- 1Schothorst Feed Research, Lelystad, Netherlands
- 2Hankkija Ltd, Hyvinkää, Finland
With the ban of zinc oxide (ZnO) at high dosages in piglet diets in Europe by 2022, alternative nutritional solutions are being tested to support piglet immune defence during their weaning, the most critical and stressful moment of pig production. The present study evaluated the effect of zinc oxide (ZnO; 2,500 mg/kg diet) and resin acid concentrate (RAC; 200 mg/kg diet) on the immune defence of weaned piglets challenged with lipopolysaccharide (LPS). Piglets were challenged at days 7 and 21 post-weaning, and blood was sampled 1.5 and 3.0 h after each challenge to determine serum levels of pro- and anti-inflammatory cytokines. The levels of serum tumour necrosis factor alpha (TNF-α) and interleukin 8 (IL-8) increased at days 7 and 21, and those of IL-6 at day 21 when challenged piglets were fed a diet supplemented with ZnO. In challenged piglets fed with RAC, the serum levels of IL-1β, IL-6, IL-8, IL-10 and TNF-α were increased at days 7 and 21, except for that of IL-1β, which was not affected at day 21. The increased levels of these cytokines indicate the successful immune-modulatory effect of ZnO and RAC, which appears as a candidate to replace ZnO in weaned piglets' diets.
Introduction
Weaning usually occurs 3 to 4 weeks after birth, which is a critical moment for piglets because they are suddenly exposed to several environmental, dietary and physiological stress conditions (1). These stresses on an immature digestive tract and immune system leads to post-weaning diarrhoea and reduced feed intake (2). Due to the abrupt lack of passive immunity acquired via maternal milk, these piglets are exposed to inflammatory processes (3). Moreover, active immunity has not yet fully established, so that immune support is warranted (4). Often, these piglets will experience dysbiosis and intestinal inflammation, factors that predispose them to gastrointestinal infections, which are linked to 17% of piglet mortality in Europe (5).
In the past, antibiotics were added to post-weaning diets to avoid post-weaning intestinal disturbances and to promote growth. However, this strategy was banned due to the risk of antimicrobial resistance, favouring the use of non-antimicrobial alternatives to support piglet health around weaning (6). One of the most effective nutritional strategies has been the supplementation of post-weaning diets with therapeutic levels of zinc oxide (ZnO) (7, 8). This essential trace element is often added to the piglet diet not only because of its antimicrobial properties but also because it improves body weight gain (BWG) and supports immunity (9). To achieve such effects, ZnO is generally added at doses ranging from 2,000 to 4,000 mg/kg diet (10, 11). The nutritional zinc requirement for piglets around weaning is lower (80–100 mg) (12) than that supplemented in the feed, resulting in Zn excretion in the faeces, which leads to environmental contamination. To avoid this, as of 2022 the supplementation of pig diets with therapeutic levels of ZnO will be banned in Europe (13). Hence, alternative feed additives should be able to support the intestinal integrity and immune system of piglets at weaning.
Among different feed additive alternatives, resin acid concentrates (RAC) appear promising because of their anti-inflammatory properties. Resin acids derived from coniferous trees are phytochemicals that have been used since ancient times in Asian and Scandinavian traditional human medicine for treating wounds (14, 15). Aguirre et al. (16) reported that supplementation of resin acids in broiler diets supported mucosal integrity via suppression of intestinal matrix metalloproteinase (MMP) activity, which degrades collagen fibres. In the same study, in-feed resin acids reduced duodenal inflammatory T-cell abundance. Supplementation of RAC in sow diets showed a beneficial effect on colostrum IgG levels (17). To test whether feed additives are able to support piglet health, immune system activation and growth, a model that can challenge the immune system is desirable. Among different challenge models, a lipopolysaccharide (LPS) challenge model in weaned piglets is chosen because of its elucidation of the integrated pathophysiology of infection and inflammation. The LPS molecule is present on the outer surface of all gramme-negative bacteria, is widely used as an immune stimulant and has been used to model bacterial infection in experimental farm animals (18).
In the organism, LPS can bind to many macromolecules, such as albumin and lipopolysaccharide binding protein (LBP) (19). The formed LBP-LPS complex is then transferred to the membrane-bound receptors or CD14, enabling interactions with Toll-like receptors (TLRs) on cell membranes (20). As a result, pro-inflammatory cytokines, including tumour necrosis factor alpha (TNF-α) and interleukins (ILs) IL-1β, IL-6, and IL-12 will be released via signalling through TLRs (21). Specifically, TNF-α is produced very early in inflammation followed by waves of IL-1β and then by IL-6. IL-10 is one of the most potent anti-inflammatory cytokines and is required for protection in many animal models of inflammation, and it has important roles in the regulation of gut homeostasis during host defence (22, 23). The association between IL-10 and inflammatory intestinal disease has been demonstrated in both humans and animal models (23). Cytokine and chemokine interactions are complex, but many previous studies only focused on two or three pro-inflammatory cytokines, including IL-1β, IL-6, and TNF-α (24), with or without the anti-inflammatory cytokine IL-10 (18). To properly interpret the effects of a dietary intervention on the inflammatory response, it is necessary to include other cytokines in the analysis, such as IL-8, IFN-α, IFN-γ, and IL-4. On this basis, the aim of the present study was to evaluate the effect of dietary resin acid concentrate (RAC) in comparison with ZnO on the immune responses of weaned piglets subjected to a LPS challenge.
