutrient source for microorganisms
Cerumen is composed of a variety of lipids, proteins and mucins and as such, earwax may potentially serve as an ideal nutrient source for certain bacteria and yeast (81). M. pachydermatis, for instance, commonly isolated from otitic ears in dogs, is dependent on exogenous lipid sources due to the lack of a gene coding for fatty acid synthase in its genome (82). Huang et al. and Masuda et al. showed that M. pachydermatis can use different ceruminous FA for its growth in vitro [myristic acid, palmitic acid, margaric acid, stearic acid, oleic acid and linoleic acid (46, 47, 60)]. As such, cerumen appears to be a good nutrient source for M. pachydermatis in vitro. Whether other microorganisms can benefit from the presence of specific components in cerumen for their growth is unclear for now.
However, as discussed above, based on the high lipid content and the presence of both antimicrobial lipids and proteins, the presence of cerumen in the EEC could also complicate the growth and survival of certain microorganisms. Several studies tried to evaluate the effect of cerumen on the in vitro growth of bacteria. Campos et al. observed an enhancement of the growth of S. aureus, Staphylococcus epidermidis, Proteus mirabilis, E. coli, Serratia marcescens and Pseudomonas aeruginosa by adding a 3% cerumen suspension to bacterial cultures, whereas Chai et al. and Stone and Fulghum observed a decrease in viability of the same bacterial species using the same concentration of cerumen suspension (83–85). However, these studies are hard to compare due to differences in applied culture medium, origin of the samples and sample processing. In a more recent study (86), a cerumen suspension was used to assess the inhibitory effect toward pathogens frequently involved in OE in humans: S. aureus, P. aeruginosa and C. albicans. Again, both an enhancement (which was the case for 4 out of 31 samples for S. aureus; 2 out of 31 samples for C. albicans and 27 out of 31 samples for E. coli) and an inhibition [which was the case for 27 out of 31 samples for S. aureus, 31 out of 31 samples for P. aeruginosa, 29 out of 31 samples for C. albicans and 4 out of 31 samples for E. coli(control)] in growth was observed (86).
Based on the general inhibitory effects of cerumen in vitro as shown in the last study, it can be postulated that etiological factors involved in OE (86) intervene with this antimicrobial effect of cerumen, leading to the colonization of pathogens. To demonstrate, the regular insertion of water in the EEC of swimmers is associated with OE (51). Polluted water is a vehicle for certain pathogens such as P. aeruginosa and other Gram-negative bacteria (87, 88). Yet, additional factors are needed to allow these pathogens to colonize the EEC. For example, the excessive amount of water into the EEC could—in addition to the maceration of the stratum corneum (51)—generate a mixture with a lower lipid content and a dilution of antimicrobial molecules, thereby facilitating the growth and colonization of pathogens.
Lastly, as cerumen reflects the (patho)physiology of the body with genetic material, lipids, proteins, trace elements, internal and external metabolites reaching earwax from the blood circulation (89), it is not yet clear how the general (patho) physiological state of the host may add to the cerumen’s role as barrier or nutrient source for microorganisms or which specific components may be important.
4.2 Studies on the healthy ear microbiome
In the past decades, a few studies tried to identify the microbial community in the healthy EEC of humans, dogs and horses by using culture-dependent methods (26, 39, 59, 90–98). Interestingly, the genera Staphylococcus, Corynebacterium and Bacillus were frequently identified by culture in different studies in humans, dogs and horses. Streptococcus and Micrococcus were found in the EEC of both humans and dogs but not in horses. Malassezia species were identified in humans (Malassezia slooffiae, Malassezia sympodialis, M. furfur and Malassezia obtusa), dogs (M. pachydermatis) and horses (Malassezia nana, M. pachydermatis and Malassezia globosa).
More recently, several studies tried to identify the microorganisms present in the healthy canine EEC using next-generation-sequencing (NGS), a culture-independent method (63, 99–107). Most bacteria identified during these studies belong to the following phyla: Firmicutes, Proteobacteria, Actinobacteria, Bacteroidetes and Fusobacteria. Ascomycota and Basidiomycota were the most abundant phyla for fungi. A recent study suggests that the microbiome of the canine EEC does not significantly differ from the microbiome of the tympanic bulla microbiome in healthy beagle dogs (108). Four NGS-based studies on the microbial community within the human EEC identified Firmicutes and Actinobacteria as the most abundant phyla (109–112). Studies on the microorganisms present in the equine EEC based on NGS data are currently lacking.
In Figure 2, we tried to display the most abundant genera identified in different NGS-based studies on the microbial community in the human and canine EEC, as reported by the respective authors. Yet, as most studies applied different techniques for DNA-extraction, PCR and data processing, this comparison should be interpreted carefully.
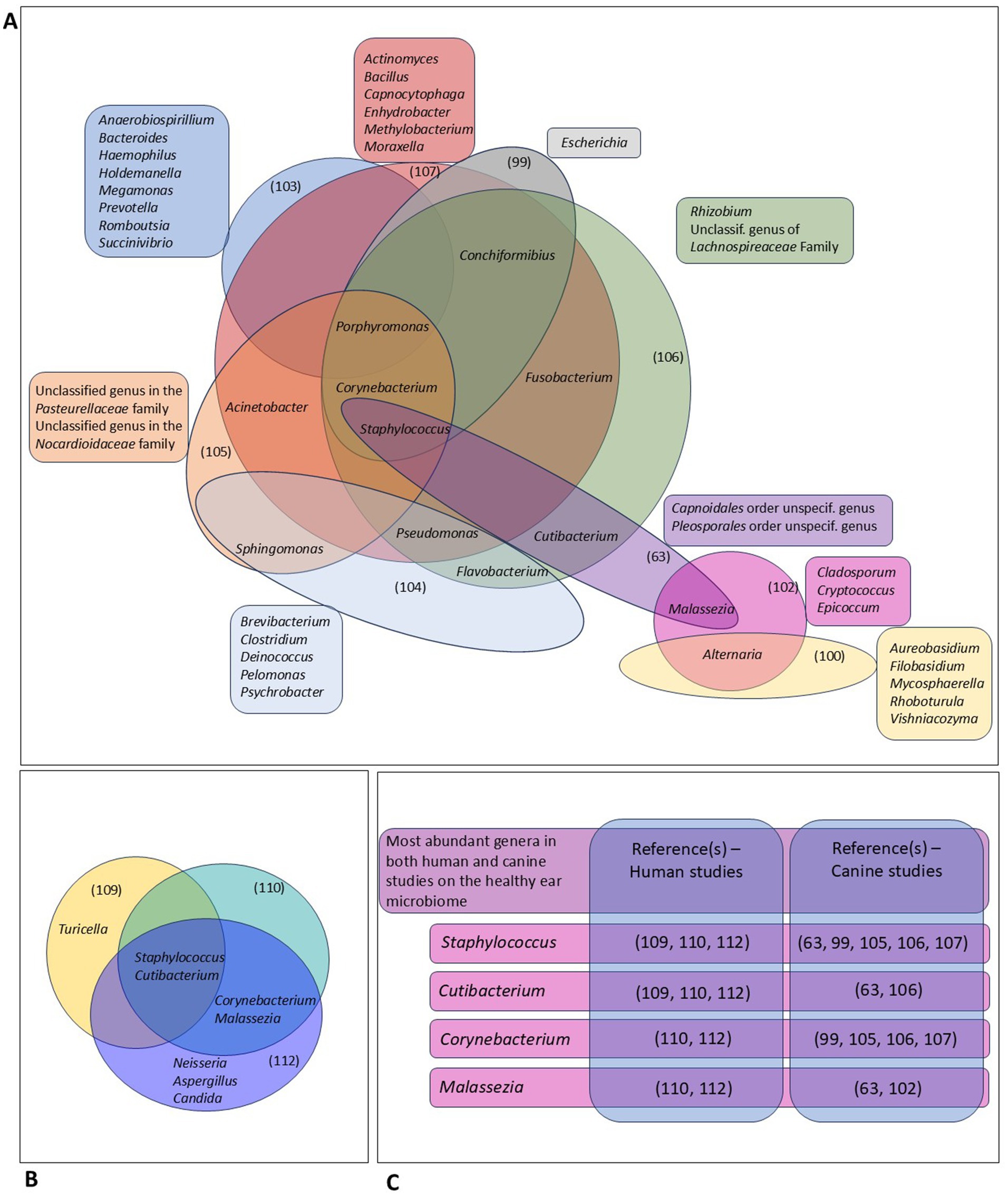
Figure 2. Comparative analysis of different studies on the ear microbiome in dogs and humans using next-generation sequencing (63, 99, 100, 102–107, 109, 110, 112). (A) This panel gives an overview of the most abundant genera in different studies on the canine ear microbiome, as reported by the respective authors. Genera that were among the most abundant genera in the respective study, and not in other studies, are displayed outside the diagram. Genera that were among the most abundant genera in at least 2 different studies are included in the diagram. (B) Comparison of the most abundant genera identified in 2 different studies on the human ear microbiome. (C) This panel shows highly abundant genera identified in both canine and human studies.
