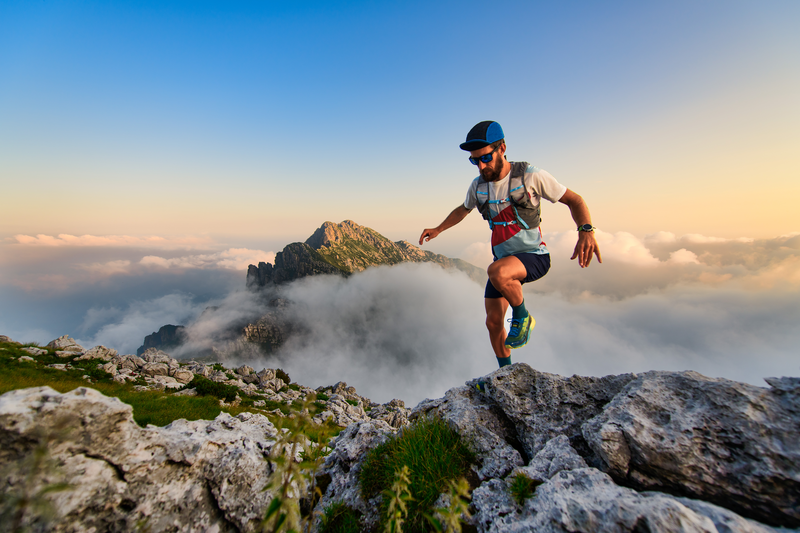
95% of researchers rate our articles as excellent or good
Learn more about the work of our research integrity team to safeguard the quality of each article we publish.
Find out more
ORIGINAL RESEARCH article
Front. Vet. Sci. , 07 January 2022
Sec. Animal Nutrition and Metabolism
Volume 8 - 2021 | https://doi.org/10.3389/fvets.2021.813629
This article is part of the Research Topic Natural Feed Additives in Animal Nutrition – Their Potential as Functional Feed View all 22 articles
Microcin C7 is an antimicrobial peptide produced by Escherichia coli, composed of a heptapeptide with a modified adenosine monophosphate. This study was performed to evaluate the effects of Microcin C7 as a potential substrate to traditional antibiotics on growth performance, immune functions, intestinal barrier, and cecal microbiota of broilers. In the current study, 300 healthy Arbor Acres broiler chicks were randomly assigned to one of five treatments including a corn–soybean basal diet and basal diet supplemented with antibiotic or 2, 4, and 6 mg/kg Microcin C7. Results showed that Microcin C7 significantly decreased the F/G ratio of broilers; significantly increased the levels of serum cytokine IL-10, immunoglobulins IgG and IgM, and ileal sIgA secretion; significantly decreased the level of serum cytokine TNF-α. Microcin C7 significantly increased villus height and V/C ratio and significantly decreased crypt depth in small intestine of broilers. Microcin C7 significantly increased gene expression of tight junction protein Occludin and ZO-1 and significantly decreased gene expression of pro-inflammatory and chemokine TNF-α, IL-8, IFN-γ, Toll-like receptors TLR2 and TLR4, and downstream molecular MyD88 in the jejunum of broilers. Microcin C7 significantly increased the number of Lactobacillus and decreased the number of total bacteria and Escherichia coli in the cecum of broilers. Microcin C7 also significantly increased short-chain fatty acid (SCFA) and lactic acid levels in the ileum and cecum of broilers. In conclusion, diet supplemented with Microcin C7 significantly improved growth performance, strengthened immune functions, enhanced intestinal barrier, and regulated cecal microbiota of broilers. Therefore, the antimicrobial peptide Microcin C7 may have the potential to be an ideal alternative to antibiotic.
Antibiotics are used widely in livestock production as an effective antimicrobial drug because they play important roles in disease treatment, disease prevention, and growth promotion (1, 2). Global consumption of antimicrobials in livestock production was approximately 131,109 tons in 2013 and is projected to be 200,235 tons by 2030 (3). Antibiotic use brings enormous economic benefit, but excessive use can lead to development of antimicrobial resistance and drug residues in animal products consumed by humans (1, 4). Antimicrobial resistance makes treatment of bacterial infections with antibiotics less effective and creates a significant threat to the health of livestock and humans (5, 6). Moreover, the number of newly developed and approved antibiotics is decreasing dramatically (7). Therefore, it is of great urgency to find novel alternatives for antibiotics in livestock production.
AMPs are small cationic molecules with amphipathic structures, widely distributed in living organisms (8, 9). AMPs have a broad-spectrum antimicrobial activity against bacteria, fungi, viruses, and protozoa (9). Different from traditional antibiotics, AMPs kill pathogens by lysing cytomembranes or damaging critical intracellular targets (10, 11), which makes it difficult for pathogens to develop antimicrobial resistance (12). In addition to direct bactericidal activity, AMPs are important constituents of the innate immune system and serve as immune regulators. As part of the immune system, AMPs express chemotactic activities, modulate TLR-dependent inflammatory responses, and promote wound healing (9, 13). AMPs secreted by Paneth cells are of great importance in the maintenance of host intestinal health by limiting pathogen colonization and shaping the composition of indigenous microbial communities (14). The impairment of intestinal epithelial barrier and intestinal microecology caused by Paneth cell dysfunction is one of the important pathogenies of inflammatory bowel diseases, such as Crohn's disease and colitis (15). AMPs have important application value in the poultry industry and were reported to have beneficial effect on growth performance, nutrient digestibility, immune functions, and intestinal morphology, integrity, and microflora in broilers (16–18). Hence, AMPs can be potential antibiotic alternatives, which have attracted researchers' interests.
Microcin C7 is a ribosome synthetic heptapeptide with a modified adenosine monophosphate covalently attached to the C-terminus (19), produced by Escherichia coli cells harboring a plasmid-borne mccABCDE (20). Microcin C7 acts as a ‘Trojan horse’ antimicrobial peptide and exerts its antimicrobial activity by specifically targeting aspartyl-adenylate, which inhibits protein synthesis (21). Nanomolar concentrations of Microcin C7 exhibit antimicrobial activity against gram-negative strains phylogenetically similar to E. coli, including Klebsiella, Salmonella, Shigella, and one strain of Proteus (22). The features of high safety, stability, and strong antimicrobial ability make Microcin C7 a practical antimicrobial agent that can be applied in the poultry industry, but little is known about its efficacy at present. Hence, the purpose of this study was to determine the effect of dietary supplementation of Microcin C7 on growth performance, immune and intestinal barrier functions, and cecal microbiota of broilers.
In this study, chicks were reared on net-floor cages in closed and ventilated environment. Ten chicks were reared per pen (100 cm × 100 cm × 30 cm) with a separate feeding trough and nipple drinkers. A 24 h constant-lighting program was used. Broilers had ad libitum access to feed and water. Room temperature in the first week was controlled between 30 and 34°C, lowered by 3°C each week until a constant temperature of 22°C. Relative humidity was controlled between 50 and 60%. All chicks were inoculated with ND-IB combined vaccine on the 7th day, infectious bursa vaccine on the 14th day, chicken pox vaccine on the 21st day, and Newcastle disease vaccine on the 35th day. The experiment was carried out in the Fengguangde experimental base of the TIEQILISHI Group, Mianyang, Sichuan Province. The animal care protocol was approved by the China Agricultural University Animal Care and Use Committee (No. AW03901202-1-2), and the experiment is conducted in strict accordance to the animal care protocol. The basal diet (Table 1) was antibiotic free and formulated to meet nutritional requirements of broiler chickens recommended by NRC (2012). Coccidiostats were included in the diet.
One-day-old healthy Arbor Acres broiler chickens (n = 300; initial body weight (BW) = 46.67 ± 0.29 g) were obtained from Chengdu Xinjin Yunda poultry breeding cooperative. All chicks were individually weighed and randomly assigned to 1 of 5 treatments with 6 replicates of 10 broilers. Experimental treatments included a corn–soybean basal diet with no additions, a basal diet supplemented with 45 mg/kg chlortetracycline and 30 mg/kg bacitracin methylene disalicylate, and a basal diet supplemented with 2, 4, or 6 mg/kg Microcin C7. Microcin C7, which was isolated from the metabolites of Lactobacillus johnsonii by microbial fermentation, was supplied by Angeli (Chongqing) Biotechnology, Co. Ltd (Chongqing, China). The concentration of dietary Microcin C7 was determined based on findings of our previous studies (not published).
