- College of Animal Science and Technology, Northeast Agricultural University, Harbin, China
An experiment was conducted to investigate the influences of supplemental lysophospholipids (LPL) on the growth performance, nutrient digestibility, and fecal bacterial profile, and short-chain fatty acids (SCFAs) of beef cattle. Thirty-six Angus beef cattle [565 ± 10.25 kg body weight (BW)] were grouped by BW and age, and randomly allocated to 1 of 3 treatment groups: (1) control (CON, basal diet); (2) LLPL [CON supplemented with 0.5 g/kg LPL, dry matter (DM) basis]; and (3) HLPL (CON supplemented with 0.75 g/kg, DM basis). The Angus cattle were fed a total mixed ration that consisted of 25% roughage and 75% concentrate (dry matter [DM] basis). The results reveal that LPL inclusion linearly increased the average daily gain (P = 0.02) and the feed efficiency (ADG/feed intake, P = 0.02), while quadratically increasing the final weight (P = 0.02) of the beef cattle. Compared with CON, the total tract digestibilities of DM (P < 0.01), ether extract (P = 0.04) and crude protein (P < 0.01) were increased with LPL supplementation. At the phylum-level, the relative abundance of Firmicutes (P = 0.05) and ratio of Firmicutes: Bacteroidetes (P = 0.04) were linearly increased, while the relative abundances of Bacteroidetes (P = 0.04) and Proteobacteria (P < 0.01) were linearly decreased with increasing LPL inclusion. At the genus-level, the relative abundances of Clostridium (P < 0.01) and Roseburia (P < 0.01) were quadratically increased, and the relative abundances of Ruminococcus was linearly increased (P < 0.01) with LPL supplementation. Additionally, increasing the dose of LPL in diets linearly increased the molar proportion of butyrate (P < 0.01) and total SCFAs (P = 0.01) concentrations. A conclusion was drawn that, as a promising feed additive, LPL promoted growth performance and nutrient digestibility, which may be associated with the change of fecal microbiome and SCFAs.
Introduction
Lipids, which mainly include fats and oils, are commonly added to livestock diets as a concentrated energy source, providing fat-soluble vitamins and essential fatty acids (FAs), promoting the absorption of fat-soluble nutrients and being significant factors in biochemistry, physiology, and nutrition (1, 2). The use of fats as a partial substitute for grains in diets can effectively improve the energy concentration and fattening performance of beef cattle, which is a crucial method for producing marble beef and improving the carcass quality of cattle (3). However, the use of excessive fat (6–7% of the dietary dry matter [DM]) in the ruminant diet can lead to excess visceral fat accumulation and loss of vitamins A and E, furthermore decreasing the growth performance and digestibility of beef cattle (4, 5). As suggested by Souza et al. (6), the digestibility of fatty acids will be decreased with the increase of fatty acid flow to the intestine and the insufficient secretion of intestinal bile salts and pancreatic lipase. In previous studies, it has been found that emulsifiers can compensate for the insufficient of bile secretion and promote the incorporation of fatty acids into micelles, so as to improve fat digestibility (7).
Lysophospholipids (LPL), as a promising feed additive, have been widely used in the diets of non-ruminant animals to improve production and feed efficiency. According to studies by Zhao et al. (8) and Brautigan et al. (9), increases in growth performance, feed efficiency, and dietary nutrient absorption were observed when diets supplemented with LPL were fed to pigs and poultry. Studies have shown that LPL increased dietary fat absorption due to the emulsification property thereof and upregulated of the expression of various genes such as GAS6 and RAMP2 in the intestinal epithelium (9). Despite such findings, there is a scarcity of research on the effect of dietary LPL inclusion in ruminants.
Gut microbiota, serving as an important barrier in the host, has been known to influence the health of animals. Gut microbiota is significant factor in nutritional, metabolic and immunological functions, while also having effects on the growth performances and feed efficiency of livestock animals (10). The composition of gut microbiota is strongly influenced by diet, animal age, host genetics and feed additive (11, 12). Jang et al. (13) reported that supplementation of LPL in lactation diets increased the Firmicutes to Bacteroidetes ratio and improved intestinal health. Moreover, Qiu et al. (14) demonstrated that the supplementation of choline, as one of the main components of LPL, can enhance the growth performance and gut health of weaned piglets by altering the gut microbiota and metabolites. Therefore, further clarifying the effects of LPL inclusion in the rations of beef cattle on gut microbiome is of considerable significance. We hypothesized that adding LPL would improve growth performance, feed efficiency and nutrient digestibility due to regulating gut microbiota, and promoting the gut health of beef cattle. Thus, the objective of the present study was to investigate the effect of supplementing diets with LPL on the performance, apparent digestibility, fecal bacterial communities, and SCFAs of beef cattle.
Materials and methods
All experimental procedures involving animals in the present study were approved by the Institutional Animal Care Committee (Protocol number: NEAU- [2011]-9), Northeast Agricultural University (Harbin, China).
Lysophospholipids products
The LPL product used in the present experiment was a hydrolyzed soy lecithin, which is composed of phospholipids, free fatty acids and LPL (30%), and was provided by Jianming (China) Technology Co., LTD (Zhuhai, China).
Animals, experimental design, and diets
Thirty-six Angus beef cattle were blocked for body weight (BW) (565 ± 10.3 kg) and randomly assigned to 1 of 3 treatments (12 beef cattle per treatment): (1) control (CON; basal diet); (2) LLPL [CON supplemented with low LPL, 0.5 g/kg of dry matter (DM) basis]; and (3) HLPL (CON supplemented with high LPL, 0.75 g/kg of DM basis). All the cattle were housed in individual pens (4 × 3 m2) with free access to water and were fed ad libitum twice daily at 800 and 1,700 h. The cattle were fed for 74 days. During the first 14 days, the feed intake of concentrates was gradually increased, until the ratio of concentrate to roughage in the final diet (the first 10 days) reached 75:25, following which the feed intake was gradually increased until reaching the arbitrary feed intake. The dietary ingredients and chemical composition are presented in Table 1. The Chinese wild rye grass (Leymus chinensis) which is widely distributed throughout the Eurasian Steppe, including the Songnen Plain and the eastern Inner Mongolian Plateau in China, is a perennial species of Gramineae.