In dogs, different genera such as Alternaria, Acinetobacter, Conchiformibius, Corynebacterium, Flavobacterium, Fusobacterium, Porphyromonas and Sphingomonas were identified in at least 2 different studies. Pseudomonas, Staphylococcus and Malassezia—common OE-pathogens—were also frequently identified in healthy canine ears, with Staphylococcus being described in 5 different studies. Still, many genera were only identified in one single study, which can represent the variations in applied techniques and data reporting, differences in breed and/or environment and living circumstances as well as strong individual variability and/or instability of the community of microorganisms present in the outer ear canal.
The genera Staphylococcus and Cutibacterium have been identified in 3 NGS-studies describing the human ear microbiome, whereas Allaiococcus and Malassezia were identified in 2 of the described studies. Interestingly, all genera that were highly abundant in both the human and canine healthy EEC represent facultatively pathogenic bacteria. Staphylococcus and Corynebacterium species are known OE-pathogens in humans and dogs (see Table 1). However, Cutibacterium and Malassezia are, so far, only associated with human and canine OE, respectively. In our opinion, species-specific differences in anatomy and physiology of the EEC, the composition of cerumen, underlying conditions as well as microenvironmental factors, may account—at least partly—for the capability of lipophilic Cutibacterium to colonize the human and not the canine EEC. The association of the genus Malassezia with canine OE only might be related to similar variations, parallel to differences in pathogenic features of the dog specific M. pachydermatis, compared to the Malassezia species identified in human ear canals.
Based on present data, it may be interesting to consider how these bacteria and other microorganisms are able to survive and grow in the microenvironment of the EEC. In other words, which of the microorganisms identified in the studies described above, are just passing by and which species have specifically developed certain strategies to survive, grow, and even colonize in this microenvironment? Recently, the interest in the human and canine ear microbiome is increasing and new, culture-independent methods offer the ability to obtain a more thorough understanding of this microbiome. However, NGS cannot easily distinguish between viable and dead microorganisms, as it is based on the presence of bacterial DNA at a certain sampling moment. Moreover, the studies described above used different techniques for sample collection, DNA extraction and data analysis, which makes it hard to compare the results. Ear samples may—similar to skin samples—be considered as low-biomass samples, which require caution during processing to avoid contamination. Recent recommendations for obtaining reliable results using 16S rRNA sequencing on low-biomass-samples suggest a minimal concentration of 106 bacteria per sample and an improved protocol for DNA extraction and PCR (113). Unfortunately—to the best of our knowledge—no quantitative data are available on the total number of bacteria present in the ear samples analyzed by NGS in the different studies investigating the ear microbiome.
Lastly, until now, no information is available yet on the microbiome-host interactions and the potential benefits of the presence of a microbiome in the EEC. We conclude that—at this moment—the existence, complexity and stability of an ear microbiome has not been sufficiently demonstrated in available studies and needs further research.
4.3 Effect of allergic dermatitis on the microorganisms in the EEC
The skin of atopic humans is defined by a decrease in bacterial diversity, richness and evenness and a change in skin microbiome composition, with higher abundances of Staphylococcus species (112, 114) and Cutibacterium species (112). The use of artificial intelligence and machine learning may aid in the interpretation of complex metagenomics and even transcriptomics data to differentiate between healthy and atopic dermatitis patients (115). Whether this dysbiosis is a cause or result of atopic dermatitis is currently unknown. As the pathogenesis of human and CAD is very similar (116), CAD can potentially affect the colonization capabilities of facultative pathogens in the EEC as well. A few studies tried to compare the microbial composition and diversity of the EEC of healthy and atopic dogs (99, 102, 104), however, a significant decrease in microbial diversity in the ears of atopic dogs compared to healthy dogs was not demonstrated so far. Nonetheless, some interesting differences between healthy and atopic ears were observed, such as an increase in the abundance of the genera Staphylococcus, Sphingomonas, Ralstonia, Methylotenera, Lactobacillus, Hymenobacter, Chryseobacterium, Alcaligenes and Malassezia and a decrease in the abundance of the genera Macrococcus, Brevibacterium and Escherichia in the EEC of atopic dogs compared to healthy individuals. It was recently described that 16S amplicon profiling appears to be a more sensitive method to describe bacterial populations in canine otitis cases compared with conventional culture-dependent methods (117).
Studies on the effect of CD in humans and IBH in horses on the microbial communities in the EEC of these species are currently lacking.
5 The development of allergic dermatitis and OE: impact of neonatal events and the gut and skin microbiome
Although one of the main topics of the current review is the effect of the characteristic changes of AD on the ear microbiome to better understand the development of OE in AD patients, it is intriguing to discuss another possible correlation between the microbiome, allergic disease and OE. Recent insights point toward the importance of the early-life (gut) microbiome as an important factor in the development of allergic disease in humans (12, 118, 119) and dogs and cats (120).
Without going into detail, it has been suggested that prenatal and early-life postnatal events that may interfere with the establishment of the child’s microbiome, such as diet, antimicrobial exposure, environmental bacterial load and early-life cytomegalovirus infection, may increase the risk of allergic dermatitis (121–125) and even otitis media (126) in these children or dogs (127). It was recently even suggested that artificial intelligence-supported analysis of the gut microbiota may be used to accurately diagnose atopic dermatitis in humans (115).
In addition, the auditory-gut-brain axis has very recently become a topic of research interest, suggesting that connections have been found between the external ear, the central auditory system and the gut microbiome (128). An interesting study found that colonization of the gut with Candida albicans in mice was associated with the presence of pro-inflammatory cytokines in the ear (129). Furthermore, inflammatory bowel disease in humans is associated with hearing loss in autoimmune inner ear disease and Cogan’s syndrome (130).
To our knowledge, the correlation between OE and prenatal/early postnatal disruption of the skin, ear or gut microbiome has not yet been studied in humans or animals, but could be included in future epidemiologic surveys to further understand the risk factors associated with the development of OE.
6 The effect of OE prevention and treatment on the ear microbiome
While allergic dermatitis and the presence of OE can have a significant effect on the microbial community of the EEC, also preventive interventions or OE treatments, often including the use of agents with antimicrobial properties (8, 131), may affect the ear microbiome. Aqueous canine otic treatment products aim to approximate the pH range of a healthy dog’s ear in order to be less likely to disrupt the micro-environment of the EEC (132) or may even be used to re-acidify it (133). Preventive use of a topical anti-inflammatory glucocorticoid in atopic dogs without clinical signs of otitis does not seem to affect the ear canal microbiota and mycobiota (134). Treatment of dogs with OE with an anti-inflammatory pomegranate otic treatment mainly had an effect on the mycobiome with markedly lowered M. pachydermatis presence after treatment (135). Chermprapai et al. (136) showed that a topical antimicrobial treatment increased the diversity of bacterial and fungal compositions in course of time on both AD and healthy skin in dogs. Matsui et al. (137) showed that doxycycline not only inhibited Staphylococcus aureus strains isolated from skin lesions of patients with atopic dermatitis in a mouse model, but also had a strong inhibitory effect on epidermal Langerhans cells and Th2 cell development, thereby suppressing the acute inflammation.
Even though probiotics are currently being considered for the prevention of otitis media in human medicine, no similar attempts have yet been reported in either human or veterinary medicine for otitis externa to the best of our knowledge (138–140). On the other hand, the microbiome has been suggested as a potential early-life target to prevent the development of allergic dermatitis and may therefore also indirectly add to the prevention of AD-related complications, such as otitis externa (12).
7 Discussion
This review provides a cross-species comparison of the current knowledge on the EEC, revealing structural and physiological differences between humans, dogs and horses. The relevance of these species-specific differences is highlighted by the example of atopic dermatitis, which manifests differently in humans and dogs, despite a similar pathophysiology. In humans, atopic dermatitis shows a low prevalence of otitis externa (OE), whereas in dogs, it shows a high prevalence. This review attempts to explain this difference in predisposition by identifying differences in anatomy, lipid composition and differences in the composition of the potential ear microbiome (141).
