- 1Touro University College of Osteopathic Medicine, Vallejo, CA, United States
- 2Department of Neurosurgery, Stanford University, Stanford, CA, United States
- 3Division of Pediatric Neurosurgery, Lucile Packard Children’s Hospital, Palo Alto, CA, United States
- 4Department of Neurosurgery, Duke University, Durham, NC, United States
The optimal management of severe traumatic brain injury (TBI) in the pediatric population has not been well studied. There are a limited number of research articles studying the management of TBI in children. Given the prevalence of severe TBI in the pediatric population, it is crucial to develop a reference TBI management plan for this vulnerable population. In this review, we seek to delineate the differences between severe TBI management in adults and children. Additionally, we also discuss the known molecular pathogenesis of TBI. A better understanding of the pathophysiology of TBI will inform clinical management and development of therapeutics. Finally, we propose a clinical algorithm for the management and treatment of severe TBI in children using published data.
Introduction
TBI is defined as injury to the head and to the underlying brain caused by an external force, causing an impairment in brain function. According to the Center for Diseases Control and Prevention (CDC), approximately 224,000 people in the United States in 2017 were hospitalized due to TBI, and 7.8% of these people were children ages 0–17 years old (Peterson, 2021). There were around 61,000 deaths related to TBI in the United States in 2017 and around 4.5% of these deaths were in children (Peterson, 2021). TBI also affects the pediatric population globally. For example, in Korea, 31.9% of children that were admitted to hospitals were diagnosed with TBI between June 2008 and May 2009 (Kim et al., 2012). Similarly, it was reported in India that 21% of children admitted to the emergency department in a large teaching hospital were due to TBI (Tabish et al., 2006).
The age and sex of a child also seems to be a factor that influences the likelihood of sustaining a TBI. Several studies report that very young children (0–2 years old) and adolescents (15–18 years old) both are the most likely to experience TBI, while children whose age are in between are less likely to experience TBI by comparison (Langlois and Thomas, 2004; Keenan and Bratton, 2006; Schneier et al., 2006; Amaranath et al., 2014; Greene et al., 2014; Majdan et al., 2014; Dewan et al., 2016). Studies indicate that male children are more likely to be hospitalized or die due to TBI compared to their female counterparts (Langlois and Thomas, 2004; Keenan and Bratton, 2006; Peterson, 2021). However, in the context of athletics, the rate of concussion in girls’ sports are higher than those in boys’ sports (Dick, 2009; Covassin and Elbin, 2011; Lincoln et al., 2011; Marar et al., 2012; Rosenthal et al., 2014; Arambula et al., 2019). While uncertain, possible reasons for difference in concussion rate may be secondary to anatomic differences between sexes, such as head mass and neck girth (Covassin and Elbin, 2011; Arambula et al., 2019). Further research exploring the underlying mechanisms of sex differences in TBI would be of value in guiding treatment recommendations.
Across all ages, the most common causes of TBI are falls, being struck by an object, motor vehicle accident, assault, and self-harm (Control, 2014; Capizzi et al., 2020). According to the CDC data, the most common mechanisms of injury for TBI that result in death are self-harm, falls, and motor vehicle accidents (Control, 2014; Capizzi et al., 2020). The mechanisms of injury for TBI depends also heavily on the region in the world. For example, in Bronx, New York, it was found that 34% of TBIs were due to violence, while 32% of TBI cases were from falls, and 27% from traffic accidents (Cooper et al., 1983; Bruns and Hauser, 2003). However, in France, the majority of TBI cases were caused by traffic accidents (60% of TBI cases) (Tiret et al., 1990; Bruns and Hauser, 2003). In children, the most common causes of TBI are falls and motor vehicle collisions (Dewan et al., 2016). Importantly, international differences in reporting procedures may limit the comparability of data between nations and healthcare systems.
In the United States, around 10% of all cases of TBIs are severe TBI (Wagner, 2016; Capizzi et al., 2020). From the 2006 Kid’s Inpatient Database, which includes data from more than 3,000 hospitals in the United States, it is estimated that 45,875 children were hospitalized due to TBI, while 7,889 children were hospitalized due to severe TBI (Stanley et al., 2012). Severe TBI in children is a large economic burden in the United States, with medical costs and productivity losses costing around $60.4 billion dollars (Corso et al., 2006; Bell et al., 2017).
Given the prevalence of severe TBI in the pediatric population, it is crucial for there to be a guideline TBI management plan particularly for this population. However, despite this need, there are a limited number of research articles studying the management of severe TBI in children. In this review, we seek to delineate the differences between severe TBI management in adults and children. In addition, we summarize the molecular pathogenesis of TBI. Lastly, we propose a clinical algorithm for the management and treatment of severe TBI in children using published data.
Classification of TBI
Classification of TBI is based on clinical severity or physical causes (Saatman et al., 2008; Hawryluk and Manley, 2015). However, due to the diverse causes of TBI and our limited understanding of the pathophysiology of the different types of TBI, classification of TBI is not as refined and robust as the classification for other diseases (Saatman et al., 2008; Hawryluk and Manley, 2015). Classification by physical mechanism is based on whether the injury is blunt or penetrating (Hawryluk and Manley, 2015). Under classification by physical mechanism, we can also classify TBI as primary or secondary (Hawryluk and Manley, 2015; Capizzi et al., 2020). Primary injury is when a direct hit or indirect hit (acceleration-deceleration mechanism) causes the damage, and secondary injury is related to cellular and molecular damage, vasogenic edema (extracellular edema), and cytogenic edema (intracellular edema) (Elovic and Galang, 2010; Wagner, 2016; Capizzi et al., 2020).
Another method of classifying TBI is through the pathoanatomy (diffuse vs. focal). Focal injury is mostly due to direct contact while diffuse is more likely due to acceleration-deacceleration forces (Gennarelli, 1985; Hawryluk and Manley, 2015). Focal injury can be further broken down into different types: brain contusion, intraparenchymal brain hemorrhage, subdural hematoma, epidural hematoma, and subarachnoid hemorrhage (Andriessen et al., 2010).
Classification of TBI can also be based off severity. According to the CDC, the classification of the severity of TBI depends on the Glasgow Coma Scale (GCS), with mild TBI having a GCS score of 13–15, moderate TBI having a GCS score of 9–12, and severe TBI having a GCS score of lower than 9 (Teasdale and Jennett, 1974; 1976). Classification of TBI can also be based on the loss of consciousness, with 0–30 min of loss of consciousness as mild TBI, between 30 min and 24 h as moderate TBI, and more than 24 h as severe TBI (Defense, 2016; Capizzi et al., 2020).
In addition to these different classifications, there are additional classifications that are not widely utilized including, the Brussel Coma Grades and Innsbruck Coma Scale (Gerstenbrand and Lücking, 1970; Brihaye et al., 1978; Hawryluk and Manley, 2015). There are efforts to develop next generation classification of TBI in a more precise and personalized fashion, where treatment is based off of biomarkers within individual patients (Okonkwo et al., 2013; Hawryluk and Manley, 2015).