Materials and Methods
Animals and Housing
In total, 48 healthy piglets, 24 females and 24 males (Tempo × Great Yorkshire and Landrace), with an average body weight of 7.5 ± 0.36 kg and an average age of 26 ± 0.9 days entered the experiment at the day of weaning. Before weaning, the piglets were fed creep feed from approximately day 7 of age, and immediately after weaning they were fed the experimental diets. A randomisation process was used to allocate piglets to replicates based on weaning weight. Piglets were housed in groups of six with half females and half males per pen (2.00 × 1.13 m) with partially slatted floors in climate-controlled units in the Schothorst Feed Research (SFR) weaner facility. Experimental diets were fed from weaning until 22 days post-weaning. The ambient temperature was gradually decreased from 29°C at the day of weaning to 22°C at 22 days post-weaning. Room temperature and relative humidity were recorded daily.
Diets and Experimental Design
Diets were prepared as mash and were formulated free of antimicrobial growth promoters. The nutrient content of the diets met the requirement according to the NRC recommendation (12), as shown in Supplementary Table 1. RAC is a feed additive powder with 37.5% resin acids dried onto 62.5% of food grade carrier, produced using a standardised manufacturing procedure by Hankkija Oy (Hyvinkää, Finland). The 200 mg/kg dose of RAC thus provided 75 mg resin acids per kg feed. A basal diet was prepared and divided into three portions. The first portion became diet A and was regarded as the negative control diet (NC). To the second portion (basis for diet B), 2,500 mg ZnO/kg feed was added and it became diet B (i.e., NC + ZnO). To the third portion, 200 mg RAC/kg feed was added, and it became diet C (i.e., NC + RAC).
The experiment was performed in a completely randomised block design with four treatments and 12 replicates, where each piglet was an experimental unit, as described in Table 1. In brief, the 48 piglets used in the present study were housed in groups of 6 piglets (3 female and 3 male piglets) per pen. Piglets from treatment 1 were challenged with an intramuscular (i.m.) injection of phosphate buffered saline (PBS), and piglets from treatments 2 to 4 were challenged with an i.m. injection of LPS (L2630—LPS from Escherichia coli 0111:B4, Sigma-Aldrich®, Saint Louis, MI, USA) at a dose of 50 μg/kg live body weight. Piglets from treatments 1 and 2 were fed diet A and were regarded as treatment PBS + NC and treatment LPS + NC, respectively. Piglets from treatment 3 were fed diet B and consisted of treatment LPS + ZnO. Piglets from treatment 4 were fed diet C, and it became treatment LPS + RAC.
Challenge
On days 7 and 21 post-weaning, piglets from treatments 2 to 4 were challenged via i.m. injection with LPS. Piglets from treatment 1 were used as negative control and were treated in a similar way to the LPS-challenged group, but PBS was used. On days 7 and 21 post-weaning, blood was collected by venepuncture of the jugular vein at 1.5 and 3.0 h post i.m. injection of PBS or LPS. All the piglets were sampled. The sampling moments of 1.5 and 3.0 h post-challenge were chosen because a response to LPS infection reaches its peak between 1.0 and 3.0 h and declines slowly afterwards (25). An extra blood sample was obtained on day 14, when no PBS or LPS was administered to piglets. Blood was collected in 5-mL vacutainer tubes, centrifuged (3,000 g for 10 min), and the harvested serum was stored at −80°C until analysis. Faecal samples were collected the day after the challenge day at days 8 and 22 post-weaning and were stored at −80°C until analysis.
Measurements
Performance and Health Status
Piglets were individually weighed at days 0 and 21 post-weaning. Feed intake per pen on day 21 post-weaning was recorded. Average daily gain (ADG) and the feed conversion ratio (FCR) were consequently calculated from days 0 to 21. Faecal consistency was visually recorded twice a week by an experienced panel on a scale from 1 (liquid faeces) to 8 (hard and dry faeces). Faecal score 6 was considered the optimal faecal score. To monitor the health status of all piglets, rectal temperature was measured at 6 h after the LPS injection on days 7 and 21, and on day 8. All piglets were observed daily for abnormal behaviour and clinical signs of illness. Medical treatments were registered.