Additionally, this review compiles, for the first time to the author’s knowledge, studies on the molecular composition of cerumen in humans, dogs and horses, identifying interesting species-specific differences. Cerumen is known to trap microorganisms and protect the epithelium, thereby preventing adhesion and exerting direct antimicrobial effects through molecules such as oleic acid and antimicrobial proteins. Together, these findings indicate that the EEC creates a hostile environment for microbes across all observed host species.
Given the different prevalence of allergy-induced OE in at least three mammalian species, this review aimed to identify key factors in the development of OE in patients with allergic dermatitis. In cases of OE, certain pathogens, such as Staphylococcus and Malassezia species, manage to survive and multiply within the hostile environment of the EEC, as various. It is hypothesized that in allergic dermatitis-related OE, the pathophysiological processes may counteract the antimicrobial properties of the EEC. Studies suggest that allergic dermatitis impacts skin parameters such as pH, lipid content, and the presence of antimicrobial molecules. Nevertheless, more research is needed to understand the effect of allergic dermatitis on the EEC and to unravel the link between allergic dermatitis and OE.
Recently, there has been increasing interest in investigating the existence of an ear microbiome, suggesting that microorganisms can also survive and multiply in a healthy ear canal. Currently, little evidence exists on which species can counter the antimicrobial features of cerumen and form a stable, balanced ear microbiome. Although inter-and intra-species comparisons of available studies on the ear microbiome are challenging, it appears that the few species identified across most studies are facultative pathogenic micro-organisms associated with ear infections. Considering the low biomass of ear samples and the associated challenges related to appropriate experimental set-up and interpretation of results, there is still a need for high quality ear microbiome studies both in human and veterinary medicine.
The microbiome has been suggested as a potential target for treatment and prevention of OE. However, the impact of the microbiome—including the ear microbiome and the gut microbiome (“auditory-gut-brain axis”)—on the development and persistence of ear infections such as OE, otitis media, and otitis interna requires further investigation.
In conclusion, this review identifies several gaps in the research on the unique environment within the EEC in three mammalian species with known allergic dermatitis-related prevalence of OE. More research, combining known and yet-to-be-explored processes in different species using a ‘One Health’ approach, could provide exciting new insights. These insights may lead to improved prevention and therapeutic options in both human and veterinary medicine.
Author contributions
CH: Writing – original draft, Writing – review & editing, Conceptualization, Investigation. FP: Investigation, Supervision, Validation, Writing – review & editing, Conceptualization. IC: Investigation, Supervision, Validation, Writing – review & editing. SV: Investigation, Writing – review & editing. FH: Investigation, Validation, Writing – review & editing. SL: Writing – review & editing. FB: Investigation, Supervision, Validation, Writing – review & editing, Conceptualization.
Funding
The author(s) declare that no financial support was received for the research, authorship, and/or publication of this article.
Conflict of interest
IC is Chief Scientific Officer at YUN N.V., a Belgian biotech company researching and commercializing the use of probiotic lactobacilli. CH was employed by YUN N.V.
The remaining authors declare that the research was conducted in the absence of any commercial or financial relationships that could be construed as a potential conflict of interest.
Publisher’s note
All claims expressed in this article are solely those of the authors and do not necessarily represent those of their affiliated organizations, or those of the publisher, the editors and the reviewers. Any product that may be evaluated in this article, or claim that may be made by its manufacturer, is not guaranteed or endorsed by the publisher.
Supplementary material
The Supplementary material for this article can be found online at: https://www.frontiersin.org/articles/10.3389/fvets.2024.1413684/full#supplementary-material
Abbreviations
EEC, External Ear Canal; OE, Otitis Externa; AD, Allergic dermatitis; CD, Contact dermatitis; IBH, Insect-Bite-Hypersensitivity; CAD, Canine atopic dermatitis; Th, T-helper cell; FA, Fatty acid; AMP, Antimicrobial peptides; MIC, Minimum inhibitory concentration.
References
1. Rowlands, S, Devalia, H, Smith, C, Hubbard, R, and Dean, A. Otitis externa in UK general practice: a survey using the UK general practice research database. Br J Gen Pract. (2001) 51:533.
2. Sander, R. Otitis externa: a practical guide to treatment and prevention. Am Fam Physician. (2001) 63:941–2.
3. Paterson, S. Topical ear treatment - options, indications and limitations of current therapy. J Small Anim Pract. (2016) 57:668–78. doi: 10.1111/jsap.12583
4. Gotthelf, LN. Diagnosis and treatment of otitis media in dogs and cats. Vet Clin North America-Small Animal Prac. (2004) 34:469–87. doi: 10.1016/j.cvsm.2003.10.007
5. Odelros, E, Kendall, A, Wulcan, S, and Bergvall, K. Otitis externa in eight horses – clinical signs, treatment and prognosis. Vet Dermatol. (2019) 30:430. doi: 10.1111/vde.12763
6. Beers, S, and Abramo, T. Otitis externa review. Pediatr Emerg Care. (2004) 20:250–4. doi: 10.1097/01.pec.0000121246.99242.f5
7. Sood, S, Strachan, DR, Tsikoudas, A, and Stables, GI. Allergic otitis externa. Clin Otolaryngol Allied Sci. (2002) 27:233–6. doi: 10.1046/j.1365-2273.2002.00584.x
8. Nuttall, T. Managing recurrent otitis externa in dogs: what have we learned and what can we do better? J Am Vet Med Assoc. (2023) 261:S10–22. doi: 10.2460/javma.23.01.0002
9. Heward, E, Cullen, M, and Hobson, J. Microbiology and antimicrobial susceptibility of otitis externa: a changing pattern of antimicrobial resistance. J Laryngol Otol. (2018) 132:314–7. doi: 10.1017/S0022215118000191
10. Mohammed, H, Mather, MW, Lumb, J, Butler, CC, and Wilson, JA. Otitis externa: what is the problem with getting it right? A mixed-methods study in primary and secondary care. J Laryngol Otol. (2022) 136:486–91. doi: 10.1017/S0022215121003649
11. Lebeer, S, Oerlemans, EFM, Claes, I, Henkens, T, Delanghe, L, Wuyts, S, et al. Selective targeting of skin pathobionts and inflammation with topically applied lactobacilli. Cell Rep Med. (2022) 3. doi: 10.1016/j.xcrm.2022.100521
12. Kloepfer, KM, McCauley, KE, and Kirjavainen, PV. The microbiome as a gateway to prevention of allergic disease development. J Allergy Clin Immunol Pract. (2022) 10:2195–204. doi: 10.1016/j.jaip.2022.05.033
13. Santoro, D, Fagman, L, Zhang, Y, and Fahong, Y. Clinical efficacy of spray-based heat-treated lactobacilli in canine atopic dermatitis: a preliminary, open-label, uncontrolled study. Vet Dermatol. (2021) 32:114–e23. doi: 10.1111/vde.12915
14. Nassau, S, and Fonacier, L. Allergic contact dermatitis. Med Clin North Am. (2020) 104:61–76. doi: 10.1016/j.mcna.2019.08.012
15. Girolomoni, G, de Bruin-Weller, M, Aoki, V, Kabashima, K, Deleuran, M, Puig, L, et al. Nomenclature and clinical phenotypes of atopic dermatitis. Ther Adv Chronic Dis. (2021) 12, 1–20. doi: 10.1177/20406223211002979
16. Langan, SM, Irvine, AD, and Weidinger, S. Atopic dermatitis. Lancet. (2020) 396:345–60. doi: 10.1016/S0140-6736(20)31286-1
17. Halliwell, R. Revised nomenclature for veterinary allergy. Vet Immunol Immunopathol. (2006) 114:207–8. doi: 10.1016/j.vetimm.2006.08.013
18. Marsella, R, and De Benedetto, A. Atopic dermatitis in animals and people: an update and comparative review. Vet Sci. (2017) 4:37. doi: 10.3390/vetsci4030037
19. Saridomichelakis, MN, Farmaki, R, Leontides, LS, and Koutinas, AF. Aetiology of canine otitis externa: a retrospective study of 100 cases. Vet Dermatol. (2007) 18:341–7. doi: 10.1111/j.1365-3164.2007.00619.x
20. Perry, ET, and Shelley, WB. The histology of the human ear canal with special reference to the. J Invest Dermatol. (1955) 25:439–51. doi: 10.1038/jid.1955.149
21. Main, T, and Lim, D. The human external auditory canal secretory system: an ultrastructural study. Laryngoscope. (1976) 86:1164–76. doi: 10.1288/00005537-197608000-00008
22. Hanger, HC, and Mulley, GP. Cerumen: its fascination and clinical importance: a review. J R Soc Med. (1992) 85:346–9.
