- 1Department of Biointeraction, Multidisciplinary Institute of Health, Universidade Federal da Bahia, Vitória da Conquista, Brazil
- 2Department of Microbiology, State University of Santa Cruz (UESC), Ilhéus, Brazil
- 3Department of Microbiology, Institute of Biomedical Science, University of São Paulo, São Paulo, Brazil
The Mollicutes class encompasses wall-less microbes with a reduced genome. They may infect plants, insects, humans, and animals including those on farms and in livestock. Ureaplasma diversum is a mollicute associated with decreased reproduction mainly in the conception rate in cattle, as well as weight loss and decreased quality in milk production. Therefore, U. diversum infection contributes to important economic losses, mainly in large cattle-producing countries such as the United States, China, Brazil, and India. The characteristics of Mollicutes, virulence, and pathogenic variations make it difficult to control their infections. Genomic analysis, prevalence studies, and immunomodulation assays help better understand the pathogenesis of bovine ureaplasma. Here we present the main features of transmission, virulence, immune response, and pathogenesis of U. diversum in bovines.
Mollicutes
The Mollicutes Class (phylum Tenericutes) comprises about 200 species. Generally, they are miniscule and considered the smallest self-replicating free-living microorganisms (1–3). Fourteen genera and their representatives are found widely in plants; for example, Candidatus Phytoplasma asteris, Candidatus Phytoplasma australiense, Candidatus Phytoplasma trifolii- (4–9); in animals—Mycoplasma bovis, Mycoplasma gallisepticum, U. diversum (10–12), and in humans—Mycoplasma pneumoniae, Mycoplasma genitalium, Ureaplasma parvum, Ureaplasma urealyticum (13–15). The genera Mycoplasma, Ureaplasma, and Acholeplasma are known to encompass species inhabiting animals as commensals, saprophytes, or pathogens, as shown in Figures 1BII,III (1, 3, 17).
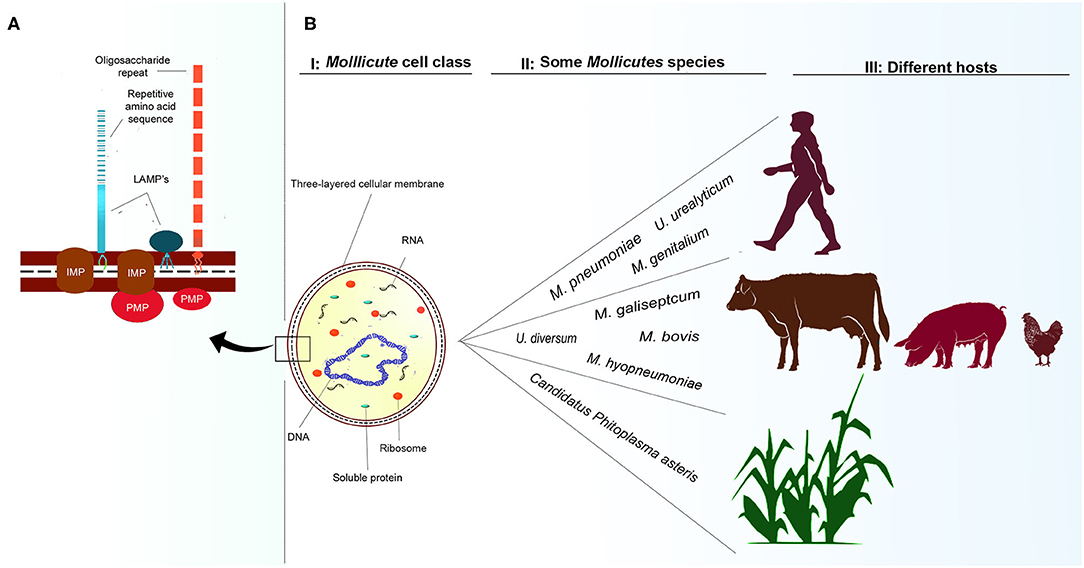
Figure 1. Cellular representation of Mollicutes and some hosts. (A): Mollicutes cell membrane. Representation of LAMPs anchored to the outer membrane of proteins. IMP, integral membrane protein; LAMPs, lipoproteins; PMP, peripheral membrane protein based on the representation of Chambaud et al. (16). (BI): Single-cell structure of Mollicutes demonstrating cell membrane, ribosome, mRNA molecules, DNA molecule, and soluble cytoplasmic proteins. (BII, BIII): some species that infect humans, animals and plants, and some of their respective hosts.
Lacking a cell wall, these bacteria, essentially have a trilaminated cell membrane with incorporated sterols, ribosomes, and a circular double-stranded DNA molecules, as shown in Figure 1BI (1, 2). They reproduce by binary fission and generate pleomorphic or filamentous and multinucleated forms observed through electron microscopy (18, 19). Mycoplasmataceae may be cultured in solid medium and in broth medium. Due to the small size growth in broth, they do not cause turbidity, and bacterial growth is verified an alteration in the pH revealed by the colorimetric change of an acid-base indicator present in the culture medium. In Agar medium, they produce small fried-egg-shaped colonies ranging from 50 to 500 μm (20). Due to their lack of cell wall, Gram staining may allow them to be observed in an optical microscope in a red colored mass.
It is important to highlight that some species produce a polar bleb (21). This structure has been studied mainly for its adherence and the ability of these bacterial cells to travel on glass by caterpillar-like motions (22). In addition, the structure of a complex of organized proteins in M. pneumoniae, a human pneumonic species, has been well-described, elucidating how such proteins are able to adhere to and cause pneumonia in animals (22, 23).
The presence of filamentous wire and rod networks indicate the presence of cytoskeleton-like structures and these may also help in maintaining the morphology. Such filaments may also be involved in division and sliding motility in some mycoplasmas (2, 18, 20).
Mollicute metabolism is quite variable. Species that metabolize carbohydrates for energy production are classified as glucose fermenters. Non-fermenting Mollicutes lack the arginine dehydrolase pathway to obtain adenosine triphosphate (ATP) (24, 25). In these bacteria, the tricarboxylic acid cycle is incomplete, and they also lack quinones and cytochromes. Consequently, the possibility of effective oxidative phosphorylation as a power generating mechanism is ruled out (2, 26). Some mycoplasmas may rely on fermentative or non-fermentative metabolism depending on the conditions of the microenvironment (27). Ureaplasma spp. present a differentiated metabolism; they require urea to generate ATP. Figure 2 and section 3.1 (urea production and modulation in prostaglandin synthesis) provide more details on the production and toxicity of urea produced by Ureaplasma spp. (29). All metabolic diversity of Mollicutes contributes to the colonization of different niches and hosts.
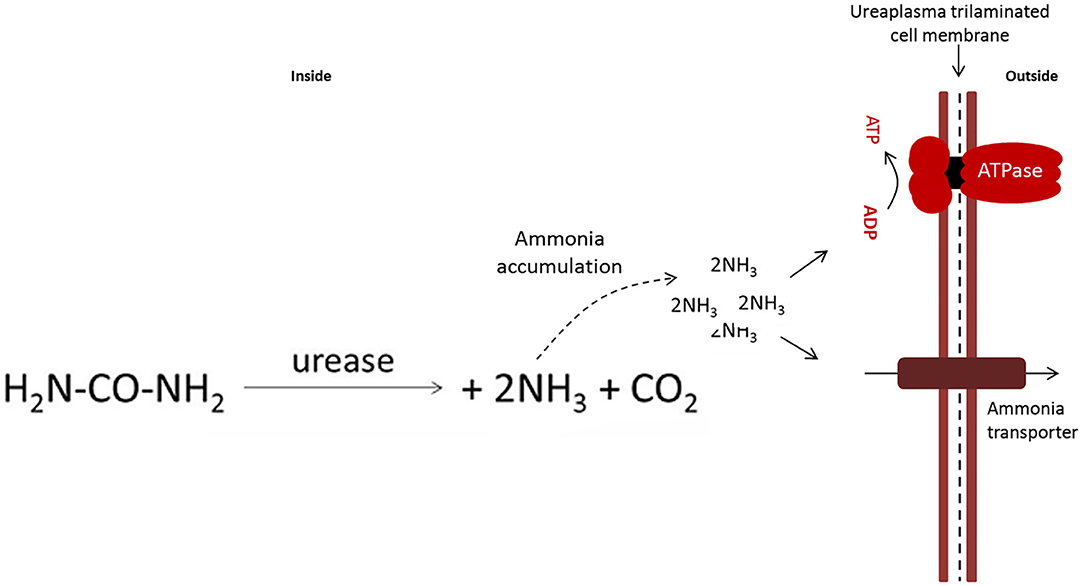
Figure 2. Equation of the conversion of urea to ammonia catalyzed by the enzyme urease present in U. diversum, based on the model by Marques et al. (28). The accumulation of intracellular ammonia generates a gradient that is used to produce ATP. ATP is used as energy and ammonia causes damage to host tissues.
The genome size also permits classification of the mycoplasmas into two groups. One is the genera Mycoplasma and Ureaplasma, with about 580–1,350 kilobase pairs (Kbp), and the others are the genera Acholeaplasma, Spiroplasma, and Anaeroplasma with 790–2,200 Kbp (14, 30–32). The non-essential genes were lost through genomic reduction. These microorganisms have evolved from Gram-positive bacteria by degenerative evolution to lose their peptidoglycan cell wall and some metabolic genes (33). Therefore, Mollicutes require additional supplements for laboratory growth. Thus, the uptake of extracellular nutrients including many biosynthetic precursors such as amino acids, nucleotides, and sterols are essential (34, 35).
In humans, Mollicutes usually cause urogenital and respiratory tract infections (36, 37). In animals, these bacteria prefer to colonize the mucous surfaces of the respiratory, genital, oropharyngeal, ophthalmic tract, as well as the mammary gland, and joints. They usually cause subclinical symptoms or can persist in healthy carrier animals maintaining a persistent parasitic lifestyle (20, 38). Pathogenic infections rarely lead to death; however, in some cases, the infection may be associated with chronic conditions due to the immunomodulation process in the affected region (18, 39, 40). The Mollicute cell membrane has a range of protein structures (Figure 1A), including lipid-associated membrane proteins (LAMPs) that represent important virulence factors. These structures will be described later in section: LAMPs and other surface molecules (41–43).
Mollicutes that infect herds are related to losses in farming, mainly disrupting animal weight gain and reproduction of cows, goats, pigs, chickens, and other species of agronomic interest (44–46). U. diversum is an important mollicute isolated from animals, and it induces a strong inflammatory response in the bovine respiratory and genital tract and is related to reproductive disorders. U. diversum has particular characteristics that make it challenging to control in infectious diseases in beef and dairy cattle, in addition to being a serious obstacle to artificial insemination and embryo transfer (47, 48).
Ureaplasma Diversum
Bovine ureaplasmas (phylum Tenericutes, class Mollicutes, order Mycoplasmatales, family Mycoplasmataceae genus Ureaplasma) were first isolated in 1967 from tissue specimens of the vagina, urethra, and bladder wall of cows (49). For some time, these microorganisms were called Bovine tiny (T)-strain mycoplasma. This denomination reflected the morphological similarities with U. urealyticum cultures, at the time called human T-strain mycoplasma due to photomicrographs of agar T colonies published in the first report on these bacteria (50). In 1956, the term T-strain was applied to distinguish these microbes growing in solid medium with different morphology from other mycoplasmas described at the time (51).
In an attempt to establish distinguishing features, several studies were published comparing morphological characteristics and protein band profiles, by electrophoresis, from T-strains isolated from cattle with human isolates (52, 53). Serological tests showed differences between strains; however, the differences were not sufficiently marked to establish differences between species (53, 54). The protein profiles of ureaplasma by polyacrylamide gel electrophoresis showed non-shared peptides, indicating that they were different microorganisms (54). The confirmation was detailed in the DNA composition and the guanine plus cytosine (G + C) content. The human T-strain presented C+G 27.4 mol% (55) and bovine T-strains had 29.3% (52). Other T-strains were isolated from different animals including sheep and goats and the molecular methods allowed for these to be differentiated from bovine isolates (53, 56).
In 1974, the perception that isolated T-strains differed from conventional mycoplasmas prompted the creation of the genus Ureaplasma to encompass Mollicutes possessing the enzyme urease (51). “Bovine T-strains Mycoplasma” formed the species U. diversum. The species-specific epithet (diversum, from Latin meaning different) refers to the differences in polypeptides and G + C content compared to U. urealyticum, and the differences in the peptide profiles of the antigenic structures (determined by electrophoresis) from different isolates (54).
Many studies investigated serological grouping of U. diversum isolates. Initial studies using rabbit antisera, conducted in different laboratories by Howard et al. (57) and Ogata et al. (58), selected eight and seven strains, respectively, to represent the totality of antigens synthesized by ureaplasmas. Later, the new isolates, allowed for identifying expanding serotypes to eleven (59). Rabbit-produced antisera showed three distinct groups, two of which were serologically similar but not identical (57, 58). An important step in serological clustering was taken by Howard and Gourlay in 1981 when they selected one strain from each of the three antigenic clusters for antiserum production in calves (60). The antisera produced were tested in various U. diversum strains from different countries (Denmark, the United States, Canada, Ireland, England, and Japan), from symptomatic or healthy animals, and isolated ureaplasma from different anatomical regions (lung, foreskin, semen, urogenital tract, endometrium, and eye). The strains reacted with at least one of the three bovine antisera and were, therefore, classified into three clusters: cluster A, which is represented by strain A417; cluster B, represented by strain D48 or C, represented by strain T44 (59, 60).
Serotype distribution studies found that most strains were type-B strains isolated from cows with vulvovaginitis and infertility. However, there is no direct relationship between serotype and a specific disease (61). In general, all three serotypes are found in the reproductive tract of cattle (62). Despite the possibility of allocating isolates in clusters A, B, or C, Howard (63) previously warned that the serological relationship of antigens possessed by bovine ureaplasma determined by antisera or protein profile electrophoresis detect the relationship of some selected strains and not the complete dissemination of antigens present in U. diversum. In fact, studies by Marques et al. (64) reported high variability in the sequence of 16S rRNA gene fragments from ureaplasma isolated from healthy and unhealthy cattle. The significant number of single nucleotide polymorphisms (SNPs) found compared to the U. diversum sequence deposited at GenBank indicated intraspecific variability within these organisms. This variability may be translated into antigenic diversity of different strains and, consequently, generates different forms of interaction with the host (42, 64–66).
In morphological terms, U. diversum share the general characteristics of the Mollicute Class. They are pleomorphic (coccoid or coccobacillary), approximately ranging from 400 to 500 nm in diameter, microaerophilic (28, 54), with an optimal growth temperature of 37°C, but can grow between 33 and 37°C. The ideal growth pH ranges from 6.0 to 7.0. In a solid medium, they produce colonies of relatively small size like other microorganisms classified in the genus Ureaplasma (54). Observations from photomicrographs indicate that these colonies can range from 100 to 175 μm in diameter (67). Urea is an absolute requirement for growth. Hydrolysis of urea leads to ammonia formation; therefore, it increases in vitro pH (68), which is a striking feature of ureaplasmas differentiating them from other Mollicutes (2, 69). Although they also do not have a cell wall, they have a polysaccharide coating on the membrane forming a capsule 11–17 nm in diameter consisting of arabinose, xylose, mannose, galactose and glucose (28).