Serum Analysis
Serum levels of IL-10, IL-1β, IL-6, TNF-α, IL-12, IL-8, IFN-α, IFN-γ, and IL-4 were analysed using the Luminex × MAP technology, a multiplexed sandwich immunoassay (Thermo Fisher Scientific Inc., Waltham, USA). The measurements were determined using the ProcartPlex Porcine Cytokine and Chemokine Panel 1 (catalogue number EPX090-60829-901) according to the manufacturer's instructions (Thermo Fisher Scientific Inc., Waltham, USA). The concentrations of the different cytokines were expressed as pg/mL and calculated according to a standard curve. The limit of detection level was set as 95% of the minimum detected value within the standard range. The limits of detection for IL-10, IL-1β, IL-6, IL-8, TNF-α, IL-12, IFN-α, IFN-γ, and IL-4 were 22.09, 4.03, 4.6, 10.96, 19.02, 11.36, 0.47, 38.01, and 0.54 pg/mL, respectively.
Faecal Analysis
Briefly, faeces were weighed and triturated in hexadecyltrimethylammonium bromide buffer solution (HTAB, Sigma-Aldrich, ST. Louis, USA) and centrifuged (1,000 g, 15 min, at 4°C) to obtain the faecal extract. Then, total peroxidase (TPO) activity was evaluated by a modified method of Bradley et al. (26) based on oxidation of o-dianisidine by hydrogen peroxide, with or without addition of faecal extract. The results were reported as TPO units/g faeces). Limit of quantification = 0.56 U/mg faeces.
Statistical Analysis
Statistical analysis was carried out with GenStat® for Windows (20th edition; VSN International, Hemel Hempstead, UK). All parameters were analysed by ANOVA with Fisher's least significant difference (LSD) to compare treatment means. Post-hoc multiple comparisons between treatment groups were tested via Tukey's test. For serum analysis the piglet was the experimental unit, classification factors included in the model for serum parameters were the treatment (1, 2, 3, and 4), day of challenge (7 and 21), time post-challenge (1.5 and 3.0 h) and their interactions (treatment × day of challenge × time post-challenge). Classification factors included in the model for performance and faecal score was the treatment (1, 2, 3, and 4), where the pen was the experimental unit. Values with P ≤ 0.05 were considered statistically significant.
Results
General Observations
All piglets were healthy prior to LPS challenge. After the LPS challenge, piglets developed signs including vomiting, loss of activity and lethary. All the piglets recovered to normal within 24 h after the LPS challenge.
The growth performance at pen level is presented in Table 2. No differences in body weight were observed among experimental treatments. Likewise, no differences in performance were observed in the overall experimental period. The faecal score was similar among experimental treatments, except that the LPS-challenged piglets fed with the ZnO diet showed dryer faeces.
The rectal temperature of individual piglets is presented in Figure 1. On day 7, the rectal temperature of piglets who received the LPS challenge increased substantially compared with that of piglets who only received PBS (P = 0.006). On day 8, there were no differences in rectal temprature across the treaments. On day 21, piglets from treatments LPS + NC and LPS + RAC had the highest rectal temprature, followed by piglets from the treatment LPS + ZnO, and piglets from treament PBS + NC showed the lowest rectal temprautre (P = 0.002).
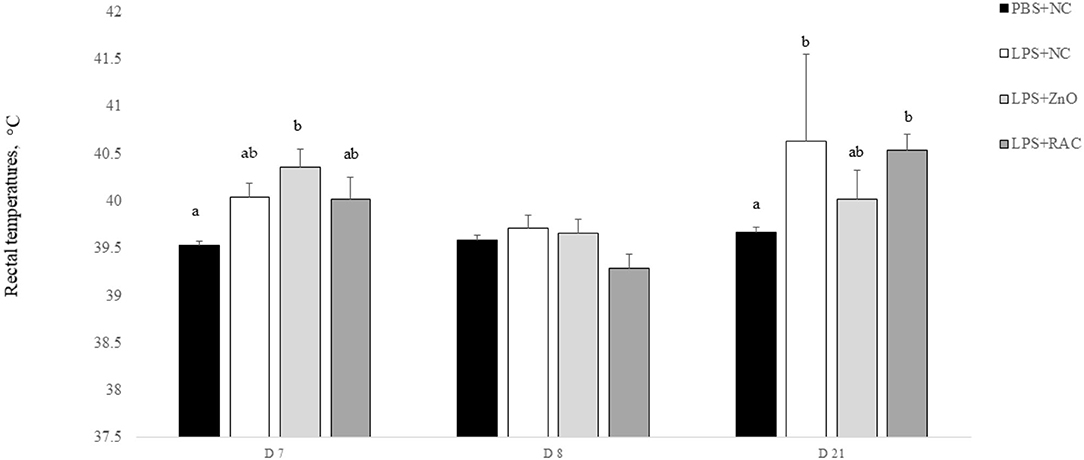
Figure 1. Mean (±SEM) rectal temperature of piglets from the PBS+NC, LPS+NC, LPS + ZnO, and LPS + RAC groups. a,b Different superscripts indicate significant differences among treatments per sampling day (P ≤ 0.05)1. 1Rectal temperature was measured in all piglets at 6 h after the LPS injection on days 7 and 21, and on day 8.
Serum Cytokine Profile
At day 14, all the assessed cytokines were at basal levels. Therefore, statistical analysis considered data from days 7 and 21, at both time points, i.e., 1.5 and 3.0 h post-challenge. Serum levels of IL-4, IFN-α, and IFN-γ were below the limit of detection. Serum level of IL-1β was below the limit of detection for 80% of the piglets in all the treatments.