23. Alvord, LS, and Farmer, BL. Anatomy and orientation of the human external ear. J Am Acad Audiol. (1997) 8:383–90.
24. Harvey, RG, Delauche, AJ, and Joseph, Harari. “The Normal ear.,” ear diseases of the dog and cat. London: Manson/Veterinary Press (2001). p. 9–40
25. Heine, PA. Anatomy of the ear. Vet Clin North America-Small Animal Prac. (2004) 34:379–95. doi: 10.1016/j.cvsm.2003.10.003
26. Sargent, SJ, Frank, LA, Buchanan, BR, Donnell, RL, and Morandi, F. Otoscopic, cytological, and microbiological examination of the equine external ear canal. Vet Dermatol. (2006) 17:175–81. doi: 10.1111/j.1365-3164.2006.00515.x
27. Sommerauer, S, Muelling, CKW, Seeger, J, and Schusser, GF. Anatomy and anaesthesia of the equine external ear canal. J Vet Med Series C: Anatomia Histologia Embryologia. (2012) 41:395–401. doi: 10.1111/j.1439-0264.2012.01148.x
28. Netter, FH. “Ear.,” atlas of human anatomy E-book: Digital EBook. Philadelphia: Elsevier (2018). p. 120–121
29. Zhang, S. The ear In: Springer Science & Business Media, editor. An atlas of histology. New York, NY: Springer New York (1999). 393–403.
30. Mozaffari, M, Nash, R, and Tucker, AS. Anatomy and development of the mammalian external Auditory Canal: implications for Understanding Canal disease and deformity. Front Cell Dev Biol. (2021) 8:1760. doi: 10.3389/fcell.2020.617354
31. Imokawa, G, Abe, A, Jin, K, Higaki, Y, Kawashima, M, and Hidano, A. Decreased level of ceramides in stratum Corneum of atopic dermatitis: an etiologic factor in atopic dry skin? J Invest Dermatol. (1991) 96:523–6. doi: 10.1111/1523-1747.ep12470233
32. Janssens, M, Van Smeden, J, Gooris, GS, Bras, W, Portale, G, Caspers, PJ, et al. Increase in short-chain ceramides correlates with an altered lipid organization and decreased barrier function in atopic eczema patients. J Lipid Res. (2012) 53:2755–66. doi: 10.1194/jlr.P030338
33. Chermprapai, S, Broere, F, Gooris, G, Schlotter, Y, Rutten, V, and Bouwstra, J. Altered lipid properties of the stratum corneum in canine atopic dermatitis. Biochim Biophys Acta Biomembr. (2018) 1860:526–33. doi: 10.1016/j.bbamem.2017.11.013
34. Franco, J, Rajwa, B, Gomes, P, and Hogenesch, H. Local and systemic changes in lipid profile as potential biomarkers for canine atopic dermatitis. Meta. (2021) 11:670. doi: 10.3390/METABO11100670
35. Baurecht, H, Irvine, AD, Novak, N, Illig, T, Bühler, B, Ring, J, et al. Toward a major risk factor for atopic eczema: Meta-analysis of filaggrin polymorphism data. J Allergy Clin Immunol. (2007) 120:1406–12. doi: 10.1016/j.jaci.2007.08.067
36. De Benedetto, A, Rafaels, NM, McGirt, LY, Ivanov, AI, Georas, SN, Cheadle, C, et al. Tight junction defects in patients with atopic dermatitis. J Allergy Clin Immunol. (2011) 127:773–786.e7. doi: 10.1016/j.jaci.2010.10.018
37. Levander, MS, and Grodzinsky, E. Variation in Normal ear temperature. Am J Med Sci. (2017) 354:370–8. doi: 10.1016/j.amjms.2017.05.013
38. Huang, HP, and Huang, H. Effect of ear type, sex, age, body weight, and climate on temperatures in the external acoustic meatus of dogs. Am J Vet Res. (1999) 60:1173–6. doi: 10.2460/ajvr.1999.60.09.1173
39. Yoshida, N, Naito, F, and Fukata, T. Studies of certain factors affecting the microenvironment and microflora of the external ear of the dog in health and disease. J Vet Med Sci. (2002) 64:1145–7. doi: 10.1292/jvms.64.1145
40. Proksch, E. pH in nature, humans and skin. J Dermatol. (2018) 45:1044–52. doi: 10.1111/1346-8138.14489
41. Lambers, H, Piessens, S, Bloem, A, Pronk, H, and Finkel, P. Natural skin surface pH is on average below 5, which is beneficial for its resident flora. Int J Cosmet Sci. (2006) 28:359–70. doi: 10.1111/j.1467-2494.2006.00344.x
42. Bradley, CW, Morris, DO, Rankin, SC, Cain, CL, Misic, AM, Houser, T, et al. Longitudinal evaluation of the skin microbiome and association with microenvironment and treatment in canine atopic dermatitis. J Invest Dermatol. (2016) 136:1182–90. doi: 10.1016/j.jid.2016.01.023
43. Rippke, F, Schreiner, V, Doering, T, and Maibach, HI. Stratum corneum pH in atopic dermatitis: impact on skin barrier function and colonization with Staphylococcus Aureus. Am J Clin Dermatol. (2004) 5:217–23. doi: 10.2165/00128071-200405040-00002
44. Bortz, JT, Wertz, PW, and Downing, DT. Composition of cerumen lipids. J Am Acad Dermatol. (1990) 23:845–9. doi: 10.1016/0190-9622(90)70301-W
45. Stránský, K, Valterová, I, Kofroňová, E, Urbanová, K, Zarevúcka, M, and Wimmer, Z. Non-polar lipid components of human cerumen. Lipids. (2011) 46:781–8. doi: 10.1007/s11745-011-3564-y
46. Huang, HP, Fixter, LM, and Little, CJ. Lipid content of cerumen from normal dogs and otitic canine ears. Vet Rec. (1994) 134:380–1. doi: 10.1136/vr.134.15.380
47. Huang, HP, Little, CJ, and Fixter, LM. Effects of fatty acids on the growth and composition of Malassezia pachydermatis and their relevance to canine otitis externa. Res Vet Sci. (1993) 55:119–23. doi: 10.1016/0034-5288(93)90045-H
48. Marsella, R, Olivry, T, and Carlotti, D-N. International task force on canine atopic dermatitis. Current evidence of skin barrier dysfunction in human and canine atopic dermatitis. Vet Dermatol. (2011) 22:239–48. doi: 10.1111/j.1365-3164.2011.00967.x
49. Knox, S, and O’Boyle, NM. Skin lipids in health and disease: a review. Chem Phys Lipids. (2021) 236:105055. doi: 10.1016/j.chemphyslip.2021.105055
50. Cvitas, I, Oberhänsli, S, Leeb, T, Dettwiler, M, Müller, E, Bruggman, R, et al. Investigating the epithelial barrier and immune signatures in the pathogenesis of equine insect bite hypersensitivity. PLoS One. (2020) 15:e0232189. doi: 10.1371/journal.pone.0232189
51. Wang, MC, Liu, CY, Shiao, AS, and Wang, T. Ear problems in swimmers. J Chin Med Assoc. (2005) 68:347–52. doi: 10.1016/S1726-4901(09)70174-1
52. Senapati, S, Das, S, and Batra, SK. Mucin-interacting proteins: from function to therapeutics. Trends Biochem Sci. (2010) 35:236–45. doi: 10.1016/j.tibs.2009.10.003
53. Roussel, P, Lamblin, G, Lhermitte, M, Houdret, N, Lafitte, JJ, Perini, JM, et al. The complexity of mucins. Biochimie. (1988) 70:1471–82. doi: 10.1016/0300-9084(88)90284-2
54. Feig, MA, Hammer, E, Völker, U, and Jehmlich, N. In-depth proteomic analysis of the human cerumen-a potential novel diagnostically relevant biofluid. J Proteome. (2013) 83:119–29. doi: 10.1016/j.jprot.2013.03.004
55. Bansil, R, and Turner, BS. Mucin structure, aggregation, physiological functions and biomedical applications. Curr Opin Colloid Interface Sci. (2006) 11:164–70. doi: 10.1016/j.cocis.2005.11.001
56. Jeong, DY, Ryu, MS, Yang, HJ, Jeong, SY, Zhang, T, Yang, HJ, et al. Pediococcus acidilactici intake decreases the clinical severity of atopic dermatitis along with increasing mucin production and improving the gut microbiome in Nc/Nga mice. Biomed Pharmacother. (2020) 129:110488. doi: 10.1016/j.biopha.2020.110488
57. Drake, DR, Brogden, KA, Dawson, DV, and Wertz, PW. Thematic review series: skin lipids-antimicrobial lipids at the skin surface. J Lipid Res. (2008) 49:4–11. doi: 10.1194/jlr.R700016-JLR200
58. Wertz, PW. Lipids and the permeability and antimicrobial barriers of the skin. J Lipids. (2018) 2018:1–7. doi: 10.1155/2018/5954034
59. Huang, H-P. Studies of the microenvironment and microflora of the canine external ear canal. PhD thesis, University of Glasgow. (1993).