Sample collection
The feed offered and refused for individual beef cattle was weighed every day throughout the trial to calculate DM intake (DMI). To determine the average daily gain (ADG), all the cattle were weighed at the end of the diet adaptation period (d 15), at the midpoint on 2 consecutive days (d 29 and 30), and at the end of the experiment on two consecutive days (d 59 and 60). Samples of dietary ingredients, diets, and refusals during the experimental period were collected and stored at −20°C, and later pooled by beef cattle and period. Then, all the feed samples were dried at 55°C for 48 h, ground to pass through a 1-mm screen, and stored for chemical analysis.
Fecal samples (about 500 g fresh) were collected from the rectum of individual animal daily at 600, 1,200, 1,800, and 2,400 h from d 28 to 30, and from d 58 to 60 according to the method of Yang et al. (15). The collected fecal samples were pooled by animal and by sampling period, dried at 55°C for 48 h, and ground to pass through a 1-mm screen for subsequent chemical analysis. Meanwhile, ~5 g spot fecal samples were collected, and then stored instantly in liquid nitrogen until determination of bacterial communities and SCFAs.
Chemical analysis
Samples of individual feed ingredients and refusals were dried at 55°C for 48 h for DM (934.01), crude protein (CP; 976.05), ether extract (EE; 920.39), and ash (942.05) determination (16). Following the procedure described by Van Soest et al. (17), the neutral detergent fiber (NDF) content was analyzed using heat-stable α-amylase and sodium sulfite. The acid detergent fiber (ADF) content was analyzed according to AOAC (1990; method 954.01). The total-tract apparent nutrient digestibility was determined using acid detergent insoluble ash as an internal marker. The acid detergent insoluble ash contents of feeds and feces were determined by analyzing samples for ADF, as previously described, and the ash contents were determined by incinerating the Ankom bags for 8 h at 450°C in a muffle furnace (18). The DM, EE, CP, NDF, and ADF were analyzed using similar methods as described for the analyses of feed and fecal samples. The apparent digestibility was measured according to the method described by Merchen (19).
The fecal samples for determining microbial profiles were delivered to Personalbio Biotechnology Co., Ltd. (Shanghai, China) for 16S rRNA high-throughput Sequencing according to the method described by Zhu et al. (20). In brief, OMEGA Soil DNA Kits (M5635-02) (Omega Bio-Tek, Norcross, GA, USA) were used for DNA extraction of fecal samples according to the manufacturer's instructions. The quantity and quality of extracted DNA were determined by NanoDrop NC2000 spectrophotometer and agarose gel electrophoresis, and stored at −80°C until further processing. The V3–V4 region of the bacteria 16S rRNA gene was amplified by polymerase chain reaction (PCR) (1 cycle 95°C for 5 min, followed by 25 cycles at 95°C for 30 s, 50°C for 30 s and 72°C of 40 s, and a final extension of 72°C for 7 min). The amplification was performed using the forward primer 338F (5′-ACTCCTACGGGAGGCAGCA-3′) and the reverse primer 806R (5′-5′-GGACTACHVGGGTWTCTAAT-3′). The amplified product concentration according to PCR was mixed with equal concentration. Amplicons were purified by 2% agarose gels and recycled by using the AxyPrep DNA Gel Extraction Kit (Axygen Biosciences, Union City, CA, USA) according to the manufacturer's instructions, being for being quantified by means of the Quant-iT PicoGreen dsDNA Assay Kit.
The concentrations of SCFAs were determined in feces using gas chromatography (GC-2010, Shimadzu Corporation, Kyoto, Japan) according to the described method by Zhao et al. (21). In brief, the fecal samples (200 mg) were homogenized in 2 ml of ultrapure water by Vortex-Genie for 2 min. The mixture was centrifuged at 10,000 rpm for 10 min at 4°C, then the supernatant was filtered through a 0.22 μm filter membrane, which was repeated three times. The filtered supernatant was mixed with 25% (W/V) metaphosphoric acid at a ratio of 5:1 for the SCFAs assay.
Statistical analyses
All data were analyzed using the Proc Mixed procedure of SAS (version 9.2; SAS Institute Inc., Cary, NC). The statistical model included week, treatment, and interaction of treatment × week as fixed effects, as well as beef cattle within treatment as a random effect. The significance among treatments was analyzed using Tukey's multiple comparison test. To analyze the linear and quadratic effects of LPL inclusion levels, a polynomial orthogonal contrast was conducted. Significant differences were declared at P ≤ 0.05 and trends at 0.05 < P ≤ 0.10.
Results
Growth performance
As shown in Table 2, supplementation of LPL in the diets did not affect the DMI. However, increasing the supplemental dose of LPL linearly increased the ADG (P = 0.02) and feed efficiency (P = 0.02), and quadratically increased the final BW (P = 0.02). In comparison with CON, supplementation of LPL tended to increase the ADG (P = 0.07) and the feed efficiency (P = 0.06).
Nutrient digestibility
The digestibility of DM (P < 0.01), EE (P = 0.04) and CP (P < 0.01) increased linearly with increasing doses of LPL. However, the apparent digestibilities of ADF and NDF showed no significant difference among the treatment groups (Table 3). The digestibilities of DM (P < 0.01), EE (P = 0.04), and CP (P < 0.01) were higher for LLPL and HLPL compared with CON.
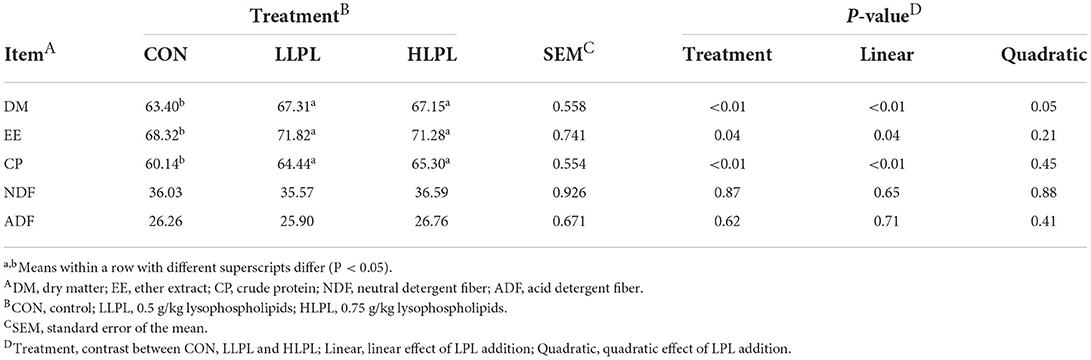
Table 3. Effect of dietary lysophospholipids supplementation on nutrient digestibility of beef cattle.