The feeding period was divided into two stages: the starter stage (Day 1–Day 21) and the finisher stage (Day 22–Day 42). Broilers were weighed individually at the start of the trial and the end of each phase prior to the morning feeding. Feed consumption was measured at the end of each phase. All feed remaining in the feeder was weighed and subtracted from the daily allowance to determine the actual daily feed intake. The ADG, ADFI, and F/G ratio were calculated. After weighing, two broilers per pen were randomly selected, euthanized, and sampled. Blood was collected under wings, then let stand for 10 min, and centrifuged at 3,000 rpm for 15 min at 4°C, and the supernatant was collected and stored at −20°C for subsequent serum biochemical parameter measurements. After exsanguination from the jugular vein, feathers were removed, and the abdominal cavity was opened. Samples (2 cm) of the duodenum, jejunum, and ileum were excised and washed in PBS and immediately fixed in 4% (v/v) paraformaldehyde solution for characterization of small intestinal morphology. The jejunal and ileal mucosa (about 0.2 g) were scraped, and scrapings were placed in a sterile 2 ml cryopreservation tube, frozen rapidly with liquid nitrogen, and then stored at −80°C for gene expression and total sIgA analysis. Contents of jejunum, ileum, and cecum were removed and placed in a sterile 2 ml cryopreservation tube, frozen rapidly with liquid nitrogen, and then stored at −80°C for microflora measurement.
Serum samples were thawed and thoroughly mixed immediately before testing. Concentrations of serum immunoglobulins A, G, and M and cytokines IFN-γ, IL-1β, IL-10, and TNF-α were measured using commercially available avian ELISA kits (Nanjing Jiancheng Bioengineering Institute, Nanjing, Jiangsu, China), according to standard procedures described by the manufacturer.
Embedded tissue was deparaffinized and hydrated, cut into slices (5 μm), and then stained with hematoxylin and eosin for morphology measurements as described by Wang et al. (23). Villus height was measured from the tip of the villus to the crypt–villus junction. Crypt depth was defined as the depth of the invagination between adjacent villi (24). All morphological measurements (villus height and crypt depth) were measured on the stained sections under a microscope at ×40 combined magnification (Nikon Eclipse Ci-E, Japan). At least 15 intact, well-oriented crypt–villus units were measured in triplicate per broiler for each intestinal section. Reported values are means from 15 crypt–villus units.
Total RNA was isolated from frozen jejunal mucosal samples (50 mg) according to the instructions of the RNAiso Reagent (TaKaRa Bio Inc., Beijing, China). Purity and concentration of total mRNA in samples were evaluated using a spectrophotometer (NanoDrop-2000, Thermo Fisher Scientific, Waltham, MA) at 260 and 280 nm, respectively. Ratios of absorption (260:280 nm) between 1.8 and 2.0 for all samples were accepted as “pure” for RNA. RNA (1 μg) was used to generate cDNA using the M5 Super plus qPCR RT kit with a gDNA remover according to the manufacturer's instructions (MF166-plus-01, Mei5bio, Beijing). Primer sequences for tight junction proteins including ZO-1, Occludin, Claudin 3, Jam-2, mucoprotein Mucin 2, cytokine IFN-γ, TNF-α, IL-8, pattern recognition receptors TLR2 and TLR4, and key linker molecular MyD88 in jejunal mucosa were designed using the GenBank database from the National Center for Biotechnology Information (NCBI) and primer design software. Quantitative real-time PCR was performed with HiPer SYBR Premix EsTaq with Tli RNase H (MF787-01, Mei5bio, Beijing) using a StepOnePlus real-time PCR system (Applied Biosystems) on 96-well plates with 10 μl of total reaction volume of 5 μl HiPer SYBR Premix, 4 μl cDNA, 0.3 μl of forward and 0.3 μl of reverse primers (10 nmol), and 0.4 μl double-distilled water. Each reaction was run in duplicate. The PCR cycling protocol included one cycle of pre-incubation at 95°C for 30 s; 40 cycles of denaturation at 95°C for 5 s and annealing at 60°C for 30 s; one cycle of melting at 95°C for 5 s, 60°C for 60 s, and 95°C for 5 s; and one cycle of cooling at 50°C for 30 s. GAPDH was used as an internal control in this study. Average expression of the target genes relative to GAPDH was determined using the 2−ΔΔCt method as described by Livak and Schmittgen (25). Primers for qRT-PCR were synthesized by Sangon Biotech (Shanghai, China; Table 2).
Ileal mucosa was homogenized with 0.1 M PBS, and the supernatant was centrifuged at 3,000 rpm for 15 min. BCA protein quantitative kit (HuaXingBio, Beijing, China) was used to determine the total protein content of the ileal mucosa homogenate according to the manufacturer's instructions. Concentration of sIgA was determined by an enzyme-linked immunosorbent assay (Nanjing Jiancheng Bioengineering Institute, Nanjing, Jiangsu, China) according to the manufacturer's instructions. Data were acquired using a Microplate reader (SpectraMax M3, Molecular Devices, San Jose, CA, USA) equipped with SoftMax Pro Software. Relative abundance of sIgA in ileal mucosa was expressed as the ratio of sIgA to the total protein concentration of ileal mucosa homogenate.
Microbial DNA was isolated from ileal and cecal contents by the stool DNA kit (TIANGEN Biotech, Beijing, China) according to the manufacturer's instructions and was stored at −20°C. Numbers of total bacteria, Lactobacillus, and Escherichia coli were quantified using real-time PCR in a StepOnePlus system. Each reaction was run in a 10 μl volume containing 5 μl HiPer SYBR Premix EsTaq (MF787-01, Mei5bio, Beijing), 0.3 μl of forward and 0.3 μl of reverse primers (100 nM), 1 μl template DNA, and 3.4 μl of double-distilled water for detecting total bacteria. The PCR profile was processed as follows: one cycle of pre-incubation at 94°C for 180 s; 40 cycles of denaturation at 94°C for 30 s, annealing at 62°C for 30 s, and extension at 72°C for 60 s; one cycle of melting at 95°C for 5 s and 60°C for 60 s; and one cycle of cooling at 50°C for 30 s. To detect Lactobacillus and E. coli, each reaction was completed in a 10 μl volume containing 5 μl FastFire qPCR PreMix (Probe) (TIANGEN Biotech, Beijing, China), 0.25 μl of forward and 0.25 μl of reverse primers (100 nM), 0.15 μl probes, 1.5 μl template DNA, and 2.85 μl of double-distilled water. The PCR profile was determined as follows: one cycle of pre-incubation at 95°C for 600 s and 40 cycles of denaturation at 95°C for 15 s and annealing at 60°C for 60 s. All samples were analyzed in duplicate. Briefly, primers and fluorescent oligonucleotide probes (Table 3) were obtained from previous studies (26, 27) and commercially synthesized by Beijing Chenhuida Biotechnology Co.
Table 3. Sequences of the primer and probe for detection specific for intestinal microflora of broiler.
For the quantification of bacteria in the test samples, specific standard curves were drawn by constructing standard plasmids as described by Han et al. (28). Briefly, the standard strains of Lactobacillus (ATCC33323) and E. coli (V99 CGMCC1, 12881) were cultured anaerobically or aerobically in respective cultures including 1% glucose at 37°C from 12 to 48 h. The 16S rDNA genes of Lactobacillus and E. coli were amplified using PCR. Then, the specific PCR product was purified using a Gel Extraction Kit (Beijing ComWin Biotech Co., Ltd., Beijing, China) and cloned into pEASY-Blunt vector (TransGen Biotech, Beijing, China). After verification of the sequence, the recombinant plasmid was isolated using the TIANprep Mini Plasmid Kit (TIANGEN Biotech, Beijing, China). The standard plasmids of Lactobacillus and E. coli were constructed successfully. The copy numbers of bacteria were calculated using the following formula: (6.0233 * 1023 copies/mol * DNA concentration (μg/μl))/(660 * 106 * DNA size (bp)). Bacterial counts were performed using serial dilutions (10:1 to 10:8 dilutions) of plasmid DNA to generate the standard curve for total bacteria, Lactobacillus and E. coli. Each standard curve was constructed by linear regression of mean cycle threshold values against the logarithm of template copy numbers, ranging from 3 to 9 log10 copies. Target copy number of each sample was calculated from the standard curve (29, 30).
Samples of ileal and cecal contents were thawed and thoroughly mixed immediately before testing. Concentrations of acetic, propionic, butyric and valeric, and lactic acids and total SCFAs were determined with a Dionex ICS-3000 Ion Chromatography System as described by Tong et al. (29).
Data were analyzed using the ANOVA method in SPSS 20.0, and all data were checked for normal distribution and homogeneous variance. Each pen was regarded as an experimental unit for measurement of growth performance. Each euthanized broiler was regarded as an experimental unit for measurement of other indicators. Different experimental units were independent from each other. Treatment means were separated using Duncan's multiple comparison test. Data in tables are reported as means and pooled standard errors. Data in figures are reported as means ± SEM. Linear and quadratic comparisons were applied to determine the dose effect of Microcin C7 in broilers using orthogonal contrasts in SPSS 20.0. Significant differences were declared when P < 0.05, and statistical trends declared when 0.05 < P < 0.10.