60. Masuda, A, Sukegawa, T, Mizumoto, N, Tani, H, Miyamoto, T, Sasai, K, et al. Study of lipid in the ear canal in canine otitis externa with Malassezia pachydermatis. J Vet Med Sci. (2000) 62:1177–82. doi: 10.1292/jvms.62.1177
61. Wille, JJ, and Kydonieus, A. Palmitoleic acid isomer (C16:1Δ6) in human skin sebum is effective against gram-positive bacteria. Skin Pharmacol Appl Ski Physiol. (2003) 16:176–87. doi: 10.1159/000069757
62. Subramanian, C, Frank, MW, Batte, JL, Whaley, SG, and Rock, CO. Oleate hydratase from Staphylococcus aureus protects against palmitoleic acid, the major antimicrobial fatty acid produced by mammalian skin. J Biol Chem. (2019) 294:9285–94. doi: 10.1074/jbc.RA119.008439
63. Tang, S, Prem, A, Tjokrosurjo, J, Sary, M, Van Bel, MA, Rodrigues-Hoffmann, A, et al. The canine skin and ear microbiome: a comprehensive survey of pathogens implicated in canine skin and ear infections using a novel next-generation-sequencing-based assay. Vet Microbiol. (2020) 247:108764. doi: 10.1016/j.vetmic.2020.108764
64. Ní Raghallaigh, S, Bender, K, Lacey, N, Brennan, L, and Powell, FC. The fatty acid profile of the skin surface lipid layer in papulopustular rosacea. Br J Dermatol. (2012) 166:279–87. doi: 10.1111/j.1365-2133.2011.10662.x
65. Sales-Campos, H, Reis de Souza, P, Crema Peghini, B, Santana da Silva, J, and Ribeiro, CC. An overview of the modulatory effects of oleic acid in health and disease. Mini-Rev Med Chem. (2013) 13:201–10.
66. Yoon, BK, Jackman, JA, Valle-González, ER, and Cho, NJ. Antibacterial free fatty acids and monoglycerides: biological activities, experimental testing, and therapeutic applications. Int J Mol Sci. (2018) 19:1114. doi: 10.3390/ijms19041114
67. Agrawal, K, Hassoun, LA, Foolad, N, Borkowski, K, Pedersen, TL, Sivamani, RK, et al. Effects of atopic dermatitis and gender on sebum lipid mediator and fatty acid profiles. Prostaglandins Leukot Essent Fat Acids. (2018) 134:7–16. doi: 10.1016/j.plefa.2018.05.001
68. Berdyshev, E, Goleva, E, Bronova, I, Dyjack, N, Rios, C, Jung, J, et al. Lipid abnormalities in atopic skin are driven by type 2 cytokines. JCI Insight. (2018) 3:e98006. doi: 10.1172/jci.insight.98006
69. Schwaab, M, Gurr, A, Neumann, A, Dazert, S, and Minovi, A. Human antimicrobial proteins in ear wax. Eur J Clin Microbiol Infect Dis. (2011) 30:997–1004. doi: 10.1007/s10096-011-1185-2
70. Gallo, RL, and Hooper, LV. Epithelial antimicrobial defence of the skin and intestine. Nat Rev Immunol. (2012) 12:503–16. doi: 10.1038/nri3228
71. Suwanchote, S, Waitayangkoon, P, Chancheewa, B, Inthanachai, T, Niwetbowornchai, N, Edwards, SW, et al. Role of antimicrobial peptides in atopic dermatitis. Int J Dermatol. (2021) 61:532–40. doi: 10.1111/IJD.15814
72. Santoro, D, Archer, L, and Kelley, K. A defective release of host defense peptides is present in canine atopic skin. Comp Immunol Microbiol Infect Dis. (2019) 65:65–9. doi: 10.1016/j.cimid.2019.04.008
73. Santoro, D, Marsella, R, Bunick, D, Graves, TK, and Campbell, KL. Expression and distribution of canine antimicrobial peptides in the skin of healthy and atopic beagles. Vet Immunol Immunopathol. (2011) 144:382–8. doi: 10.1016/j.vetimm.2011.08.004
74. Clausen, ML, Slotved, HC, Krogfelt, KA, Andersen, PS, and Agner, T. In vivo expression of antimicrobial peptides in atopic dermatitis. Exp Dermatol. (2016) 25:3–9. doi: 10.1111/exd.12831
75. Asahina, R, and Maeda, S. A review of the roles of keratinocyte-derived cytokines and chemokines in the pathogenesis of atopic dermatitis in humans and dogs. Vet Dermatol. (2017) 28:16–e5. doi: 10.1111/vde.12351
76. Santoro, D. Comparison of the quantity and antimicrobial activity of host defence peptides in ear canals between healthy and atopic dogs: a preliminary study. Vet Dermatol. (2023) 34:452–9. doi: 10.1111/vde.13164
77. Denti, D, Caldin, M, Ventura, L, and De Lucia, M. Prolonged twice-daily administration of oclacitinib for the control of canine atopic dermatitis: a retrospective study of 53 client-owned atopic dogs. Vet Dermatol. (2022) 33:149–e42. doi: 10.1111/vde.13053
78. Moyaert, H, Van Brussel, L, Borowski, S, Escalada, M, Mahabir, SP, Walters, RR, et al. A blinded, randomized clinical trial evaluating the efficacy and safety of lokivetmab compared to ciclosporin in client-owned dogs with atopic dermatitis. Vet Dermatol. (2017) 28:593–e145. doi: 10.1111/vde.12478
79. Buommino, E, De Filippis, A, Parisi, A, Nizza, S, Martano, M, Iovane, G, et al. Innate immune response in human keratinocytes infected by a feline isolate of Malassezia pachydermatis. Vet Microbiol. (2013) 163:90–6. doi: 10.1016/j.vetmic.2012.12.001
80. Donnarumma, G, Paoletti, I, Buommino, E, Orlando, M, Tufano, MA, and Baroni, A. Malassezia furfur induces the expression of beta-defensin-2 in human keratinocytes in a protein kinase C-dependent manner. Arch Dermatol Res. (2004) 295:474–81. doi: 10.1007/s00403-003-0445-0
81. Gerchman, Y, Patichov, R, and Zeltzer, T. Lipolytic, proteolytic, and cholesterol-degrading bacteria from the human cerumen. Curr Microbiol. (2012) 64:588–91. doi: 10.1007/s00284-012-0113-8
82. Puig, L, Bragulat, MR, Castellá, G, and Cabañes, FJ. Characterization of the species Malassezia pachydermatis and re-evaluation of its lipid dependence using a synthetic agar medium. PLoS One. (2017) 12:e0179148. doi: 10.1371/journal.pone.0179148
83. Chai, T-J, and Chai, TC. Bactericidal activity of cerumen. Antimicrob Agents Chemother. (1980) 18:638–41. doi: 10.1128/AAC.18.4.638
84. Stone, M, and Fulghum, RS. Bactericidal activity of wet cerumen. Ann Otol Rhinol Laryngol. (1984) 93:183–6. doi: 10.1177/000348948409300217
85. Campos, A, Betancor, L, Arias, A, Rodríguez, C, Hernández, AM, López Aguado, D, et al. Influence of human wet cerumen on the growth of common and pathogenic bacteria of the ear. J Laryngol Otol. (2000) 114:925–9. doi: 10.1258/0022215001904635
86. Lum, CL, Jeyanthi, S, Prepageran, N, Vadivelu, J, and Raman, R. Antibacterial and antifungal properties of human cerumen. J Laryngol Otol. (2009) 123:375–8. doi: 10.1017/S0022215108003307
87. Wright, DN, and Alexander, JM. Effect of water on the bacterial Flora of swimmers’ ears. Arch Otolaryngol. (1974) 99:15–8. doi: 10.1001/ARCHOTOL.1974.00780030019003
88. Rice, SA, Van Den Akker, B, Pomati, F, and Roser, D. A risk assessment of Pseudomonas aeruginosa in swimming pools: a review. J Water Health. (2012) 10:181–96. doi: 10.2166/wh.2012.020
89. Shokry, E, and Filho, NRA. Insights into cerumen and application in diagnostics: past, present and future prospective. Biochem Med (Zagreb). (2017) 27:503. doi: 10.11613/BM.2017.030503
90. Perry, ET, and Nichols, AC. Studies on the growth of bacteria in the human ear canal. J Invest Dermatol. (1956) 27:165–70. doi: 10.1038/jid.1956.89
91. Brook, I. Microbiological studies of the bacterial flora of the external auditory canal in children. Acta Otolaryngol. (1981) 91:285–7. doi: 10.3109/00016488109138509