Figure 2 depicts the serum levels of IL-6, IL-8, IL-10, IL-12, and TNF-α. A significant interaction between treatments and days on the IL-6 level was observed (P = 0.004). At day 7 and day 21, IL-6 serum levels were significantly higher in piglets from treatments LPS + ZnO and LPS + RAC compared with piglets from treatments PBS + NC and LPS + NC. At day 7, piglets from treatment LPS + RAC showed higher serum IL-6 than piglets from treatment LPS + ZnO. However, at day 21, piglets from treatments LPS + RAC and LPS + ZnO showed similar serum IL-6 level. For IL-8, a significant effect of the experimental treatment (P < 0.001), day (P = 0.005) and time (P = 0.032) were observed. Piglets from treatment LPS + RAC showed the highest levels of serum IL-8, followed by piglets from LPS + ZnO, and piglets from PBS + NC and LPS + NC showed the lowest serum IL-8 levels. IL-8 levels were higher at day 7 than day 21, and were higher at 1.5 h than 3 h after sampling. A significant interaction between the experimental treatment and sampling time was observed on the IL-10 serum level (P < 0.001), and no day effect was observed. Serum levels of IL-10 were significantly increased only in LPS-challenged piglets fed RAC diets sampled 1.5 h after the challenge. The serum IL-12 level was significantly higher in piglets from treatment LPS + RAC than in the other treatments (P < 0.001). For TNF-α, there were significant interactions between the treatment and sampling time (P < 0.001), and between the day and sampling time (P = 0.020). At day 7, TNF-α serum levels were significantly increased in challenged piglets fed the RAC or ZnO diets, and sampled 1.5 h after challenge. Similar effects were observed at day 21, where challenged piglets fed the NC diet also presented increased TNF-α serum levels, but significantly lower than those piglets from treatments LPS + ZnO and LPS + RAC.
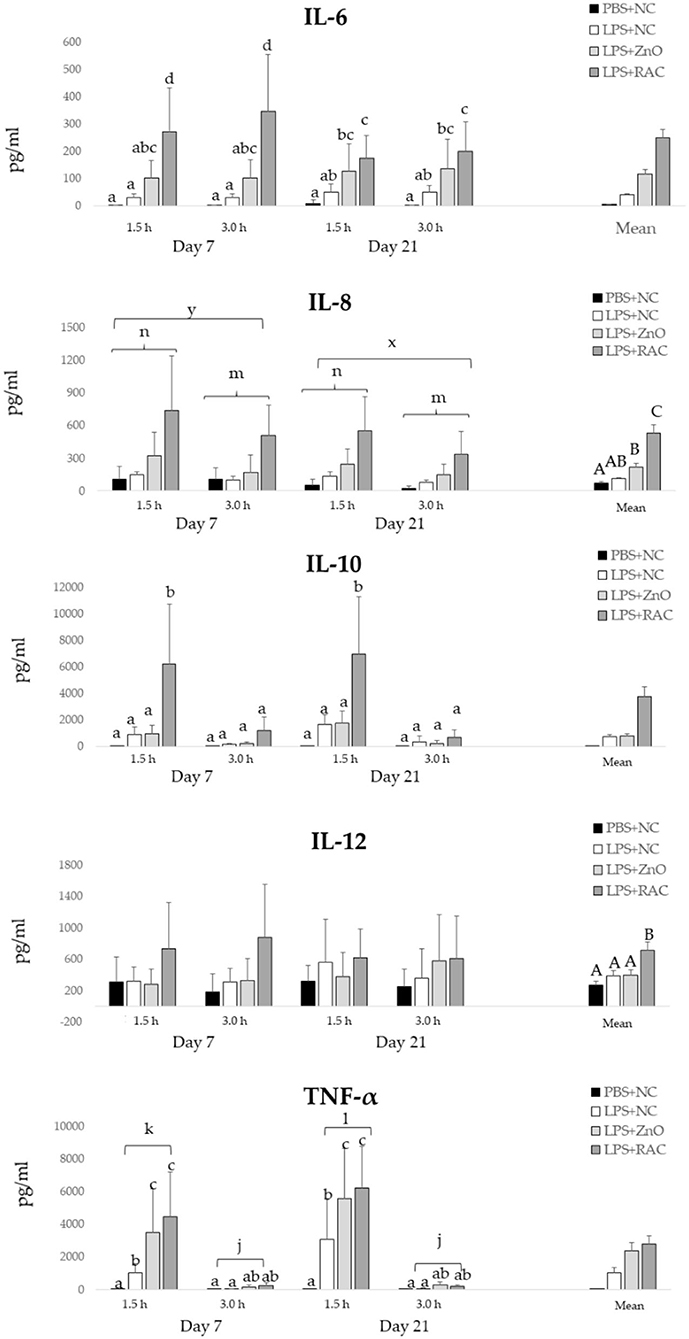
Figure 2. Mean (±SEM) serum levels of IL-6, IL-8, IL-10, IL-12, and TNF-α in piglets from the PBS+NC, LPS+NC, LPS + ZnO, and LPS + RAC groups. a−d Different superscripts indicate significant differences among treatments and day or sampling time (P ≤ 0.05), A−C Different superscripts indicate significant differences among treatments (P ≤ 0.05), x,y Different superscripts indicate significant differences among days (P ≤ 0.05), m,n Different superscripts indicate significant differences among sampling time (P ≤ 0.05), j−l Different superscripts indicate significant differences among day and sampling time (P ≤ 0.05)1. 1Blood samples were collected from all piglets (n = 48) on days 7 and 21 post-weaning, and at 1.5 and 3.0 h post i.m. injection of PBS or LPS.