Sequence and fecal bacterial community composition
After removing incorrect and chimeric sequences, 728961 sequencing reads were generated from all the 15 samples. Each sample returned 29953~51192 sequences, with an average length of 421 bp (Supplementary Table 1). After ASV picking and chimera checking, 42079 amplicon sequence variants (ASVs) were obtained for all the samples at 100% sequence similarity. In total, 6778, 6445, and 5958 ASVs were clustered in the CON, LLP and HLPL groups, respectively; further, 2194 ASVs were exclusive in all three treatments (Supplementary Figure 1). Similarly, the Good's coverages of all samples exceeded 99%, thereby verifying the accuracy and reproducibility of the sequencing.
A total of 15 bacterial phyla were detected in all the samples. Firmicutes (70.28%), Bacteroidetes (25.54%), and Spirochaetes (1.32%) were the most predominant phyla (Supplementary Table 2). At the genus level, a total of 249 bacterial genera were detected, and the six most dominant genera were successively unclassified Ruminococcaceae (23.87%), unidentified Bacteroidales (11.64%), Clostridium (6.35%), unidentified Peptostreptococcaceae (6.62%), unidentified Peptostreptococcaceae (4.23%), and 5-7N15 (3.74%) (Supplementary Table 3).
Fecal bacterial communities
The alpha bacterial diversity is shown in Table 4. The indexes of Chao1, Shannon, Simpson, Observed species and Faith's PD did not differ among treatments. The results of the rarefaction curves indicate that with the increase in sequence number, the number of ASV in feces from each sample showed a trend of increasing first and then becoming stable. As such, the sequencing depth in the present study was sufficient for evaluating dominant members of the fecal bacterial community (Supplementary Figure 2).
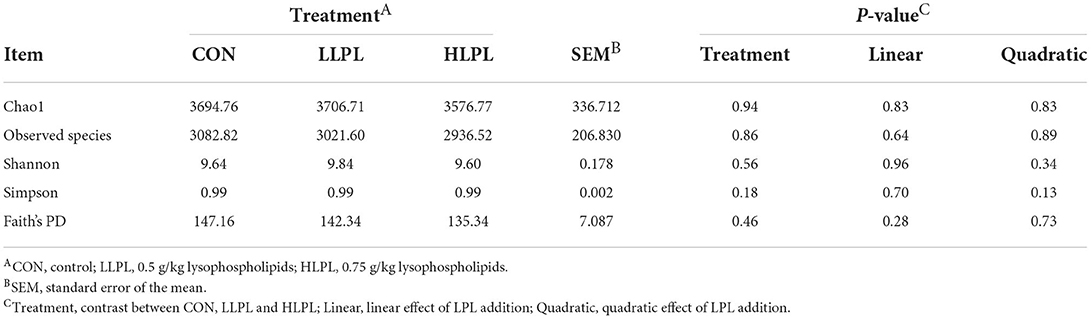
Table 4. Effect of dietary lysophospholipids supplementation on Alpha diversity index of fecal bacteria.
As revealed by principal coordinates analysis (PCoA) based on unweighted UniFrac metrics, there was a distinct clustering of the microbiota composition between CON and LPL treatments (Figure 1). The results of ANOSIM analysis reveal a significant difference in bacterial community composition between the treatments of CON and LLPL (R = 0.350, P = 0.029). There was a trend of difference between the treatments of LLPL and HLPL (R = 0.244, P = 0.099). In addition, there was no significant difference between the CON and HLPL treatments (R = 0.096, P = 0.206).
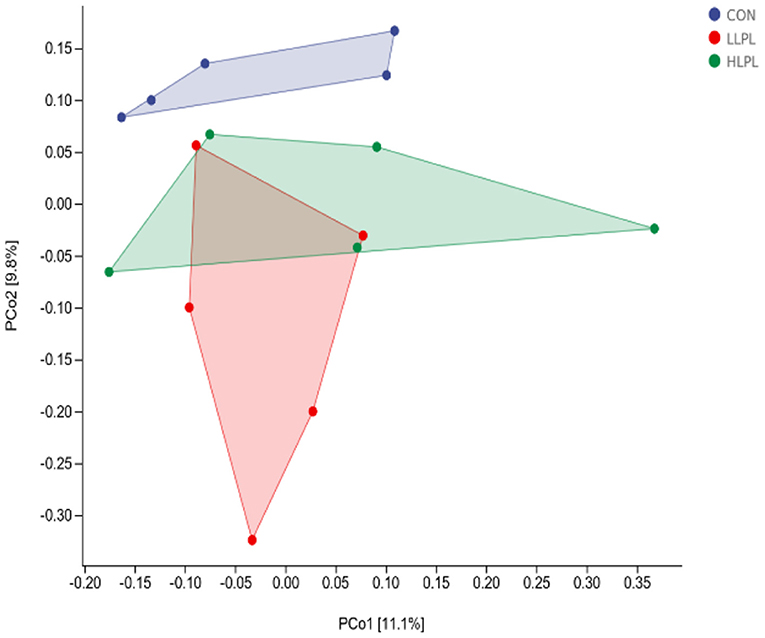
Figure 1. Principal coordinate analysis (PCoA) of the fecal microbial communities based on unweighted UniFrac. CON, control; LLPL, 0.5 g/kg lysophospholipids; HLPL, 0.75 g/kg lysophospholipids.