92. Stroman, DM, Roland, PS, Dohar, J, and Burt, W. Microbiology of normal external auditory canal. Laryngoscope. (2001) 111:2054–9. doi: 10.1097/00005537-200111000-00035
93. Crespo, M, Abarca, M, and Cabañes, F. Occurrence of Malassezia spp. in the external ear canals of dogs and cats with and without otitis externa. Med Mycol. (2002) 40:115–21. doi: 10.1080/mmy.40.2.115.121
94. Nardoni, S, Mancianti, F, Corazza, M, and Rum, A. Occurrence of Malassezia species in healthy and dermatologically diseased dogs. Mycopathologia. (2004) 157:383–8. doi: 10.1023/B:MYCO.0000030416.36743.dd
95. Cafarchia, C, Gallo, S, Capelli, G, and Otranto, D. Occurrence and population size of Malassezia spp. in the external ear canal of dogs and cats both healthy and with otitis. Mycopathologia. (2005) 160:143–9. doi: 10.1007/s11046-005-0151-x
96. Aldrovandi, AL, Osugui, L, and Acqua Coutinho, SD. Is Malassezia nana the main species in horses’ ear canal microbiome? Braz J Microbiol. (2016) 47:770–4. doi: 10.1016/j.bjm.2016.04.017
97. Shokri, H. Occurrence and distribution of Malassezia species on skin and external ear canal of horses. Mycoses. (2016) 59:28–33. doi: 10.1111/myc.12430
98. Alshahni, MM, Alshahni, RZ, Fujisaki, R, Tamura, T, Shimizu, Y, Yamanishi, C, et al. A case of topical Ofloxacin-induced Otomycosis and literature review. Mycopathologia. (2021) 186:871–6. doi: 10.1007/s11046-021-00581-x
99. Ngo, J, Taminiau, B, Fall, PA, Daube, G, and Fontaine, J. Ear canal microbiota - a comparison between healthy dogs and atopic dogs without clinical signs of otitis externa. Vet Dermatol. (2018) 29:425–e140. doi: 10.1111/vde.12674
100. Korbelik, J, Singh, A, Rousseau, J, and Weese, JS. Analysis of the otic mycobiota in dogs with otitis externa compared to healthy individuals. Vet Dermatol. (2018) 29:417–e138. doi: 10.1111/vde.12665
101. Rodrigues Hoffmann, A, Patterson, AP, Diesel, A, Lawhon, SD, Ly, HJ, Stephenson, CE, et al. The skin microbiome in healthy and allergic dogs. PLoS One. (2014) 9:e83197. doi: 10.1371/journal.pone.0083197
102. Meason-Smith, C, Diesel, A, Patterson, AP, Older, CE, Mansell, JM, Suchodolski, JS, et al. What is living on your dog’s skin? Characterization of the canine cutaneous mycobiota and fungal dysbiosis in canine allergic dermatitis. FEMS Microbiol Ecol. (2015) 91:139. doi: 10.1093/femsec/fiv139
103. Korbelik, J, Singh, A, Rousseau, J, and Weese, JS. Characterization of the otic bacterial microbiota in dogs with otitis externa compared to healthy individuals. Vet Dermatol. (2019) 30:228–e70. doi: 10.1111/vde.12734
104. Apostolopoulos, N, Glaeser, SP, Bagwe, R, Janssen, S, Mayer, U, Ewers, C, et al. Description and comparison of the skin and ear canal microbiota of non-allergic and allergic German shepherd dogs using next generation sequencing. PLoS One. (2021) 16:e0250695. doi: 10.1371/journal.pone.0250695
105. Borriello, G, Paradiso, R, Catozzi, C, Brunetti, R, Roccabianca, P, Riccardi, MG, et al. Cerumen microbial community shifts between healthy and otitis affected dogs. PLoS One. (2020) 15:e0241447. doi: 10.1371/journal.pone.0241447
106. Bradley, C, Lee, F, Rankin, S, Kalan, L, Horwinski, J, Morris, D, et al. The otic microbiota and mycobiota in a referral population of dogs in eastern USA with otitis externa. Vet Dermatol. (2020) 31:225–e49. doi: 10.1111/vde.12826
107. Kasai, T, Fukui, Y, Aoki, K, Ishii, Y, and Tateda, K. Changes in the ear canal microbiota of dogs with otitis externa. J Appl Microbiol. (2021) 130:1084–91. doi: 10.1111/jam.14868
108. Leonard, C, Picavet, PP, Fontaine, J, Clercx, C, Taminiau, B, Daube, G, et al. The middle ear microbiota in healthy dogs is similar to that of the external ear canal. Vet Sci. (2023) 10. doi: 10.3390/vetsci10030216
109. Sjövall, A, Aho, V, Hyyrynen, T, Kinnari, T, Auvinen, P, Silvola, J, et al. Microbiome of the healthy external Auditory Canal. Otol Neurotol. (2021) 42:e609–14. doi: 10.1097/MAO.0000000000003031
110. Burton, M, Krumbeck, JA, Wu, G, Tang, S, Prem, A, Gupta, AK, et al. The adult microbiome of healthy and otitis patients: definition of the core healthy and diseased ear microbiomes. PLoS One. (2022) 17:e0262806. doi: 10.1371/journal.pone.0262806
111. Costello, E, Lauber, C, Hamady, M, Fierer, N, Gordon, J, and Knight, R. Bacterial community variation in human body habitats across space and time. Science. (2009) 326:1694–7. doi: 10.1126/science.1177486
112. Lee, JS, Lee, SM, Son, HS, Yoon, YN, Shin, JE, Sul, WJ, et al. Analysis of the microbiome of the ear canal in Normal individuals and patients with chronic otitis externa. Ann Dermatol. (2022) 34:461–71. doi: 10.5021/ad.22.153
113. Villette, R, Autaa, G, Hind, S, Holm, JB, Moreno-Sabater, A, and Larsen, M. Refinement of 16S rRNA gene analysis for low biomass biospecimens. Sci Rep. (2021) 11:1–12. doi: 10.1038/s41598-021-90226-2
114. Bjerre, RD, Bandier, J, Skov, L, Engstrand, L, and Johansen, JD. The role of the skin microbiome in atopic dermatitis: a systematic review. Br J Dermatol. (2017) 177:1272–8. doi: 10.1111/bjd.15390
115. Jiang, Z, Li, J, Kong, N, Kim, JH, Kim, BS, Lee, MJ, et al. Accurate diagnosis of atopic dermatitis by combining transcriptome and microbiota data with supervised machine learning. Sci Rep. (2022) 12:1–13. doi: 10.1038/s41598-021-04373-7
116. Santoro, D, and Rodrigues, HA. Canine and human atopic dermatitis: two faces of the same host-microbe interaction. J Invest Dermatol. (2016) 136:1087–9. doi: 10.1016/j.jid.2016.03.015
117. Leonard, C, Thiry, D, Taminiau, B, Daube, G, and Fontaine, J. External ear canal evaluation in dogs with chronic Suppurative otitis externa: comparison of direct cytology, bacterial culture and 16S amplicon profiling. Vet Sci. (2022) 9:366–6. doi: 10.3390/vetsci9070366
118. Chang, YC, Wu, MC, Wu, HJ, Liao, PL, and Wei, JCC. Prenatal and early-life antibiotic exposure and the risk of atopic dermatitis in children: a nationwide population-based cohort study. Pediatr Allergy Immunol. (2023) 34:e13959. doi: 10.1111/PAI.13959
119. Halling, AS, Fritz, BG, Gerner, T, Rinnov, MR, Bay, L, Knudgaard, MH, et al. Reduced skin microbiome diversity in infancy is associated with increased risk of atopic dermatitis in high-risk children. J Invest Dermatol. (2023) 143:2030–2038.e6. doi: 10.1016/j.jid.2023.03.1682
120. Tizard, IR, and Jones, SW. The microbiota regulates immunity and immunologic diseases in dogs and cats. Vet Clin NA: Small Animal Prac. (2018) 48:307–22. doi: 10.1016/j.cvsm.2017.10.008
121. Deckers, J, Lambrecht, BN, and Hammad, H. How a farming environment protects from atopy. Curr Opin Immunol. (2019) 60:163–9. doi: 10.1016/j.coi.2019.08.001
122. Turkalj, M, Drkulec, V, Haider, S, Plavec, D, Banić, I, Malev, O, et al. Association of bacterial load in drinking water and allergic diseases in childhood. Clin Exp Allergy. (2020) 50:733–40. doi: 10.1111/cea.13605