Pathophysiology and Molecular Pathogenesis of TBI
The molecular pathogenesis of TBI occurs in two phases: an acute primary injury and a delayed secondary injury (Table 1) (Pearn et al., 2017; Sulhan et al., 2020). The primary phase occurs during impact, which leads to the disruption of cellular membranes, resulting in a dysregulation of concentration gradients for potassium, sodium, and calcium (Figure 1) (Wolf et al., 2001; Cuccurullo, 2010; Dixon, 2017; Pearn et al., 2017; Eapen and Cifu, 2018; Capizzi et al., 2020; Sulhan et al., 2020). Increased intracellular calcium levels activates a protease called calpain, leading to the degradation of the cytoskeleton (Pearn et al., 2017; Sulhan et al., 2020). Additionally, calcium also activates N-methyl-D-aspartate (NMDA) receptors, causing cell depolarization and increased mitochondrial calcium levels, which leads to the buildup of reactive oxygen species (ROS), leading to cell death (Pearn et al., 2017). Oxidative metabolism is also altered, which results in lactate production, acidosis, and edema (Pearn et al., 2017; Sulhan et al., 2020).
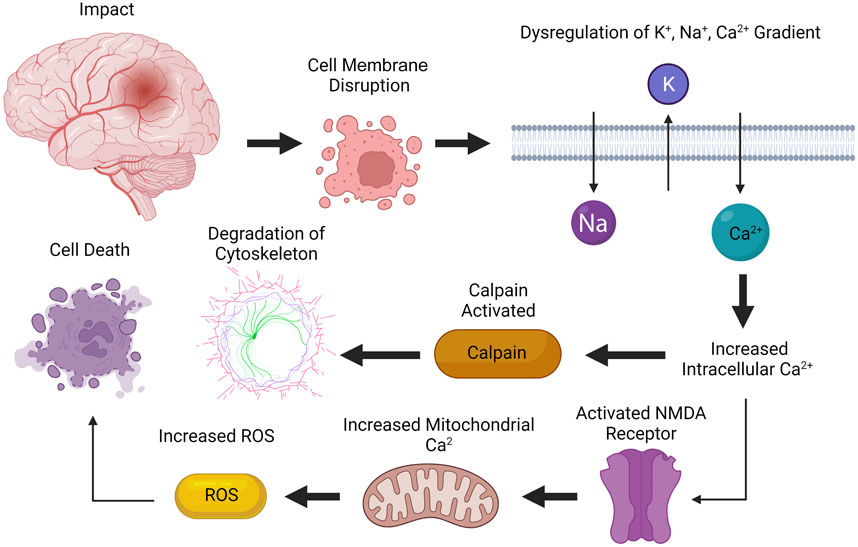
FIGURE 1. Primary phase of injury from TBI. The primary phase of injury occurs during impact, leading to disruption of cellular membranes. Increased intracellular calcium levels activates calpain, leading to degradation of cytoskeleton. Calcium also activates NMDA receptors, causing increased mitochondrial calcium levels, which increases the concentration of reactive oxygen species (ROS), leading to cell death. Created with BioRender.com.
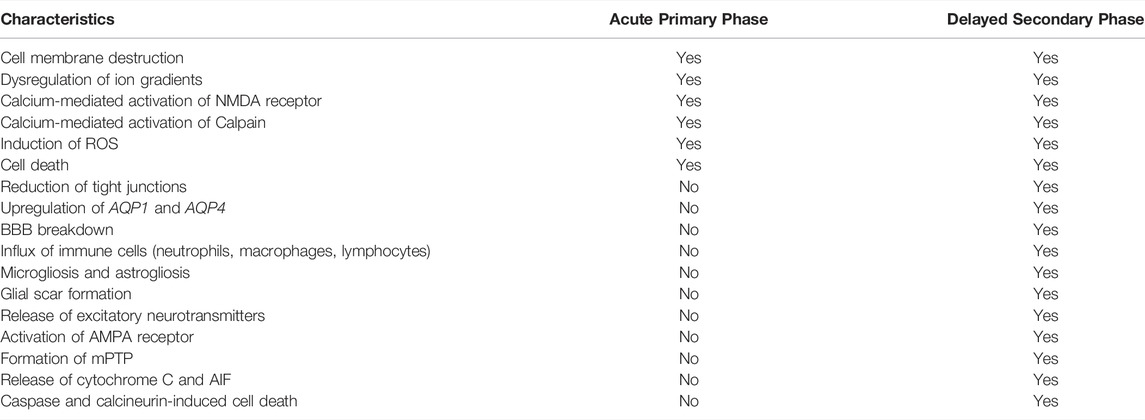
TABLE 1. Differences in TBI pathophysiology between the acute primary phase and the delayed secondary phase of injury.
The second delayed phase consists of the breakdown of the blood brain barrier (BBB), induction of neuroinflammation, excitotoxity, oxidative stress, apoptosis, and mitochondria dysfunction, leading to further damage (Figure 2) (Pearn et al., 2017; Ng and Lee, 2019). Upregulation of aquaporin 1 (AQP1) and aquaporin 4 (AQP 4) on endothelial cells and reduction of tight junction proteins are factors that causes BBB disruption (Liu et al., 2017; Sulhan et al., 2020). The production of metalloproteinases (MMPs) and ROS also leads to the disruption of the integrity of the BBB (Sulhan et al., 2020). In rodent models, it is found that there is a biphasic increase in BBB permeability (Shapira et al., 1993; Baldwin et al., 1996; Başkaya et al., 1997; Hicks et al., 1997; Chodobski et al., 2011), with the first opening associated with the influx of neutrophils (Chodobski et al., 2011). The mechanism of the second increase in BBB permeability is less well studied (Chodobski et al., 2011).
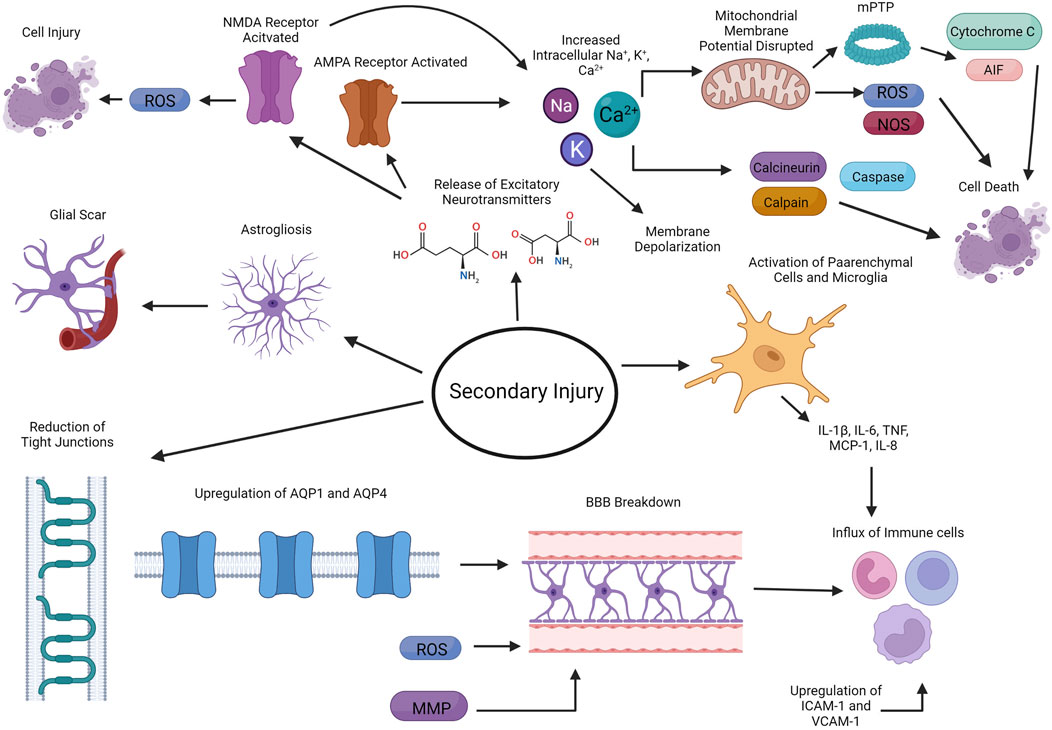
FIGURE 2. Secondary delayed phase of injury from TBI. The second delayed phase of injury consists of the breakdown of the blood brain barrier (BBB), induction of neuroinflammation, excitotoxity, oxidative stress, apoptosis, and mitochondria dysfunction. Created with BioRender.com.