Faecal TPO Activity
TPO activity in the faeces are presented in Supplementary Figure 1. No treatment or day effect was found. On day 8, the faecal TPO activity were 5.4, 4.6, 4.7, and 4.7 U/mL for treatments PBS+NC, LPS+NC, LPS+ZnO, and LPS+RAC, respectively. On day 22, the faecal TPO activity was numerically the greatest in treatment LPS+NC (7.4 U/mL), and it was 5.6, 5.9, and 6.3 U/mL for treatments PBS+NC, LPS+ZnO, and LPS+RAC, respectively.
Discussion
In the present study, we demonstrated an immune-modulatory effect when LPS challenged piglets were fed diets containing ZnO or RAC. All the piglets were obtained from the same commercial farm, with the same genetics, and were randomised based on litter, weaning body weight and gender, to guarantee a similar health and immune background. Moreover, they were housed in the same room and the environment was similar, supporting that the present data resulted from dietary intervention.
Although the performance analysis was used as a secondary and indicative parameter only, the similar feed intake and growth regardless of the treatments showed that the present challenge was mild. In general, it is accepted that activation of the immune system will require extra resources, otherwise the growth performance of piglets might be negatively affected. Numerically, piglets fed with LPS + ZnO apparently have a better performance in comparison with those fed with other diets. It is important to bear in mind that this trial was performed with a limited number of piglets to evaluate the effect of treatments on animal performance, and these data are only preliminary. To determine the dietary effects on growth, a larger number of replicates per treatment should be used. So, the main objective of this LPS challenge was to analyse the effect of different diets on immune system activation at systemic level. Increased faecal TPO activity indicates gut inflammation in weaned piglets (27). Only a numerically increased TPO activity was found in piglets challenged with LPS at the end of the study, suggesting that the current LPS challenge was not able to cause immediate inflammation on the gut level.
Both ZnO and RAC present immunomodulatory activity, as previously demonstrated. In vivo studies showed that dietary ZnO decreased intestinal inflammation in piglets by directly interfering with the expression of cytokines (28). Moreover, ZnO not only modulates cytokines, but acts in the development and function of the immune system (9, 29). Recently, the immunomodulatory properties of RAC was reported, by modulating gut microbiota and reducing inflammatory biomarkers (30). A direct anti-inflammatory effect of resin acid was demonstrated in mice lungs submitted to allergic inflammation (31), as well as by acting as a ligand for PPARs in macrophages and adipocytes (32). In-feed resin acids have inhibited the inflammation-associated collagen breakdown in the jejunum and ileum, and reduced the numbers of inflammatory T-cells in the duodenum of broiler chickens (16).
At 6 h after the LPS challenge on days 7 and 21, piglets that received the LPS challenge showed fever, and their rectal temperatures were above 40°C. The observed values are similar to those found in other studies using LPS as an immune system stimulator in pigs (33, 34). Within 24 h, the rectal temperature recovered, consistent with a previous study (34). The cytokine production profile after the LPS challenge was time dependent. Previous studies found that the peak level of the pro-inflammatory cytokine TNF-α occurred at 1 h after the LPS challenge, decreasing to pre-challenge levels at ~3 h. Another pro-inflammatory cytokine, IL-6, peaked ~3 h after the LPS challenge and subsequently fell at ~6 h (34, 35). The current study demonstrated a consistent pattern of TNF-α and IL-6 production in response to the LPS challenge. Stimulation of the innate immune system activates the production of pro-inflammatory cytokines. Activated macrophages, monocytes, neutrophils, and other cells are able to produce TNF-α, which initiates the inflammatory response but also limits the extent and duration of the inflammatory process (36). Such a balance is confirmed by the concomitant production of the anti-inflammatory cytokine IL-10, which is also produced by macrophages, monocytes and B and T cells. Alterations in the balance of TNF-α and IL-10 production will affect macrophage function and cell survival (37).