123. Sbihi, H, Simmons, KE, Sears, MR, Moraes, TJ, Becker, AB, Mandhane, PJ, et al. Early-life cytomegalovirus infection is associated with gut microbiota perturbations and increased risk of atopy. Pediatr Allergy Immunol. (2022) 33:e13658. doi: 10.1111/pai.13658
124. Stefanaki, E, Kalaitzidou, I, Aristou, M, Lakoumentas, J, Galanakis, E, and Xepapadaki, P. Prenatal antibiotic exposure increases the risk of infant atopic dermatitis: data from a Greek cohort. Eur Ann Allergy Clin Immunol. (2023) 55:271–7. doi: 10.23822/EurAnnACI.1764-1489.266
125. Zheng, H, Zeng, X, Xie, Q, Wu, Y, Liu, Q, Chen, Q, et al. Early life environmental antibiotic exposure and preschool allergic diseases: a biomonitoring-based prospective study in eastern China. Front Public Health. (2022) 10. doi: 10.3389/fpubh.2022.1043942
126. Kamphorst, K, Oosterloo, BC, Reichwein, LC, Vlieger, AM, and van Elburg, RM. The association between exposure to antibiotics in the first week of life and later otitis media: the INCA study. Int J Pediatr Otorhinolaryngol. (2023) 164:111415. doi: 10.1016/j.ijporl.2022.111415
127. Hemida, MBM, Salin, S, Vuori, KA, Moore, R, Anturaniemi, J, Rosendahl, S, et al. Puppyhood diet as a factor in the development of owner-reported allergy/atopy skin signs in adult dogs in Finland. J Vet Intern Med. (2021) 35:2374–83. doi: 10.1111/jvim.16211
128. Graham, AS, Ben-Azu, B, Tremblay, M-È, Torre, P III, Senekal, M, Laughton, B, et al. A review of the auditory-gut-brain axis. Front Neurosci. (2023) 17:1183694. doi: 10.3389/fnins.2023.1183694
129. Sonoyama, K, Miki, A, Sugita, R, Goto, H, Nakata, M, and Yamaguchi, N. Gut colonization by Candida albicans aggravates inflammation in the gut and extra-gut tissues in mice. Med Mycol. (2011) 49:237–47. doi: 10.3109/13693786.2010.511284
130. Fousekis, FS, Saridi, M, Albani, E, Daniel, F, Katsanos, KH, Kastanioudakis, IG, et al. Ear involvement in inflammatory bowel disease: a review of the literature. J Clin Med Res. (2018) 10:609. doi: 10.14740/jocmr3465w
131. Gelardi, M, Giancaspro, R, Landi, M, Santoiemma, L, Balestra, M, Cassano, M, et al. Perspectives of Italian physicians and patients in the treatment of otitis externa: a real-life study. J Personalized Med. (2023) 13:1083. doi: 10.3390/jpm13071083
132. Song, Y, Abdella, S, Afinjuomo, F, Weir, EJ, Tan, JQE, Hill, P, et al. Physicochemical properties of otic products for canine otitis externa: comparative analysis of marketed products. BMC Vet Res. (2023) 19:39–9. doi: 10.1186/S12917-023-03596-2
133. Panzuti, P, Mosca, M, Fantini, O, Noel, G, Cappelle, J, and Pin, D. Effect of an ear cleaner instillation containing lipacids in a model of re-acidification of the external auditory canal in dogs. Vet Dermatol. (2022) 33:402–6. doi: 10.1111/vde.13101
134. Léonard, C, Taminiau, B, Ngo, J, Fantini, O, Daube, G, and Fontaine, J. Preventive use of a topical anti-inflammatory glucocorticoid in atopic dogs without clinical sign of otitis does not affect ear canal microbiota and mycobiota. Vet Dermatol. (2021) 32:355–e98. doi: 10.1111/vde.12977
135. Puigdemont, A, D’Andreano, S, Ramió-Lluch, L, Cuscó, A, Francino, O, and Brazis, P. Effect of an anti-inflammatory pomegranate otic treatment on the clinical evolution and microbiota profile of dogs with otitis externa. Vet Dermatol. (2021) 32:158–e37. doi: 10.1111/vde.12930
136. Chermprapai, S, Ederveen, THA, Broere, F, Broens, EM, Schlotter, YM, van Schalkwijk, S, et al. The bacterial and fungal microbiome of the skin of healthy dogs and dogs with atopic dermatitis and the impact of topical antimicrobial therapy, an exploratory study. Vet Microbiol. (2019) 229:90–9. doi: 10.1016/j.vetmic.2018.12.022
137. Matsui, K, Nojima, Y, Kajiwara, Y, Busujima, K, and Mori, Y. Topical application of doxycycline inhibits Th2 cell development mediated by Langerhans cells and exerts a therapeutic effect on atopic dermatitis. J Pharm Pharm Sci. (2020) 23:86–99. doi: 10.18433/jpps30847
138. Czibulka, A. Probiotics for Otolaryngologic disorders. Otolaryngol Clin N Am. (2022) 55:939–46. doi: 10.1016/j.otc.2022.06.003
139. Jörissen, J, van den Broek, MFL, De Boeck, I, Van Beeck, W, Wittouck, S, Boudewyns, A, et al. Case-control microbiome study of chronic otitis media with effusion in children points at Streptococcus salivarius as a Pathobiont-inhibiting species. mSystems. (2021) 6. doi: 10.1128/msystems.00056-21
140. Bourdillon, AT, and Edwards, HA. Review of probiotic use in otolaryngology. Am J Otolaryngol. (2021) 42. doi: 10.1016/j.amjoto.2020.102883
141. Kim, SK, Han, SJ, Hong, SJ, and Hong, SM. Microbiome of acute otitis externa. J Clin Med. (2022) 11:7074. doi: 10.3390/jcm11237074
142. Hill, PB, Lo, A, Eden, CAN, Huntley, S, Morey, V, Ramsey, S, et al. Survey of the prevalence, diagnosis and treatment of dermatological conditions in small animals in general practice. Vet Rec. (2006) 158:533–9. doi: 10.1136/vr.158.16.533
143. O’Neill, DG, Church, DB, PD, MG, Thomson, PC, and Brodbelt, DC. Prevalence of disorders recorded in dogs attending primary-care veterinary practices in England. PLoS One. (2014) 9:e90501. doi: 10.1371/journal.pone.0090501
144. O’Neill, DG, Volk, AV, Soares, T, Church, DB, Brodbelt, DC, and Pegram, C. Frequency and predisposing factors for canine otitis externa in the UK - a primary veterinary care epidemiological view. Canine Med Genet. (2021) 8:7. doi: 10.1186/S40575-021-00106-1
145. Clark, WB, Brook, I, Bianki, D, and Thompson, DH. Microbiology of otitis externa. Otolaryngol Head Neck Surg. (1997) 116:23–5. doi: 10.1016/S0194-59989770346-2
146. Roland, P, and Stroman, D. Microbiology of acute otitis externa. Laryngoscope. (2002) 112:1166–77. doi: 10.1097/00005537-200207000-00005
147. Ninkovic, G, Dullo, V, and Saunders, NC. Microbiology of otitis externa in the secondary care in United Kingdom and antimicrobial sensitivity. Auris Nasus Larynx. (2008) 35:480–4. doi: 10.1016/j.anl.2007.09.013
148. Lee, H, Kim, J, and Nguyen, V. Ear infections: otitis externa and otitis media. Prim Care. (2013) 40:671–86. doi: 10.1016/J.POP.2013.05.005
149. Aboutalebian, S, Ahmadikia, K, Fakhim, H, Chabavizadeh, J, Okhovat, A, Nikaeen, M, et al. Direct detection and identification of the Most common Bacteria and Fungi causing otitis externa by a stepwise multiplex PCR. Front Cell Infect Microbiol. (2021) 11:644060. doi: 10.3389/fcimb.2021.644060
150. De Martino, L, Nocera, FP, Mallardo, K, Nizza, S, Masturzo, E, Fiorito, F, et al. An update on microbiological causes of canine otitis externa in Campania region, Italy. Asian Pac J Trop Biomed. (2016) 6:384–9. doi: 10.1016/j.apjtb.2015.11.012
151. Schaffartzik, A, Hamza, E, Janda, J, Crameri, R, Marti, E, and Rhyner, C. Equine insect bite hypersensitivity: what do we know? Vet Immunol Immunopathol. (2012) 147:113–26. doi: 10.1016/j.vetimm.2012.03.017
152. Peeters, L, Verlinden, T, Brebels, M, Buys, N, and Janssens, S. Environmental factors affecting the prevalence of insect bite hypersensitivity in Belgian warmblood horses in Vlaanderen. Commun Agric Appl Biol Sci. (2011) 76:205–9.