The results of the relative abundances (top 10) of the bacterial phyla and genus in the feces of cattle are shown in Table 5 and Figure 2. At the phylum level, with increases in dietary LPL levels, the relative abundances of Firmicutes (P = 0.05) linearly increased, and the relative abundances of Bacteroidetes (P = 0.04) linearly decreased. Thus, increasing the supplementation of LPL linearly increased (P < 0.01) the F:B ratio (Firmicutes: Bacteroidetes). Moreover, with the inclusion of LPL, a linear decrease (P < 0.01) in the relative abundance of Proteobacteria was observed. In comparison with CON, the F:B ratio was increased (P < 0.01), whereas the relative abundances of Proteobacteria were decreased (P < 0.01) with LPL supplementation. At the genus level, with the increase in supplemental dose of LPL, the relative abundances of Clostridium (P < 0.01) and Roseburia (P < 0.01) quadratically increased, and the relative abundances of Ruminococcus linearly increased (P < 0.01). Moreover, the relative abundances of Ruminococcus (P = 0.01), Treponema (P < 0.01), and Roseburia (P < 0.01) were higher for LLPL and HLPL compared with CON.
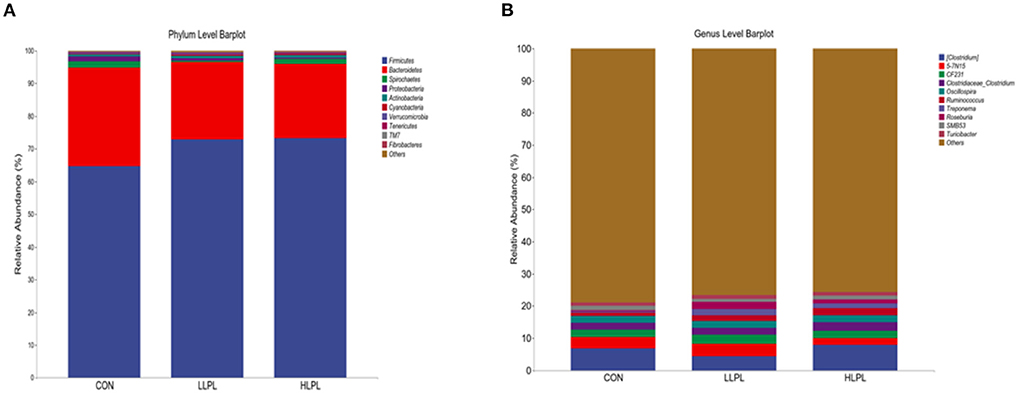
Figure 2. Fecal bacterial phyla and genera in three treatments. (A) The bacterial taxonomic composition of fecal samples from the three treatments at the phylum level. (B) The bacterial taxonomic composition of fecal samples from the three treatments at the genus level (top 10, according to relative abundance). CON, control; LLPL, 0.5 g/kg lysophospholipids; HLPL, 0.75 g/kg lysophospholipids.
Association and model predictive analysis
Redundancy analysis (RDA) of the correlation between the microbiota and their metabolites (SCFAs; Figure 3A). The correlation between microbiota distribution and metabolites were as follows: Total SCFAs > Propionate > Isobutyrate > Isovalerate > Butyrate > A/P > Valerate > Acetate. In addition, RDA indicated that there was positive correlation among Total SCFAs, Propionate, and Butyrate, and negative correlation between Total SCFAs and Isobutyrate, Isovalerate, A/P and Acetate. Co-occurrence network analysis was conducted between the top 30 bacterial genera and their metabolites (Figure 3B). The results indicated that Succinivibrio is positively correlated with Total SCFAs, Butyrate and valerate, and Roseburia is positively Butyrate. Corynebacterium is positively correlated with Propionate and Total SCFAs. Phascolarctobacterium is positively correlated with A/P and Valerate, however Bifidobacterium is negatively correlated with Isovalerate.
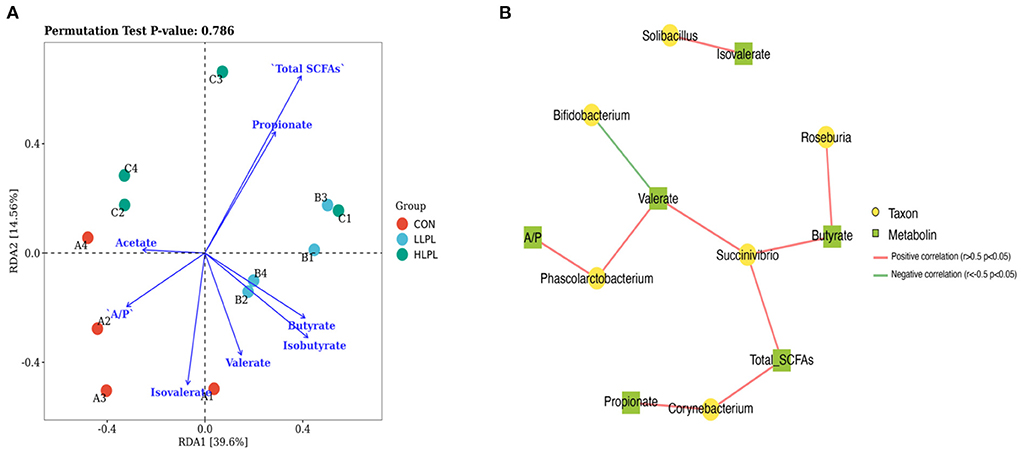
Figure 3. Association and model predictive analysis. (A) RDA analysis of the correlation between the microbiota and their metabolites. Each point represents a sample; each arrow represents a metabolite (SCFAs). (B) Co-occurrence network analysis among the microbiota and metabolites. Each co-occurring pair among microbial populations at the genus level and their metabolites has an absolute Spearman rank correlation above 0.5 [red line, positive correlation (r ≥ 0.5); green line, negative correlation (r ≤ −0.5)] with a FDR-corrected significance level under 0.05.
Total VFA concentration and VFA profiles
Increasing the dose of LPL in diets, the molar proportion of butyrate (P < 0.01), and total SCFAs (P = 0.01) concentrations linearly increased, as shown in Table 6. However, proportions of acetate, propionate, valerate, isobutyrate, and isovalerate, and the ratio of acetate to propionate showed no significant difference among the treatment groups. Compared with CON, the supplementation of LPL resulted in higher total SCFAs concentrations (P = 0.02) and molar proportion of butyrate (P < 0.01).