Among LPS-challenged piglets, supplementation of ZnO at 2,500 mg/kg diet or with RAC at 200 mg/kg stimulated TNF-α at days 7 and 21 (1.5 h post-challenge) and the production of IL-8 and IL-6, regardless of challenge day and sampling time. The effect of RAC supplementation on IL-6 and IL-8 was stronger than that of ZnO supplementation, but similar effects on TNF-α were found. Interestingly, among LPS-challenged piglets, IL-10 production was only increased in piglets fed the RAC diet at days 7 and 21 (1.5 h post-challenge). The increased levels of these cytokines indicate the successful immune modulatory effect of both ZnO and RAC, with some differences between the two. Both TNF-α and IL-10 were stimulated in the first 1.5 h post-challenge. Given the fact that the challenged piglets fed supplemented diets were not more ill than those fed the control diet and no differences in animal performance were observed, it can be suggested that both ZnO and RAC supported this pro- and anti-inflammatory balance. Notably, ZnO did not stimulate higher IL-10 production than the control diet. Increased IL-10 has been related to improved intestinal barrier function (38). However, one must bear in mind that the present study evaluated systemic response.
Scheller et al. (39) demonstrated that although IL-6 is a pro-inflammatory cytokine, it also has regenerative activities that, if absent, would result in a more aggravated inflammatory process. Piglets fed RAC-supplemented diets showed an increase in IL-6 serum levels, followed by those fed ZnO, indicating that immune modulation was taking place during the challenge and that probably the RAC were able to counteract the LPS challenge as well as support tissue regeneration. Regarding IL-8, also called chemokine CXCL8, this cytokine is particularly important in aiding migration of additional neutrophils to inflammatory sites (40). Challenged piglets fed a diet supplemented with RAC showed the strongest IL-8 activation, followed by piglets fed the ZnO diet. Again, this is an indication that RAC has a robust immune modulatory effect in LPS-challenged piglets.
Conclusions
In conclusion, an immune-modulatory effect is observed in LPS-challenged piglets fed a diet supplemented with 2,500 mg ZnO/kg or 200 mg RAC/kg. Based on the serum cytokine profile analysis, the stimulation of anti- and pro-inflammatory cytokines was more pronounced in piglets fed a diet supplemented with 200 mg RAC/kg than in those fed the diet supplemented with 2,500 mg ZnO/kg.
Data Availability Statement
The original contributions presented in the study are included in the article/supplementary material, further inquiries can be directed to the corresponding author/s.
Ethics Statement
The study was conducted according to the restrictions of the Animal and Human Welfare Codes in The Netherlands, and approved by the Ethics Committee and Institutional Review Board of Schothorst Feed Research (AVD246002015279, June 3rd, 2019).
Author Contributions
XG and FM: conceptualization and funding acquisition. XG, HK, JV, and FM: methodology. XG, RS, and FM: validation and writing—original draft preparation. XG and RS: formal analysis. XG: investigation and project administration. FM, HK, and JV: resources. XG, RS, HK, JV, and FM: writing—review and editing. All authors have read and agreed to the published version of the manuscript.
Conflict of Interest
HK and JV were employed by company Hankkija Ltd.
The remaining authors declare that the research was conducted in the absence of any commercial or financial relationships that could be construed as a potential conflict of interest.
Publisher's Note
All claims expressed in this article are solely those of the authors and do not necessarily represent those of their affiliated organizations, or those of the publisher, the editors and the reviewers. Any product that may be evaluated in this article, or claim that may be made by its manufacturer, is not guaranteed or endorsed by the publisher.
Acknowledgments
The authors thank the animal caretakers, laboratory technicians and project office team from SFR for the administrative and technical support.
Supplementary Material
The Supplementary Material for this article can be found online at: https://www.frontiersin.org/articles/10.3389/fvets.2021.761742/full#supplementary-material
References
1. Revilla M, Friggens NC, Broudiscou LP, Blanc F, Ravon L, Mercat MJ, et al. Towards the quantitative characterisation of piglets' robustness to weaning: a modelling approach. Animal. (2019) 13:2536–46. doi: 10.1017/S1751731119000843
2. Zhou J, Dong Z, Wan D, Wang Q, Haung J, Huang P, et al. Effects of iron on intestinal development and epithelial maturation of suckling piglets. J Anim Sci. (2020) 98:213. doi: 10.1093/jas/skaa213
3. Pié S, Lallès JP, Blazy F, Laffitte J, Sève B, Oswald IP. Weaning is associated with an upregulation of expression of inflammatory cytokines in the intestine of piglets. J Nutr. (2004) 134:641–7. doi: 10.1093/jn/134.3.641
4. Blecha F, Pollman DS, Nichols DA. Weaning pigs at an early age decreases cellular immunity. J Anim Sci. (1983) 56:396–400. doi: 10.2527/jas1983.562396x
5. Gresse R, Chaucheyras-Durand F, Fleury MA, van de Wiele T, Forano E, Blanquet-Diot S. Gut microbiota dysbiosis in postweaning piglets: understanding the keys to health. Trends Microbiol. (2017) 25:851–73. doi: 10.1016/j.tim.2017.05.004
6. Lin J, Nishino K, Roberts MC, Tolmasky M, Aminov RI, Zhang L. Mechanisms of antibiotic resistance. Front Microbiol. (2015) 6:34. doi: 10.3389/fmicb.2015.00034
7. Starke IC, Pieper R, Neumann K, Zentek J, Vahjen W. The impact of high dietary zinc oxide on the development of the intestinal microbiota in weaned piglets. FEMS Microbiol. Ecol. (2014) 87:416–27. doi: 10.1111/1574-6941.12233
8. Vahjen W, Pieper R, Zentek J. Increased dietary zinc oxide changes the bacterial core and enterobacterial composition in the ileum of piglets. J Anim Sci. (2011) 89:2430–9. doi: 10.2527/jas.2010-3270
9. Bonetti A, Tugnoli B, Piva A, Grilli E. Towards zero zinc oxide: feeding strategies to manage post-weaning diarrhea in piglets. Animals. (2021) 11:642. doi: 10.3390/ani11030642
10. Poulsen HD. Zinc oxide for weanling piglets. Acta Agric Scand Sect A Anim Sci. (1995) 45:159–67. doi: 10.1080/09064709509415847
11. Hu CH, Song J, You ZT, Luan ZS Li WF. Zinc oxide-Montmorillonite hybrid influences diarrhea, intestinal mucosal integrity, and digestive enzyme activity in weaned pigs. Biol Trace Elem Res. (2012) 149:190–6. doi: 10.1007/s12011-012-9422-9
12. NRC. National Research Council: Nutrient Requirements of Swine. 11th revised ed. Washington, DC: The National Academies Press (2012).