Neuroinflammation also plays an important role in pathophysiology of TBI. Parenchymal cells along with microglia in the brain start producing proinflammatory cytokines such as IL-1β, IL-6 and TNF, which leads to the influx of inflammatory cells into the brain, including neutrophils, monocytes/macrophages, and lymphocytes (Ahn et al., 2004; Buttram et al., 2007; Goodman et al., 2008; Lotocki et al., 2009; Frugier et al., 2010; Semple et al., 2010; Chodobski et al., 2011; Aungst et al., 2014; Pearn et al., 2017; Ng and Lee, 2019). Chemokines, including MCP-1 and IL-8, are also upregulated in the injured tissue, which further helps recruit other leukocytes to the site of injury (Kossmann et al., 1997; Buttram et al., 2007; Bye et al., 2007; Semple et al., 2010; Ng and Lee, 2019). An increase in expression of VCAM-1 and ICAM-1 on endothelial cells and leukocytes/endothelial cells, respectively, facilitates the recruitment of leukocytes to site of injury (Carlos et al., 1997; Rancan et al., 2001; Ng and Lee, 2019). Astrogliosis and microgliosis also occurs, further contributing to the neuroinflammation occurring at the site injury (Chiu et al., 2016). Ultimately, astrocytes then form a glia scar, which serves as a barrier to separate the damaged tissue from healthy tissue (Fitch and Silver, 2008). Although neuroinflammation is crucial to the repair and recovery from TBI, chronic and persistent neuroinflammation is thought to also contribute to neurodegeneration (Simon et al., 2017).
In addition to neuroinflammation, TBI also induces the release of excitatory neurotransmitters such as glutamate and aspartate from neurons (Chamoun et al., 2010). These neurotransmitters can then bind to NMDA and α-amino-3-hydroxy-5-methyl-4-isoxazolepropionic acid receptor (AMPA) receptors, leading to the influx of sodium, calcium and potassium, which subsequently depolarizes these neurons (Ng and Lee, 2019). Calcium in particular can activate calcineurin, calpain, and caspases, which leads to apoptosis while activation of NMDA ultimately leads to the production of ROS, further exacerbating neural injury (Ng and Lee, 2019).
Mitochondria dysfunction is another consequence of TBI. Increases in calcium concentrations are buffered by the mitochondria via a variety of transporters, including the mitochondrial calcium uniporter and uncoupling proteins (Williams et al., 2013). This buffering can lead to disruption of the mitochondria membrane potential, which drives further increase in ROS and reactive nitrogen species (RNS) (Lamade et al., 2020). Upon loss of mitochondria membrane potential, the mitochondrial permeability transition pore (mPTP) formation begins (Szabó and Zoratti, 1991). The formation of mPTP allows for the release of proteins including cytochrome c and apoptosis-inducing factor (AIF), leading to both caspase-dependent and independent cell death (Lamade et al., 2020).
TBI in Pediatric Population Versus Adult Population
There exist major physiological and anatomical differences between adults and children which impact management of TBI (Supplementary Table S1). Since the fontanelles and sutures may be open in very young pediatric patients, it is possible that these patients can mitigate the rise in intracranial pressure (ICP) levels (Figaji, 2017). The opening of the fontanelles and sutures increase cerebral compliance in young children (Figaji, 2017). It should also be noted that BP and ICP are generally lower in pediatric patients, and even a small increase can lead to significant adverse events (Figaji, 2017). If autoregulation is impaired in a pediatric patient, then high BP can be harmful (Figaji, 2017). While there are recommended values for cerebral perfusion pressure (CPP) in children, the evidence for these values are not well supported (Figaji, 2017).
Children also have smaller lung volumes, which must be taken into consideration when managing ventilatory status. Caution must be taken to not hyperventilate the child, which can lead to hypocapnia and ischemia (Figaji, 2017). The airway of a pediatric patient is also more prone to obstruction (Figaji, 2017). While some guidelines, such as the 2019 Management of Pediatric Severe Traumatic Brain Injury by Kochanek et al. (2019b) include hypothermia as a second-tier therapy, there is little to no evidence showing the effectiveness of this type of therapy in children (Hutchison et al., 2008; Adelson et al., 2013; Crompton et al., 2017; Figaji, 2017; Lewis et al., 2017). However, there is evidence supporting the prevention of hyperthermia in children (Figaji, 2017). There are also no standardized formulations for hyperosmolar therapy for children (Kochanek et al., 2012; Bell et al., 2013; Figaji, 2017). Finally, the use of decompressive craniectomy is seen as controversial (Taylor et al., 2001; Figaji et al., 2003; Figaji et al., 2006; Kan et al., 2006; Rutigliano et al., 2006; Jagannathan et al., 2007; Figaji et al., 2008; Adamo et al., 2009; Appelboom et al., 2011; Pérez Suárez et al., 2011; Figaji, 2017).
Management of TBI in Adults Versus Children
Neuroimaging
During the initial management of severe TBI (GCS 8 or less), both adults and children should undergo a computerized tomography (CT) scan (Wintermark et al., 2015). In addition to initial head CT, children also require additional imaging of the cervical spine and head along with radiographs and focused assessment with sonography for trauma (FAST). Cervical spine imaging in pediatric TBI is recommended secondary to the high association of injury to posterior ligamentous complex in abusive head trauma and accidental TBI (Choudhary et al., 2014; Baerg et al., 2017; Oh et al., 2017; Henry et al., 2021). Since many TBI pediatric cases may also involve trauma to other areas of the body, using FAST to detect trauma to the abdominal region is recommended. In children, it is important to not exclude the possibility of elevated ICP, despite a normal initial CT scan (Bailey et al., 2012; Kochanek et al., 2019b). Given that CT imaging findings may not detect intracranial hypertension, serial CT scans have limited value in guiding ongoing treatment particularly in the case of mild TBI (Tabori et al., 2000; Figg et al., 2006; da Silva et al., 2008; Rangel-Castilla et al., 2008; Bata and Yung, 2014). It is also not recommended to repeat CT scans performed more than 24 h after admission in severe TBI pediatric patients, unless ICP is increased or if patient has neurologic deterioration (Kochanek et al., 2019b). In contrast, adults with severe TBI may benefit from repeat head CT, as more evident radiographic changes can inform clinical management (Brown et al., 2004; Wang et al., 2006; Thomas et al., 2010).