Host Interaction With the Microorganism and Pathogenicity
U. diversum colonizes cattle mainly by the respiratory and genital/reproductive tract (47, 70–74). This microorganism is considered an opportunistic pathogen found in mucous membranes and secretions of the vulva, vagina, and udder of cows, causing severe granular vulvovaginitis, salpingitis, endometritis, mastitis, placentitis, and fetal alveolitis that may result in miscarriage or birth of weak calves (10, 31, 68, 75–77). The risk of infections is higher in younger cows, resulting in fewer births (78). Nevertheless, the infection is not dependent upon the presence of clinical symptoms, since the first isolates were, apparently, in the genital/reproductive tract of healthy cows (49). U. diversum was also isolated from the nasal cavity of asymptomatic calves (68). The presence of these microorganisms in healthy cattle herds makes it difficult to identify risk factors for infection, which makes establishing infection control strategies more difficult.
U. diversum can be sexually transmitted, and there is significant potential for horizontal and, in some cases, vertical transmission between animals (62, 79). One of the main forms of transmission is through coitus (natural reproduction), but infected bulls also spread ureaplasma through artificial insemination and breeding. The Mycoplasma spp. and Ureaplasma spp. are present in secretions, especially semen, preputial and vaginal mucus, conjunctival secretion, and milk (80, 81). Artificial insemination and embryo transfer are also potential sources of contamination (47, 80).
Pathogenicity in Cows
Granular vulvitis is common in ureaplasma-infected cows (82–85). The disease appears 1–3 days after vulvar inoculation in heifers (82, 83). Initially, acute infection involves mucopurulent discharge and mild vulvar epithelium hyperemia. The granularity can be classified as mild or severe. The mild granularity, usually starting from 24 to 48 h, appears laterally to the clitoris, with granules smaller than 1 mm in diameter and with light coloring. They are usually best observed with an oblique light source. Severe granularity is readily apparent without the need for additional light and usually involves the lateral vulvar wall as well as the clitoris area. The granules are usually red and larger, 1–2 mm in diameter (82, 83, 85). Heifers with severe granularity also have slightly edematous vulvar lips and purulent or mucoid discharge. Ureaplasmas are easily recovered from vulvar cultures of infected cows, fetuses, and calves after autopsy (47). Histological slides are characterized by focal and/or diffuse lymphocyte infiltrates both inside and just below the vulva mucosa, thus confirming vulvitis (47, 82, 83). In the vagina, vulva, and clitoris there are usually very serious lesions characteristic of Granular Vulvovaginitis Syndrome (GVS) (47, 85, 86).
During infection, the Fallopian tubes, uterus, cervix, vagina, and vulva fragments, as well as the lower reproductive tract, are slightly disturbed and present acute inflammatory process (82, 83). The histopathological findings of the reproductive tract of naturally infected U. diversum females are consistent with lesions that hinder fertilization (47, 87). Therefore, animals with GVS should be identified initially in the herds because, in addition to reduced fertility rates caused by tissue damage, they may contribute to spreading the microorganism (85). Chronic infection is also possible and can cause a variety of harm to animals (82, 83). Asymptomatic cows may chronically carry U. diversum and function as pathogen reservoirs and are, therefore, a major source of infection. Bovine ureaplasmas have surface compounds, such as variable surface lipoproteins (VSPs) that allow them to evade the host's immune system, invade cells, and help develop chronic infection (28, 42, 88–90).
Chronic GVS due to U. diversum if not diagnosed, may progress to endometritis (ascending route) and results in miscarriage or infertility (81, 91, 92). The descending pathway was also demonstrated by intrauterine and cervical inoculation in heifers. Inoculating these ureaplasmal strains in the uterus induces granulous vulvitis, endometritis, and salpingitis. Cervicitis can occur between 2 and 5 days after inoculation. Cervical damage alters the pH of the local mucus and provides an adverse environment for sperm survival (82, 83). Thus, the pathogenesis in the reproductive tract of cows should be considered significant, as granular vulvitis, endometritis, salpingitis, or cervicitis may result in reproductive losses (92). In oviduct tissue specimens, the most frequent lesions observed histologically are epithelial desquamation with basement membrane exposure, lumen clot formation, curvature, or absence of cilia, as well as a cytoplasmic projection of many epithelial cells toward the lumen (82, 83). In the cervix and uterine body, the lesions are also severe, destroying the epithelial cell cilia and resulting in ulcerated areas. Epithelial lesions such as vacuolization of epithelial cells, spongiosis, loss of epithelium with basement membrane exposure, and lymphocyte accumulation are observed in fornix tissue specimens histologically (85, 92).
Because ureaplasmas have no rigid cell wall and require a microaerophilic environment to grow, the survival of these organisms is compromised in the womb (93). However, more favorable situations, such as in the estrous cycle, when the cervix is open, or during artificial insemination, the infection becomes more probable. U. diversum seeks whole cells for parasitism since adherence and parasitism of epithelial cells are essential conditions for their survival. Supporting these findings Lingwood et al. (94) showed that U. diversum is able to bind to the surface of male sperm and in endometrial cells. Later Kim et al. (95) established an in vitro model of endometrial infection. These early studies supported the growth of ureaplasmas within uterine endoemetric cells. The local effect of infection is likely to occur through the process of constant reinfection rather than the release of other opportunistic organisms (85). In humans Ureaplasma spp. were also detected by next-generation sequencing (in a study that also used PCR and culture for detection) as the most prevalent uterine colonizer in pregnant women with and without chorioamnionitis, specifically, individuals in premature births with severe chorioamnionitis had high abundance of U. parvum (96). There is also a positive association between the detection of U. parvum in samples of placental tissue and abortion (97). Therefore, these studies suggest that ureaplasmas that infects humans or animals can colonize/infect the placenta and amniotic fluid for long periods during pregnancy in the absence or presence of adverse outcomes.
U. diversum infection/colonization in fetal lungs or after the endobronchial inoculation of this ureaplasma is associated with abortion and newborn death in cows. The death of calves may be linked to the development of catarrhal lobular pneumonia (98–100). A high prevalence of U. diversum has been detected in cases of abortion, calf morbidity (Table 1). Pulmonary pathology in fetuses usually presents with lymphocyte infiltration and conjunctivitis (92, 100, 104, 106, 107, 111, 112). Also, typical features of an abortion caused by U. diversum include a relatively fresh fetus and frequently retained and inflamed placenta (113). Postmortem examinations performed on fetuses, late abortion, and weak-born calves tested positive for U. diversum in tissue specimens collected from the placenta, lungs and abomasal fetal fluid. These findings were accompanied by diagnoses of placentitis, conjunctivitis, and pulmonary pathology, suggesting the establishment of an immune-mediated response. In these animals, the presence of neutrophilic bronchopneumonia may range from moderate to severe and may be diffuse or acute (47).
Milk production is also affected mainly due to the development of bovine mastitis, inflammation of the mammary gland, which is one of the main diseases in dairy herds (114). Bovine ureaplasma produces clinical mastitis along with visible changes in milk and udders (115). Mastitis caused by Mollicutes is less common than mastitis caused by other bacteria, but results in severe udder disease (116), in dairy herds, Mollicutes can cause clinical, subclinical or chronic mastitis (117). When experimentally inoculated in the udder of cows U. diversum excretion and an increase in the number of cells in milk were detected. In some cases, milk secretion ceases completely. Histopathological examination of the udder sections reveals neutrophil infiltration along with interstitial hyperemia (115), This is a major problem for milk production and animal welfare in large dairy herds (114).
Pathogenicity in Bulls
In bulls, U. diversum is involved in cases of seminal vesiculitis, balanoposthitis, epididymitis and other pathologies caused by morphological and functional changes in sperm (88, 91, 102). Ureaplasmas can colonize both the proximal and distal portions of the urethra and the preputial cavity, which allows for semen contamination (102, 118). The preputial cavity and urethra appear to be the main means of semen contamination. Thus, procedures to reduce the number of microorganisms in the foreskin may not be effective enough in preventing semen contamination (119). Several studies have identified U. diversum in bull reproductive tract fluids including fresh semen (120, 121), preputial mucus samples and distal urethral secretion (74). Microorganism adherence interferes with spermatogenesis, sperm transport, capacitation, and fertilization (102).
Bulls positive for U. diversum but without genital lesions may have abnormally-tailed (folded and coiled) sperm, as well as sperm surface abnormalities (small bulges). They also have irregularities and infection of bacteria in the entire length of their bodies from head to tail. This suggests impaired sperm function and possibly infertility (74, 102).
Interference With Artificial Insemination (AI) and Embryo Transfer
Some viral, bacterial, protozoan and parasitic organisms may be transmitted through bovine semen. In some cases, the presence of infectious agents is sufficient for their transmission to heifers or cows through natural reproduction or artificial insemination (AI) (122).
Le Grand et al. (121) demonstrated a high frequency (74%) of ureaplasma in the semen of bulls intended for AI. In this sense, U. diversum infects semen destined for AI, colonizes blastocysts and fetuses and induces gestational losses or birth of weak calves. In studies by Crane and Hughes, (47) the losses between suspected or confirmed embryogenic pregnancies for infection with this bacterium were 67%. In these studies heifers did not have fertility problems, rather pregnancy loss and stillbirths were the prominent clinical problems. This suggests that embryos are the source of the U. diversum infection, abortions, stillbirths, and in weak neonates could be how the disease manifests.
In AI, it is important to control the population of microorganisms in the semen and, thus, prevent the introduction of diseases in individual animals or herds (123). However, foreskin in healthy semen donors contains numerous bacterial species that can mix with semen during ejaculation and during collection. Some of these bacteria can pose a significant risk for inseminated females. Therefore, it is important that control is achieved through the use of antibiotics and appropriate hygiene techniques (124). Along with the advent of AI techniques, embryo processing techniques have been developed to reduce the risk of transfer of infectious diseases by bovine embryos derived from this technique (125). Ureaplasmas are recognized as one of the few potential pathogens that are not removed from embryos through the IETS (International Embryo Transfer Society) recommended washing and cleaning procedures (113, 126).
Penicillin and streptomycin as well as gentamicin are the most common antibiotics used to control microorganisms in embryo transfer techniques, without any apparent effect on the development of embryos of domestic animals. They are effective against a variety of Gram-positive and Gram negative bacteria. Generally these antibiotics are used in combination with each other (124, 127). Despite this, for structural (as already discussed) and resistance issues, standard antibiotics present in embryo processing media are not effective against Mollicutes (124). Therefore, antibiotics that act on the cell wall, such as penicillins, do not act agaisnt U. diversum (due to the absence of a cell wal in these bacteria). In theory, antibiotics that are unable to penetrate biological membranes (such as gentamicin) would also not be 100% effective against bovine ureaplasmas because these microorganisms are capable of embedding themselves in sperm and blastocysts (42, 102). Some alternatives to fight mycoplasmas are being developed, such as an enzymatic treatment (128), immunological methods (with the use of specific antibodies against the infectious agent) and photosensitive dyes and chemical compounds with germicidal effects (124, 127, 129). All of these alternatives can contribute to the development of effective control methods against molicutes that infect semen and embryos destined for AI and embryo transfer.
Forms of contamination during the embryo transfer procedure are also possible. U. diversum present in the vulva of donor cows (cows from which the embryos will be removed) can contaminate the catheter or discharge solutions and remain in recovered bovine embryos. U. diversum can also be carried in the vulva of donor cows or recipient cows (cows that will have an embryo inserted in their uterus) to the animal's uterus during AI and embryo transfer (47). Veterinary diagnostic laboratories generally do not use polymerase chain reaction (PCR) methodologies to detect Ureaplasma spp.; most of the time the culture method is used. Therefore, some abortions caused by U. diversum can be misdiagnosed. Colonization of the reproductive system at the time of embryo collection allows U. diversum to adhere and disturb embryo development (47).
Gaeti et al. (87) found by PCR methodology a conception rate for embryo transfer of 64.8% in heifers positive for ureaplasma, including those with vulvar lesions. This prevalence is similar to the average conception rates of embryo transfer programs in cattle ranging from 50 to 60.4% (130, 131). Indeed, these percentages suggest initially that U. diversum does not affect conception rates and that embryo transfer does not appear to increase the risk of infertility among infected heifers (87). However, a good conception rate may not correlate with the absence of miscarriages and the birth of healthy calves. In humans the infection by Ureaplasma species was associated with chorioamnionitis (regardless of gestational age), spontaneous abortions or miscarriages, and neonatal respiratory diseases. The presence of these microorganisms in the upper genital tract of non-pregnant women suggests that these microorganisms may infect the embryo at the time of implantation and can adversely affect the health of the pregnancy and neonate (132–134). U. diversum may remain in cells internalized at early fetal development, such as in the blastocyst, without cytopathic effects (42). Thus, placenta, ocular conjunctiva, and lung tissue could be colonized at late stages of development or after calving; thus causing pulmonary placentitis, conjunctivitis, and pathology in calves (47).
Emerging Pig Isolation Cases
Mollicutes usually exhibit high specificity to host tissues. However, some Mycoplasma ssp. have been isolated from unusual hosts (18, 135, 136). It is possible that by overcoming the species barrier, Mollicutes have colonized other hosts and become pathogenic regardless of their phylogenetic distance (135).
For many years now, U. diversum has been isolated from cattle and associated with various diseases in the genital and respiratory tract, but the ability of these bacteria to colonize swine has recently aroused interest in the scientific community. In 2013, Lobo et al. (71) isolated Ureaplasma spp. of pig lung with typical lesions of enzootic pneumonia. The association of ureaplasma with porcine lung lesions led to studying U. diversum in these hosts. In 2014, the first study reported that U. diversum may infect swine and be associated with pneumonia. However, unlike cattle, these bacteria were only isolated from sick animals (72).
In swine, U. diversum showed a higher affinity to lung tissue (72, 108). In Canada, a study in tissues/organs of swine detected U. diversum only in lung tissue. In the same study a coinfection with other mycoplasma and ureaplasma species such as M. hyopneumoniae, M. hyorhinis was also identified (108). The role of U. diversum in swine remains unknown. The main techniques used to identify U. diversum in pigs have been conventional PCR or qPCR. Although these techniques have high sensitivity and specificity, the inclusion of serological tests can contribute to a better characterization and knowledge of the swine isolates. Therefore, it is not yet possible to determine whether this species is an opportunistic pathogen or a primary agent involved in Swine Respiratory Complex diseases (71, 72, 108).
Impacts on the Livestock
Reproductive disorders, animal development and positive cultures to U. diversum or association with other bacteria and viruses, makes this ureaplasma an important threat to raising cattle for large producers (86, 118). This requires special care and quality control for meat production and dairy activity in countries such as Brazil, the United States, China, and India (137–141).
Artificial insemination, embryo transfer, in vitro embryo production and the use of somatic cell nuclear transfer or cloning has delivered several benefits to herd productivity (142). However, these techniques do not eliminate the occurrence of Mollicutes and reproductive disorders in cattle (143, 144).