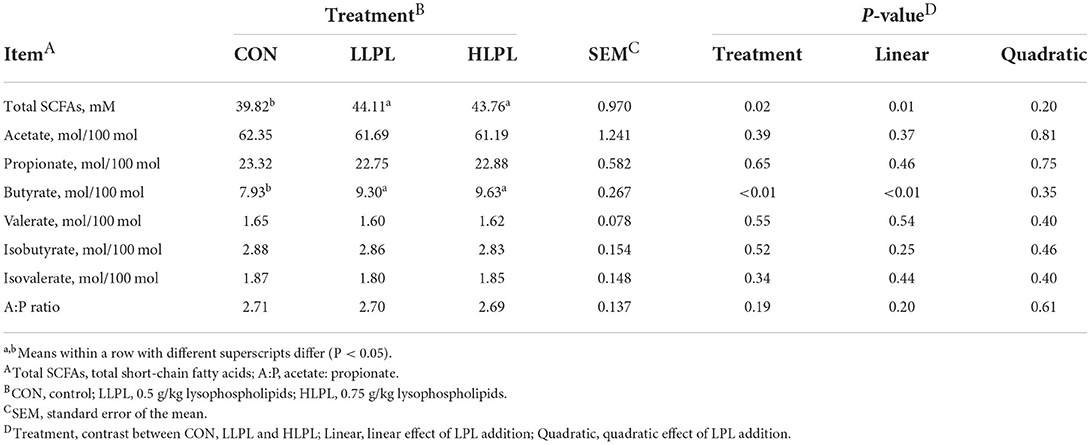
Table 6. Effect of dietary lysophospholipids supplementation on the fermentation of SCFAs in beef cattle.
Discussion
Growth performance
To the best of our knowledge, this is the first study to evaluate the effect of LPL inclusion on the growth performance of finishing cattle. Previous studies of LPL as feed additives have mostly focused on improving the growth performance and feed efficiency of monogastric animals (22, 23). Consistent with the findings in monogastric animals, increasing the supplemental dose of LPL in diets was found to linearly increase the ADG and decrease the FCR in the present study, indicating an improvement in growth performance. In prior research, phospholipids (a source of LPL) in the rumen were reported to escape microbial degradation and increase emulsification in the small intestine (24, 25). The improved performance of cattle with LPL supplementation may have been due to the increase in the absorption of nutrients in the small intestine in the current study. To the present knowledge, the effects of LPL as a feed additive on growth performance in ruminants have only been examined in 2 studies. Reis et al. (7) conducted a recent experiment and found that supplementation with LPL improved growth performance and feed efficiency without affecting the DMI of dairy cows. However, Song et al. (26) suggested that supplementation with LPL (0.3 or 0.5% w/w) did not affect the growth performance of Hanwoo heifers. The inconsistent results between Song et al. (26) and the present study could be partly attributed to the different types of LPL product, sources of phospholipids and fat, enzymatic (phospholipase) hydrolysis processes to produce LPL, and concentrations of LPL in the product. Notably, LPL has been widely used in the diets of non-ruminants as an additive, and the consistent effects of LPL on animal growth performance have been observed, which indicates that the degree of ruminal bypass of LPL might be critical for the effects of LPL on the growth performance of beef cattle (8, 22, 27).
Nutrient digestibility
Numerous studies on vitro digestion have shown that dietary emulsifiers can modulate the direct contact of lipid substrates and lipase, and thus, promote lipid digestion (28, 29). LPL could improve the nutrient digestibility of non-ruminant animals, which can be mainly attributed to the emulsification characteristics thereof (23, 30). In the present study, the linearly increased in the digestibilities of DM, EE, and CP with increasing supplementation of LPL, which is consistent with the findings of Song et al. (26) who found that supplementation of dietary LPL (0.3 or 0.5% w/w) increased the DM digestibility of beef cattle. In the present study, an assumption was made that the nutrient digestibility of EE was improved due to LPL being able to effectively reduce the size of fat globules and form smaller micelles in the guts of animals, thereby increasing the larger surface areas of lipid droplets for pancreatic lipases to interact so that more fatty acids would be incorporated (7, 31). In addition, the improved digestibility of CP could be attributed to LPL modifying the lipid bilayer of the membrane and increasing the number and size of the membranous pores, thereby altering the fluidity of the membrane and the transmembrane permeabilities of the nutrients (32, 33). However, LPL supplementation in ruminants does not always have the same effect on nutrient digestibility. Huo et al. (34) reported that supplementation of 0.05% LPL (DM basis) in the diets of lambs increased the digestibilities of DM, CP and OM. However, in feeding 0.05 and 0.075% LPL of the dietary DM to dairy cows, Lee et al. (25) observed a slight decrease in DM digestibility compared with the control group. The inconsistent results between Lee et al. (25) and the present study could be partially attributed to differences in diet, genetics and enzymatic (phospholipase) hydrolysis processes to produce LPL.
Fecal bacterial community composition
The results of PCoA with the unweighted UniFrac metrics reveal that the samples of the LPL group were clearly separated compared with the samples of the CON, indicating that the microbial composition of cattle in the LPL group was different from that of the CON group. The AMOVA results also show that there were significant differences between LLPL and CON. As revealed by numerous findings, gut microbiota are significant factors in animal health and growth, being responsible for nutrient metabolism, energy utilization and regulation of the immune responses in livestock (35, 36). To the present knowledge, no information is available on gut microbiota affected by dietary emulsifier supplementation in cattle. In the present study, Firmicutes and Bacteroidetes were the most predominant phyla in the fecal microbial communities, which is consistent with previous studies (37, 38). Additionally, a linear increase in the relative abundance of Firmicutes and a linear decrease in the relative abundance of Bacteroidetes with increasing supplemental dose of LPL were observed, resulting in an increased ratio of F:B (Firmicutes:Bacteroidetes), which could be beneficial for weight gain (39). A recent experiment conducted by Jang et al. (13) showed that LPL supplementation in diets increased the F:B ratio in the jejunum of sow, which is consistent with the present study (37). In addition, the increase in Firmicutes and decrease in Bacteroidetes and Proteobacteria in the feces seemed to have a positive impact with LPL on the gut health of cattle. Such positive impact could be attributed to Firmicutes mainly including beneficial bacteria and Proteobacteria mainly including harmful bacteria (35). The increase in Proteobacteria can lead to an imbalance of gut microbes and metabolic disorders (40). Moreover, study has reported that Bacteroides in animal fecal matter (41) has been known as fecal indicator bacteria (FIB). Fang et al. (42) found that Ruminococcus was positively correlated with the finishing weight, whereas the microbial taxa related to intestinal damage and inflammation showed opposite effects. Consistent with such findings, the abundance of Ruminococcus increased in the present study, which could partly explain the increased ADG with LPL supplementation.