Broilers fed 6 mg/kg Microcin C7 tended to consume more feed in the starter period (P = 0.07; Table 4) and grow faster over the entire supplemental period (P = 0.09; Table 4) than the antibiotic groups. The F/G ratio of broilers fed 4 mg/kg Microcin C7 over the entire supplemental period was decreased compared to that of the control group (P = 0.03; Table 4). The F/G ratio of broilers fed antibiotics over the entire supplemental period was also significantly decreased compared with that of the control group (P = 0.03; Table 4). A linearly higher ADG was observed in broilers fed Microcin C7 during the finisher supplemental period (P = 0.05; Table 4). A quadratically lower F/G ratio was observed in broilers fed Microcin C7 during the entire supplemental period (P = 0.02; Table 4). A quadratically lower ADFI was observed in broilers fed Microcin C7 during the finisher supplemental period (P = 0.04; Table 4). A linearly increased trend of ADFI (P = 0.08, Table 4) was observed in broilers fed Microcin C7 during the finisher supplemental period.
On Day 42, broilers fed with antibiotic, 4 and 6 mg/kg Microcin C7, had increased concentration of the anti-inflammatory cytokine IL-10 (Figure 1) and IgG and IgM (Figure 2) compared with the control group (P < 0.05). Broilers fed with antibiotic and Microcin C7 had decreased concentration of pro-inflammatory cytokine TNF-α (Figure 1) compared with the control group (P < 0.05). However, all groups were similar with each other on Day 21 (P > 0.05). On Day 42, a linearly higher content of IL-10 (Figure 1) and IgG and IgM (Figure 2) was observed in broilers fed Microcin C7 (P < 0.05). A linearly lower content of TNF-α was observed in broilers fed Microcin C7 (P < 0.05, Figure 1).
Figure 1. Effect of Microcin C7 on concentration of serum cytokines of broilers. (A) Concentration of pro-inflammatory cytokine IL-1β. (B) Concentration of pro-inflammatory cytokine IFN-γ. (C) Concentration of pro-inflammatory cytokine TNF-α. (D) Anti-inflammatory cytokine IL-10 on days 21 and 42. Within the same day, treatments that are significantly different from each other are indicated by different letters above the bar (P < 0.05). Bars represent means ± SEM for six broilers per treatment. Antibiotic control = broilers fed a basal diet with 45 mg/kg aureomycin plus 30 mg/kg bacitracin methylene disalicylate. Control = broilers fed a basal diet. Microcin C7 = broilers fed a basal diet containing 2, 4, or 6 mg/kg Microcin C7.
Figure 2. Effect of Microcin C7 on concentration of serum immunoglobulin of broilers. (A) Concentration of IgA. (B) Concentration of IgG. (C) Concentration of IgM. Within the same day, treatments that are significantly different from each other are indicated by different letters above the bar (P < 0.05). Bars represent means ± SEM for six broilers per treatment. Antibiotic control = broilers fed a basal diet with 45 mg/kg aureomycin plus 30 mg/kg bacitracin methylene disalicylate. Control = broilers fed a basal diet. Microcin C7 = broilers fed a basal diet containing 2, 4, or 6 mg/kg Microcin C7.
On Day 21, dietary addition of 2, 4, and 6 mg/kg Microcin C7 increased the V/C ratio of ileum and significantly increased the V/C ratio of duodenum and the villus height of jejunum compared with the control group (P < 0.05; Table 5). Dietary addition of 2 and 6 mg/kg Microcin C7 increased the villus height of the duodenum compared with the control group (P < 0.05; Table 5). Dietary addition of 4 and 6 mg/kg Microcin C7 increased the V/C ratio of jejunum compared with the control group (P < 0.05; Table 5). Dietary addition of 2 and 4 mg/kg Microcin C7 decreased the crypt depth of the duodenum compared with the control group (P < 0.05; Table 5). Dietary addition of 4 and 6 mg/kg Microcin C7 decreased crypt depth of ileum compared with the control group (P < 0.05; Table 5). However, on Day 21, dietary addition of antibiotic decreased villus height and villus height/crypt depth ratio in the duodenum and increased crypt depth and decreased the villus height/crypt depth ratio in the ileum (P < 0.05; Table 5). A linearly higher villus height and V/C ratio was observed in the small intestine of broilers fed Microcin C7 (P < 0.05; Table 5), and a linearly lower crypt depth was observed in the ileum of broilers fed Microcin C7 (P < 0.05; Table 5).
On Day 42, dietary addition of 2, 4, and 6 mg/kg Microcin C7 increased the villus height of the duodenum and ileum and the V/C ratio of the jejunum and ileum compared with the control group (P < 0.05; Table 5). Dietary addition of 4 and 6 mg/kg Microcin C7 increased the V/C ratio of duodenum compared with the control group (P < 0.05; Table 5). Dietary addition of 6 mg/kg Microcin C7 increased the villus height of the jejunum compared with the control group (P < 0.05; Table 5). Dietary addition of 4 and 6 mg/kg Microcin C7 decreased the crypt depth of the jejunum compared with the control group (P < 0.05; Table 5). Dietary addition of 2, 4, and 6 mg/kg Microcin C7 decreased the crypt depth of the ileum compared with the control group (P < 0.05; Table 5). On Day 42, dietary addition of antibiotic increased the villus height of the duodenum and villus height and V/C ratio of the ileum and decreased the crypt depth of the ileum compared with the control group (P < 0.05; Table 5). However, dietary addition of antibiotic decreased the villus height of the jejunum compared with the control group (P < 0.05; Table 5). A linearly higher villus height and a higher V/C ratio were observed in the small intestine of broilers fed Microcin C7 (P < 0.05; Table 5), and a linearly lower crypt depth was observed in the duodenum (P = 0.03; Table 5), jejunum (P < 0.05; Table 5), and ileum (P < 0.05; Table 5) of broilers fed Microcin C7.
There was no significant difference in intestinal morphology between all groups (Supplementary Figure S1).
On Day 42, diets supplemented with 4 mg/kg Microcin C7 significantly upregulated expression of Occludin compared with the control group (P < 0.05; Figure 3). Diets supplemented with 2 and 4 mg/kg Microcin C7 upregulated the expression of ZO-1 compared with the control group (P < 0.05; Figure 3). However, diets supplemented with Microcin C7 had no significant effect on the expression of Claudin 3, Jam-2, or Mucin 2 compared with control group. A quadratically higher gene expression of Occludin and ZO-1 was observed in broilers fed Microcin C7 (P < 0.05; Figure 3).
Figure 3. Effect of Microcin C7 on gene expression in the jejunal mucosa of broilers. (A) Relative mRNA expression of Claudin-3. (B) Relative mRNA expression of ZO-1. (C) Relative mRNA expression of Occludin. (D) Relative mRNA expression of Jam-2. (E) Relative mRNA expression of Mucin 2. (F) Relative mRNA expression of IL-8. (G) Relative mRNA expression of IFN-γ. (H) Relative mRNA expression of TNF-α. (I) Relative mRNA expression of TLR2. (J) Relative mRNA expression of TLR4. (K) Relative mRNA expression of MyD88. Within the same day, treatments that are significantly different from each other are indicated by different letters above the bar (P < 0.05). Bars represent means ± SEM for six broilers per treatment. Antibiotic control = broilers fed a basal diet with 45 mg/kg aureomycin plus 30 mg/kg bacitracin methylene disalicylate. Control = broilers fed a basal diet. Microcin C7 = broilers fed a basal diet containing 2, 4, or 6 mg/kg Microcin C7.
On Day 42, diets supplemented with 6 mg/kg Microcin C7 or antibiotics significantly downregulated the expression of TNF-α and IL-8 compared with the control group (P < 0.05; Figure 3). Diets supplemented with Microcin C7 or antibiotics significantly downregulated expression of IFN-γ compared with the control group (P < 0.05; Figure 3). A linearly lower gene expression of TNF-α (P = 0.05), IL-8 (P < 0.05), and IFN-γ (P < 0.05) was observed in broilers fed Microcin C7 (Figure 3).
On Day 42, diets supplemented with 6 mg/kg Microcin C7 or antibiotics significantly downregulated expression of TLR4 and TLR2 compared with the control group (P < 0.05; Figure 3). Diets supplemented with Microcin C7 or antibiotics significantly downregulated MyD88 compared with the control group (P < 0.05; Figure 3). A linearly lower gene expression of TLR4 (P < 0.05), TLR2 (P = 0.03), and MyD88 (P < 0.05) was observed in broilers fed Microcin C7 (Figure 3).