Several Mollicutes have been isolated in the bovine urogenital and respiratory tract or aborted fetuses, including M. bovis, M. bovigenitalium, U. diversum, among others (11, 93, 104, 110, 114, 145). U. diversum is related to reproductive disorders, weight loss and reduced milk yield in cattle. These disorders also increase veterinarian and pharmaceutical costs causing significant economic losses in the livestock sector (79, 146, 147).
Diagnosis
The first techniques used for U. diversum diagnosis were culturing and producing typical colonies, urea hydrolysis, specific serology, and DNA or protein profiles. The medium, known as Hayflick's medium, presently used in most diagnostic labs for U. diversum identification has undergone few changes over the years. Hayflick's medium contains a combination of mycoplasma broth, yeast extract, horse serum, urea crystals, phenyl red, and penicillin (42, 59). The urease activity and ammonia production raises the pH of specific broths. In solid medium the precipitation of cations Mn, due to urease activity, produces brown-colored colonies (10, 148). Usually with lysed isolates and the immunodiffusion methodology, ureaplasma are serologically characterized with polyclonal antisera, usually rabbit or calves (79). Some studies have shown that molecular diagnosis methods provide better results to detect bovine ureaplasma. PCR assays have shown high specificity and several recently published studies have used the rRNA 16S gene as a basis for primer construction (68, 79, 149).
Conventional PCR using primers UD1 (forward: 5′-CCG GAT AAT AAC ATT TTG-3′) and UD2 (reverse: 5′-CCT TGC GGT AGC ATC GA-3′) as well as nested PCR using UD3 (5′-AAT GTC GGC TCG CTT ATG AG-3′) and UD4 (5′ -CCT GTC ATA TTG TTA ACC TCC GC-3′) were tested to identify U. diversum in animals with signs and symptoms of granular vulvitis or reproductive dysfunction. Nested PCR diagnosis showed high specificity (73.1%), efficiency (82.7%), and sensitivity (100.0%) compared with culture method: metabolites and serological tests (79). This PCR methodology, with use of the same pairs of primers targeting the gene encoding 16S rRNA, was used by Buzinhani et al. (86) for detecting U. diversum in isolates from different Brazilian farms and also showed a higher sensitivity compared to culture techniques.
Marques et al. (68) developed primers and TaqMan probes based on the 16S rRNA gene for quantitative detection of U. diversum by real-time PCR (qPCR). The test that was developed demonstrated high sensitivity and specificity for ureaplasmal detection and quantification in swab samples, being able to detect quantities as low as only 10 copies of the genome/reaction. The qPCR methodology was 100 times more sensitive than conventional PCR, and there was no cross-reactivity with other Mollicutes or eubacteria. In fact, the qPCR sensitivity and specificity make this technique useful for the diagnosis of U. diversum as also mentioned in other studies, as shown in Table 1 (108, 149).
Mollicutes in general are fastidious bacteria and difficult to culture and detect; this hinders the collection of epidemiological data and understanding of pathogenesis (10). The importance of U. diversum infections in cattle, the use of PCR in diagnosis, and sharp detection of this mollicute in clinical materials will help better control this microorganism (10, 68, 79, 86, 149).
Prevalence
The prevalence varies according to several factors (Table 1) including several important ones that follow: (1) the cattle population size in a country studied. In Brazil, which has one of the largest cattle herds in the world (150), prevalence may even vary between different regions; (2) health status of the host animal (healthy or unhealthy); (3) sex and stage of animal development (cow, bull, young calf, calf, fetus); (4) anatomical area studied (regions of the lower or upper reproductive tract, respiratory tract, eye conjunctiva) and (5) the diagnostic technique used (culture, PCR). The variations and prevalence of U. diversum are presented in Table 1, which compiles some data on the recent infection prevalence in cattle and swine.
Virulence of U. DIVERSUM
Mollicutes have virulence mechanisms and also use host cell nutrients. Virulence factors include the following: (1) the production of toxic primary metabolic compounds such as ammonia or oxygen hydroxide (2) Adhesion and invasion in host cells; (3) LAMP compounds, and (4) modulation of apoptosis mechanisms. Many of these mechanisms have been studied in U. diversum; however, some virulence and pathogenic factors remain unexplored (28, 42).
Urea Production and Modulation in Prostaglandin Synthesis
Ureaplasma do not have a complete arginine dehydrolase pathway (151). Thus, urea hydrolysis plays an important role in energy metabolism of ureaplasmas and is an important energy source through the production of ATP (152–154) as shown in Figure 2. Ajello et al. (155) showed that ammonia can be produced by the degrading L-histidine. This substance can also be produced by the activity of L-histidine ammonialyase detected in ureaplasmas. There is no data in the literature on the pathogenic effects of the expression of the urease gene by U. diversum; however, in these bacteria, the urease genomic cluster was identified, with more than 90% homology to the human Ureaplasma spp. coding sequence (CDS) (28, 90). Hydrolysis of urea in ureaplasma generates an electrochemical gradient through accumulation of intracellular ammonia/ammonium. The gradient fosters a chemiosmotic potential that generates ATP (156). Thus ATP production is associated with three enzymatic components: urease, (three-subunit urease+accessory proteins), an ammonia/ammonium transporter, and a FOF1-ATPase– Figure 2—(28, 153, 154).
Ureaplasmal ammonia release in a tissue irritates the mucous membranes of the urogenital and respiratory tracts. The mucosal cells also have targets for adhesion and colonization of ureaplasma strains (153, 154, 157, 158). In addition, the ammonia may also intoxicate adjacent tissues.
Another virulence factor is the significant decrease in prostaglandin E2 and F2a by endometrial cells after ureaplasmal infection (95). Prostaglandins are necessary for implantation of embryos and maintenance of pregnancy in cattle (95, 159). Ureaplasmas interfere with prostaglandin biosynthesis due to their phospholipases in membranes (160). The genes for phospholipase D family proteins and triacylglycerol lipases in bovine ureaplasmas may increase the release of arachidonic acid and inhibit the substrate for prostaglandin synthesis (28). The phospholipase D gene was found in 40% of 45 U. diversum isolates from different regions of Brazil (65). The presence of prostaglandins in humans and other animals has also been associated with other Mollicutes. These molecules were observed in human fetal membranes infected by U. parvum (161).
Prostaglandins are strong regulators of cellular activities in reproductive processes. Prostaglandins are necessary for implantation of embryos and maintenance of pregnancy (160). Alteration of this context by ureaplasmas, probably by the activity of phospholipases, can induce labor (153). Prostaglandins can have a pro-inflammatory action on the host's reproductive system; inflammation ultimately initiates preterm labor (162). This is in line with several studies associating bovine U. diversum with premature births (47, 86). Despite this, the role of phospholipases in U. diversum needs to be studied in more detail.
LAMPs and Other Surface Molecules
Structurally the cell membrane of Ureaplasma spp. has the following three layers: two border electron-dense layers with a middle less dense layer, which interacts with the external environment, as shown in Figure 1 (163, 164). LAMPs represent a mixture of cell surface-expressed mycoplasmal lipoproteins interacting with the host cells and are the major molecular patterns associated with pathogens in various mollicute species (16, 164, 165). They are attached to the membrane mainly by electrostatic interactions and thus can be easily released (16, 166). A large number of expressed lipoproteins account for a significant portion of the membrane mass (16, 167–169). These LAMPs play an important role at the site of infection causing inflammation by activating or inhibiting apoptosis: Probably through the breakdown of extracellular ATP into adenosine, this inhibits the growth of various types of cells and even induces apoptosis (170, 171); in the ATP-binding cassette (ABC) transport system (172); in the virulence of different strains, mainly due to the fact that many LAMPs act as VSPs (173, 174), in cell adhesion, as described in section Cell adhesion and invasion (175, 176), and in immunomodulation of the host response, as described in the section Inflammation and Immunomodulation (42).
In the bovine ureaplasma genome, genes encoding 37 unknown lipoproteins were found, as well as one VSP with 36% identity with the M. pulmonis variable surface antigen lipoproteins (VsA) (28, 90). VSPs undergo constant variations (16, 177). A variety of mechanisms may be involved in these antigenic variations, including phase variation (178), variation in the number of tandem repeats at the carboxylic terminus (179, 180), masking by another surface antigen (181), and (177). Studies with other Mollicutes show that VSPs are related to cell adhesion (182, 183), and induce proinflammatory or anti-inflammatory response (175).
The presence of multiple band antigen (MBA) coding genes in U. diversum plays a role in antigenic variation as mechanisms for rapid adaptation to changes in the microenvironment (28). The MBA contains a signal peptide and an acylation site in the N-terminus. The C-terminus is composed of multiple serial repeats (184, 185). Uchida et al. (186) demonstrated that MBA from U. parvum is a potential virulence factor and causes intrauterine fetal death and preterm delivery after injection into the uterus on day 15 of gestation in pregnant mice. This study also showed that the N-terminus diacylated lipopeptide is essential for the onset of inflammation that causes reproductive disorders (186). Antigenic variation of MBA has not yet been investigated in U. diversum, but in U. parvum these variations are correlated with the severity of the infection. Knox et al. (187), using a pregnant sheep model, showed that larger numbers of mba/MBA size variants generate low inflammation response within the chorioamnion and little or no chorioamnionitis. In ureaplasma isolated from human placentas, the variation in the size of mba/MBA was associated with a reduced incidence of histological chorioamnionitis and significantly lower levels of the cord blood proinflammatory cytokines (134). In this way, by avoiding the immune recognition of the host through antigenic variations, so that ureaplasmas collaborate for a less severe infectious pattern that would cause choriomyionitis, and on the other hand, these microorganisms are established as an asymptomatic chronic infection (188). In the intrauterine fetus, this establishment may cause chorioamnionitis, preterm birth or fetal death (134, 186, 188).
In the U. diversum genome, genes encoding hemolysin were found, being an enzyme that causes blood cell lysis, and MIB-MIP system genes (MIB: Mycoplasma Ig binding protein; MIP: Mycoplasma Ig protease). MIB acts as an IgG-binding protein, while MIP cleaves the IgG heavy chain (28, 90). U. diversum genome has several CDS for potentially immunogenic molecules (LAMPs, VSPs, MBA, hemolysin, and MIB-MIP system); however, the role of most of these molecules has not yet been thoroughly investigated. Identifying the function of these immunogenic molecules may represent a significant advance in the understanding of the pathogenesis caused by ureaplasmas. Modification of some membrane antigens can provide an effective strategy to evade the host immune system (189).
Cell Adhesion and Invasion
U. diversum is a facultative intracellular microorganism (88). The adhesins are responsible for adhesion and facilitate the process of cell invasion. These molecules also enable the ureaplasmas to use the host cell nutrients and facilitate the cell invasion process. Invasion is a complex process involving mollicute adhesins and cell receptors (190). Once adhered, these bacteria interact with membrane receptors or interfere with the transport mechanism of host cells rendering them vulnerable to cytotoxic metabolites and cytolytic enzyme activities (190, 191).
Macromolecules of different Mollicutes have been identified and related to cell adhesion. Among the most commonly studied are M. pneumonia P1 and P30 proteins (23, 192), and M. gallisepticum GapA and CrmA proteins: cytadherence-related molecules (193). In M. bovis proteins P26 (194), α-enolase, adhesion-related factor (195), and members of the VSPs family with adhesion function were identified (175). The CDS for various surface proteins have been found in the U. diversum genome. In addition, the presence of capsules and LAMPs were associated with increased virulence and promote adhesion to the host cell (28).
The invasiveness is closely related to cell adhesion (31, 36). The invasion has already been described for several species of Mollicutes including cellular invasion by M. penetrans, M. genitalium (196) and, M. gallisepticum (197). Cellular internalization by U. diversum was first described by Marques et al. (198) using Confocal Laser Scanning Microscopy. Internalization in Hep-2 cells was detected 1 min after infection. The microorganisms after 3, 8, and 24 h were detected around the perinuclear regions; however, the nuclear invasion was not verified (198). The invasion in the bovine sperm described by Buzinhani et al. (88) emphasizes the role of these microorganisms in reducing sperm motility and in causing seminal vesiculitis, and epididymitis. Regarding the reduction of reproductive efficiency, Santos-Junior et al. (42) demonstrated that U. diversum is able to embed within blastocysts and induce higher gene expression of interleukin 1 beta (IL-1β) and tumor necrosis factor alpha (TNF-α) compared to uninfected blastocysts. This suggests the existence of an early signaling system to respond to bacterial infections capable of protecting embryos against mollicute infections (199).
Cell invasion is an important virulence factor in Mollicutes and can be considered a strategy for persistence in immunocompetent hosts. These hosts also provide essential nutrients for Mollicutes (89, 198, 200, 201). Intracellular localization also protects Mollicutes from antibiotics and hinders their elimination from the infected cell cultures (2). Gentamicin is an example of an antibiotic that cannot penetrate mammalian cells when in low concentrations, therefore, this antibiotic is not effective against U. diversum and other internalized Mollicutes in mammalian cells (198). The mechanisms inherent in the adhesion process have not been completely elucidated in Mollicutes. Some approaches show that plasminogen plays an important role in M. fermentans invasion. When M. fermentans is exposed to plasminogen, they are able to invade HeLa cells; however, the unexposed cells lose their invasive abilities, showing the plasminogen dependence on the invasion process (202). Phospholipases are also related to cell invasion; these enzymes, already identified in U. diversum, are able to cleave membrane phospholipids and gain access to the host cell cytoplasm (191, 198, 200, 203).
Apoptosis
The LAMPs of Mollicutes may also modulate apoptosis mechanisms (171). Mycoplasma bovis induces apoptotic death of bovine lymphocytes through a mechanism in which lipoproteins play an important role (204). Several other Mollicutes demonstrate the ability to modulate apoptosis in different cell lines (205–207).
The ability of U. diversum to induce cellular apoptosis was found after inoculation in Hep-2 cells. The number of apoptotic cells was higher than uninfected cells. However, the number of apoptotic cells decreased over time. Such reduction may be related to the persistence of this microorganism in the intracellular environment where it can be protected from host immune factors (89). In infectious diseases, apoptosis can be characterized as a defense mechanism against intracellular microbes that do not necessarily have any mechanism to impede them. However, excessive apoptosis of immune cells can affect the immune response at the primary site of infection and thus facilitate the diffusion of mycoplasma (205, 208).
Inflammation and Immunomodulation
The immune reaction against Mollicutes is mediated by T and B lymphocytes (209). Therefore, it is suggested that a chronic adaptive response develops with consequent immunoglobulin secretion, antibody opsonization, complement system activation and infiltration of neutrophils and macrophages (85, 210).
U. diversum in bovine vaginal lesions revealed epithelial necrosis, infiltration and cell proliferation (85). In uterine tissue specimens, the glandular epithelium is damaged by infiltrating leukocytes and eosinophils in the submucosa. Edema, connective tissue proliferation, muscle fiber necrosis, and small areas of epithelial ulceration may be observed histologically (82, 83). Pulmonary histopathology of fetuses and calves is characterized by significant infiltration and accumulation of lymphocytes throughout the lung, near the vessels and bronchioles, as well as in the alveolar tissue (47).
Ureaplasma spp. surface proteins are the main initiators of the inflammatory response during infection (28, 42, 164, 183). The interaction between LAMPs and toll-like receptors (TLRs) initiates signal transduction pathways that promote inflammation-related gene transcription (211). However, an anti-inflammatory profile can also be established. The ureaplasma strains may induce the production of different cytokine profiles depending on the surface antigens (41, 43).