13. Kociova S, Dolezelikova K, Horky P, Skalickova S, Baholet D, Bozdechova L, et al. Zinc phosphate-based nanoparticles as alternatives to zinc oxide in diet of weaned piglets. J Anim Sci Biotechnol. (2020) 11:59. doi: 10.1186/s40104-020-00458-x
14. Sipponen A, Kuokkanen O, Tiihonen R, Kauppinen H, Jokinen JJ. Natural coniferous resin salve used to treat complicated surgical wounds: pilot clinical trial on healing and costs. Int J Dermatol. (2012) 51:726–32. doi: 10.1111/j.1365-4632.2011.05397.x
15. Jokinen JJ, Sipponen A. Refined spruce resin to treat chronic wounds: rebirth of an old folkloristic therapy. Adv Wound Care. (2016) 5:198–207. doi: 10.1089/wound.2013.0492
16. Aguirre M, Vuorenmaa J, Valkonen E, Kettunen H, Callens C, Haesebrouck F, et al. In-feed resin acids reduce matrix metalloproteinase activity in the ileal mucosa of healthy broilers without inducing major effects on the gut microbiota. Vet Res. (2019) 50:15. doi: 10.1186/s13567-019-0633-3
17. Hasan S, Saha S, Junnikkala S, Orro T, Peltoniemi O, Oliviero C. Late gestation diet supplementation of resin acid-enriched composition increases sow colostrum immunoglobulin G content, piglet colostrum intake and improve sow gut microbiota. Animal. (2019) 13:1599–606. doi: 10.1017/S1751731118003518
18. Adewole DI, Kim IH, Nyachoti CM. Gut health of pigs: challenge models and response criteria with a critical analysis of the effectiveness of selected feed additives—a review. Asian-Australas J Anim Sci. (2016) 29:909–24. doi: 10.5713/ajas.15.0795
19. Schumann RR. Recognition of bacterial endotoxin and modulation of the inflammatory response: the LBP/CD14 pathway during the acute phase. Sepsis. (1998) 2:149–55.
20. Triantafilou M, Triantafilou K. Lipopolysaccharide recognition: CD14, TLRs and the LPS-activation cluster. Trends Immunol. (2002) 23:301–4. doi: 10.1016/S1471-4906(02)02233-0
21. Medzhitov R. Toll-like receptors and innate immunity. Nat Rev Immunol. (2001) 1:135–45. doi: 10.1038/35100529
22. Mazmanian SK, Round JL, Kasper DL. A microbial symbiosis factor prevents intestinal inflammatory disease. Nature. (2008) 453:620. doi: 10.1038/nature07008
23. Maloy KJ, Powrie F. Intestinal homeostasis and its breakdown in inflammatory bowel disease. Nature. (2011) 474:298–306. doi: 10.1038/nature10208
24. Han J, Bian L, Liu X, Zhang F, Zhang Y, Yu N. Effects of Acanthopanax senticosus polysaccharide supplementation on growth performance, immunity, blood parameters and expression of pro-inflammatory cytokines genes in challenged weaned piglets. Asian Australas J Anim Sci. (2014) 27:1035. doi: 10.5713/ajas.2013.13659
25. Brückmann R, Tuchscherer M, Tuchscherer A, Gimsa U, Kanitz E. Early-life maternal deprivation predicts stronger sickness behaviour and reduced immune responses to acute endotoxaemia in a pig model. Int J Mol Sci. (2020) 21:5212. doi: 10.3390/ijms21155212
26. Bradley PP, Christenson RD, Rothstein G. Cellular and extra cellular myeloperoxidase in pyogenic inflammation. Blood. (1982) 1:618–22. doi: 10.1182/blood.V60.3.618.bloodjournal603618
27. Lemoine N, Fautrel A, Techer C, Guillou C. Validation of a quantitative biomarker of gut inflammation in weaned piglets. In: Proceedings of 14th International Symposium on Digestive Physiology of Pigs, Poster 250. Brisbane, QLD (2018).