However, neither Microcin C7 nor antibiotics had any significant effects on expression of tight junction proteins, adhesion junction proteins, pro-inflammatory cytokines, chemokines, pattern recognition receptor, and MyD88 on Day 21 compared with the control group (P > 0.05; Figure 3). However, a linearly lower gene expression of TLR2 was observed in broilers fed Microcin C7 on Day 21 (P < 0.05; Figure 3).
On Day 21, broilers fed with 6 mg/kg Microcin C7 had significantly increased sIgA concentration compared with the control group (P < 0.05; Figure 4). A linearly higher content of sIgA was observed in broilers fed Microcin C7 (P < 0.01; Figure 4). On Day 42, broilers fed with Microcin C7 had significantly increased sIgA compared with the control group (P < 0.05). A linearly higher content of sIgA was observed in broilers fed Microcin C7 (P = 0.02; Figure 4). However, feeding antibiotics to broilers had no significant effect on sIgA concentration (P > 0.05).
Figure 4. Effect of Microcin C7 on relative abundance of sIgA in the ileal mucosa of broilers. Relative abundance of sIgA in the ileal mucosa was expressed as the ratio of sIgA to the total protein concentration of ileal mucosa homogenate. Within the same day, treatments that are significantly different from each other are indicated by different letters above the bar (P < 0.05). Bars represent means ± SEM for six broilers per treatment. Antibiotic control = broilers fed a basal diet with 45 mg/kg aureomycin plus 30 mg/kg bacitracin methylene disalicylate. Control = broilers fed a basal diet. Microcin C7 = broilers fed a basal diet containing 2, 4, or 6 mg/kg Microcin C7.
On Day 21, diet supplemented with 6 mg/kg Microcin C7 decreased the population of E. coli compared with the control group (P < 0.05; Figure 5). A linearly lower content of the number of E. coli (log10CFU) was observed in broilers fed Microcin C7 (P < 0.01; Figure 5). However, there was no significant difference in the number of total bacteria and Lactobacillus compared with controls. On Day 42, diets supplemented with 6 mg/kg Microcin C7 decreased the population of total bacteria (P < 0.05; Figure 5) and increased the population of Lactobacillus compared with the control group (P < 0.05; Figure 5). Diets supplemented with Microcin C7, regardless of concentration, decreased the population of E. coli (P < 0.05; Figure 5). A linearly lower content of the number of total bacteria (log10CFU; P < 0.01) and E. coli (log10CFU; P = 0.02) was observed in broilers fed Microcin C7 (P < 0.01; Figure 5). A linearly higher content of the number of Lactobacillus (log10CFU) was observed in broilers fed Microcin C7 (P < 0.01; Figure 5).
Figure 5. Effect of Microcin C7 on cecal total bacteria, E. coli and Lactobacillus populations in ceca of broilers. (A) Number [log10(CFU/g)] of total bacteria. (B) Number [log10(CFU/g)] of E. coli. (C) Number [log10(CFU/g)] of Lactobacillus. Within the same day, treatments that are significantly different from each other are indicated by different letters above the bar (P < 0.05). Bars represent means ± SEM for 6 broilers per treatment. Bacterial number is expressed as log10 CFU per gram of wet cecal digesta. Antibiotic control = broilers fed a basal diet with 45 mg/kg aureomycin plus 30 mg/kg bacitracin methylene disalicylate. Control = broilers fed a basal diet. Microcin C7 = broilers fed a basal diet containing 2, 4, or 6 mg/kg Microcin C7.
On Day 21, broilers fed with 6 mg/kg Microcin C7 had increased acetic acid, butyric acid, and valeric acids in ileal contents compared with broilers fed the control diet (P < 0.05, Table 6). A linearly higher content of acetic acid, butyric acid, and valeric acid was observed in ileal contents of broilers fed Microcin C7 (P < 0.05; Table 6). On Day 42, broilers fed 6 mg/kg Microcin C7 had increased lactic acid, acetic acid, and total SCFAs in ileal digesta compared to control broilers (P < 0.05, Table 6). A linearly higher content of lactic acid, acetic acid, propionic acid, butyric acid, and total SCFAs was observed in ileal contents of broilers fed Microcin C7 (P < 0.05; Table 6).
Table 6. Effect of Microcin C7 on lactic acid and SCFA in ileal digesta (mg/kg of wet ileal digesta)1,2.
In cecal digesta, the lactic acid, acetic acid, propionic acid, and total SCFAs were higher in broilers fed 6 mg/kg Microcin C7 on Day 21 (P < 0.05; Table 7). A linearly higher content of lactic acid, acetic acid (P < 0.05; Table 7), propionic acid (P = 0.05; Table 7), and total SCFAs (P < 0.05; Table 7) were observed in cecal contents of broilers fed Microcin C7. On Day 42, birds fed the highest concentration of Microcin C7 displayed increased lactic acid and propionic acid concentrations compared with control-fed broilers (P < 0.05; Table 7). A linearly higher content of lactic acid and propionic acid was observed in cecal contents of broilers fed Microcin C7 (P < 0.05; Table 7).
Table 7. Effect of Microcin C7 on lactic acid and SCFA in cecal digesta (mg/kg of wet ileal digesta)1,2.
Microcin C7 is a ‘Trojan horse’ antimicrobial peptide which can be imported effectively into bacterial cells and inhibits protein synthesis through a mechanism of action that limits development bacterial resistance to drugs (31). In this study, we have demonstrated that broilers fed Microcin C7 tended to express increased ADFI and ADG and a decreased F/G ratio. The positive effect of antimicrobial peptides on growth performance of broilers has been reported previously. Choi et al. reported that diets supplemented with 90 mg/kg of the antimicrobial peptide AMP-A3 improved the overall BW gain of Ross 308 chicks (32). Xie et al. reported that diets supplemented with 100 g/t Partt ABP (mainly composed of plectasin) and 100 g/t full-tide ABP (mainly composed of cecropin) reduced the F/G ratio of commercial 818 broiler chickens during the overall feeding period (33). Hu et al. reported that addition of swine gut intestinal antimicrobial peptides (SGAMPs) improved ADG and reduced the F/G ratio and histological lesions of Arbor Acre broilers under chronic heat stress (34). In the present study, a high dose of Microcin C7 (6 mg/kg) elicited improved growth performance, and an intermediate dose of Microcin C7 (4 mg/kg) supported the lowest F/G ratio, which indicated the beneficial effects of Microcin C7 on the performance of broilers. Broilers fed with antibiotics did not show improved performance except for the F/G ratio during the entire period compared with birds fed control diets, and the effect is not as good as that of Microcin C7.
Apart from their direct antimicrobial activities, antimicrobial peptides also play an important role in innate immunity and serve as regulators of pro- and anti-inflammatory responses (13, 14). Blood biochemistry is an important indicator of animal health. When the body is exposed to pathogens, immune cells produce pro-inflammatory cytokines and chemokines to help eliminate invaders. However, the rapid and excessive expression of pro-inflammatory cytokines such as IL-1β, TNF-α, and IFN-γ, often called a “cytokine storm”, causes cell and organ damage (35, 36). In contrast, anti-inflammatory cytokines such as IL-4 and IL-10 resist the development of inflammatory responses. The balance of pro- and anti-inflammatory cytokines is important for the resolution of inflammatory diseases (37). Wang et al. reported that the antimicrobial peptide Microcin J25 can significantly downregulate pro-inflammatory cytokines, TNF-α, IL-1β, and IL-6, in serum of broilers challenged with E. coli or Salmonella (38). Yu et al. also reported that diets supplemented with Microcin J25 significantly reduced cytokines IL-1β, IL-6, and TNF-α and increased IL-10 in serum of weanling piglets (36). In the present study, Microcin C7 and the antibiotic diet significantly decreased TNF-α and increased IL-10 in serum on Day 42, which agrees with a previous study (38, 39). This result showed that Microcin C7 has the potential to suppress inflammatory responses and help maintain a desirable balance of immune responses.
Immunoglobulins that have antibiotic activity are secreted by plasma cells after immune stimulation and can directly participate in humoral immunity. Immunoglobulins can neutralize toxins and pathogen infections through specific binding with corresponding antigens, as part of the antigen-clearing activities of B cells (40). Hurtado et al. reported that the human antimicrobial peptide LL-37 increased the sensitivity of human peripheral B cells, enhancing B-cell activation and increasing IgM and IgG production (41). Shan et al. also reported that artificially synthesized antimicrobial peptide lactoferrin effectively increased serum IgA, IgG, and IgM in weanling piglets (42). Similarly, the present study demonstrated that Microcin C7 and dietary antibiotic increased serum IgG and IgM concentrations. This result showed that Microcin C7 has the potential to improve the immune responses elicited by invasion of pathogens.