153. Bizikova, P, Santoro, D, Marsella, R, Nuttall, T, Eisenschenk, MNC, and Pucheu-Haston, CM. Review: clinical and histological manifestations of canine atopic dermatitis. Vet Dermatol. (2015) 26:79–e24. doi: 10.1111/vde.12196
154. Martin, S, Esser, P, Weber, F, Jakob, T, Freudenberg, M, Schmidt, M, et al. Mechanisms of chemical-induced innate immunity in allergic contact dermatitis. Allergy. (2011) 66:1152–63. doi: 10.1111/j.1398-9995.2011.02652.x
155. Kurotaki, T, Narayama, K, Oyamada, T, Yoshikawa, H, and Yoshikawa, T. Immunopathological study on equine insect hypersensitivity (“kasen”) in Japan. J Comp Pathol. (1994) 110:145–52. doi: 10.1016/S0021-9975(08)80186-7
156. Zur, G, Ihrke, PJ, White, SD, and Kass, PH. Canine atopic dermatitis: a retrospective study of 266 cases examined at the University of California, Davis, 1992-1998. Part I. Clinical features and allergy testing results. Vet Dermatol. (2002) 13:89–102. doi: 10.1046/j.1365-3164.2002.00285.x
157. Cole, LK. Anatomy and physiology of the canine ear. Vet Dermatol. (2009) 20:412–21. doi: 10.1111/j.1365-3164.2009.00849.x
158. Huang, H-P. Studies of the microenvironment and microflora of the canine external ear canal - theses. Glasgow: University of Glasgow (1993).
159. Blanke, A, Aupperle, H, Seeger, J, Kubick, C, and Schusser, GF. Histological study of the external, middle and inner ear of horses. Anat Histol Embryol. (2015) 44:401–9. doi: 10.1111/ahe.12151
160. Mueller, EN, Bergmann, LK, Anciuti, AN, Tillmann, MT, and Nobre, M. Study of the difference in rectal and ear canal temperature according to the conformation of the acoustic conch in dogs. Semin Cienc Agrar. (2012) 33:1907–10. doi: 10.5433/1679-0359.2012v33n5p1907
161. Mittal, A, and Kumar, S. Role of pH of external Auditory Canal in acute otitis externa. Indian J Otolaryngol Head Neck Surg. (2014) 66:86. doi: 10.1007/s12070-013-0684-0
162. Grono, LR. Studies of the microclimate of the external auditory canal in the dog. II. Hydrogen ion concentration of the epithelial surface of the external auditory meatus. Res Vet Sci. (1970) 11:312–5. doi: 10.1016/S0034-5288(18)34294-2
163. Gray, RF, Sharma, A, and Vowler, SL. Relative humidity of the external auditory canal in normal and abnormal ears, and its pathogenic effect. Clin Otolaryngol. (2005) 30:105–11. doi: 10.1111/j.1365-2273.2004.00950.x
164. Nakatsuji, T, Kao, MC, Fang, JY, Zouboulis, CC, Zhang, L, Gallo, RL, et al. Antimicrobial property of lauric acid against propionibacterium acnes: its therapeutic potential for inflammatory acne vulgaris. J Invest Dermatol. (2009) 129:2480–8. doi: 10.1038/jid.2009.93
165. Bergsson, G, Arnfinnsson, J, Steingrímsson, Ó, and Thormar, H. Killing of gram-positive cocci by fatty acids and monoglycerides. APMIS. (2001) 109:670–8. doi: 10.1034/j.1600-0463.2001.d01-131.x
166. Greenway, DLA, and Dyke, KGH. Mechanism of the inhibitory action of linoleic acid on the growth of Staphylococcus aureus. J Gen Microbiol. (1979) 115:233–45. doi: 10.1099/00221287-115-1-233
167. Watanabe, T, Yamamoto, Y, Miura, M, Konno, H, Yano, S, and Nonomura, Y. Systematic analysis of selective bactericidal activity of fatty acids against Staphylococcus aureus with minimum inhibitory concentration and minimum bactericidal concentration. J Oleo Sci. (2019) 68:291–6. doi: 10.5650/jos.ess18220
168. Dilika, F, Bremner, PD, and Meyer, JJM. Antibacterial activity of linoleic and oleic acids isolated from Helichrysum pedunculatum: a plant used during circumcision rites. Fitoterapia. (2000) 71:450–2. doi: 10.1016/S0367-326X(00)00150-7
169. Yoon, JY, Park, WJ, and Lee, JE. Presence of hBD-1 and hBD-2 in human cerumen and external auditory canal skin. Acta Otolaryngol. (2008) 128:871–5. doi: 10.1080/00016480701785020
170. Stoeckelhuber, M, Matthias, C, Andratschke, M, Stoeckelhuber, BM, Koehler, C, Herzmann, S, et al. Human ceruminous gland: ultrastructure and histochemical analysis of antimicrobial and cytoskeletal components. Anat Rec A Discov Mol Cell Evol Biol. (2006) 288:877–84. doi: 10.1002/ar.a.20356
171. Yoon, YJ, and Lee, EJ. Spatial distribution of antimicrobial peptides and mast cells in the skin of the external auditory canal. J Laryngol Otol. (2011) 125:1–5. doi: 10.1017/S0022215111002271
172. Yasui, T, Tsukise, A, Fukui, K, Kuwahara, Y, and Meyer, W. Aspects of glycoconjugate production and lysozyme-and defensins-expression of the ceruminous glands of the horse (Equus przewalskii f. dom.). Eur J Morphol. (2005) 42:127–34. doi: 10.1080/09243860500202507
Keywords: allergic dermatitis, microbiome, otitis externa, ear microbiome, atopic dermatitis, external ear canal
Citation: Houtsaeger C, Pasmans F, Claes I, Vandenabeele S, Haesebrouck F, Lebeer S and Boyen F (2024) The role of the microbiome in allergic dermatitis-related otitis externa: a multi-species comparative review. Front. Vet. Sci. 11:1413684. doi: 10.3389/fvets.2024.1413684
Edited by:
Carlos Eduardo Fonseca-Alves, Paulista University, BrazilReviewed by:
Adriana Belas, Lusofona University, PortugalAlexandre Cavalca, Universidade Estadual Paulista, Brazil
Copyright © 2024 Houtsaeger, Pasmans, Claes, Vandenabeele, Haesebrouck, Lebeer and Boyen. This is an open-access article distributed under the terms of the Creative Commons Attribution License (CC BY). The use, distribution or reproduction in other forums is permitted, provided the original author(s) and the copyright owner(s) are credited and that the original publication in this journal is cited, in accordance with accepted academic practice. No use, distribution or reproduction is permitted which does not comply with these terms.
*Correspondence: Cyrelle Houtsaeger, Q3lyZWxsZS5Ib3V0c2FlZ2VyQFVHZW50LmJl