ICP Management
Initial management of ICP includes elevating the head at 30° while maintaining the neck in a neutral position (Marehbian et al., 2017). In adults, it is recommended that the ICP is kept at 22 mmHg or lower (Carney et al., 2017; Schizodimos et al., 2020), because values above 22 mmHg are associated with increased levels of mortality (Carney et al., 2017). In children, it is suggested that the ICP should be kept lower than 20 mmHg (Kochanek et al., 2019b), since ICP higher than 20 mmHg is associated with poor clinical outcomes, including lower survival rates and disability (Esparza et al., 1985; Michaud et al., 1992; Jagannathan et al., 2008; Kukreti et al., 2014). Infusion of hypertonic saline (3% saline 0.1–1.0 ml/kg of body weight per hour) in children is recommended to keep ICP lower than 20 mmHg (Kochanek et al., 2019b). It is recommended that children with severe TBI (Kochanek et al., 2019b) and adults with GCS 3-8 and abnormal CT scan results (hematomas, contusions, swelling, herniation, or compressed basal cisterns) should have their ICP monitored (Carney et al., 2017). Additionally, the use of ICP monitoring is also warranted in adults with severe TBI even if they have a normal CT scan, given that two of the following are true: patient is older than 40 years old, patient has unilateral or bilateral motor posturing, or if patient’s systolic blood pressure is less than 90 mmHg (Carney et al., 2017).
CSF Drainage
Cerebrospinal fluid (CSF) drainage is used to lower ICP in both adults and children (Kochanek et al., 2019b). Both lumbar and ventricular drains seem to be effective in lowering ICP (Tuettenberg et al., 2009; Chau et al., 2019). Continuous CSF drainage is preferred over intermittent drainage due to it being more effective and having better control of ICP (Carney et al., 2017). Guidelines suggest that CSF drainage should be used when patient’s GCS is less than 6 during the first 12 h post-injury (Carney et al., 2017). Attempts to analyze the optimal timing of CSF diversion were limited due to high levels of bias and heterogeneity among published data (Chau et al., 2020). However, the majority of studies identified in this report simultaneously placed ICP monitors and ventricular drains or utilize a ventricular drain for both ICP monitoring and subsequent CSF drainage (Chau et al., 2020).
Fluids
Isotonic saline can be used to maintain euvolemia (Myburgh et al., 2007). In children, more than 2 h of serum sodium levels above 170 mEq/L should be avoided due to complications of anemia and thrombocytopenia (Kochanek et al., 2019b). Sustained serum sodium levels above 160 mEq/L should also be avoided since it can cause complications related to deep vein thrombosis (Kochanek et al., 2019b). Notably, serum osmolarity of greater than 320 mOsm/L can result in neurological and renal side-effects (Bullock, 1995).
Blood Pressure
It is recommended that systolic blood pressure be maintained at or above 100 mmHg for patients 50–69 years old, and at or above 110 mmHg for patients between 15 and 49 years old and patients above 70 years old (Carney et al., 2017). The blood pressure needed to maintain minimum CPP allowing for metabolic needs to be met in children with severe TBI is not known, but largely depends on the age of the child (Figaji, 2017). Nonetheless, systolic blood pressure should be above the fifth percentile for the child’s age, since higher systolic blood pressure is thought to improve clinical outcomes (Samant et al., 2008; Suttipongkaset et al., 2018).
Endotracheal Intubation
Endotracheal intubation is needed to keep the patient’s PaO2 above 60 mmHg (Dellazizzo et al., 2018). In children with major trauma, the use of cuffed endotracheal tubes is recommended (Kleinman et al., 2010). However, complication rates from using cuffed endotracheal tubes in children is similar to that of uncuffed endotracheal tubes in critically ill children (Deakers et al., 1994; Khine et al., 1997).
Ventilation
Patients should maintain a PaO2 of more than 60 mmHg (Bratton et al., 2007) while maintaining PaCO2 more than 30 mmHg is recommended using end-tidal CO2 to measure CO2 levels (Stocchetti et al., 2005; Bratton et al., 2007). Lowering PaCO2 to less than 30 via hyperventilation is sometimes acceptable as a temporary way to resolve an ICP crisis (Diringer et al., 2000). However, it is not recommended to prolong hyperventilation such that it lowers PaCO2 to 25 mmHg or less and when using hyperventilation, measurements of BtpO2 should be used (Carney et al., 2017). It is also not recommended to use hyperventilation during the first 24 h after TBI, which is when cerebral blood flow is reduced (Carney et al., 2017). In children, PaCO2 should be maintained between 35 and 40 mmHg (Sharp and Tieves, 2015), and it is not recommended to induce hyperventilation so that PaCO2 is lower than 30 mmHg during the first 48 h after TBI (Kochanek et al., 2019b). However, if hyperventilation is needed to treat refractory intracranial hypertension, it is suggested that neuromonitoring is used to detect cerebral ischemia (Kochanek et al., 2019b).
Antiseizure Medications
Antiseizure pharmacological agents are recommended to prevent post-traumatic seizures (PTS) after TBI (Carney et al., 2017). Levetiracetam can be used, although there are insufficient data to say whether levetiracetam or phenytoin is better in early PTS (Carney et al., 2017; Kochanek et al., 2019b). In general, for preventing late PTS, phenytoin or valproate are not recommended while phenytoin can be used for preventing early PTS (Carney et al., 2017). Phenytoin has been shown to significantly reduce seizures during the first week after severe TBI, but this anti-seizure effect is lost after longer periods of time (Temkin et al., 1990).
Antifibrinolytic Therapy
A loading dose of 1 g of tranexamic acid can be infused over a time span of 10 min, and then an additional infusion of 1 g over 8 h afterwards (Slomski, 2019). Currently, it is unclear if there are benefits of administering tranexamic acid for severe TBI with intracranial hemorrhage (Bossers et al., 2021).
Venous Thromboembolism Prophylaxis
In the general population, intermittent pneumatic compression should be used when the patient is admitted to the hospital (Abdel-Aziz et al., 2015). In the adult population, low molecular weight heparin (LMWH), enoxaparin, or low-dose unfractionated heparin can be used in combination with intermittent pneumatic compression (Carney et al., 2017). However, with the use of these drugs, there is an increased risk of worsening of intracranial hemorrhage (Carney et al., 2017). Therefore, the clinician should make the judgement to use them based on imaging results and stability of the TBI (Carney et al., 2017). While there is limited data on venous thromboembolism prophylaxis in the pediatric TBI population, there is evidence that LMWH prophylaxis is more effective than unfractionated heparin in preventing venous thromboembolism (van Erp et al., 2021).