28. Grilli E, Tugnoli B, Vitari F, Domeneghini C, Morlacchini M, Piva A, et al. Low doses of microencapsulated zinc oxide improve performance and modulate the ileum architecture, inflammatory cytokines and tight junctions expression of weaned pigs. Animal. (2015) 9:1760–8. doi: 10.1017/S1751731115001329
29. Gammoh NZ, Rink L. Zinc and the immune system. In: Nutrition and Immunity. Cham: Springer International Publishing (2019). pp. 127–58.
30. Uddin MK, Hasan S, Mahmud MR, Peltoniemi O, Oliviero C. In-feed supplementation of resin acid-enriched composition modulates gut microbiota, improves growth performance, and reduces post-weaning diarrhea and gut inflammation in piglets. Animals. (2021) 11:2511. doi: 10.3390/ani11092511
31. Gao Y, Zhaoyu L, Xiangming F, Chunyi L, Jiayu P, Lu S, et al. Abietic acid attenuates allergic airway inflammation in a mouse allergic asthma model. Int Immunopharmacol. (2016) 38:261–6. doi: 10.1016/j.intimp.2016.05.029
32. Kang M-S, Hirai S, Goto T, Kuroyanagi K, Lee J-Y, Uemura T, et al. Dehydroabietic acid, a phytochemical, acts as ligand for PPARs in macrophages and adipocytes to regulate inflammation. Biochem Biophys Res Commun. (2008) 369:333–8. doi: 10.1016/j.bbrc.2008.02.002
33. Rakhshandeh A, de Lange CF. Evaluation of chronic immune system stimulation models in growing pigs. Animal. (2012) 6:305–10. doi: 10.1017/S1751731111001522
34. Petry A, McGilvray W, Rakhshandeh AR, Rakhshandeh A. Technical note: assessment of an alternative technique for measuring body temperature in pigs. J Anim Sci. (2017) 95:3270–4. doi: 10.2527/jas.2017.1566
35. Frank JW, Carroll JA, Allee GL, Zannelli ME. The effects of thermal environment and spray-dried plasma on the acute-phase response of pigs challenged with lipopolysaccharide. J Anim Sci. (2003) 81:1166–76. doi: 10.2527/2003.8151166x
36. Akdis M, Aab A, Altunbulakli C, Azkur K, Costa RA, Crameri R, et al. Interleukins (from IL-1 to IL-38), interferons, transforming growth factor β, and TNF-α: receptors, functions, and roles in diseases. J Allergy Clin. Immunol. (2016) 138:984–1010. doi: 10.1016/j.jaci.2016.06.033
37. Rojas M, Olivier M, Gros P, Barrera LF, Garcia LF. TNF-alpha and IL-10 modulate the induction of apoptosis by virulent Mycobacterium tuberculosis in murine macrophages. J Immunol. (1999) 162:6122–33.
38. Liu P, Pieper R, Rieger J, Vahjen W, Davin R, Plendl J, et al. Effect of dietary zinc oxide on morphological characteristics, mucin composition and gene expression in the colon of weaned piglets. PLoS ONE. (2014) 9:e91091. doi: 10.1371/journal.pone.0091091
39. Scheller J, Chalaris A, Schmidt-Arras D, Rose-John S. The pro- and anti-inflammatory properties of the cytokine interleukin-6. Biochim Biophys Acta. (2011) 1813:878–8. doi: 10.1016/j.bbamcr.2011.01.034
Keywords: weaning, stress, piglets, infection, zinc oxide, resin acid concentrate
Citation: Guan X, Santos RR, Kettunen H, Vuorenmaa J and Molist F (2021) Effect of Resin Acid and Zinc Oxide on Immune Status of Weaned Piglets Challenged With E. coli Lipopolysaccharide. Front. Vet. Sci. 8:761742. doi: 10.3389/fvets.2021.761742
Received: 20 August 2021; Accepted: 26 November 2021;
Published: 23 December 2021.
Edited by:
Shihai Zhang, South China Agricultural University, ChinaReviewed by:
Jai-Wei Lee, National Pingtung University of Science and Technology, TaiwanKai Qiu, Chinese Academy of Agricultural Sciences (CAAS), China
Copyright © 2021 Guan, Santos, Kettunen, Vuorenmaa and Molist. This is an open-access article distributed under the terms of the Creative Commons Attribution License (CC BY). The use, distribution or reproduction in other forums is permitted, provided the original author(s) and the copyright owner(s) are credited and that the original publication in this journal is cited, in accordance with accepted academic practice. No use, distribution or reproduction is permitted which does not comply with these terms.
*Correspondence: Xiaonan Guan, xguan@schothorst.nl; Regiane R. Santos, RSantos@schothorst.nl