The small intestine is the primary location for absorption and transport of nutrients (33). Morphological changes reflect the health status of the gut. Increased villus height is related to increased villus absorption surface area, which can enhance nutrient absorption and potentially improve growth performance. Increased villus height also suggests increased epithelial turnover and activation of cell mitosis (34). However, increased crypt depth may reduce secretion of digestive enzymes and nutrient absorption and eventually lower broiler's growth performance (43). Therefore, greater villus height, decreased crypt depth, and greater V/C ratio suggest enhanced ability of the gut to absorb nutrients. Some studies have shown beneficial effects of antimicrobial peptides on intestinal morphology. Wen et al. reported that the antimicrobial peptide CADN had a positive effect on villus height and villus height/crypt depth ratio, but a negative effect on crypt depth of the duodenum and ileum of broilers at 42 days of age (44). Choi et al. reported that broilers fed increasing levels of the antimicrobial peptide-A3 in diets had linearly increased villus height of the duodenum, jejunum, and ileum (32). Fan et al. reported that a mutated rabbit defensin NP-1 improved duodenal morphology by increasing the length of long and thin villi (45). In line with previous studies, the present study shows that Microcin C7 significantly increased villus height, decreased crypt depth, and increased the V/C ratio in the small intestine. The result suggests that Microcin C7 has the potential to maintain a favorable structure of the intestine, promote absorptive capacity, and help maintain gut health.
Intestinal epithelial cells constitute barrier surfaces that separate the host from the external environment. Tight junctions connect adjacent intestinal epithelial cells and regulate intestinal permeability. Tight junctions are composed of junction adhesion molecules, the transmembrane protein Occludin, members of the claudin family, and linker proteins such as the zonula occludens protein family (46, 47). Tight junctions act as a barrier to harmful molecules and provide a pore for the permeation of ions, solutes, and water as appropriate (48). Disruption of the tight junction barrier increases paracellular permeability to luminal antigens, inflammatory factors, and bacterial translocation, which leads to sustained inflammation, tissue damage, and reduced nutrient retention (48, 49). In the present study, we demonstrated that dietary Microcin C7, especially at 4 mg/kg, increased mRNA expression of tight junction proteins, Occludin and ZO-1, but had no significant effect on Claudin-3 and Jam-2. The ability of 6 mg/kg Microcin C7 to promote gene expression of tight junction protein ZO-1 and Occludin was not as good as 2 and 4 mg/kg Microcin C7. I guess it may due to the high anti-inflammatory properties of 6 mg/kg Microcin C7, which may have an irritant effect on the gastrointestinal tract in the meantime. However, compared with the control group, 6 mg/kg Microcin C7 had no side effect on the mRNA expression of tight junction protein. Identical conclusions were reported by Xie et al. who found that a diet supplemented with 100 g/t Partt ABP (mainly composed of plectasin) and 100 g/t full-tide ABP (mainly composed of cecropin) increased expression of ZO-1 and Claudin-3 (33). Feng et al. also reported that antimicrobial peptide Cathelicidin-BF increased the expression level of ZO-1, Occludin, and Claudin-1 in the jejunum and colon of weaned piglets compared with the control group (50). Mucus, which covers the intestinal epithelial surface, is one of the key components of gut barrier integrity (51). Mucin 2 is the main component of the intestinal mucus and acts as a surface cleaner and the first line of immune defense against pathogens (52, 53). Xie et al. reported that a diet supplemented with 100 g/t Partt ABP (mainly composed of plectasin) and 100 g/t full-tide ABP (mainly composed of cecropin) increased expression of Mucin 2 (33). However, our results reported herein show that Microcin C7 had no significant effect on Mucin 2. The difference from Xie et al. may be the result of different types of antimicrobial peptides, broilers, or rearing environments used in two studies.
The gut harbors approximately 80% of the immune cells of the whole body and considered to be the largest immunological organ in the body. Consequently, the gut plays a central role in immune regulation and defense against pathogens and influences the overall health of the body (54). Intestinal epithelial cells sense bacterial invasions and activate the immune system by means of pattern recognition receptors (PRRs), such as Toll-like receptors (TLRs) (55). When pathogens invade, TLR signaling pathways are activated, which promote inflammation and cause inflammatory bowel diseases (IBD). TLR2 senses the presence of bacterial lipoproteins, lipoteichoic acids, peptidoglycan, and zymosan. TLR4 is the predominant receptor for LPS from gram-negative organisms. TLR2 and TLR4 expressions are highly upregulated in IBD patients (56). The activation of TLR signaling transduction pathway is initiated by the LPS-LBP-CD14 complex, which signals through the induction of a key linker molecular MyD88, serine kinase IL-1R-associated kinase 4 (IRAK4), and adaptor protein TNF receptor-associated factor 6 (TRAF6). These molecules lead to activation of the transcription factor NF-κB and activating protein 1 followed by cascades of mitogen-activated protein kinases (57, 58). This triggers the induction of numerous genes including pro-inflammatory cytokines such as IL-1β, IL-6, TNF-α, IFN-γ, chemokines such as IL-8, and antigen-presenting molecules. Some researchers showed the immune-regulating ability of antimicrobial peptides. Xie et al. reported that a diet supplemented with 100 g/t Partt ABP (mainly composed of plectasin) and 100 g/t full-tide ABP (mainly composed of cecropin) significantly decreased the expression of pro-inflammatory cytokines IL-17A and IFN-α, which suppress intestinal inflammation (33). Feng reported that the antimicrobial peptide Cathelicidin-BF suppressed pro-inflammatory cytokines IL-6 and IL-22 and the chemokine IL-8 in the jejunum and ileum (50). Yi et al. reported that the antimicrobial peptide Cathelicidin-WA decreased gene and protein expressions of TLR4 and MyD88 in the jejunum (59). In line with previous studies, we demonstrated herein that Microcin C7 suppressed mRNA expression of TLR-2, TLR4, MyD88; pro-inflammatory cytokines TNF-α and IFN-γ; and the chemokine IL-8. This result showed that Microcin C7 has the potential to attenuate intestinal inflammation and aid in the regulation of the immune system.
Secretory IgA is the predominant mucosal immunoglobulin in mammals and birds. sIgA provides immunological defense by preventing pathogens from adhering to and penetrating the mucosal epithelium and helping maintain symbiotic relationships with commensal bacteria (60). Classically, sIgA eliminates pathogens by inactivating bacterial enzymes and toxins and blocking bacterial attachment (60, 61). Apart from that, sIgA also plays an important role in induction of tolerance to innocuous food antigens and commensal bacteria under normal conditions (62). Bao et al. reported that administration of a pig antimicrobial peptide enhanced sIgA expression in both the duodenum and jejunum of broilers compared with the control group (61). In another study, researchers showed that antimicrobial peptides derived from rabbit sacculus rotundus increased the area of IgA-secreting cells in various intestinal segments (63). The present study showed that administration of Microcin C7 could enhance sIgA expression in the ileum of broilers. However, the dietary antibiotic had no effect on the expression of sIgA. Antibiotic has a similar effect to Microcin C7. This result showed that in addition to bactericidal ability, Microcin C7 can also strengthen the host's immunity in the intestinal mucosa.
Antimicrobial peptides have broad-spectrum activity against gram-negative and gram-positive bacteria (64, 65), fungi (66), eukaryotic parasites (67), and viruses (68) in vitro (69). Previous researchers demonstrated that antimicrobial peptides maintain the equilibrium of intestinal microecology by suppressing harmful pathogens and promoting proliferation of beneficial microorganisms in vivo, which improved intestinal health. Wen and He reported that the antimicrobial peptide CADN decreased aerobic bacterial counts in both jejunal and cecal digesta of broilers in a dose-dependent manner (44). Choi et al. reported that broilers fed antimicrobial peptide-A3 had fewer excreta coliforms, total anaerobic bacteria, and Clostridium spp. than broilers fed control diets (32). Zhang et al. reported that dietary antimicrobial peptide plectasin significantly decreased the quantity of E. coli and increased the ratio between Lactobacilli and E. coli in the ileal contents of 21-day-old yellow-feathered chickens (16). Tang et al. also reported that the antimicrobial peptide lactoferrin significantly decreased the number of E. coli and increased the number of Lactobacilli and Bifidobacterium in the ileum, caecum, and colon of weaned piglets (70). In agreement with previous studies, our study reported herein showed that Microcin C7 decreased total bacteria and E. coli and increased Lactobacillus in the cecum of broilers, which indicated that Microcin C7 has the potential to selectively regulate gut microbiota. Antibiotics also can potentially regulate intestinal microbiota, but effects were not as good as Microcin C7.