Management of Coagulopathy
There is a variation in what is considered a low platelet count in TBI patients. Current evidence suggests the maintenance of platelet count of patients at above 100,000–175,000/μl, using platelet transfusions if platelet necessary (Joseph et al., 2014). While this recommendation is based on studies in the adult TBI population, there are also several studies demonstrating the unfavorable impact of coagulopathy in pediatric TBI (Kamal et al., 2011; Podolsky-Gondim et al., 2018). In the pediatric TBI population, trauma-related coagulopathy (platelet count < 150,000/μl, fibrinogen < 180 mg/dl, aPTT > 1.2, or prothrombin time > 1.3) in TBI pediatric patients was associated with worse neurological outcomes (Podolsky-Gondim et al., 2018).
Glucose Management
In severe TBI patients, studies recommended that blood glucose levels are in the range of 140–180 mg/dl, since hyperglycemia or hypoglycemia should be avoided (Sharma and Vavilala, 2012). Increased complications and mortality rates have been associated in TBI patients with hyperglycemia (Alvis-Miranda et al., 2014). However, the SHINE trial demonstrated that stroke patients with an average glucose level of 118 mg/dl did not have significantly more favorable functional outcomes compared to stroke patients with an average glucose level of 179 mg/dl (Johnston et al., 2019). Given these results, the benefit of strict glucose control in children is unclear.
Temperature Management
In both adults and children, normothermia should be maintained (Carney et al., 2017; Kochanek et al., 2019b). Fever should be prevented by using antipyretic medications, cooling blankets, endovascular temperature management catheters, etc. (Puccio et al., 2009). Deliberate deep hypothermia should not be used to improve outcomes in adult patients (Carney et al., 2017; Kochanek et al., 2019b). In children, protocols recommending moderate hypothermia (32°C–33 °C) for the control refractory intracranial hypertension exist, but there is limited evidence of its efficacy on overall outcomes (Kochanek et al., 2019b).
Sedation and Analgesia
Patients with ICP elevation should be given a sedative agent and opioids (Rajajee et al., 2017). Fentanyl generally has greater efficacy compared to morphine (Wenderoth et al., 2013). Since propofol is effective in reducing cerebral metabolism and ICP, with a short duration permitting intermittent neurological assessments, it is the general preferred sedative agent (Farling et al., 1989; Pinaud et al., 1990; Flower and Hellings, 2012). However, it is important to also note that propofol may not improve mortality (Carney et al., 2017). Barbiturates are not recommended for prevention of intracranial hypertension (Carney et al., 2017). In children, etomidate is recommended for sedation as it can reduce ICP and improve CPP (Bramwell et al., 2006). If etomidate is not available, then ketamine has been proposed as an alternative (Scherzer et al., 2012). However, several studies demonstrated that ketamine has been associated with increase in ICP, while others have demonstrated its efficacy in decreasing ICP in the pediatric population, therefore making its benefit uncertain (Gibbs, 1972; Wyte et al., 1972; Yoram Ben and Watemberg, 2006; Bar-Joseph et al., 2009; Ketharanathan et al., 2017). Prolonged use of propofol may increase levels of lactic acid and therefore, it is not recommended for usage beyond rapid sequence induction (Flower and Hellings, 2012). The FDA does not recommend the prolonged use of propofol for sedation or refractory intracranial hypertension (Kochanek et al., 2019b). Other agents that can be used are succinylcholine, which is useful when there are airway complications and has greater safety than rocuronium (Hardcastle et al., 2014). Finally, it is recommended to avoid using midazolam and/or fentanyl during ICP crises because of the risk of cerebral hypoperfusion (Kochanek et al., 2019b). Another important consideration regarding long-term administration of sedatives and analgesic drugs is the risk of physiological dependence in pediatric patients (Tobias, 2000; Ista et al., 2007). Multiple studies have demonstrated that those who experience TBI during childhood and/or adolescence are more vulnerable to substance abuse disorders (Corrigan et al., 2013; Ilie et al., 2016; Cannella et al., 2019). Given this risk of dependence, clinicians should consider expeditious weaning of analgesic agents after the acute phase of injury.
Nutrition
In adults, it is recommended to use transgastric jejunal feeding (to reduce risk of ventilator-associated pneumonia) at least on the 5th day after TBI and at most on the 7th day after TBI (Carney et al., 2017). In children, it is recommended to start nutritional support within 72 h of TBI, as it is shown to approve outcomes (Kochanek et al., 2019b). It is not suggested to use immune-modulating diet in children (Kochanek et al., 2019b).
Infection Prophylaxis
Although early tracheostomy is used to reduce the number of days the patient is on mechanical ventilation (provided that the benefits outweigh the risk of complications), there is no evidence that early tracheostomy reduces rate of pneumonia or mortality (Carney et al., 2017). Reducing ventilator-associated pneumonia is not recommended since it increases risk of acute respiratory distress syndrome (Carney et al., 2017). Lastly, antimicrobial-impregnated catheters can be used during external ventricular drainage as a prevention against infections (Carney et al., 2017).
Corticosteroids
In both adults and children, the use of corticosteroids is not recommended method reduce ICP or improve outcomes (Carney et al., 2017; Kochanek et al., 2019b).
Emergent Pathway if Clinical Evidence of Rapid Neurological Deterioration
In the first days after TBI, the patient is closely monitored in the intensive care unit with multimodal monitoring. If signs or symptoms of cerebral herniation appears even without ICP elevation, such as pupillary dilation, hypertension/bradycardia, and extensor posturing (Kochanek et al., 2019a), then the following should occur: endotracheal intubation, elevation of head to 30–45°, brief hyperventilation targeting a paCO2 of 30 mmHg (Stevens et al., 2015), administration of 1–1.5 g/kg mannitol (Qureshi et al., 2000) or 23.4% sodium chloride 30–60 ml over 10 min (Koenig et al., 2008), and maintenance of mean arterial pressure (MAP) to between 80 and 100 mmHg. In children, we recommend giving 0.5–1 g/kg mannitol or hypertonic saline (1–3 ml/kg up to a maximum of 250 ml for 3% saline or 0.5 ml/kg up to a maximum of 30 ml for 23.4% saline) over 10 min (Kochanek et al., 2019a). It is recommended in children that a bolus of 3% hypertonic saline is given when there is intracranial hypertension, with the effective doses for acute use ranging between 2 and 5 ml/kg over 10–20 min (Kochanek et al., 2019b). After these steps have occurred, an emergency CT scan should be obtained to determine if emergent surgery or a ventriculostomy at the bedside is necessary (Kochanek et al., 2019a).