Fecal fermentation characteristics
Short chain fatty acids are the gut microbiota metabolites produced from fermentation of complex carbohydrates, and are significant factors in maintaining intestinal health, regulation of glucose and lipid metabolism and epithelial barrier function (43, 44). In the present study, the increased the total concentration of SCFAs and molar proportion of butyrate in feces with increasing dietary supplementation of LPL is consistent with report by Qiu et al. (14) that choline, as one of the main components of LPL, could increase the concentration of SCFAs and molar proportion of butyrate of weaned piglets. Using RDA analysis, total SCFAs, Propionate, and Butyrate were significantly associated with feces microbiota. Furthermore, Spearman correlation analysis showed that Roseburia is positively Butyrate. The beneficial effects of LPL supplementation on SCFAs production were supported by the observation of enrichment of SCFA-producing bacteria, such as Roseburia and Clostridium (45, 46). Gut microbial butyrate metabolic pathways have been reported to increase energy intake and improve intestinal histology (for example, villi length, and crypt depth) in livestock, thereby having beneficial effects on livestock body weight (37, 47, 48). Moreover, Bedford and Gong (49) reported that butyrate has modulating capacity on energy and metabolism, which has a positive effect on body weight gain in animals. Therefore, the linearly increased ADG and decreased FCR could be a result of the greater proportion of butyrate with supplemented LPL in the diets of beef cattle in the present study.
Conclusion
Supplementation of beef cattle diets with LPL could promote growth performance, feed efficiency and apparent digestibility, which may be related to the change of relative abundance of bacterial communities, total SCFAs concentration and SCFAs profiles. The findings of the present study provide essential insights into the use of LPL as a growth promoter in beef cattle, and imply that manipulating the gut microbial community could be an efficient strategy for improving the finishing weight in the beef cattle industry. Furthermore, this study provides quantitative information that 0.75 g/kg LPL may be the optimal supplemental level for beef cattle finishing diets.
Data availability statement
The datasets presented in this study can be found in online repositories. The names of the repository/repositories and accession number(s) can be found in the article/supplementary material.
Ethics statement
The animal study was reviewed and approved by Institutional Animal Care Committee, Northeast Agricultural University (Harbin, China).
Author contributions
MZ and YoZ designed the experiments. MZ, HB, YuZ, RW, GL, and PJ conducted the experiments. MZ and HB analyzed the data. MZ, YoZ, and PJ wrote the manuscript. All authors approved the final manuscript.
Funding
This study was financially supported by China Agriculture Research System of MOF and MARA.
Acknowledgments
The authors thank the Northeast Agricultural University for the supporting facility.
Conflict of interest
The authors declare that the research was conducted in the absence of any commercial or financial relationships that could be construed as a potential conflict of interest.
Publisher's note
All claims expressed in this article are solely those of the authors and do not necessarily represent those of their affiliated organizations, or those of the publisher, the editors and the reviewers. Any product that may be evaluated in this article, or claim that may be made by its manufacturer, is not guaranteed or endorsed by the publisher.
Supplementary material
The Supplementary Material for this article can be found online at: https://www.frontiersin.org/articles/10.3389/fvets.2022.927369/full#supplementary-material
Supplementary Figure 1. ASV VennDiagram across treatments.
Supplementary Figure 2. Rarefaction curves of the operational taxonomic units (ASV).
Supplementary Table 1. Summary of sequencing data and average length.
Supplementary Table 2. Relative abundances at the phylum level across samples.
Supplementary Table 3. Relative abundances at the genus level across samples.
References
1. Hossain ME, Das GB. Effect of crude soybean oil sediment as a substitute for refined soybean oil in broiler diet. Iran J Appl Anim Sci. (2014) 4:535–40. doi: 10.9774/GLEAF.978-1-909493-38-4_2
2. Rossi R, Pastorelli G, Cannata S, Corino C. Recent advances in the use of fatty acids as supplements in pig diets: a review. Anim Feed Sci Tech. (2010) 162:1–11. doi: 10.1016/j.anifeedsci.2010.08.013
3. Andrae JG, Duckett SK, Hunt CW, Pritchard GT, Owens FN. Effects of feeding high-oil corn to beef steers on carcass characteristics and meat quality. J Anim Sci. (2001) 79:582–8. doi: 10.2527/2001.793582x
4. Beauchemin K, Kreuzer M, O'mara F, McAllister T. Nutritional management for enteric methane abatement: a review. Aust J Exp Agr. (2008) 48:21–7. doi: 10.1071/EA07199
5. Hess BW, Moss GE, Rule DC. A decade of developments in the area of fat supplementation research with beef cattle and sheep. J Anim Sci. (2008) 86:E188–204. doi: 10.2527/jas.2007-0546
6. Souza JD, Westerrn M, Lock AL. Abomasal infusion of an exogenous emulsifier improves fatty acid digestibility and milk fat yield of lactating dairy cows. J Dairy Sci. (2020) 103:6167–77. doi: 10.3168/jds.2020-18239