Proinflammatory Profile Induction
In several Mollicutes the production of proinflammatory cytokines is associated with interactions of LAMPs with TLRs (41). The signaling pattern differs among Mollicutes and the TLRs are part of the signaling process (41, 212, 213). Therefore, intracellular recruitment of myeloid differentiation factor 88 (MyD88), activation of nuclear factor-κB (NF-κB) and activator protein 1 (AP-1) occurs (41, 211). These transcription factors play a central role in the induction of cytokine and chemokine profiles (214, 215), such as interleukin (IL-8), Monocyte Chemoattractant Protein-1 (MCP-1), Macrophage-1a Inflammatory Protein (MIP-1a), Macrophage and Granulocyte Colony-Stimulating Factor (GM-SFs), as well as prostaglandin and nitric oxide (18, 216). These molecules are associated with a strong inflammatory response (217–219).
Inoculation of viable or inactivated U. diversum in bovine macrophages increases TLR gene expression and association with IL-1β and TNF-α production, and induction of a strong inflammatory state (28). These findings are consistent with the clinical status of cow infections. These cytokines appear to play a central role in the response to Mollicutes. Several studies have described their involvement both in vivo (220) and in vitro (41, 221, 222). Human mononuclear cells infected with Mycoplasma pneumoniae, M. hyorhinis, M. arginine, M. salivarium, M. orale, M. gallisepticum also have increased expression of IL-1β and TNF-α (169).
Silva et al. (223) found that U. diversum intrauterine infection in a mouse model induced TNF-α production. U. diversum was also able to induce TNF-α, IL-1β, and IL-6 production in macrophage culture (224). Inoculation of viable or inactive strains and different concentrations of LAMPs induced higher expression of IL-1β, TNF-α, TLR2, and TLR4 gene in bovine macrophages (42). A common feature of these studies was the dose-dependent relationship (even to microbial load or LAMP amount). Thus, as suggested by Marques et al. (68), The higher bacterial load of U. diversum may be directly related to the intensity of the clinical conditions of infected cows.
In infections due to Mollicutes, the signal transduction leading to the production of proinflammatory cytokines indicated is dependent on NF-kB or AP-1 (225). Many studies show that stimulation of TLR1, TLR2, and TLR6 by LAMPs results in the activation of these transcription factors. TLR2 is known to form heterodimers with TLR1 (TLR2/TLR1) and TLR6 (TLR2/TLR6), thereby distinguishing different microbial components, including Mollicutes LAMPs (226–228). LAMPs derived from M. genitalium can activate NF-kB via TLR1, TLR2, and TLR6 (211). The same occurs in THP-1 cells infected with M. pneumoniae (229) and in macrophages infected with U. parvum LAMPs (164, 230). Wang et al. (41) found that overexpression of TLR2 and MyD88 in bovine embryo lung cells depends on the stimulation by M. bovis LAMPs and that these lipoproteins activate IL-1β production via NF-kB via TLR2 and MyD88.
U. diversum also induces high TLR2 expression in murine and bovine macrophages (28, 42). This demonstrates the fundamental role of this receptor in signaling pathways that lead to the production of a proinflammatory cytokine profile. However, studies evaluating the expression of TLR 2 and TLR 4, as well as the use of TLR4, TLR2/4, and NFK-B blockers associated with TLR2 (Pam3CysSK4) and TLR4 (LPS) ligand, have shown that TLR4 has an important function in signaling that leads to the expression of IL-1β and TNF-α. In this case, the TLR4 blockade inhibited the expression of these cytokines, suggesting that U. diversum and its lipoproteins interact with TLR4 in signaling that acts via NF-kB to stimulate the inflammatory response, as shown in Figure 3 (42). However, due to the antigenic variety of U. diversum, it is possible to suggest that other signaling pathways may coexist.
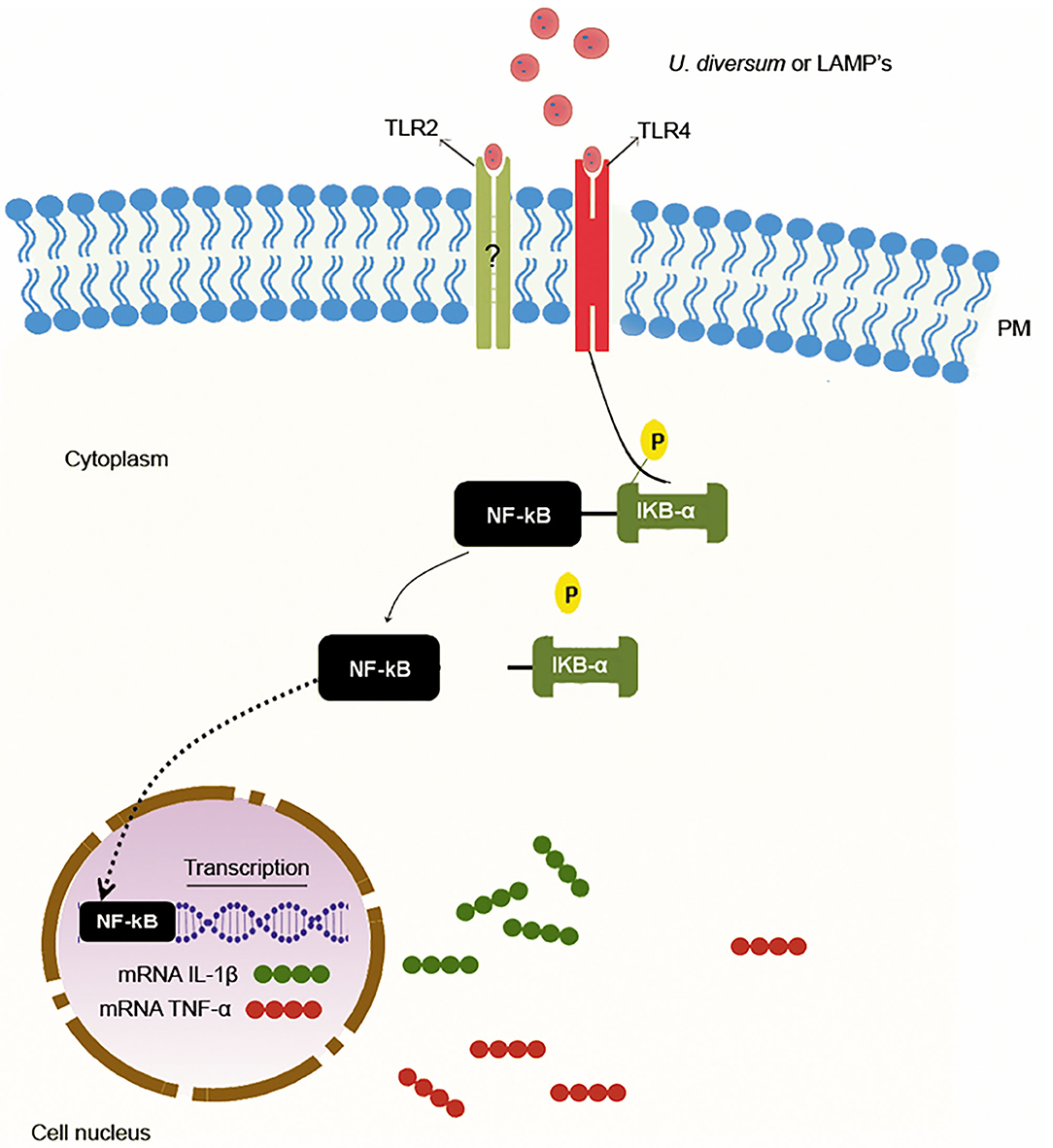
Figure 3. Proinflammatory response induction mechanism by U. diversum. The interaction of U. diversum or LAMPs activates NF-kB. Once activated, NF-kB translocates to the nucleus and acts as a transcription factor for IL-1β and TNF-α.
Other studies have investigated TLR4 signaling in Mollicutes. Shimizu et al. (231) showed that M. pneumoniae induces strong inflammatory responses in TLR2 knockout mouse macrophages and the inhibited response in TLR4 inhibitor treated macrophages suggesting the establishment of TLR4 mediated inflammatory response. Both TLR2 and 4 may be involved in signaling by some Mollicutes. It has been found in Mycoplasma arthritidis that TLR 2 and TLR4 interact with HLA-DR by increasing the binding and antigen presentation of this mollicute to murine T cells (213). Furthermore, U. urealyticum LAMPs interact with TLR-2 and TLR-4 leading to immunomodulation with the release of proinflammatory mediators (212).
Induction of an Anti-inflammatory Profile
LAMPs can also downregulate the immune system. M. fermentans lipoproteins have been shown to induce IL-10 production in human monocytes (232). Combining M. flocculare with M. hyopneumoniae also increases the ability of bone-marrow-derived dendritic cells (BM-DC) to secrete IL-10 (233). IL-10 may act as an anti-inflammatory cytokine necessary to maintain cellular homeostasis or favor the Th2 pathway by inhibiting IL-12 production (31, 234).
Andrade et al. (65) noted that some U. diversum strains induced significant expression of IL-10 and IL-17 when inoculated into bovine macrophages. The expression of IL-17 does not prevent anti-inflammatory modulation. Thus, the high antigen variability contributes to U. diversum modulating the immune system in different ways. This type of signaling is common in some Mollicutes; M. bovis-infected bovine monocytes, with increased IL-10 secretion (235).
Despite the role of IL-17 in neutrophil recruitment, it can also act synergistically with IL-10. Corroborating this theory Jimbo et al. (236) showed that IL-17 does not increase bovine neutrophil survival after M. bovis infection. Thus, the increase in IL-10 and IL-17, reported in U. diversum infected bovine macrophages (65), may be responsible for the extended time of infections since this cytokine prevents the activation of effector mechanisms for pathogen destruction (233, 237).
Perspectives
The economic loss from U. diversum infection is reflected in bovine meat, milk, and semen production and marketing industries. In veterinary research, PCR technology for U. diversum diagnosis is a new paradigm for detecting this infectious agent. The early, rapid diagnosis and control of bovine ureaplasmal infections must be consolidated soon to reduce the costs in keeping cattle healthy.
The sequencing of the U. diversum genome has provided more information about the species, especially regarding the antigenic variation (28, 90). Now CDS for various LAMPs, VSPs, and other antigens are available. An alternative that seems promising for future studies would be to review the heterologous expression of these antigens by recombinant DNA technology, immunomodulation, and antibody production assays.
Studies of U. diversum LAMPs showed their potential to increase the host proinflammatory cytokines (42). However, molecular cloning and expression in heterologous systems of these proteins should provide a better understanding of this ureaplasma virulence. However, the culturing techniques for U. diversum must also be improved, as the high nutritional requirements make laboratory isolation and cultivation difficult. This aspect, added to the high specificity of ureaplasmas to host tissues, also limits studies of pathogen-host interaction. This limitation has been solved in other fastidious microorganisms through the cultures of organoids from target tissues of infection (238). However, use of this technology is not yet a reality for studying mollicutes. The heterologous protein expression in Escherichia coli is already known and this should facilitate more specific studies. The other approach should include the ability to better understand the effects of purified antigens. These technologies have already been applied in other Mollicutes and should help improve understanding of the pathogenesis and virulence of bovine ureaplasma.
Author Contributions
MS, JT, and LM: conceived and designed. MS, NM, GC, BB, JT, and LM: wrote the paper. All authors: read and approved the final manuscript.
Conflict of Interest
The authors declare that the research was conducted in the absence of any commercial or financial relationships that could be construed as a potential conflict of interest.
References
1. Razin S. Molecular biology and genetics of mycoplasmas (Mollicutes). Microbiol Rev. (1985) 49:419–55. doi: 10.1128/MR.49.4.419-455.1985
2. Razin S, Hayflick L. Highlights of mycoplasma research–an historical perspective. Biologicals. (2010) 38:183–90. doi: 10.1016/j.biologicals.2009.11.008
3. Vos P, Garrity G, Jones D, Krieg NR, Ludwig W, Rainey FA, et al. Bergey's Manual of Systematic Bacteriology: Volume 3: The Firmicutes. Heidelberg; Dordrecht; New York City, NY: Springer Science & Business Media (2011). p. 1016–8.
4. Hiruki C, Wang K. Clover proliferation phytoplasma: 'Candidatus Phytoplasma trifolii'. Int J Syst Evol Microbiol. (2004) 54:1349–53. doi: 10.1099/ijs.0.02842-0
5. Lee IM, Gundersen-Rindal DE, Davis RE, Bottner KD, Marcone C, Seemüller E. ‘Candidatus Phytoplasma asteris’, a novel phytoplasma taxon associated with aster yellows and related diseases. Int J Syst Evol Microbiol. (2004) 54:1037–48. doi: 10.1099/ijs.0.02843-0
6. Tran-Nguyen LT, Gibb KS. Extrachromosomal DNA isolated from tomato big bud and Candidatus Phytoplasma australiense phytoplasma strains. Plasmid. (2006) 56:153–66. doi: 10.1016/j.plasmid.2006.05.009
7. Tran-Nguyen LT, Kube M, Schneider B, Reinhardt R, Gibb KS. Comparative genome analysis of “Candidatus Phytoplasma australiense” (subgroup tuf-Australia I; rp-A) and “Ca. Phytoplasma asteris” Strains OY-M and AY-WB. J Bacteriol. (2008) 190:3979–91. doi: 10.1128/JB.01301-07
8. Kumar M, Katiyar A, Madhupriya, Rao GP. First report of association of potato virus X and potato virus Y and 'Candidatus Phytoplasma trifolii' in brinjal in India. Virusdisease. (2016) 27:207–8. doi: 10.1007/s13337-016-0318-8
9. Davis RE, Zhao Y, Wei W, Dally EL, Lee IM. ‘Candidatus Phytoplasma luffae', a novel taxon associated with witches’ broom disease of loofah, Luffa aegyptica Mill. Int J Syst Evol Microbiol. (2017) 67:3127–33. doi: 10.1099/ijsem.0.001980
10. Cardoso MV, Scarcelli E, Grasso LM, Teixeira SR, Genovez ME. Ureaplasma diversum and reproductive disorder in Brazilian cows and heifers; first report. Anim Reprod Sci. (2000) 63:137–43. doi: 10.1016/S0378-4320(00)00176-7
11. Nicholas RA, Ayling RD. Mycoplasma bovis: disease, diagnosis, and control. Res Vet Sci. (2003) 74:105–12. doi: 10.1016/S0034-5288(02)00155-8
12. Buim MR, Mettifogo E, Timenetsky J, Kleven S, Ferreira AJP. Epidemiological survey on Mycoplasma gallisepticum and M. synoviae by multiplex PCR in commercial poultry. Pesquisa Veterinária Brasileira. (2009) 29:552–6. doi: 10.1590/S0100-736X2009000700009
13. Horner P, Thomas B, Gilroy CB, Egger M, Taylor-Robinson D. Role of Mycoplasma genitalium and Ureaplasma urealyticum in acute and chronic nongonococcal urethritis. Clin Infect Dis. (2001) 32:995–1003. doi: 10.1086/319594
14. Waites KB, Talkington DF. Mycoplasma pneumoniae and its role as a human pathogen. Clin Microbiol Rev. (2004) 17:697–728. doi: 10.1128/CMR.17.4.697-728.2004