ICP Pathway
If the ICP of the patient is raised greater than 20 mmHg (child) or 22 mmHg (adult) for at least 5 min, then drainage of CSF should occur (Kochanek et al., 2019a). If this is not resolved, then patient should receive a bolus and/or infusion of hypertonic saline or mannitol (Kochanek et al., 2019a). For hypertonic saline, administer 3% NaCl to reach sodium concentration of 145–155 mEq/L (Qureshi et al., 1998). Also administer a bolus of 30 ml of 23.4% NaCl if there are acute ICP elevations (Ware et al., 2005). Alternatively, 0.25–1 g/kg bolus of mannitol can also be given every 4–6 h as needed (Carney et al., 2017). It is recommended that patients receiving mannitol should have their serum osmolality maintained below 320 mmol/L (Dinsmore, 2013). It is also important to prevent arterial hypotension (Carney et al., 2017). The use of mannitol should be restricted to patients who, prior to ICP monitoring, have signs of transtentorial herniation or progressive neurological deterioration that is not due to extracranial causes (Carney et al., 2017). It is recommended in children that a bolus of 3% hypertonic saline is given when there is intracranial hypertension, with the effective doses for acute use ranging between 2 and 5 ml/kg over 10–20 min (Kochanek et al., 2019b). For refractory ICP, the use of additional analgesia/sedation is recommended (Kochanek et al., 2019a). If ICP is still above threshold, then neuromuscular blockade is needed, which should be monitored with EEG (Kochanek et al., 2019a). The next line of therapy is to administer additional hypertonic saline/hyperosmolar therapy (Kochanek et al., 2019a). If the elevated ICP is still not resolved, then a CT scan should be repeated to see if hemicraniectomy is required (Kochanek et al., 2019a). As ICP improves along with clinical neurological recovery, then the patient can be weaned off ICP, CCP, and/or PrO2 therapy (Kochanek et al., 2019a).
CPP Pathway
In adults, a CPP of 60–70 mmHg is recommended (Carney et al., 2017). If the CPP of an adult is below optimal levels and if autoregulation is impaired, it is recommended for the ICP to be lowered instead of elevating mean arterial pressure (Howells et al., 2005). Whether the minimal CPP should be 60 or 70 mmHg depends on the autoregulatory status of the patient (Carney et al., 2017). Due to the risk of respiratory failure in adults, fluids and pressors can be used to maintain a CPP of 70 mmHg (Carney et al., 2017). CPP should be in between 40 and 50 mmHg in children who are 5 years old and under (Kochanek et al., 2019b). Those who are between 5 and 17 years old should have their CPP maintained above 50 mmHg (Stevens et al., 2015). If CPP decreases, the status of the intravascular volume should be checked, and vasopressor infusion and hypertonic saline bolus should be given (Kochanek et al., 2019a). However, if CPP still decreases, then repeat a CT scan to see if surgery should be considered (Kochanek et al., 2019a).
PbrO2 Pathway
Brain tissue oxygen (PbrO2) should be kept greater than 10 mmHg if PbrO2 monitor is being used. Note that some literature suggests that PbrO2 of less than 20 mmHg is considered compromised and are associated with worse outcomes (van Santbrink et al., 1996; Bardt et al., 1998; Valadka et al., 1998; van den Brink et al., 2000; Chang et al., 2009; Nangunoori et al., 2012). If PbrO2 decreases, then raise the fraction of inspired oxygen (FiO2) on the ventilator. If PbrO2 still lowers, then give vasopressor infusion, adjust PaCO2, and optimize hemoglobin levels (Kochanek et al., 2019a). If this fails, then repeat a imaging for surgical evaluation should be considered.
Second Tier Therapies
If the patient progresses to impending herniation after the ICP, CPP, and/or PrbO2 pathway, then second tier therapies should be considered. If the repeat imaging reveals a new or growing swelling or hemorrhage, then patient should undergo evacuation and decompressive craniectomy (DC) (Kochanek et al., 2019a). Bifrontal DC is not suggested in patients who have diffuse injury (without having mass lesions) and patients who have ICP elevation of greater than 20 mmHg for more than 15 min within 1 h that are refractory to first-tier therapies (Carney et al., 2017). Also, a large frontotemporoparietal (at or greater than 12 × 15 cm or 15 cm diameter) DC is suggested over a small frontotemporoparietal DC (Carney et al., 2017). It is also appropriate to administer a dose of hypertonic saline or mannitol (Kochanek et al., 2019a). It is better to avoid sodium concentrations greater than 160 mEq/L and osmolarity of greater than 360 mOsm/L (Kochanek et al., 2019a). If hyperosmolar therapy and propofol infusion (titrated to deep sedation) does not lower ICP, then consider putting patient into barbiturate coma (Eisenberg et al., 1988; Vella et al., 2017). Moderate hypothermia (between 32 and 34°) and hyperventilation can also be considered, but this has unclear benefit on overall clinical outcomes (Kochanek et al., 2019a). Advanced neuromonitoring is also recommended, such as brain tissue oxygen monitoring to help guide bedside management of ICP.
Recovery From TBI and Long-Term Outcomes
There exists difficulty identifying the outcomes that are specifically related to TBI, since trauma that causes TBI may cause multiorgan damage (Stocchetti and Zanier, 2016). However, long-term consequences of TBI include increased likelihood of mortality, vegetative status, physical disabilities, cognitive impairment, psychiatric disorders, seizures, and unemployment rate (Stocchetti and Zanier, 2016). Younger age was associated with higher likelihood of survival at follow-up in an adult trauma center patient cohort (Schroeppel et al., 2020). Based on a retrospective study on TBI outcomes in children, it was found that children under 2 years old who experienced TBI were more likely to experience vomiting and post-traumatic epilepsy as the most common symptom (Hwang et al., 2019). For children above 2 years old, the major long-term symptoms were confusion and disorientation (Hwang et al., 2019). Despite this, children were overall found to improve in functional ability after ongoing sessions of rehabilitation, which shows the resilience in children regarding recovery (Hwang et al., 2019). Overall, physical rehabilitation is helpful in children who suffered from TBI to regain back functionality. It is also found that children who had higher functional status coming into rehabilitation and shorter time between TBI and rehabilitation tend to have higher functionality during discharge and a shorter stay at rehabilitation (Rice et al., 2005). Pediatric rehabilitation not only involves physical rehabilitation, but it also involves an interdisciplinary approach where the child also works with physical and occupational therapists, psychologists, speech therapists, and allied services (Yen and Wong, 2007). This helps the child face life challenges again and gain an enriched experience, which is related to increased neural plasticity (Yen and Wong, 2007).
Discussion
In this review, we summarized the data regarding the epidemiology, classification, molecular pathogenesis, and management of TBI in children and adults. The known pathological mechanisms that lead to decreased functioning in animal models and humans after TBI include neuroinflammation, excitotoxity, oxidative stress, apoptosis, and mitochondria dysfunction. However, future studies are needed to further elucidate the pathophysiology of pediatric TBI so that more effective clinical management and therapeutics can be developed. Despite the anatomical and physiological differences between adults and pediatric populations, there is a limited amount of literature comparing the management of TBI between these two patient groups. There is a paucity of clinical studies on TBI in children compared to the adult literature. As a result, there are unclear evidence-based guidelines for parameters such as CPP and ICP. The majority of TBI guidelines for pediatric patients are adapted from the management of TBI in adults, which may be inappropriate owing to the inherent physiological and anatomical difference between these two demographics. Here we sought to highlight the differences in management of TBI between children and adults and create a clinical management algorithm (Figure 3), which builds on the recent published guidelines from Kochanek et al. (2019). Finally, we also discussed the long-term recovery and outcomes in these patients. Future prospective clinical trials dedicated to studying imaging, intensive care unit (ICU) management, and neurosurgical intervention in the pediatric population would be of tremendous value.