SCFAs and other organic acids such as lactate and succinate are primary microbial fermented products degraded from dietary carbohydrates through an extensive set of enzymes (71). SCFAs are important fuels for intestinal epithelial cells and regulate their proliferation and differentiation, which affect gut motility. SCFAs reduce the pH of gut lumen, inhibit the growth of harmful bacteria, and strengthen gut barrier function and host metabolism (72). Yu et al. reported that dietary Microcin J25 increased lactate and SCFAs in feces of weanling piglets (39). Yi et al. also reported that the antimicrobial peptide CWA increased SCFA levels in feces of weanling piglets (59). In the current study, we found that Microcin C7 increased lactate and SCFA concentrations in the ileum and cecum of broilers, which indicates that Microcin C7 has the potential to adjust the balance of the intestinal microecology and maintain gut health. Lactobacillus is the most abundant genus in the duodenum (36–97%), jejunum (39–72%), and ileum (24–96%) at all ages. In the ileum, the abundances of Lactobacillus are stable from 7 to 21 days of age and increased to 96.7% on Day 42 (73). Therefore, in the current study, the content of lactic acid is most abundant in the ileum followed by acetic acid. A sharp increase of lactic acid from Day 21 to Day 42 can be seen in our study. In the cecum, acetic acid, propionic acid, and butyric acid are the most abundant SCFAs, but the content of lactic acid is little. Cecal acetate, propionate, butyrate, and valerate acids increase with age (73). This trend can be also seen in our study. The relatively large intra-group variation of SCFAs may be an important reason to explain the different concentrations of SCFAs between birds at age of 21 and 42 days.
In conclusion, results obtained in this study indicated that dietary supplementation with Microcin C7 can strengthen immune functions, improve intestinal villus structures, enhance intestinal barrier function, and regulate composition of intestinal microbiota, which suggests its potential to improve growth performance. Microcin C7 fed at 4 mg/kg has the best effect on the F/G ratio and expression of tight junction proteins. Microcin C7 fed at 6 mg/kg has the best effect on growth performance, immune and anti-inflammatory functions, and maintenance of intestinal homeostasis. Microcin C7 appears to have the greatest effects in the finisher stage of broiler production. This may include two reasons. First, the effect of Microcin C7 is cumulative. Second, broilers intake more Microcin C7 during the finisher stage. These results indicated that Microcin C7 can be used as a promising alternative to traditional antibiotics.
As for overall mode of action for Microcin C7 peptide, we suppose that gram-negative pathogens secrete enterotoxin, release lipopolysaccharide, impair the intestinal barrier, and further result in inflammatory bowel disease (48, 49, 56). Microcin C7 exhibits antimicrobial activity against those pathogens, resists pathogen invasion, and regulates gut microbiota. As an immunomodulator, Microcin C7 can also regulate intestinal immune functions and maintain intestinal microecological balance. Since the intestine is the largest immune and absorption organ, the enhancement of intestinal health by Microcin C7 improves the serum index and further improves growth performance. The specific mechanism responsible for these positive observations will be verified by bacterial infection or DSS-induced colitis model in our future studies.
The raw data supporting the conclusions of this article will be made available by the authors, without undue reservation.
The animal study was reviewed and approved by China Agricultural University Animal Care and Use Committee.
ZD: conceptualization, methodology, investigation, formal analysis, data curation, and writing-original draft. LS: investigation, methodology, and writing–review & editing. FW: resources, investigation, and writing–review & editing. XZ: conceptualization, methodology, and writing-review & editing. HY: methodology and writing-review & editing. LL: formal analysis and writing-review & editing. JZ: conceptualization. SQ: conceptualization, resources, supervision, investigation, and writing–review & editing. All authors have read and agreed to the published version of the manuscript.
This study was supported by the National Key Research and Development Program of China (grant number 32030105), Chongqing Rongchang Agricultural and Animal Husbandry High-tech Industry Research and Development Project (cstc2019ngzx0019), and Beijing Swine Innovation Team of Modern Agriculture Industry Technological System.
The authors declare that the research was conducted in the absence of any commercial or financial relationships that could be construed as a potential conflict of interest.
All claims expressed in this article are solely those of the authors and do not necessarily represent those of their affiliated organizations, or those of the publisher, the editors and the reviewers. Any product that may be evaluated in this article, or claim that may be made by its manufacturer, is not guaranteed or endorsed by the publisher.
We would like to thank Professor Lee J. Johnston for improving and modifying the language of this article.
The Supplementary Material for this article can be found online at: https://www.frontiersin.org/articles/10.3389/fvets.2021.813629/full#supplementary-material
AMPs, antimicrobial peptides; ADG, average daily gain; ADFI, average daily feed intake; F/G, feed/gain; V/C, villus height/crypt depth; PBS, phosphate-buffered solution; IL-1β, interleukin 1β; IL-8, interleukin 8; IL-10, interleukin 10; TNF-α, tumor necrosis factor α; IFN-γ, interferon-γ; TLR2, toll-like receptor 2; TLR4, toll-like receptor 4; IgA, immunoglobulin A; IgG, immunoglobulin G; IgM, immunoglobulin M; sIgA, secretary immunoglobulin A; ZO-1, Zonula Occludens; Jam-2, junctional adhesion molecule 2; MyD88, myeloid differentiation factor 88; SCFAs, short-chain fatty acids; ANOVA, analysis of variance; DSS, dextran sulfate sodium.
1. Mund MD, Khan UH, Tahir U, Mustafa BE, Fayyaz A. Antimicrobial drug residues in poultry products and implications on public health: a review. Int J Food Properties. (2016) 20:1433–46. doi: 10.1080/10942912.2016.1212874
2. Roth N, Kasbohrer A, Mayrhofer S, Zitz U, Hofacre C, Domig KJ. The application of antibiotics in broiler production and the resulting antibiotic resistance in escherichia coli: a global overview. Poult Sci. (2019) 98:1791–804. doi: 10.3382/ps/pey539
3. Boeckel TV, Glennon EE, Chen D, Gilbert M, Robinson TP, Grenfell BT, et al. Reducing antimicrobial use in food animals. Science. (2017) 357:1350–2. doi: 10.1126/science.aao1495
4. Harbarth S, Balkhy HH, Goossens H, Jarlier V, Kluytmans J, Laxminarayan R, et al. Antimicrobial resistance: One world, one fight! Antimicrob Resist Infect Control. (2015) 4:49. doi: 10.1186/s13756-015-0091-2
5. Mehdi Y, Letourneau-Montminy MP, Gaucher ML, Chorfi Y, Suresh G, Rouissi T, et al. Use of antibiotics in broiler production: global impacts and alternatives. Anim Nutr. (2018) 4:170–8. doi: 10.1016/j.aninu.2018.03.002
6. Daeseleire E, De Graef E, Rasschaert G, De Mulder T, Van den Meersche T, Van Coillie E, et al. Antibiotic use and resistance in animals: Belgian initiatives. Drug Test Anal. (2016) 8:549–55. doi: 10.1002/dta.2010
7. Walsh CT, Wencewicz TA. Prospects for new antibiotics: a molecule-centered perspective. J Antibiot (Tokyo). (2014) 67:7–22. doi: 10.1038/ja.2013.49
8. Pasupuleti M, Schmidtchen A, Malmsten M. Antimicrobial peptides: key components of the innate immune system. Crit Rev Biotechnol. (2012) 32:143–71. doi: 10.3109/07388551.2011.594423