15. Vuorinen S, Tuuminen T. Mycoplasma hominis may cause soft tissue infections. Duodecim. (2011) 127:2661–5.
16. Chambaud I, Wróblewski H, Blanchard A. Interactions between mycoplasma lipoproteins and the host immune system. Trends Microbiol. (1999) 7:493–9. doi: 10.1016/S0966-842X(99)01641-8
17. Naito M, Morton JB, Pawlowska TE. Minimal genomes of mycoplasma-related endobacteria are plastic and contain host-derived genes for sustained life within Glomeromycota. Proc Natl Acad Sci USA. (2015) 112:7791–6. doi: 10.1073/pnas.1501676112
18. Razin S, Yogev D, Naot Y. Molecular biology and pathogenicity of mycoplasmas. Microbiol Mol Biol Rev. (1998) 62:1094–156. doi: 10.1128/MMBR.62.4.1094-1156.1998
19. Pilo P, Frey J, Vilei EM. Molecular mechanisms of pathogenicity of Mycoplasma mycoides subsp. mycoides SC. Vet J. (2007) 174:513–21. doi: 10.1016/j.tvjl.2006.10.016
20. Razin S. Molecular properties of mollicutes: a synopsis. Mol Diagn Proced Mycoplasmol. (1995) 1:1–25. doi: 10.1016/B978-012583805-4/50003-0
21. Maniloff J. Cytoskeletal elements in mycoplasmas and other prokaryotes. BioSystems. (1981) 14:305–12. doi: 10.1016/0303-2647(81)90037-X
22. García-Morales L, González-González L, Querol E, Piñol J. A minimized motile machinery for Mycoplasma genitalium. Mol Microbiol. (2016) 100:125–38. doi: 10.1111/mmi.13305
23. Seto S, Layh-Schmitt G, Kenri T, Miyata M. Visualization of the attachment organelle and cytadherence proteins of Mycoplasma pneumoniae by immunofluorescence microscopy. Bacteriol J. (2001) 183:1621–30. doi: 10.1128/JB.183.5.1621-1630.2001
24. Olson LD, Renshaw CA, Rottem S, Boal JH. Arginine utilization by Mycoplasma fermentans is not regulated by glucose metabolism: a 13C-NMR study. FEMS Microbiol Lett. (1993) 108:47–51. doi: 10.1111/j.1574-6968.1993.tb06072.x
25. Afshar B, Nicholas RA, Pitcher D, Fielder MD, Miles RJ. Biochemical and genetic variation in Mycoplasma fermentans strains from cell line, human and animal sources. J Appl Microbiol. (2009) 107:498–505. doi: 10.1111/j.1365-2672.2009.04224.x
26. Pollack JD. Central carbohydrate pathways: metabolic flexibility and the extra role of some “housekeeping” enzymes. In: Molecular Biology and Pathogenicity of Mycoplasmas. Springer (2002). p. 163–99. doi: 10.1007/0-306-47606-1_8
27. Miles R, Taylor R, Abu-Groun E, Alimohammadi A. Diversity of energy-yielding metabolism in Mycoplasma spp. IOM Lett. (1994) 3:165–6.
28. Marques LM, Rezende IS, Barbosa MS, Guimaraes AM, Martins HB, Campos GB. Ureaplasma diversum genome provides new insights about the interaction of the surface molecules of this bacterium with the host. PLoS ONE. (2016) 11:e0161926. doi: 10.1371/journal.pone.0161926
29. Baseman JB, Tully JG. Mycoplasmas: sophisticated, reemerging, and burdened by their notoriety. Emerging Infect Dis. (1997) 3:21–32. doi: 10.3201/eid0301.970103
30. Fraser CM, Gocayne JD, White O, Adams MD, Clayton RA, Fleischmann RD, et al. The minimal gene complement of Mycoplasma genitalium. Science. (1995) 270:397–403. doi: 10.1126/science.270.5235.397
31. Rottem S. Interaction of mycoplasmas with host cells. Physiol Rev. (2003) 83:417–32. doi: 10.1152/physrev.00030.2002
32. Pich OQ, Burgos R, Planell R, Querol E, Piñol J. Comparative analysis of antibiotic resistance gene markers in Mycoplasma genitalium: application to studies of the minimal gene complement. Microbiology. (2006) 152:519–27. doi: 10.1099/mic.0.28287-0
33. Borges CL, Parente JA, Pereira M, Soares CMdA. Identification of the GTPase superfamily in Mycoplasma synoviae and Mycoplasma hyopneumoniae. Genet Mol Biol. (2007) 30:212–8. doi: 10.1590/S1415-47572007000200007
34. Pilo P, Vilei EM, Peterhans E, Bonvin-Klotz L, Stoffel MH, Dobbelaere D, et al. A metabolic enzyme as a primary virulence factor of Mycoplasma mycoides subsp. mycoides small colony. J Bacteriol. (2005) 187:6824–31. doi: 10.1128/JB.187.19.6824-6831.2005
35. Ramírez AS, Fleitas JL, Rosales RS, Poveda C, de la Fe C, Andrada M, et al. A semi-defined medium without serum for small ruminant mycoplasmas. Vet J. (2008) 178:149–52. doi: 10.1016/j.tvjl.2007.07.023
36. Taylor-Robinson D, Jensen JS. Mycoplasma genitalium: from Chrysalis to multicolored butterfly. Clin Microbiol Rev. (2011) 24:498–514. doi: 10.1128/CMR.00006-11
37. Kletzel HH, Rotem R, Barg M, Michaeli J, Reichman O. Ureaplasma urealyticum: the role as a pathogen in women's health, a systematic review. Curr Infect Dis Rep. (2018) 20:33. doi: 10.1007/s11908-018-0640-y
38. Haapala V, Pohjanvirta T, Vähänikkilä N, Halkilahti J, Simonen H, Pelkonen S, et al. Semen as a source of Mycoplasma bovis mastitis in dairy herds. Vet Microbiol. (2018) 216:60–66 doi: 10.1016/j.vetmic.2018.02.005
39. Yang J, Hooper WC, Phillips DJ, Talkington DF. Cytokines in Mycoplasma pneumoniae infections. Cytokine Growth Factor Rev. (2004) 15:157–68. doi: 10.1016/j.cytogfr.2004.01.001
40. Maunsell FP, Chase C. Mycoplasma bovis: interactions with the immune system and failure to generate an effective immune response. Vet Clin Food Anim Pract. (2019) 35:471–83. doi: 10.1016/j.cvfa.2019.08.003
41. Wang Y, Liu S, Li Y, Wang Q, Shao J, Chen Y, et al. Mycoplasma bovis-derived lipid-associated membrane proteins activate IL-1β production through the NF-κB pathway via toll-like receptor 2 and MyD88. Dev Comp Immunol. (2016) 55:111–8. doi: 10.1016/j.dci.2015.10.017
42. Santos-Junior MN, Rezende IS, Souza CLS, Barbosa MS, Campos GB, Brito LF. Ureaplasma diversum and its membrane-associated lipoproteins activate inflammatory genes through the NF-κB pathway via Toll-Like receptor 4. Front Microbiol. (2018) 9:1538. doi: 10.3389/fmicb.2018.01538
43. Yu Y, Chen Y, Wang Y, Li Y, Zhang L, Xin J. TLR2/MyD88/NF-κB signaling pathway regulates IL-1β production in DF-1-cells exposed to Mycoplasma gallisepticum LAMPs. Microb Pathog. (2018) 117:225–31. doi: 10.1016/j.micpath.2018.02.037
44. Wawegama NK, Browning GF. Improvements in diagnosis of disease caused by Mycoplasma bovis in cattle. Anim Product Sci. (2017) 57:1482–7. doi: 10.1071/AN16490
45. Reji RM, Mini M, Mani BK, Sankar S, Thirupathy V, Reshma PS, et al. Isolation and identification of Mycoplasma agalactiae associated with respiratory tract infection in goats: a case study. Pharma Innovat J. (2018) 7:21–3.
46. Leal Zimmer FMDA, Paludo GP, Moura H, Barr JR, Ferreira HB. Differential secretome profiling of a swine tracheal cell line infected with mycoplasmas of the swine respiratory tract. Proteomics J. (2019) 192:147–59. doi: 10.1016/j.jprot.2018.08.018
47. Crane MB, Hughes CA. Can Ureaplasma diversum be transmitted from donor to recipient through the embryo? Two case reports outlining U. diversum losses in bovine embryo pregnancies. Canad Vet J. (2018) 59:43.
48. Díaz JM, Prieto A, López G, Díaz P, López C, Quintela L, et al. (2019) Association of Ureaplasma diversum with reproductive disease in cattle. N Z Vet J. 67:249–56. doi: 10.1080/00480169.2019.1623733
49. Taylor-Robinson D, Haig D, Williams M. Bovine T-strain mycoplasma. Ann N Y Acad Sci. (1967) 143:517–8. doi: 10.1111/j.1749-6632.1967.tb27697.x
50. Shepard MC. The recovery of pleuropneumonia-like organisms from Negro men with and without nongonococcal urethritis. Am J Syph Gonorrhea Vener Dis. (1954) 38:113–24.
51. Shepard MC, Lunceford C, Ford D, Purcell R, Taylor-Robinson D, Razin S, et al. Ureaplasma urealyticum gen. nov., sp. nov.: proposed nomenclature for the human T (T-strain) mycoplasmas. Int J Syst Evolut Microbiol. (1974) 24:160–71. doi: 10.1099/00207713-24-2-160
52. Howard C, Gourlay R, Garwes D, Pocock D, Collins J. Base composition of deoxyribonucleic acid from bovine T-mycoplasmas. Int J Syst Evol Microbiol. (1974) 24:373–4. doi: 10.1099/00207713-24-3-373
53. Howard C, Pocock D. Comparison of ureaplasmas from sheep and goats with Ureaplasma diversum and U. urealyticum. Microbiology. (1983) 129:3197–202. doi: 10.1099/00221287-129-10-3197
54. Howard C, Gourlay R. Proposal for a second species within the genus Ureaplasma, Ureaplasma diversum sp. nov. Int J Syst Evolut Microbiol. (1982) 32:446–52. doi: 10.1099/00207713-32-4-446
55. Black FT, Christiansen C, Askaa G. Genome size and base composition of deoxyribonucleic acid from eight human T-mycoplasmas. Int J Syst Evol Microbiol. (1972) 22:241–2. doi: 10.1099/00207713-22-4-241
56. Koshimizu K, Kotani H, Yamamoto K, Magaribuchi T, Harasawa R, Ito M, et al. Serological analysis of Ureaplasmas isolated from various animals. Isr J Med Sci. (1984) 20:950–3.
57. Howard C, Gourlay R, Collins J. Serological comparison between twenty-five bovine Ureaplasma (T-mycoplasma) strains by immunofluorescence. Int J Syst Evol Microbiol. (1975) 25:155–9. doi: 10.1099/00207713-25-2-155
58. Ogata M, Kotani H, Yamamoto K. Serological comparison of bovine ureaplasmas. Nippon Juigaku Zasshi. (1979) 41:629–37. doi: 10.1292/jvms1939.41.629
59. Howard C, Gourlay R, Collins J. Serological studies with bovine ureaplasmas (T-mycoplasmas). Int J Syst Evol Microbiol. (1978) 28:473–7. doi: 10.1099/00207713-28-4-473
60. Howard CJ, Gourlay RN. Identification of Ureaplasmas from cattle using antisera prepared in gnotobiotic calves. Microbiology. (1981) 126:365–9. doi: 10.1099/00221287-126-2-365
61. Truscott RB. Ureaplasma serotypes associated with the bovine urogenital tract. Can J Comp Med. (1983) 47:471–3.
62. Mulira GL, Saunders JR, Barth AD. Isolation of Ureaplasma diversum and mycoplasmas from genital tracts of beef and dairy cattle in Saskatchewan. Can Vet J. (1992) 33:46–9.
63. Howard C. Mycoplasmas and bovine respiratory disease: studies related to pathogenicity and the immune response–a selective review. Yale J Biol Med. (1983) 56:789.
64. Marques LM, Buzinhani M, Guimaraes AM, Marques RC, Farias ST, Neto RL, et al. Intraspecific sequence variation in 16S rRNA gene of Ureaplasma diversum isolates. Vet Microbiol. (2011) 152:205–11. doi: 10.1016/j.vetmic.2011.04.007
65. Andrade YM, Santos-Junior MN, Rezende IS, Barbosa MS, Amorim AT, Silva ÍB. Multilocus sequence typing characterizes diversity of Ureaplasma diversum strains, and intra-species variability induces different immune response profiles. BMC Vet Res. (2020) 16:163. doi: 10.1186/s12917-020-02380-w
66. Santos-Junior MN, Santos RS, Neves WS, Fernandes JM, de Brito Guimarães BC, Barbosa MS, et al. Immunoinformatics and analysis of antigen distribution of Ureaplasma diversum strains isolated from different Brazilian states. BMC Vet Res. (2020) 16:379. doi: 10.1186/s12917-020-02602-1
67. Dando SJ, Sweeney EL, Knox CL. Ureaplasma. In: Trujillo ME, Dedysh S, DeVos P, Hedlund B, Kämpfer P, Rainey FA, Whitman WB, editors. Bergey's Manual of Systematics of Archaea and Bacteria. Hoboken, NJ: John Wiley & Sons, Inc. (2019). p. 1–28. doi: 10.1002/9781118960608.gbm01264.pub3
68. Marques LM, Amorim AT, Martins HB, Rezende IS, Barbosa MS, Lobao TN. A quantitative TaqMan PCR assay for the detection of Ureaplasma diversum. Vet Microbiol. (2013) 167:670–4. doi: 10.1016/j.vetmic.2013.07.031
69. Paralanov V, Lu J, Duffy LB, Crabb DM, Shrivastava S, Methé BA, et al. Comparative genome analysis of 19 Ureaplasma urealyticum and Ureaplasma parvum strains. BMC Microbiol. (2012) 12:88. doi: 10.1186/1471-2180-12-88
70. Argue B, Chousalkar KK, Chenoweth PJ. Presence of Ureaplasma diversum in the Australian cattle population. Aust Vet J. (2013) 91:99–101. doi: 10.1111/avj.12009
71. Lobo E, Ramírez A, Poveda J. Detección de Ureaplasma spp. en pulmones de cerdos, con lesiones compatibles a la neumonía enzoótica porcina, procedentes de la región occidental y central de Cuba. Revista de Salud Animal. (2013) 35:69–71.