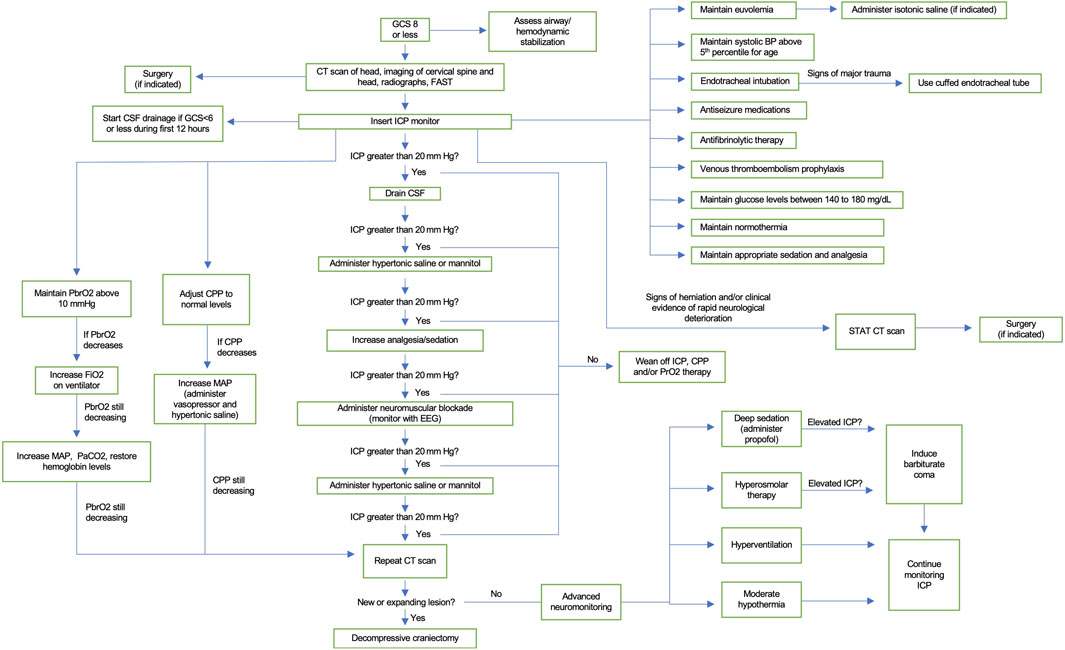
FIGURE 3. Clinical algorithm for the treatment of severe TBI in children. Baseline care includes neurological examination and assessment of airway and hemodynamic stability. Guidelines for ICP monitoring, volume status, blood pressure, endotracheal intubation, glucose management, temperature, sedation, analgesia, antiseizure medications, antifibrinolytic therapy, and venous thromboembolism prophylaxis are detailed. Uncontrolled ICP, PrbO2, or CCP will lead to a repeat imaging. If a new expanding lesion is found, then decompressive craniectomy is indicated. If negative, second tier therapies can be used, including deep sedation, hyperosmolar therapy, hyperventilation, and moderate hypothermia.
Author Contributions
AL contributed to literature search and manuscript draft. KK and GG guided, edited, and supervised preparation of the manuscript.
Conflict of Interest
The authors declare that the research was conducted in the absence of any commercial or financial relationships that could be construed as a potential conflict of interest.
Publisher’s Note
All claims expressed in this article are solely those of the authors and do not necessarily represent those of their affiliated organizations, or those of the publisher, the editors and the reviewers. Any product that may be evaluated in this article, or claim that may be made by its manufacturer, is not guaranteed or endorsed by the publisher.
Supplementary Material
The Supplementary Material for this article can be found online at: https://www.frontiersin.org/articles/10.3389/ftox.2022.910972/full#supplementary-material
References
Abdel-Aziz, H., Dunham, C. M., Malik, R. J., and Hileman, B. M. (2015). Timing for Deep Vein Thrombosis Chemoprophylaxis in Traumatic Brain Injury: an Evidence-Based Review. Crit. Care. 19 (1), 96. doi:10.1186/s13054-015-0814-z
Adamo, M. A., Drazin, D., and Waldman, J. B. (2009). Decompressive Craniectomy and Postoperative Complication Management in Infants and Toddlers with Severe Traumatic Brain Injuries. Ped. 3 (4), 334–339. doi:10.3171/2008.12.Peds08310
Adelson, P. D., Wisniewski, S. R., Beca, J., Brown, S. D., Bell, M., Muizelaar, J. P., et al. (2013). Comparison of Hypothermia and Normothermia after Severe Traumatic Brain Injury in Children (Cool Kids): a Phase 3, Randomised Controlled Trial. Lancet Neurology. 12 (6), 546–553. doi:10.1016/s1474-4422(13)70077-2
Ahn, M.-J., Sherwood, E. R., Prough, D. S., Yie Lin, C., and DeWitt, D. S. (2004). The Effects of Traumatic Brain Injury on Cerebral Blood Flow and Brain Tissue Nitric Oxide Levels and Cytokine Expression. J. Neurotrauma. 21 (10), 1431–1442. doi:10.1089/neu.2004.21.1431
Alvis-Miranda, H. R., Navas-Marrugo, S. Z., Velasquez-Loperena, R. A., Adie-Villafañe, R. J., Velasquez-Loperena, D., Castellar-Leones, S. M., et al. (2014). Effects of Glycemic Level on Outcome of Patients with Traumatic Brain Injury: A Retrospective Cohort Study. Bull. Emerg. Trauma. 2 (2), 65–71.