7. Reis M, Toledo A, da Silva A, Poczynek M, Fioruci E, Cantor M, et al. Supplementation of lysolecithin in milk replacer for Holstein dairy calves: effects on growth performance, health, and metabolites. J Dairy Sci. (2021) 104:5457–66. doi: 10.3168/jds.2020-19406
8. Zhao PY, Li HL, Hossain MM, Kim IH. Effect of emulsifier (Lysophospholipids) on growth performance, nutrient digestibility and blood profile in weanling pigs. Anim Feed Sci Technol. (2015) 207:190–5. doi: 10.1016/j.anifeedsci.2015.06.007
9. Brautigan DL, Li R, Kubicka E, Turner SD, Wong EA. Lysolecithin as feed additive enhances collagen expression and villus length in the jejunum of broiler chickens. Poult Sci. (2017) 96:2889–98. doi: 10.3382/ps/pex078
10. Sidhu M, van der Poorten D. The gut microbiome. Aust Fam Physician. (2017) 46:206–11. doi: 10.3316/informit.741870194405168
11. Plaizier JC, Li S, Danscher AM, Derakshani H, Andersen PH, Khafipour E. Changes in microbiota in rumen digesta and feces due to a grain-based subacute ruminal acidosis (SARA) challenge. Microb Ecol. (2017) 74:485–95. doi: 10.1007/s00248-017-0940-z
12. Scott KP, Gratz SW, Sheridan PO, Flint HJ, Duncan SH. The influence of diet on the gut microbiota. Pharmacol Res. (2013) 69:52–60. doi: 10.1016/j.phrs.2012.10.020
13. Jang KB, Purvis JM, Kim SW. Supplemental effects of dietary lysophospholipids in lactation diets on sow performance, milk composition, gut health, and gut-associated microbiome of offspring. J Anim Sci. (2020) 98:skaa227. doi: 10.1093/jas/skaa054.309
14. Qiu Y, Liu S, Hou L, Li K, Wang L, Gao K, et al. Supplemental choline modulates growth performance and gut inflammation by altering the gut microbiota and lipid metabolism in weaned piglets. J Nutr. (2021) 151:20–9. doi: 10.1093/jn/nxaa331
15. Yang JS, Zheng J, Fang XP, Jiang X, Sun YK, Zhang Y. Effects of dietary N-carbamylglutamate on growth performance, apparent digestibility, nitrogen metabolism and plasma metabolites of fattening Holstein bulls. Animals. (2021) 11:126. doi: 10.3390/ani11010126
16. Chemists A. Official Methods of Analysis: Changes in Official Methods of Analysis Made at the Annual Meeting. Supplement Association of Official Analytical Chemists (1990).
17. Van Soest PJ, Robertson JB, Lewis BA. Methods for dietary fiber, neutral detergent fiber, and nonstarch polysaccharides in relation to animal nutrition. J Dairy Sci. (1991) 74:3583–97. doi: 10.3168/jds.S0022-0302(91)78551-2
18. Senevirathne N, Anderson J, Metzger L. Growth performance, nutrient utilization, and health of dairy calves supplemented with condensed whey solubles. J Dairy Sci. (2019) 102:8108–19. doi: 10.3168/jds.2019-16314
19. Merchen NR. Digestion, Absorption and Excretion in Ruminants. Ruminant Animal Digestive Physiology & Nutrition (1988).
20. Zhu XL, Bi ZC, Yang C, Guo YH, Yuan JL, Li LJ, et al. Effects of different doses of omega-3 polyunsaturated fatty acids on gut microbiota and immunity. Food Nutr Res. (2021) 65:6263. doi: 10.29219/fnr.v65.6263
21. Zhao X, Fu HY, Qiu SN, Teng T, Shi BM. Effects of early protein restriction on the growth performance and gut development of pigs fed diets with or without antibiotic. Animal. (2019) 14:1392–401. doi: 10.1017/S1751731119002921
22. Polycarpo G, Burbarelli M, Carao A, Merseguel C, Dadalt J, Maganha S, et al. Effects of lipid sources, lysophospholipids and organic acids in maize-based broiler diets on nutrient balance, liver concentration of fat-soluble vitamins, jejunal microbiota and performance. Br Poult Sci. (2016) 57:788–98. doi: 10.1080/00071668.2016.1219019
23. Zampiga M, Meluzzi A, Sirri F. Effect of dietary supplementation of lysophospholipids on productive performance, nutrient digestibility and carcass quality traits of broiler chickens. Ital J Anim Sci. (2016) 15:521–8. doi: 10.1080/1828051X.2016.1192965
24. Jenkins T, Gimenez T, Cross D. Influence of phospholipids on ruminal fermentation in vitro and on nutrient digestion and serum lipids in sheep. J Anim Sci. (1989) 67:529–37. doi: 10.2527/jas1989.672529x
25. Lee C, Morris DL, Copelin JE, Hettick JM, Kwon IH. Effects of lysophospholipids on short-term production, nitrogen utilization, and rumen fermentation and bacterial population in lactating dairy cows. J Dairy Sci. (2019) 102:3110–20. doi: 10.3168/jds.2018-15777
26. Song WS, Yang J, Hwang IH, Cho S, Choi N-J. Effect of dietary lysophospholipid (Lipidol Tm) supplementation on the improvement of forage usage and growth performance in Hanwoo heifer. J Korean S Grassl Forage Sci. (2015) 35:232–7. doi: 10.5333/KGFS.2015.35.3.232
27. Jenkins K, Kramer J, Emmons D. Effect of lipids in milk replacers on calf performance and lipids in blood plasma, liver, and perirenal fat. J Dairy Sci. (1986) 69:447–59. doi: 10.3168/jds.S0022-0302(86)80423-4
28. Bai G, He W, Yang Z, Fu H, Qiu S, Gao F, et al. Effects of different emulsifiers on growth performance, nutrient digestibility, and digestive enzyme activity in weanling pigs. J Anim Sci. (2019) 97:4235–41. doi: 10.1093/jas/skz276
29. Liang L, Zhang X, Wang X, Jin Q, McClements DJ. Influence of dairy emulsifier type and lipid droplet size on gastrointestinal fate of model emulsions: in vitro digestion study. J Agric Food Chem. (2018) 66:9761–9. doi: 10.1021/acs.jafc.8b02959