72. Burgher Y, Miranda L, Rodriguez-Roche R, de Almeida Campos AC, Lobo E, Neves T, et al. Ureaplasma diversum in pneumonic lungs of swine. Infect Genet Evol. (2014) 21:486–8. doi: 10.1016/j.meegid.2013.07.003
73. Azevedo JB, Silva GS, Rocha PS, Pitchenin LC, Dutra V, Nakazato L, et al. Presence of Ureaplasma diversum in the genital tracts of female dairy cattle in Mato Grosso State, Brazil. Trop Anim Health Prod. (2017) 49:311–6. doi: 10.1007/s11250-016-1194-3
74. Sosa C, Tirante L, Chaves J, Pol M, Ambrogi A, Giraudo JA, et al. Identificación de especies de Mycoplasma y de Ureaplasma diversum en rodeos lecheros de Argentina. Revista Argentina de Microbiol. (2018) 50:31–5. doi: 10.1016/j.ram.2017.02.010
75. Howard C, Gourlay R, Brownlie J. The virulence of T-mycoplasmas, isolated from various animal species, assayed by intramammary inoculation in cattle. J Hygiene. (1973) 71:163–70. doi: 10.1017/S0022172400046337
76. Rae DO, Chenoweth PJ, Brown MB, Genho PC, Moore SA, Jacobsen KE. Reproductive performance of beef heifers: effects of vulvo-vaginitis, Ureaplasma diversum and prebreeding antibiotic administration. Theriogenology. (1993) 40:497–508. doi: 10.1016/0093-691X(93)90403-R
77. Petit T, Spergser J, Aurich J, Rosengarten R. Prevalence of Chlamydiaceae and Mollicutes on the genital mucosa and serological findings in dairy cattle. Vet Microbiol. (2008) 127:325–33. doi: 10.1016/j.vetmic.2007.08.022
78. León BA, Campos E, Bolaños H, Caballero M. Risk factors for Ureaplasma diversum infections in cattle of a tropical environment. Rev Biol Trop. (1995) 43:21–5.
79. Cardoso MV, Blanchard A, Ferris S, Verlengia R, Timenetsky J, Florio Da Cunha RA. Detection of Ureaplasma diversum in cattle using a newly developed PCR-based detection assay. Vet Microbiol. (2000) 72:241–50. doi: 10.1016/S0378-1135(99)00203-5
80. Britton AP, Ruhnke HL, Miller RB, Johnson WH, Leslie KE, Rosendal S. In vitro exposure of bovine morulae to Ureaplasma diversum. Can J Vet Res. (1987) 51:198–203.
81. Miller R, Chelmonska-Soyta A, Smits B, Foster R, Rosendal S. Ureaplasma diversum as a cause of reproductive disease in cattle. Vet Clin North Am Food Anim Pract. (1994) 10:479–90. doi: 10.1016/S0749-0720(15)30533-8
82. Doig PA, Ruhnke HL, Palmer NC. Experimental bovine genital ureaplasmosis. I. Granular vulvitis following vulvar inoculation. Can J Comp Med. (1980) 44:252–58.
83. Doig PA, Ruhnke HL, Palmer NC. Experimental bovine genital ureaplasmosis. II. Granular vulvitis, endometritis and salpingitis following uterine inoculation. Can J Comp Med. (1980) 44:259–66.
84. Ball HJ, Mackie DP. The ovine mammary gland as an experimental model to determine the virulence of animal ureaplasmas. J Hyg. (1985) 95:375–82. doi: 10.1017/S0022172400062793
85. Pôrto R, Oliveira B, Ferraz H, Caixeta L, Viu MA, Gambarini ML. Histopatology of the reproductive tract of Nellore pubertal heifers with genital ureaplasmosis. An Acad Bras Cienc. (2017) 89:2987–96. doi: 10.1590/0001-3765201720160803
86. Buzinhani M, Metiffogo E, Timenetsky J. Detecção de Mycoplasma spp. e Ureaplasma diversum em vacas com distúrbios reprodutivos Detection of Mycoplasma spp. and Ureaplasma diversum in cown with reproductive disorders. Arquivo Brasileiro de Medicina Veterinária e Zootecnia. (2007) 59:1368–75. doi: 10.1590/S0102-09352007000600003
87. Gaeti JG, Lana MV, Silva GS, Lerner L, de Campos CG, Haruni F, et al. Ureaplasma diversum as a cause of pustular vulvovaginitis in bovine females in Vale Guapore, Mato Grosso State, Brazil. Trop Anim Health Prod. (2014) 46:1059–63. doi: 10.1007/s11250-014-0614-5
88. Buzinhani M, Yamaguti M, Oliveira RC, Cortez BA, Marques LM, Machado-Santelli GM, et al. Invasion of Ureaplasma diversum in bovine spermatozoids. BMC Res Notes. (2011) 4:455. doi: 10.1186/1756-0500-4-455
89. Amorim AT, Marques LM, Santos AM, Martins HB, Barbosa MS, Rezende IS. Apoptosis in HEp-2 cells infected with Ureaplasma diversum. Biol Res. (2014) 47:38. doi: 10.1186/0717-6287-47-38
90. Marques LM, Guimaraes AM, Martins HB, Rezende IS, Barbosa MS, Campos GB. Genome sequence of Ureaplasma diversum strain ATCC 49782. Genome Announc. (2015) 3:e00314-15. doi: 10.1128/genomeA.00314-15
91. Eaglesome M, Garcia M, Stewart R. Microbial agents associated with bovine genital tract infections and semen. Part II. Haemophilus somnus, Mycoplasma spp and Ureaplasma spp, Chlamydia; pathogens and semen contaminants; treatments of bull semen with antimicrobial agents. Vet Bull. (1992) 62:887–910.
92. Barkallah M, Gharbi Y, Hassena AB, Slima AB, Mallek Z, Gautier M, et al. Survey of infectious etiologies of bovine abortion during mid- to late gestation in dairy herds. PLoS ONE. (2014) 9:e91549. doi: 10.1371/journal.pone.0091549
93. Gale SP. The effects of two Ureaplasma diversum strains on early pregnancy in heifers. Can J Vet Res. (1987) 51:536–8.
94. Lingwood CA, Quinn PA, Wilansky S, Nutikka A, Ruhnke HL, Miller RB. Common sulfoglycolipid receptor for mycoplasmas involved in animal and human infertility. Biol Reprod. (1990) 43:694–7. doi: 10.1095/biolreprod43.4.694
95. Kim JJ, Quinn PA, Fortier MA. Ureaplasma diversum infection in vitro alters prostaglandin E2 and prostaglandin F2a production by bovine endometrial cells without affecting cell viability. Infect Immun. (1994) 62:1528–33. doi: 10.1128/IAI.62.5.1528-1533.1994
96. Prince AL, Ma J, Kannan PS, Alvarez M, Gisslen T, Harris RA. The placental membrane microbiome is altered among subjects with spontaneous preterm birth with and without chorioamnionitis. Am J Obstet Gynecol. (2016) 214:627.e1–16. doi: 10.1016/j.ajog.2016.01.193
97. Oliveira CNT, Oliveira MTS, Oliveira HBM, Silva LSC, Freire RS, Júnior MS, et al. Association of spontaneous abortion and Ureaplasma parvum detected in placental tissue. Epidemiol Infect. (2020) 148:e126. doi: 10.1017/S0950268820001302
98. Howard CJ, Gourlay RN, Thomas LH, Stott EJ. Induction of pneumonia in gnotobiotic calves following inoculation of Mycoplasma dispar and ureaplasmas (T-mycoplasmas). Res Vet Sci. (1976) 21:227–31. doi: 10.1016/S0034-5288(18)33377-0
99. Ruhnke HL, Palmer NC, Doig PA, Miller RB. Bovine abortion and neonatal death associated with Ureaplasma diversum. Theriogenology. (1984) 21:295–301. doi: 10.1016/0093-691X(84)90415-1
100. Ter Laak EA, Noordergraaf JH, Dieltjes RP. Prevalence of mycoplasmas in the respiratory tracts of pneumonic calves. Zentralblatt Veterinarmedizin Reihe B. (1992) 39:553–62. doi: 10.1111/j.1439-0450.1992.tb01205.x
101. Smith A, Chousalkar KK, Chenoweth PC. Polymerase chain reaction for detection of Ureaplasma diversum from urogenital swabs in cattle in Australia. Aust Vet J. (2012) 90:275–6. doi: 10.1111/j.1751-0813.2012.00939.x
102. Hobson N, Chousalkar KK, Chenoweth PJ. Ureaplasma diversum in bull semen in Australia: its detection and potential effects. Aust Vet J. (2013) 91:469–73. doi: 10.1111/avj.12113
103. Nascimento-Rocha JM, Oliveira Filho BD, Arnhold E, Porto RN, Lima SF, Gambarini ML. Assessment of cow and farm level risk factors associated with Ureaplasma diversum in pasture-based dairy systems-A field study. Anais da Academia Brasileira de Ciências. (2017) 89:1779–83. doi: 10.1590/0001-3765201720160813
104. Marques LM, Buzinhani M, Oliveira RC, Yamaguti M, Ferreira JB, Neto RL, et al. Prevalence of mycoplasmas in the respiratory tracts of calves in Brazil. Vet Rec. (2007) 161:699–700. doi: 10.1136/vr.161.20.699
105. Voltarelli DC, de Alcântara BK, Lunardi M, Alfieri AF, de Arruda Leme R, Alfieri AA. A nested-PCR strategy for molecular diagnosis of mollicutes in uncultured biological samples from cows with vulvovaginitis. Anim Reprod Sci. (2018) 188:137–43. doi: 10.1016/j.anireprosci.2017.11.018
106. Thomas A, Ball H, Dizier I, Trolin A, Bell C, Mainil J, et al. Isolation of mycoplasma species from the lower respiratory tract of healthy cattle and cattle with respiratory disease in Belgium. Vet Rec. (2002) 151:472–6. doi: 10.1136/vr.151.16.472
107. Gagea MI, Bateman KG, van Dreumel T, McEwen BJ, Carman S, Archambault M, et al. Diseases and pathogens associated with mortality in Ontario beef feedlots. J Vet Diagn Invest. (2006) 18:18–28. doi: 10.1177/104063870601800104
108. Burgher Y, Koszegi M, St-Sauveur VG, Fournier D, Lafond-Lambert C, Provost C, et al. Canada: first report of Ureaplasma diversum, a bovine pathogen, in the respiratory tract of swine in Canada. Can Vet J. (2018) 59:1333–7.
109. Syrjälä P, Anttila M, Dillard K, Fossi M, Collin K, Nylund M, et al. Causes of bovine abortion, stillbirth and neonatal death in Finland 1999–2006. Acta Vet Scand. (2007) 49:5186. doi: 10.1186/1751-0147-49-S1-S3
110. Szacawa E, Jawor P, Dudek K, Bednarek D, Stefaniak T. Screening for Mollicutes microorganisms in perinatal calf mortality cases in Polish dairy herds. Pol J Vet Sci. (2018) 21:441–4. doi: 10.24425/122616
111. Muenster OA, Ose EE, Matsuoka T. The incidence of Mycoplasma dispar, Ureaplasma and conventional Mycoplasma in the pneumonic calf lung. Can J Comp Med. (1979) 43:392–8.
112. Anderson ML. Infectious causes of bovine abortion during mid- to late-gestation. Theriogenology. (2007) 68:474–86. doi: 10.1016/j.theriogenology.2007.04.001
113. Britton AP, Miller RB, Ruhnke HL, Johnson WH. The recovery of ureaplasmas from bovine embryos following in vitro exposure and ten washes. Theriogenology. (1988) 30:997–1003. doi: 10.1016/S0093-691X(88)80062-1
114. Nicholas RA, Fox LK, Lysnyansky I. Mycoplasma mastitis in cattle: to cull or not to cull. Vet J. (2016) 216:142–7. doi: 10.1016/j.tvjl.2016.08.001
115. Gourlay RN, Howard CJ, Brownlie J. The production of mastitis in cows by the intramammary inoculation of T-mycoplasmas. J Hyg. (1972) 70:511–21. doi: 10.1017/S0022172400063099
116. Fox LK, Hancock DD, Mickelson A, Britten A, Kaaden OR. Bulk tank milk analysis: factors associated with appearance of Mycoplasma sp. in milk. J Vet Med Series B. (2003) 50:235–40. doi: 10.1046/j.1439-0450.2003.00668.x
117. González RN, Wilson DJ. Mycoplasmal mastitis in dairy herds. Vet Clin Food Anim Pract. (2003) 19:199–221. doi: 10.1016/S0749-0720(02)00076-2
118. Fish NA, Rosendal S, Miller RB. The distribution of mycoplasmas and ureaplasmas in the genital tract of normal artificial insemination bulls. Can Vet J. (1985) 26:13–5.
120. Ball HJ, Logan EF, Orr W. Isolation of mycoplasmas from bovine semen in Northern Ireland. Vet Rec. (1987) 121:322–4. doi: 10.1136/vr.121.14.322
121. Le Grand D, Poumarat F, Martel J. Infectious genital disease by Ureaplasma diversum: investigations on bovine in France [granular vulvitis; dot immunobinding]. Vet Res. (1995) 26:11–20.
122. Givens MD. Review: risks of disease transmission through semen in cattle. Animal. (2018) 12:s165–71. doi: 10.1017/S1751731118000708
123. Thibier M, Guerin B. Hygienic aspects of storage and use of semen for artificial insemination. Anim Reprod Sci. (2000) 62:233–51. doi: 10.1016/S0378-4320(00)00161-5
124. Bielanski A. Disinfection procedures for controlling microorganisms in the semen and embryos of humans and farm animals. Theriogenology. (2007) 68:1–22. doi: 10.1016/j.theriogenology.2007.03.025
125. Stringfellow D, Givens M. Preventing disease transmission through the transfer of in-vivo-derived bovine embryos. Livestock Product Sci. (2000) 62:237–51. doi: 10.1016/S0301-6226(99)00160-8
126. Stringfellow DA, Givens MD. Epidemiologic concerns relative to in vivo and in vitro production of livestock embryos. Anim Reprod Sci. (2000) 60–61:629–42. doi: 10.1016/S0378-4320(00)00104-4
127. Zhou H, McKiernan SH, Ji W, Bavister BD. Effect of antibiotics on development in vitro of hamster pronucleate ova. Theriogenology. (2000) 54:999–1006. doi: 10.1016/S0093-691X(00)00408-8
128. Stringfellow DA, Seidel SM. Manual of International Embryo Transfer Society. 3rd ed. Savoy, IL: International Embryo Transfer Society, Inc. (1998). p. 167–70.