9. Zasloff M. Antimicrobial peptides of multicellular organisms. Nature. (2002) 415:389–95. doi: 10.1038/415389a
10. Guilhelmelli F, Vilela N, Albuquerque P, Derengowski Lda S, Silva-Pereira I, Kyaw CM. Antibiotic development challenges: the various mechanisms of action of antimicrobial peptides and of bacterial resistance. Front Microbiol. (2013) 4:353. doi: 10.3389/fmicb.2013.00353
11. Sierra JM, Fuste E, Rabanal F, Vinuesa T, Vinas M. An overview of antimicrobial peptides and the latest advances in their development. Expert Opin Biol Ther. (2017) 17:663–76. doi: 10.1080/14712598.2017.1315402
12. Wang S, Zeng X, Yang Q, Qiao S. Antimicrobial peptides as potential alternatives to antibiotics in food animal industry. Int J Mol Sci. (2016) 17:603. doi: 10.3390/ijms17050603
13. Lai Y, Gallo RL. Amped up immunity: How antimicrobial peptides have multiple roles in immune defense. Trends Immunol. (2009) 30:131–41. doi: 10.1016/j.it.2008.12.003
14. Gallo RL, Hooper LV. Epithelial antimicrobial defence of the skin and intestine. Nat Rev Immunol. (2012) 12:503–16. doi: 10.1038/nri3228
15. Holly MK, Smith JG. Paneth cells during viral infection and pathogenesis. Viruses. (2018) 10:225. doi: 10.3390/v10050225
16. Zhang X, Zhao Q, Wen L, Wu C, Yao Z, Yan Z, et al. The effect of the antimicrobial peptide plectasin on the growth performance, intestinal health, and immune function of yellow-feathered chickens. Front Vet Sci. (2021) 8:688611. doi: 10.3389/fvets.2021.688611
17. Daneshmand A, Kermanshahi H, Sekhavati MH, Javadmanesh A, Ahmadian M. Antimicrobial peptide, clf36, affects performance and intestinal morphology, microflora, junctional proteins, and immune cells in broilers challenged with e. Coli. Sci Rep. (2019) 9:14176. doi: 10.1038/s41598-019-50511-7
18. Daneshmand A, Kermanshahi H, Sekhavati MH, Javadmanesh A, Ahmadian M, Alizadeh M, et al. Effects of clfchimera peptide on intestinal morphology, integrity, microbiota, and immune cells in broiler chickens challenged with necrotic enteritis. Sci Rep. (2020) 10:17704. doi: 10.1038/s41598-020-74754-x
19. Metlitskaya A, Kazakov T, Kommer A, Pavlova O, Praetorius-Ibba M, Ibba M, et al. Aspartyl-trna synthetase is the target of peptide nucleotide antibiotic microcin c. J Biol Chem. (2006) 281:18033–42. doi: 10.1074/jbc.M513174200
20. Zukher I, Novikova M, Tikhonov A, Nesterchuk MV, Osterman IA, Djordjevic M, et al. Ribosome-controlled transcription termination is essential for the production of antibiotic microcin c. Nucleic Acids Res. (2014) 42:11891–902. doi: 10.1093/nar/gku880
21. Agarwal V, Tikhonov A, Metlitskaya A, Severinov K, Nair SK. Structure and function of a serine carboxypeptidase adapted for degradation of the protein synthesis antibiotic microcin c7. Proc Natl Acad Sci U S A. (2012) 109:4425–30. doi: 10.1073/pnas.1114224109
22. Garcia-Bustos JF, Pezzi N, Mendez E. Structure and mode of action of microcin 7, an antibacterial peptide produced by Escherichia coli. Antimicrob Agents Chemother. (1985) 27:791–7. doi: 10.1128/AAC.27.5.791
23. Wang D, Ma W, She R, Sun Q, Liu Y, Hu Y, et al. Effects of swine gut antimicrobial peptides on the intestinal mucosal immunity in specific-pathogen-free chickens. Poult Sci. (2009) 88:967–74. doi: 10.3382/ps.2008-00533
24. Uni Z, Noy Y, Sklan D. Posthatch development of small intestinal function in the poult. Poult Sci. (1999) 78:215–22. doi: 10.1093/ps/78.2.215
25. Livak KJ, Schmittgen TD. Analysis of relative gene expression data using real-time quantitative pcr and the 2(-delta delta c(t)) method. Methods. (2001) 25:402–8. doi: 10.1006/meth.2001.1262
26. Fierer N, Jackson JA, Vilgalys R, Jackson RB. Assessment of soil microbial community structure by use of taxon-specific quantitative pcr assays. Appl Environ Microbiol. (2005) 71:4117–20. doi: 10.1128/AEM.71.7.4117-4120.2005
27. Chen H, Mao X, He J, Yu B, Huang Z, Yu J, et al. Dietary fibre affects intestinal mucosal barrier function and regulates intestinal bacteria in weaning piglets. Br J Nutr. (2013) 110:1837–48. doi: 10.1017/S0007114513001293
28. Han GQ, Xiang ZT, Yu B, Chen DW, Qi HW, Mao XB, et al. Effects of different starch sources on bacillus spp. In intestinal tract and expression of intestinal development related genes of weanling piglets. Mol Biol Rep. (2012) 39:1869–76. doi: 10.1007/s11033-011-0932-x
29. Tong LT, Zhong K, Liu L, Zhou X, Qiu J, Zhou S. Effects of dietary hull-less barley beta-glucan on the cholesterol metabolism of hypercholesterolemic hamsters. Food Chem. (2015) 169:344–9. doi: 10.1016/j.foodchem.2014.07.157
30. Qi H, Xiang Z, Han G, Yu B, Huang Z, Chen D. Effects of different dietary protein sources on cecal microflora in rats. Afr J Biotechnol. (2011) 10:3704–8. doi: 10.5897/AJB10.2677
31. Severinov K, Nair SK. Microcin C: biosynthesis and mechanisms of bacterial resistance. Future Microbiol. (2012) 7:281–9. doi: 10.2217/fmb.11.148
32. Choi SC, Ingale SL, Kim JS, Park YK, Kwon IK, Chae BJ. An antimicrobial peptide-a3: Effects on growth performance, nutrient retention, intestinal and faecal microflora and intestinal morphology of broilers. Br Poult Sci. (2013) 54:738–46. doi: 10.1080/00071668.2013.838746
33. Xie Z, Zhao Q, Wang H, Wen L, Li W, Zhang X, et al. Effects of antibacterial peptide combinations on growth performance, intestinal health, and immune function of broiler chickens. Poult Sci. (2020) 99:6481–92. doi: 10.1016/j.psj.2020.08.068
34. Hu F, Gao X, She R, Chen J, Mao J, Xiao P, et al. Effects of antimicrobial peptides on growth performance and small intestinal function in broilers under chronic heat stress. Poult Sci. (2017) 96:798–806. doi: 10.3382/ps/pew379
35. Chousterman BG, Swirski FK, Weber GF. Cytokine storm and sepsis disease pathogenesis. Semin Immunopathol. (2017) 39:517–28. doi: 10.1007/s00281-017-0639-8
36. Clark IA, Vissel B. The meteorology of cytokine storms, and the clinical usefulness of this knowledge. Semin Immunopathol. (2017) 39:505–16. doi: 10.1007/s00281-017-0628-y
37. Neurath MF. Cytokines in inflammatory bowel disease. Nat Rev Immunol. (2014) 14:329–42. doi: 10.1038/nri3661
38. Wang G, Song Q, Huang S, Wang Y, Cai S, Yu H, et al. Effect of antimicrobial peptide microcin j25 on growth performance, immune regulation, and intestinal microbiota in broiler chickens challenged with escherichia coli and salmonella. Animals (Basel). (2020) 10:345. doi: 10.3390/ani10020345
39. Yu HT, Ding XL, Li N, Zhang XY, Zeng XF, Wang S, et al. Dietary supplemented antimicrobial peptide microcin j25 improves the growth performance, apparent total tract digestibility, fecal microbiota, and intestinal barrier function of weaned pigs. J Anim Sci. (2017) 95:5064–76. doi: 10.2527/jas2017.1494
40. Elluru SR, Kaveri SV, Bayry J. The protective role of immunoglobulins in fungal infections and inflammation. Semin Immunopathol. (2015) 37:187–97. doi: 10.1007/s00281-014-0466-0
41. Hurtado P, Peh CA. Ll-37 promotes rapid sensing of cpg oligodeoxynucleotides by b lymphocytes and plasmacytoid dendritic cells. J Immunol. (2010) 184:1425–35. doi: 10.4049/jimmunol.0902305
42. Shan T, Wang Y, Wang Y, Liu J, Xu Z. Effect of dietary lactoferrin on the immune functions and serum iron level of weanling piglets. J Anim Sci. (2007) 85:2140–6. doi: 10.2527/jas.2006-754
43. Abd El-Hack ME, El-Saadony MT, Shafi ME, Qattan SYA, Batiha GE, Khafaga AF, et al. Probiotics in poultry feed: a comprehensive review. J Anim Physiol Anim Nutr (Berl). (2020) 104:1835–50. doi: 10.1111/jpn.13454
44. Wen LF, He JG. Dose-response effects of an antimicrobial peptide, a cecropin hybrid, on growth performance, nutrient utilisation, bacterial counts in the digesta and intestinal morphology in broilers. Br J Nutr. (2012) 108:1756–63. doi: 10.1017/S0007114511007240