30. Kim MJ, Hosseindoust AR, Choi YH, Kumar A, Jeon SM, Lee SH, et al. An evaluation of metabolizable energy content of main feed ingredients for growing pigs when adding dietary lysophospholipids. Livest Sci. (2018) 210:99–103. doi: 10.1016/j.livsci.2018.01.014
31. Boontiam W, Jung B, Kim Y. Effects of lysophospholipid supplementation to lower nutrient diets on growth performance, intestinal morphology, and blood metabolites in broiler chickens. Poult Sci. (2017) 96:593–601. doi: 10.3382/ps/pew269
32. Kelkar DA, Chattopadhyay A. The gramicidin ion channel: a model membrane protein. Biochim Biophys Acta. (2007) 1768:2011–25. doi: 10.1016/j.bbamem.2007.05.011
33. Tagesson C, Franzen L, Dahl G, Westrom B. Lysophosphatidylcholine increases rat ileal permeability to macromolecules. Gut. (1985) 26:369–77. doi: 10.1136/gut.26.4.369
34. Huo Q, Li B, Cheng L, Wu T, You P, Shen S, et al. Dietary supplementation of lysophospholipids affects feed digestion in lambs. Animals. (2019) 9:805. doi: 10.3390/ani9100805
35. Liu B, Wang W, Zhu X, Sun X, Xiao J, Li D, et al. Response of gut microbiota to dietary fiber and metabolic interaction with scfas in piglets. Front Microbiol. (2018) 9:2344. doi: 10.3389/fmicb.2018.02344
36. O'Callaghan T, Ross R, Stanton C, Clarke G. The gut microbiome as a virtual endocrine organ with implications for farm and domestic animal endocrinology. Domest Anim Endocrin. (2016) 56:S44–55. doi: 10.1016/j.domaniend.2016.05.003
37. Méndez-Salazar EO, Ortiz-López MG, Granados-Silvestre MdlÁ, Palacios-González B, Menjivar M. Altered gut microbiota and compositional changes in firmicutes and proteobacteria in mexican undernourished and obese children. Front Microbiol. (2018) 9:2693. doi: 10.3389/fmicb.2018.02693
38. Zhang J, Shi H, Wang Y, Cao Z, Yang H, Li S. Effect of limit-fed diets with different forage to concentrate ratios on fecal bacterial and archaeal community composition in Holstein heifers. Front Microbiol. (2018) 9:976. doi: 10.3389/fmicb.2018.00976
39. Thomas M, Webb M, Ghimire S, Blair A, Olson K, Fenske GJ, et al. Metagenomic characterization of the effect of feed additives on the gut microbiome and antibiotic resistome of feedlot cattle. Sci Rep. (2017) 7:12257. doi: 10.1038/s41598-017-12481-6
40. Shin NR, Whon TW, Bae JW. Proteobacteria: microbial signature of dysbiosis in gut microbiota. Trends Biotechnol. (2015) 33:496–503. doi: 10.1016/j.tibtech.2015.06.011
41. Reddy B, Dubey SK. Exploring the allochthonous pollution influence on bacterial community and co-occurrence dynamics of River Ganga water through 16S rRNA-tagged amplicon metagenome. Environ Sci Pollut Res. (2021) 28:26990–7005. doi: 10.1007/s11356-021-12342-w
42. Fang S, Chen X, Ye X, Zhou L, Xue S, Gan Q. Effects of gut microbiome and short-chain fatty acids (scfas) on finishing weight of meat rabbits. Front Microbiol. (2020) 11:1835. doi: 10.3389/fmicb.2020.01835
43. Stavropoulos SN, Friedel D, Modayil R, Parkman HP. Diagnosis and management of esophageal achalasia. BMJ. (2016) 354:i2785. doi: 10.1136/bmj.i2785
44. Hu J, Lin S, Zheng B, Cheung PCK. Short-chain fatty acids in control of energy metabolism. Crit Rev Food Sci. (2018) 58:1243–9. doi: 10.1080/10408398.2016.1245650
45. Li S, You J, Wang Z, Liu Y, Wang B, Du M, et al. Curcumin alleviates high-fat diet-induced hepatic steatosis and obesity in association with modulation of gut microbiota in mice. Food Res Int. (2021) 143:110270. doi: 10.1016/j.foodres.2021.110270
46. Guilloteau P, Martin L, Eeckhaut V, Ducatelle R, Zabielski R, Van Immerseel F. From the gut to the peripheral tissues: the multiple effects of butyrate. Nutr Res Rev. (2010) 23:366–84. doi: 10.1017/S0954422410000247
47. Jacquier V, Nelson A, Jlali M, Rhayat L, Brinch K, Devillard E. Bacillus subtilis 29784 induces a shift in broiler gut microbiome toward butyrate-producing bacteria and improves intestinal histomorphology and animal performance. Poult Sci. (2019) 98:2548–54. doi: 10.3382/ps/pey602
48. Ndou S, Tun H, Kiarie E, Walsh M, Khafipour E, Nyachoti C. Dietary supplementation with flaxseed meal and oat hulls modulates intestinal histomorphometric characteristics, digesta-and mucosa-associated microbiota in pigs. Sci Rep. (2018) 8:5880. doi: 10.1038/s41598-018-24043-5
Keywords: bacterial community, beef cattle, digestibility, growth performance, lysophospholipids
Citation: Zhang M, Bai H, Zhao Y, Wang R, Li G, Zhang Y and Jiao P (2022) Effects of supplementation with lysophospholipids on performance, nutrient digestibility, and bacterial communities of beef cattle. Front. Vet. Sci. 9:927369. doi: 10.3389/fvets.2022.927369
Received: 26 April 2022; Accepted: 30 June 2022;
Published: 22 July 2022.
Edited by:
Metha Wanapat, Khon Kaen University, ThailandReviewed by:
Wenzhu Yang, Agriculture and Agri-Food Canada (AAFC), CanadaAnusorn Cherdthong, Khon Kaen University, Thailand
Copyright © 2022 Zhang, Bai, Zhao, Wang, Li, Zhang and Jiao. This is an open-access article distributed under the terms of the Creative Commons Attribution License (CC BY). The use, distribution or reproduction in other forums is permitted, provided the original author(s) and the copyright owner(s) are credited and that the original publication in this journal is cited, in accordance with accepted academic practice. No use, distribution or reproduction is permitted which does not comply with these terms.
*Correspondence: Yonggen Zhang, emhhbmd5b25nZ2VuJiN4MDAwNDA7bmVhdS5lZHUuY24=; Peixin Jiao, cGVpeGluLmppYW8mI3gwMDA0MDtuZWF1LmVkdS5jbg==