129. D'Angelo M, Visintin JA, Richtzenhain LJ, Gonçalves RF. Evaluation of trypsin treatment on the inactivation of bovine herpesvirus Type 1 on in vitro produced pre-implantation embryos. Reproduct Domestic Anim. (2009) 44:536–9. doi: 10.1111/j.1439-0531.2008.01110.x
130. Thatcher WW, Moreira F, Santos JE, Mattos RC, Lopes FL, Pancarci SM, et al. Effects of hormonal treatments on reproductive performance and embryo production. Theriogenology. (2001) 55:75–89. doi: 10.1016/S0093-691X(00)00447-7
131. Hasler JF. Forty years of embryo transfer in cattle: a review focusing on the journal Theriogenology, the growth of the industry in North America, personal reminisces. Theriogenology. (2014) 81:152–69. doi: 10.1016/j.theriogenology.2013.09.010
132. Cassell GH, Waites KB, Watson HL, Crouse DT, Harasawa R. Infecção intrauterina por Ureaplasma urealyticum: papel na prematuridade e doença em recém-nascidos. Revisões de Microbiol Clín. (1993) 6:69–87. doi: 10.1128/CMR.6.1.69-87.1993
133. Sweeney EL, Kallapur SG, Gisslen T, Lambers DS, Chougnet CA, Stephenson A, et al. Placental infection with Ureaplasma species is associated with histologic chorioamnionitis and adverse outcomes in moderately preterm and late-preterm infants. J Infect Dis. (2016) 213:1340–7. doi: 10.1093/infdis/jiv587
134. Sweeney EL, Dando SJ, Kallapur SG, Knox CL. The human Ureaplasma species as causative agents of chorioamnionitis. Clin Microbiol Rev. (2017) 30:349–79. doi: 10.1128/CMR.00091-16
135. Chernov VM, Chernova OA, Mouzykantov AA, Ponomareva AA, Trushin MV, Gorshkov OV, et al. Phytopathogenicity of avian mycoplasma Mycoplasma gallisepticum S6: morphologic and ultracytostructural changes in plants infected with the vegetative forms and the viable but nonculturable forms of the bacterium. Microbiol Res. (2010) 165:346–50. doi: 10.1016/j.micres.2009.07.001
136. Spergser J, Macher K, Kargl M, Lysnyansky I, Rosengarten R. Emergence, re-emergence, spread and host species crossing of Mycoplasma bovis in the Austrian Alps caused by a single endemic strain. Vet Microbiol. (2013) 164:299–306. doi: 10.1016/j.vetmic.2013.02.007
137. Stock LA, Carneiro AV, Carvalho GR, Zoccal R, Martins PC, Yamaguchi LCT. Sistemas de produção e sua representatividade na produção de leite no Brasil. In: Reunião da Associação Latino-americana de Produção Animal, Cuzco. Anais: ALPA (2008). p. 17–8.
139. Portela V, Diel MI, Mumbach G, Brand SI, Silva DR, Dill RP. Caracterização preliminar do manejo nutricional de bovinos de leite no noroeste do rio grande do sul. Revista Interdisciplinar de ensino, pesquisa e extensão. (2015) 2:1–8.
140. Tupy O, Esteves SN, Túllio RR, Ferreira RdP. O potencial sustentável da bovinocultura de corte do Brasil. Revista de Política Agrícola. (2015) 24:46–54.
141. Pereira LC, Mateus RG, Carvalho CME, Mateus RG, Silva DCG, Borges OA. Avaliação da viabilidade para produção de Bezerro de corte a partir dos centros de custo. REPAE-Revista de Ensino e Pesquisa em Administração e Engenharia. (2016) 1:187–209.
142. Schmidt M. Perinatal death associated with ET, IVP and cloning in cattle. Acta Vet Scand. (2007) 49:1. doi: 10.1186/1751-0147-49-S1-S13
143. Del Fava C, Arcaro J, Pozzi C, Arcaro Júnior I, Fagundes H, Pituco E, et al. Manejo sanitário para o controle de doenças da reprodução em um sistema leiteiro de produção semintensivo. Arquivos do Instituto Biolo. (2003) 70:25–33.
144. Tavares IK, Magalhães LF, de Oliveira FC, Lima GP, Magalhães GM. A relação da evolução das técnicas de criação e o advento das biotecnologias da reprodução com a incidência de distocias em bovinos. Investigação. (2015) 14:91–7. doi: 10.26843/investigacao.v14i1.842
145. Hirose K, Kobayashi H, Ito N, Kawasaki Y, Zako M, Kotani K, et al. Isolation of Mycoplasmas from nasal swabs of calves affected with respiratory diseases and antimicrobial susceptibility of their isolates. J Vet Med B Infect Dis Vet Public Health. (2003) 50:347–51. doi: 10.1046/j.1439-0450.2003.00681.x
146. Kusiluka LJ, Kokotovic B, Ojeniyi B, Friis NF, Ahrens P. Genetic variations among Mycoplasma bovis strains isolated from Danish cattle. FEMS Microbiol. Lett. (2000) 192:113–8. doi: 10.1111/j.1574-6968.2000.tb09368.x
147. Gambarini ML, Kunz TL, Oliveira Filho BD, Porto RN, Oliveira CM, Brito WM, et al. Granular vulvovaginitis syndrome in Nelore pubertal and post pubertal replacement heifers under tropical conditions: role of Mycoplasma spp., Ureaplasma diversum and BHV-1. Trop Anim. Health Prod. (2009) 41:1421–6. doi: 10.1007/s11250-009-9330-y
148. Shepard MC, Lunceford CD. Differential agar medium (A7) for identification of Ureaplasma urealyticum (human T mycoplasmas) in primary cultures of clinical material. J Clin Microbiol. (1976) 3:613–25.
149. Kishimoto M, Tsuchiaka S, Rahpaya SS, Hasebe A, Otsu K, Sugimura S, et al. Development of a one-run real-time PCR detection system for pathogens associated with bovine respiratory disease complex. J Vet Med Sci. (2017) 79:517–23. doi: 10.1292/jvms.16-0489
150. Malafaia GC, Biscola PHN, Dias FRT. A produtividade do rebanho bovino brasileiro em carcaças aumentou 80% em 20 anos. Brasilia: Embrapa Gado de Corte-Fôlder/Folheto/Cartilha (INFOTECA-E) (2020). p. 1–2.
151. Vanyushkina AA, Fisunov GY, Gorbachev AY, Kamashev DE, Govorun VM. Metabolomic analysis of three Mollicute species. PLoS ONE. (2014) 9:e89312. doi: 10.1371/journal.pone.0089312
153. Kokkayil P, Dhawan B. Ureaplasma: current perspectives. Indian J Med Microbiol. (2015) 33:205–14. doi: 10.4103/0255-0857.154850
154. Biernat-Sudolska M, Bilska-Wilkosz A, Rojek-Zakrzewska D, Zawilińska B, Kosz-Vnenchak M. Evaluation of urease activity by the human Ureaplasma species. Folia Biol. (2017) 65:142–7. doi: 10.3409/fb65_3.143
155. Ajello F, Romano N, Massenti MF. L-histidine ammonia-lyase from a T-strain Mycoplasma (Ureaplasma urealyticum). Bollettino dell'Istituto Sieroterapico Milanese. (1977) 56:343–50.
156. Smith DG, Russell WC, Thirkell D. Adherence of Ureaplasma urealyticum to human epithelial cells. Microbiology. (1994) 140:2893–8. doi: 10.1099/00221287-140-10-2893
157. Konieczna I, Kwinkowski M, Kolesińska B, Kamiński Z, Zarnowiec P, Kaca W. Detection of antibodies against synthetic peptides mimicking ureases fragments in sera of rheumatoid arthritis patients. Protein Pept Lett. (2012) 19:1149–54. doi: 10.2174/092986612803217123
158. Viscardi RM, Kallapur SG. Role of Ureaplasma respiratory tract colonization in bronchopulmonary dysplasia pathogenesis: current concepts and update. Clin Perinatol. (2015) 42:719–38. doi: 10.1016/j.clp.2015.08.003
159. Ulbrich S, Schulke K, Groebner A, Reichenbach H.-D., Angioni C, et al. Quantitative characterization of prostaglandins in the uterus of early pregnant cattle. Reproduction. (2009) 138:371–82. doi: 10.1530/REP-09-0081
160. De Silva NS, Quinn PA. Localization of endogenous activity of phospholipases A and C in Ureaplasma urealyticum. J Clin Microbiol. (1991) 29:1498–503. doi: 10.1128/JCM.29.7.1498-1503.1991
161. Feng L, Ransom CE, Nazzal MK, Allen TK, Li YJ, Truong T, et al. The role of progesterone and a novel progesterone receptor, progesterone receptor membrane component 1, in the inflammatory response of fetal membranes to Ureaplasma parvum infection. PLoS ONE. (2016) 11:e0168102. doi: 10.1371/journal.pone.0168102
162. Kacerovsky M, Celec P, Vlkova B, Skogstrand K, Hougaard DM, Cobo T, et al. Amniotic fluid protein profiles of intraamniotic inflammatory response to Ureaplasma spp. and other bacteria. PLoS ONE. (2013) 8:e60399. doi: 10.1371/journal.pone.0060399
163. Razin S, Argaman M, Avigan J. Chemical composition of mycoplasma cells and membranes. J Gen Microbiol. (1963) 33:477–87. doi: 10.1099/00221287-33-3-477
164. Huang X, Qiao Y, Zhou Y, Ruan Z, Kong Y, Li G, et al. Ureaplasma spp. lipid-associated membrane proteins induce human monocyte U937 cell cycle arrest through p53-independent p21 pathway. Int J Med Microbiol. (2018) 308:819–28. doi: 10.1016/j.ijmm.2018.07.005
165. You XX, Zeng YH, Wu YM. Interactions between mycoplasma lipid-associated membrane proteins and the host cells. J Zhejiang Univ Sci B. (2006) 7:342–50. doi: 10.1631/jzus.2006.B0342
166. Shibata KI, Hasebe A, Into T, Yamada M, Watanabe T. The N-terminal lipopeptide of a 44-kDa membrane-bound lipoprotein of Mycoplasma salivarium is responsible for the expression of intercellular adhesion molecule-1 on the cell surface of normal human gingival fibroblasts. J Immunol. (2000) 165:6538–44. doi: 10.4049/jimmunol.165.11.6538
167. Hayashi S, Wu HC. Lipoproteins in bacteria. J Bioenerg Biomembr. (1990) 22:451–71. doi: 10.1007/BF00763177
168. Sutcliffe IC, Russell R. Lipoproteins of gram-positive bacteria. Bacteriol J. (1995) 177:1123. doi: 10.1128/JB.177.5.1123-1128.1995
169. Majumder S, Zappulla F, Silbart LK. Mycoplasma gallisepticum lipid associated membrane proteins up-regulate inflammatory genes in chicken tracheal epithelial cells via TLR-2 ligation through an NF-κB dependent pathway. PLoS ONE. (2014) 9:e112796. doi: 10.1371/journal.pone.0112796
170. Wen LT, Knowles AF. Extracellular ATP and adenosine induce cell apoptosis of human hepatoma Li-7A cells via the A3 adenosine receptor. Br Pharmacol J. (2003) 140:1009–18 doi: 10.1038/sj.bjp.0705523
171. Hopfe M, Henrich B. OppA, the ecto-ATPase of Mycoplasma hominis induces ATP release and cell death in HeLa cells. BMC Microbiol. (2008) 8:55. doi: 10.1186/1471-2180-8-55
172. Schmidt JA, Browning GF, Markham PF. Mycoplasma hyopneumoniae mhp379 is a Ca2+-dependent, sugar-nonspecific exonuclease exposed on the cell surface. Bacteriol J. (2007) 189:3414–24. doi: 10.1128/JB.01835-06
173. Vilei EM, Abdo EM, Nicolet J, Botelho A, Goncalves R, Frey J. Genomic and antigenic differences between the European and African/Australian clusters of Mycoplasma mycoides subsp. mycoides SC. Microbiology. (2000) 146:477–486. doi: 10.1099/00221287-146-2-477
174. Vilei EM, Frey J. Genetic and biochemical characterization of glycerol uptake in Mycoplasma mycoides subsp. mycoides SC: its impact on H(2)O(2) production and virulence. Clin Diagn Lab Immunol. (2001) 8:85–92. doi: 10.1128/CDLI.8.1.85-92.2001
175. Sachse K, Helbig JH, Lysnyansky I, Grajetzki C, Muller W, Jacobs E, et al. Epitope mapping of immunogenic and adhesive structures in repetitive domains of Mycoplasma bovis variable surface lipoproteins. Infect Immun. (2000) 68:680–7. doi: 10.1128/IAI.68.2.680-687.2000
176. Bolland JR, Dybvig K. Mycoplasma pulmonis Vsa proteins and polysaccharide modulate adherence to pulmonary epithelial cells. FEMS Microbiol Lett. (2012) 331:25–30. doi: 10.1111/j.1574-6968.2012.02551.x
177. Davis KL, Wise KS. Site-specific proteolysis of the MALP-404 lipoprotein determines the release of a soluble selective lipoprotein-associated motif-containing fragment and alteration of the surface phenotype of Mycoplasma fermentans. Infect Immun. (2002) 70:1129–35. doi: 10.1128/IAI.70.3.1129-1135.2002
178. Röske K, Blanchard A, Chambaud I, Citti C, Helbig JH, Prevost, et al. Phase variation among major surface antigens of Mycoplasma penetrans. Infect Immun. (2001) 69:7642–51. doi: 10.1128/IAI.69.12.7642-7651.2001
179. Simmons WL, Dybvig K. The Vsa proteins modulate susceptibility of Mycoplasma pulmonis to complement killing, hemadsorption, and adherence to polystyrene. Infect Immun. (2003) 71:5733–8. doi: 10.1128/IAI.71.10.5733-5738.2003
180. Tu AH, Clapper B, Schoeb T, Elgavish A, Zhang J, Liu L, et al. Association of a major protein antigen of Mycoplasma arthritidis with virulence. Infect Immun. (2005) 73:245–9. doi: 10.1128/IAI.73.1.245-249.2005
181. Zhang Q, Wise KS. Coupled phase-variable expression and epitope masking of selective surface lipoproteins increase surface phenotypic diversity in Mycoplasma hominis. Infect Immun. (2001) 69:5177–81. doi: 10.1128/IAI.69.8.5177-5181.2001
182. Sachse K, Pfützner H, Heller M, Hänel I. Inhibition of Mycoplasma bovis cytadherence by a monoclonal antibody and various carbohydrate substances. Vet Microbiol. (1993) 36:307–16. doi: 10.1016/0378-1135(93)90097-Q
183. Bürki S, Frey J, Pilo P. Virulence, persistence and dissemination of Mycoplasma bovis. Vet Microbiol. (2015) 179:15–22. doi: 10.1016/j.vetmic.2015.02.024
184. Watson HL, Blalock DK, Cassell GH. Variable antigens of Ureaplasma urealyticum containing both serovar-specific and serovar-cross-reactive epitopes. Infect Immun. (1990) 58:3679–88. doi: 10.1128/IAI.58.11.3679-3688.1990
185. Zheng X, Teng LJ, Watson HL, Glass JI, Blanchard A, Cassell GH. Small repeating units within the Ureaplasma urealyticum MB antigen gene encode serovar specificity and are associated with antigen size variation. Infect Immun. (1995) 63:891–8. doi: 10.1128/IAI.63.3.891-898.1995
186. Uchida K, Nakahira K, Mimura K, Shimizu T, De Seta F, Wakimoto T, et al. Effects of Ureaplasma parvum lipoprotein multiple-banded antigen on pregnancy outcome in mice. J Reprod Immunol. (2013) 100:118–27. doi: 10.1016/j.jri.2013.10.001