45. Fan C, Wu J, Xu L, Bai L, Yang H, Yan C, et al. A mutated rabbit defensin np-1 produced by chlorella ellipsoidea can improve the growth performance of broiler chickens. Sci Rep. (2019) 9:12778. doi: 10.1038/s41598-019-49252-4
46. Peterson LW, Artis D. Intestinal epithelial cells: regulators of barrier function and immune homeostasis. Nat Rev Immunol. (2014) 14:141–53. doi: 10.1038/nri3608
47. Wu Y, Zhen W, Geng Y, Wang Z, Guo Y. Pretreatment with probiotic enterococcus faecium ncimb 11181 ameliorates necrotic enteritis-induced intestinal barrier injury in broiler chickens. Sci Rep. (2019) 9:10256. doi: 10.1038/s41598-019-46578-x
48. Turner JR. Intestinal mucosal barrier function in health and disease. Nat Rev Immunol. (2009) 9:799–809. doi: 10.1038/nri2653
49. Suzuki T. Regulation of intestinal epithelial permeability by tight junctions. Cell Mol Life Sci. (2013) 70:631–59. doi: 10.1007/s00018-012-1070-x
50. Feng J, Wang L, Xie Y, Chen Y, Yi H, He D. Effects of antimicrobial peptide cathelicidin-bf on diarrhea controlling, immune responses, intestinal inflammation and intestinal barrier function in piglets with postweaning diarrhea. Int Immunopharmacol. (2020) 85:106658. doi: 10.1016/j.intimp.2020.106658
51. Paone P, Cani PD. Mucus barrier, mucins and gut microbiota: the expected slimy partners? Gut. (2020) 69:2232–43. doi: 10.1136/gutjnl-2020-322260
52. Hansson GC. Mucus and mucins in diseases of the intestinal and respiratory tracts. J Intern Med. (2019) 285:479–90. doi: 10.1111/joim.12910
53. Pelaseyed T, Bergström JH, Gustafsson JK, Ermund A, Birchenough GM, Schütte A, et al. The mucus and mucins of the goblet cells and enterocytes provide the first defense line of the gastrointestinal tract and interact with the immune system. Immunol Rev. (2014) 260:8–20. doi: 10.1111/imr.12182
54. Wittkopf N, Neurath MF, Becker C. Immune-epithelial crosstalk at the intestinal surface. J Gastroenterol. (2014) 49:375–87. doi: 10.1007/s00535-013-0929-4
55. Lavelle EC, Murphy C, O'Neill LA, Creagh EM. The role of tlrs, nlrs, and rlrs in mucosal innate immunity and homeostasis. Mucosal Immunol. (2010) 3:17–28. doi: 10.1038/mi.2009.124
56. Frolova L, Drastich P, Rossmann P, Klimesova K, Tlaskalova-Hogenova H. Expression of toll-like receptor 2 (tlr2), tlr4, and cd14 in biopsy samples of patients with inflammatory bowel diseases: upregulated expression of tlr2 in terminal ileum of patients with ulcerative colitis. J Histochem Cytochem. (2008) 56:267–74. doi: 10.1369/jhc.7A7303.2007
57. Kordjazy N, Haj-Mirzaian A, Haj-Mirzaian A, Rohani MM, Gelfand EW, Rezaei N, et al. Role of toll-like receptors in inflammatory bowel disease. Pharmacol Res. (2018) 129:204–15. doi: 10.1016/j.phrs.2017.11.017
58. Fitzgerald KA, Kagan JC. Toll-like receptors and the control of immunity. Cell. (2020) 180:1044–66. doi: 10.1016/j.cell.2020.02.041
59. Yi H, Zhang L, Gan Z, Xiong H, Yu C, Du H, et al. High therapeutic efficacy of cathelicidin-wa against postweaning diarrhea via inhibiting inflammation and enhancing epithelial barrier in the intestine. Sci Rep. (2016) 6:25679. doi: 10.1038/srep25679
60. Corthesy B. Role of secretory iga in infection and maintenance of homeostasis. Autoimmun Rev. (2013) 12:661–5. doi: 10.1016/j.autrev.2012.10.012
61. Bao H, She R, Liu T, Zhang Y, Peng KS, Luo D, et al. Effects of pig antibacterial peptides on growth performance and intestine mucosal immune of broiler chickens. Poult Sci. (2009) 88:291–7. doi: 10.3382/ps.2008-00330
62. Li Y, Jin L, Chen T. The effects of secretory iga in the mucosal immune system. Biomed Res Int. (2020) 2020:2032057. doi: 10.1155/2020/2032057
63. Liu T, She R, Wang K, Bao H, Zhang Y, Luo D, et al. Effects of rabbit sacculus rotundus antimicrobial peptides on the intestinal mucosal immunity in chickens. Poult Sci. (2008) 87:250–4. doi: 10.3382/ps.2007-00353
64. Tanhaiean A, Azghandi M, Razmyar J, Mohammadi E, Sekhavati MH. Recombinant production of a chimeric antimicrobial peptide in e. Coli and assessment of its activity against some avian clinically isolated pathogens. Microb Pathog. (2018) 122:73–8. doi: 10.1016/j.micpath.2018.06.012
65. Tanhaeian A, Shahriari Ahmadi F, Sekhavati MH, Mamarabadi M. Expression and purification of the main component contained in camel milk and its antimicrobial activities against bacterial plant pathogens. Probiotics Antimicrob Proteins. (2018) 10:787–93. doi: 10.1007/s12602-018-9416-9
66. Oshiro KGN, Rodrigues G, Monges BED, Cardoso MH, Franco OL. Bioactive peptides against fungal biofilms. Front Microbiol. (2019) 10:2169. doi: 10.3389/fmicb.2019.02169
67. El-Dirany R, Shahrour H, Dirany Z, Abdel-Sater F, Gonzalez-Gaitano G, Brandenburg K, et al. Activity of anti-microbial peptides (amps) against leishmania and other parasites: An overview. Biomolecules. (2021) 11:984. doi: 10.3390/biom11070984
68. Tahmoorespur M, Azghandi M, Javadmanesh A, Meshkat Z, Sekhavati MH. A novel chimeric anti-hcv peptide derived from camel lactoferrin and molecular level insight on its interaction with e2. Int J Pept Res Ther. (2019) 26:1593–605. doi: 10.1007/s10989-019-09972-7
69. Hancock RE, Haney EF, Gill EE. The immunology of host defence peptides: beyond antimicrobial activity. Nat Rev Immunol. (2016) 16:321–34. doi: 10.1038/nri.2016.29
70. Tang Z, Yin Y, Zhang Y, Huang R, Sun Z, Li T, et al. Effects of dietary supplementation with an expressed fusion peptide bovine lactoferricin-lactoferrampin on performance, immune function and intestinal mucosal morphology in piglets weaned at age 21 d. Br J Nutr. (2009) 101:998–1005. doi: 10.1017/S0007114508055633
71. Peng Q, Zeng XF, Zhu JL, Wang S, Liu XT, Hou CL, et al. Effects of dietary lactobacillus plantarum b1 on growth performance, intestinal microbiota, and short chain fatty acid profiles in broiler chickens. Poult Sci. (2016) 95:893–900. doi: 10.3382/ps/pev435
72. Martin-Gallausiaux C, Marinelli L, Blottiere HM, Larraufie P, Lapaque N. Scfa: Mechanisms and functional importance in the gut. Proc Nutr Soc. (2021) 80:37–49. doi: 10.1017/S0029665120006916
Keywords: antimicrobial peptides, Microcin C7, broilers, performance, immune function, intestinal health
Citation: Dai Z, Shang L, Wang F, Zeng X, Yu H, Liu L, Zhou J and Qiao S (2022) Effects of Antimicrobial Peptide Microcin C7 on Growth Performance, Immune and Intestinal Barrier Functions, and Cecal Microbiota of Broilers. Front. Vet. Sci. 8:813629. doi: 10.3389/fvets.2021.813629
Received: 12 November 2021; Accepted: 26 November 2021;
Published: 07 January 2022.
Edited by:
Iveta Placha, Slovak Academy of Sciences (SAS), SlovakiaReviewed by:
Mohammad Hadi Sekhavati, Ferdowsi University of Mashhad, IranCopyright © 2022 Dai, Shang, Wang, Zeng, Yu, Liu, Zhou and Qiao. This is an open-access article distributed under the terms of the Creative Commons Attribution License (CC BY). The use, distribution or reproduction in other forums is permitted, provided the original author(s) and the copyright owner(s) are credited and that the original publication in this journal is cited, in accordance with accepted academic practice. No use, distribution or reproduction is permitted which does not comply with these terms.
*Correspondence: Shiyan Qiao, cWlhb3NoaXlhbkBjYXUuZWR1LmNu
Disclaimer: All claims expressed in this article are solely those of the authors and do not necessarily represent those of their affiliated organizations, or those of the publisher, the editors and the reviewers. Any product that may be evaluated in this article or claim that may be made by its manufacturer is not guaranteed or endorsed by the publisher.
Research integrity at Frontiers
Learn more about the work of our research integrity team to safeguard the quality of each article we publish.