187. Knox CL, Dando SJ, Nitsos I, Kallapur SG, Jobe AH, Payton D, et al. The severity of chorioamnionitis in pregnant sheep is associated with in vivo variation of the surface-exposed multiple-banded antigen/gene of Ureaplasma parvum. Biol Reprod. (2010) 83:415–26. doi: 10.1095/biolreprod.109.083121
188. Dando SJ, Nitsos I, Kallapur SG, Newnham JP, Polglase GR, Pillow JJ, et al. The role of the multiple banded antigen of Ureaplasma parvum in intra-amniotic infection: major virulence factor or decoy? PLoS ONE. (2012) 7:e29856. doi: 10.1371/journal.pone.0029856
189. Cartner SC, Simecka JW, Briles DE, Cassell GH, Lindsey JR. Resistance to mycoplasmal lung disease in mice is a complex genetic trait. Infect Immun. (1996) 64:5326–31. doi: 10.1128/IAI.64.12.5326-5331.1996
190. Shaw JH, Falkow S. Model for invasion of human tissue culture cells by Neisseria gonorrhoeae. Infect Immun. (1988) 56:1625–32. doi: 10.1128/IAI.56.6.1625-1632.1988
191. Rottem S, Naot Y. Subversion and exploitation of host cells by mycoplasmas. Trends Microbiol. (1998) 6:436–40. doi: 10.1016/S0966-842X(98)01358-4
192. Chang HY, Jordan JL, Krause DC. Domain analysis of protein P30 in Mycoplasma pneumoniae cytadherence and gliding motility. Bacteriol J. (2011) 193:1726–33. doi: 10.1128/JB.01228-10
193. Mudahi-Orenstein S, Levisohn S, Geary SJ, Yogev D. Cytadherence-deficient mutants of Mycoplasma gallisepticum generated by transposon mutagenesis. Infect Immun. (2003) 71:3812–20. doi: 10.1128/IAI.71.7.3812-3820.2003
194. Sachse K, Grajetzki C, Rosengarten R, Hanel I, Heller M, Pfutzner H. Mechanisms and factors involved in Mycoplasma bovis adhesion to host cells. Zentralbl Bakteriol. (1996) 284:80–92. doi: 10.1016/S0934-8840(96)80157-5
195. Song Z, Li Y, Liu Y, Xin J, Zou X, Sun W. alpha-Enolase, an adhesion-related factor of Mycoplasma bovis. PLoS ONE. (2012) 7:e38836. doi: 10.1371/journal.pone.0038836
196. Dallo SF, Baseman JB. Intracellular DNA replication and long-term survival of pathogenic mycoplasmas. Microb Pathog. (2000) 29:301–9. doi: 10.1006/mpat.2000.0395
197. Winner F, Rosengarten R, Citti C. In vitro cell invasion of Mycoplasma gallisepticum. Infect Immun. (2000) 68:4238–44. doi: 10.1128/IAI.68.7.4238-4244.2000
198. Marques LM, Ueno PM, Buzinhani M, Cortez BA, Neto RL, Yamaguti M, et al. Invasion of Ureaplasma diversum in Hep-2 cells. BMC Microbiol. (2010) 10:83. doi: 10.1186/1471-2180-10-83
199. Ibrahim S, Salilew-Wondim D, Rings F, Hoelker M, Neuhoff C, Tholen E, et al. Expression pattern of inflammatory response genes and their regulatory micrornas in bovine oviductal cells in response to lipopolysaccharide: implication for early embryonic development. PLoS ONE. (2015) 10:e0119388. doi: 10.1371/journal.pone.0119388
200. Andreev J, Borovsky Z, Rosenshine I, Rottem S. Invasion of HeLa cells by Mycoplasma penetrans and the induction of tyrosine phosphorylation of a 145-kDa host cell protein. FEMS Microbiol Lett. (1995) 132:189–94. doi: 10.1111/j.1574-6968.1995.tb07832.x
201. Yavlovich A, Tarshis M, Rottem S. Internalization and intracellular survival of Mycoplasma pneumoniae by non-phagocytic cells. FEMS Microbiol Lett. (2004) 233:241–6. doi: 10.1111/j.1574-6968.2004.tb09488.x
202. Yavlovich A, Katzenell A, Tarshis M, Higazi AA, Rottem S. Mycoplasma fermentans binds to and invades HeLa cells: involvement of plasminogen and urokinase. Infect Immun. (2004) 72:5004–11. doi: 10.1128/IAI.72.9.5004-5011.2004
203. Shibata K, Sasaki T, Watanabe T. AIDS-associated mycoplasmas possess phospholipases C in the membrane. Infect Immun. (1995) 63:4174–7. doi: 10.1128/IAI.63.10.4174-4177.1995
204. Vanden Bush TJ, Rosenbusch RF. Mycoplasma bovis induces apoptosis of bovine lymphocytes. FEMS Immunol Med Microbiol. (2002) 32:97–103. doi: 10.1111/j.1574-695X.2002.tb00540.x
205. Wu Y, Qiu H, Zeng Y, You X, Deng Z, Yu M, et al. Mycoplasma genitalium lipoproteins induce human monocytic cell expression of proinflammatory cytokines and apoptosis by activating nuclear factor kappaB. Mediators Inflamm. (2008) 2008:195427. doi: 10.1155/2008/195427
206. Li Y, Jiang Z, Xue D, Deng G, Li M, Liu X, et al. Mycoplasma ovipneumoniae induces sheep airway epithelial cell apoptosis through an ERK signalling-mediated mitochondria pathway. BMC Microbiol. (2016) 16:222. doi: 10.1186/s12866-016-0842-0
207. Liu W, Zhou D, Yuan F, Liu Z, Duan Z, Yang K, et al. Surface proteins mhp390 (P68) contributes to cilium adherence and mediates inflammation and apoptosis in Mycoplasma hyopneumoniae. Microb Pathog. (2019) 126:92–100. doi: 10.1016/j.micpath.2018.10.035
208. Menaker RJ, Jones NL. Fascination with bacteria-triggered cell death: the significance of Fas-mediated apoptosis during bacterial infection in vivo. Microbes Infect. (2003) 5:1149–58. doi: 10.1016/j.micinf.2003.08.001
209. Gaunson J, Philip C, Whithear K, Browning G. The cellular immune response in the tracheal mucosa to Mycoplasma gallisepticum in vaccinated and unvaccinated chickens in the acute and chronic stages of disease. Vaccine. (2006) 24:2627–33. doi: 10.1016/j.vaccine.2005.12.008
210. Thanawongnuwech R, Young TF, Thacker BJ, Thacker EL. Differential production of proinflammatory cytokines: in vitro PRRSV and Mycoplasma hyopneumoniae co-infection model. Vet Immunol Immunopathol. (2001) 79:115–27. doi: 10.1016/S0165-2427(01)00243-4
211. He J, You X, Zeng Y, Yu M, Zuo L, Wu Y. Mycoplasma genitalium-derived lipid-associated membrane proteins activate NF-kappaB through toll-like receptors 1, 2, and 6 and CD14 in a MyD88-dependent pathway. Clin Vaccine Immunol. (2009) 16:1750–7. doi: 10.1128/CVI.00281-09
212. Peltier MR, Freeman A, Mu HH, Cole BC. Characterization of the macrophage-stimulating activity from Ureaplasma urealyticum. Am J Reprod Immunol. (2007) 57:186–92. doi: 10.1111/j.1600-0897.2006.00460.x
213. Shio MT, Hassan GS, Shah WA, Nadiri A, El Fakhry Y, Li H, et al. Coexpression of TLR2 or TLR4 with HLA-DR potentiates the superantigenic activities of Mycoplasma arthritidis derived mitogen. J Immunol. (2014) 192:2543–50. doi: 10.4049/jimmunol.1300591
214. Garcia J, Lemercier B, Roman-Roman S, Rawadi G. A Mycoplasma fermentans-derived synthetic lipopeptide induces AP-1 and NF-κB activity and cytokine secretion in macrophages via the activation of mitogen-activated protein kinase pathways. J Biol Chem. (1998) 273:34391–8. doi: 10.1074/jbc.273.51.34391
215. Rawadi G, Garcia J, Lemercier B, Roman-Roman S. Signal transduction pathways involved in the activation of NF-κB, AP-1, and c-fos by Mycoplasma fermentans membrane lipoproteins in macrophages. J Immunol. (1999) 162:2193–203.
216. Mühlradt P, Frisch M. Purification and partial biochemical characterization of a Mycoplasma fermentans-derived substance that activates macrophages to release nitric oxide, tumor necrosis factor, and interleukin-6. Infect Immun. (1994) 62:3801–7. doi: 10.1128/IAI.62.9.3801-3807.1994
217. Schröder NW, Heine H, Alexander C, Manukyan M, Eckert J, Hamann L, et al. Lipopolysaccharide binding protein binds to triacylated and diacylated lipopeptides and mediates innate immune responses. J Immunol. (2004) 173:2683–91. doi: 10.4049/jimmunol.173.4.2683
218. Kawai T, Akira S. Signaling to NF-kappaB by Toll-like receptors. Trends Mol Med. (2007) 13:460–9. doi: 10.1016/j.molmed.2007.09.002
219. Arthur JS, Ley SC. Mitogen-activated protein kinases in innate immunity. Nat Rev Immunol. (2013) 13:679–92. doi: 10.1038/nri3495
220. Muneta Y, Minagawa Y, Shimoji Y, Ogawa Y, Hikono H. Immune response of gnotobiotic piglets against Mycoplasma hyopneumoniae. J Vet Med Sci. (2008) 70:1065–70. doi: 10.1292/jvms.70.1065
221. Hwang MH, Chang ZQ, Kang EH, Lim JH, Yun HI, Rhee MH, et al. Surfactin C inhibits Mycoplasma hyopneumoniae-induced transcription of proinflammatory cytokines and nitric oxide production in murine RAW 264.7 cells. Biotechnol Lett. (2008) 30:229–33. doi: 10.1007/s10529-007-9552-x
222. Damte D, Lee S, Hwang M, Gebru E, Choi M, Lee J, et al. Inflammatory responses to Mycoplasma hyopneumoniae in murine alveolar macrophage cell lines. N Z Vet J. (2011) 59:185–90. doi: 10.1080/00480169.2011.579553
223. Silva JR, Ferreira LF, Oliveira PV, Nunes IV, Pereira ÍS, Timenetsky J, et al. Intra-uterine experimental infection by Ureaplasma diversum induces TNF-α mediated womb inflammation in mice. An Acad Bras Cienc. (2016) 88:643–52. doi: 10.1590/0001-3765201620150244
224. Chelmonska-Soyta A, Miller R, Ruhnke L, Rosendal S. Activation of murine macrophages and lymphocytes by Ureaplasma diversum. Canad J Vet Res. (1994) 58:275.
225. You X, Wu Y, Zeng Y, Deng Z, Qiu H, Yu M. Mycoplasma genitalium-derived lipid-associated membrane proteins induce activation of MAPKs, NF-kappaB and AP-1 in THP-1 cells. FEMS Immunol Med Microbiol. (2008) 52:228–36. doi: 10.1111/j.1574-695X.2007.00366.x
226. Quesniaux V, Fremond C, Jacobs M, Parida S, Nicolle D, Yeremeev V, et al. Toll-like receptor pathways in the immune responses to mycobacteria. Microbes Infect. (2004) 6:946–59. doi: 10.1016/j.micinf.2004.04.016
227. Jin MS, Kim SE, Heo JY, Lee ME, Kim HM, Paik G, et al. Crystal structure of the TLR1-TLR2 heterodimer induced by binding of a tri-acylated lipopeptide. Cell. (2007) 130:1071–82. doi: 10.1016/j.cell.2007.09.008
228. Kang JY, Nan X, Jin MS, Youn S.-J., Ryu YH, et al. Recognition of lipopeptide patterns by Toll-like receptor 2-Toll-like receptor 6 heterodimer. Immunity. (2009) 31:873–84. doi: 10.1016/j.immuni.2009.09.018
229. Shimizu T, Kida Y, Kuwano K. A dipalmitoylated lipoprotein from Mycoplasma pneumoniae activates NF-κB through TLR1, TLR2, and TLR6. J Immunol. (2005) 175:4641–6. doi: 10.4049/jimmunol.175.7.4641
230. Shimizu T, Kida Y, Kuwano K. Ureaplasma parvum lipoproteins, including MB antigen, activate NF-κB through TLR1, TLR2 and TLR6. Microbiology. (2008) 154:1318–25. doi: 10.1099/mic.0.2007/016212-0
231. Shimizu T, Kimura Y, Kida Y, Kuwano K, Tachibana M, Hashino M, et al. Cytadherence of Mycoplasma pneumoniae induces inflammatory responses through autophagy and Toll-Like receptor 4. Infect Immun. (2014) 82:3076–86. doi: 10.1128/IAI.01961-14
232. Rawadi G, Roman-Roman S. Mycoplasma membrane lipoproteins induced proinflammatory cytokines by a mechanism distinct from that of lipopolysaccharide. Infect Immun. (1996) 64:637–43. doi: 10.1128/IAI.64.2.637-643.1996
233. Fourour S, Marois-Créhan C, Martelet L, Fablet C, Kempf I, Gottschalk M, et al. Intra-Species and inter-species differences in cytokine production by porcine antigen-presenting cells stimulated by. Pathogens. (2019) 8:34. doi: 10.3390/pathogens8010034
234. Moser M, Murphy KM. Dendritic cell regulation of TH1-TH2 development. Nat. Immunol. (2000) 1:199–205. doi: 10.1038/79734
235. Mulongo M, Prysliak T, Scruten E, Napper S, Perez-Casal J. In vitro infection of bovine monocytes with Mycoplasma bovis delays apoptosis and suppresses production of gamma interferon and tumor necrosis factor alpha but not interleukin-10. Infect Immun. (2014) 82:62–71. doi: 10.1128/IAI.00961-13
236. Jimbo S, Suleman M, Maina T, Prysliak T, Mulongo M, Perez-Casal J. Effect of Mycoplasma bovis on bovine neutrophils. Vet Immunol Immunopathol. (2017) 188:27–33. doi: 10.1016/j.vetimm.2017.04.011
237. Thanawongnuwech R, Thacker B, Halbur P, Thacker EL. Increased production of proinflammatory cytokines following infection with porcine reproductive and respiratory syndrome virus and Mycoplasma hyopneumoniae. Clin Diagn Lab Immunol. (2004) 11:901–8. doi: 10.1128/CDLI.11.5.901-908.2004
Keywords: Ureaplasma diversum, Mollicutes, bovine, reproductive tract, respiratory infection
Citation: Santos Junior MN, Macêdo Neres NSd, Campos GB, Bastos BL, Timenetsky J and Marques LM (2021) A Review of Ureaplasma diversum: A Representative of the Mollicute Class Associated With Reproductive and Respiratory Disorders in Cattle. Front. Vet. Sci. 8:572171. doi: 10.3389/fvets.2021.572171
Received: 19 June 2020; Accepted: 13 January 2021;
Published: 18 February 2021.
Edited by:
Michael Kogut, United States Department of Agriculture, United StatesReviewed by:
Marie Rene Culhane, University of Minnesota, United StatesChristine Letitia Knox, Queensland University of Technology, Australia
Copyright © 2021 Santos Junior, Macêdo Neres, Campos, Bastos, Timenetsky and Marques. This is an open-access article distributed under the terms of the Creative Commons Attribution License (CC BY). The use, distribution or reproduction in other forums is permitted, provided the original author(s) and the copyright owner(s) are credited and that the original publication in this journal is cited, in accordance with accepted academic practice. No use, distribution or reproduction is permitted which does not comply with these terms.
*Correspondence: Lucas Miranda Marques, bHVjYXNtQHVmYmEuYnI=