Amaranath, J. E., Ramanan, M., Reagh, J., Saekang, E., Prasad, N., Chaseling, R., et al. (2014). Epidemiology of Traumatic Head Injury from a Major Paediatric Trauma Centre in New South Wales, Australia. ANZ J. Surg. 84 (6), 424–428. doi:10.1111/ans.12445
Andriessen, T. M. J. C., Jacobs, B., and Vos, P. E. (2010). Clinical Characteristics and Pathophysiological Mechanisms of Focal and Diffuse Traumatic Brain Injury. J. Cell. Mol. Med. 14 (10), 2381–2392. doi:10.1111/j.1582-4934.2010.01164.x
Appelboom, G., Zoller, S. D., Piazza, M. A., Szpalski, C., Bruce, S. S., McDowell, M. M., et al. (2011). Traumatic Brain Injury in Pediatric Patients: Evidence for the Effectiveness of Decompressive Surgery. Foc. 31 (5), E5. doi:10.3171/2011.8.Focus11177
Arambula, S. E., Reinl, E. L., El Demerdash, N., McCarthy, M. M., and Robertson, C. L. (2019). Sex Differences in Pediatric Traumatic Brain Injury. Exp. Neurol. 317, 168–179. doi:10.1016/j.expneurol.2019.02.016
Aungst, S. L., Kabadi, S. V., Thompson, S. M., Stoica, B. A., and Faden, A. I. (2014). Repeated Mild Traumatic Brain Injury Causes Chronic Neuroinflammation, Changes in Hippocampal Synaptic Plasticity, and Associated Cognitive Deficits. J. Cereb. Blood Flow. Metab. 34 (7), 1223–1232. doi:10.1038/jcbfm.2014.75
Baerg, J., Thirumoorthi, A., Vannix, R., Taha, A., Young, A., and Zouros, A. (2017). Cervical Spine Imaging for Young Children with Inflicted Trauma: Expanding the Injury Pattern. J. Pediatr. Surg. 52 (5), 816–821. doi:10.1016/j.jpedsurg.2017.01.049
Bailey, B. M., Liesemer, K., Statler, K. D., Riva-Cambrin, J., and Bratton, S. L. (2012). Monitoring and Prediction of Intracranial Hypertension in Pediatric Traumatic Brain Injury. J. Trauma Acute Care Surg. 72 (1), 263–270. doi:10.1097/TA.0b013e31822a9512
Baldwin, S. A., Fugaccia, I., Brown, D. R., Brown, L. V., and Scheff, S. W. (1996). Blood-brain Barrier Breach Following Cortical Contusion in the Rat. J. Neurosurg. 85 (3), 476–481. doi:10.3171/jns.1996.85.3.0476
Bar-Joseph, G., Guilburd, Y., Tamir, A., and Guilburd, J. N. (2009). Effectiveness of Ketamine in Decreasing Intracranial Pressure in Children with Intracranial Hypertension. Ped. 4 (1), 40–46. doi:10.3171/2009.1.Peds08319
Bardt, T. F., Unterberg, A. W., Härtl, R., Kiening, K. L., Schneider, G. H., and Lanksch, W. R. (1998). Monitoring of Brain Tissue PO2 in Traumatic Brain Injury: Effect of Cerebral Hypoxia on Outcome. Acta Neurochir. Suppl. 71, 153–156. doi:10.1007/978-3-7091-6475-4_45
Başkaya, M. K., Rao, A. M., Doğan, A., Donaldson, D., and Dempsey, R. J. (1997). The Biphasic Opening of the Blood-Brain Barrier in the Cortex and hippocampus after Traumatic Brain Injury in Rats. Neurosci. Lett. 226 (1), 33–36. doi:10.1016/s0304-3940(97)00239-5
Bata, S. C., and Yung, M. (2014). Role of Routine Repeat Head Imaging in Paediatric Traumatic Brain Injury. ANZ J. Surg. 84 (6), 438–441. doi:10.1111/ans.12582
Bell, M. J., Adelson, P. D., Adelson, P. D., and Wisniewski, S. R. (2017). Challenges and Opportunities for Pediatric Severe TBI-Review of the Evidence and Exploring a Way Forward. Childs Nerv. Syst. 33 (10), 1663–1667. doi:10.1007/s00381-017-3530-y
Bell, M. J., Adelson, P. D., Hutchison, J. S., Kochanek, P. M., Tasker, R. C., Vavilala, M. S., et al. (2013). Differences in Medical Therapy Goals for Children with Severe Traumatic Brain Injury-An International Study. Pediatr. Crit. Care Med. 14 (8), 811–818. doi:10.1097/PCC.0b013e3182975e2f
Bossers, S. M., Loer, S. A., Bloemers, F. W., Den Hartog, D., Van Lieshout, E. M. M., Hoogerwerf, N., et al. (2021). Association between Prehospital Tranexamic Acid Administration and Outcomes of Severe Traumatic Brain Injury. JAMA Neurol. 78 (3), 338–345. doi:10.1001/jamaneurol.2020.4596
Bramwell, K. J., Haizlip, J., Pribble, C., VanDerHeyden, T. C., and Witte, M. (2006). The Effect of Etomidate on Intracranial Pressure and Systemic Blood Pressure in Pediatric Patients with Severe Traumatic Brain Injury. Pediatr. Emerg. Care. 22 (2), 90–93. doi:10.1097/01.pec.0000199563.64264.3a
Bratton, S. L., Chestnut, R. M., Ghajar, J., McConnell Hammond, F. F., Harris, O. A., Hartl, R., et al. (2007). I. Blood Pressure and Oxygenation. J. Neurotrauma. 24 (Suppl. 1), S–7. doi:10.1089/neu.2007.9995
Brihaye, F., Frowein, R. A., Lindgren, S., Loew, F., and Stroobandt, G. (1978). Report on the Meeting of the W.F.N.S. Neuro-Traumatology Committee, Brussels, 19–23 September 1976. Acta Neurochir. 40, 181–186. doi:10.1007/BF01773126
Brown, C. V. R., Weng, J., Oh, D., Salim, A., Kasotakis, G., Demetriades, D., et al. (2004). Does Routine Serial Computed Tomography of the Head Influence Management of Traumatic Brain Injury? A Prospective Evaluation. J. Trauma Inj. Infect. Crit. Care. 57 (5), 939–943. doi:10.1097/01.ta.0000149492.92558.03
Bruns, J., and Hauser, W. A. (2003). The Epidemiology of Traumatic Brain Injury: a Review. Epilepsia. 44 (s10), 2–10. doi:10.1046/j.1528-1157.44.s10.3.x
Buttram, S. D. W., Wisniewski, S. R., Jackson, E. K., Adelson, P. D., Feldman, K., Bayir, H., et al. (2007). Multiplex Assessment of Cytokine and Chemokine Levels in Cerebrospinal Fluid Following Severe Pediatric Traumatic Brain Injury: Effects of Moderate Hypothermia. J. Neurotrauma. 24 (11), 1707–1718. doi:10.1089/neu.2007.0349
Bye, N., Habgood, M. D., Callaway, J. K., Malakooti, N., Potter, A., Kossmann, T., et al. (2007). Transient Neuroprotection by Minocycline Following Traumatic Brain Injury Is Associated with Attenuated Microglial Activation but No Changes in Cell Apoptosis or Neutrophil Infiltration. Exp. Neurol. 204 (1), 220–233. doi:10.1016/j.expneurol.2006.10.013
Cannella, L. A., McGary, H., and Ramirez, S. H. (2019). Brain Interrupted: Early Life Traumatic Brain Injury and Addiction Vulnerability. Exp. Neurol. 317, 191–201. doi:10.1016/j.expneurol.2019.03.003
Capizzi, A., Woo, J., and Verduzco-Gutierrez, M. (2020). Traumatic Brain Injury. Med. Clin. N. Am. 104 (2), 213–238. doi:10.1016/j.mcna.2019.11.001
Carlos, T. M., Clark, R. S. B., Franicola-Higgins, D., Schiding, J. K., and Kochanek, P. M. (1997). Expression of Endothelial Adhesion Molecules and Recruitment of Neutrophils after Traumatic Brain Injury in Rats. J. Leukoc. Biol. 61 (3), 279–285. doi:10.1002/jlb.61.3.279
Carney, N., Totten, A. M., O'Reilly, C., Ullman, J. S., Hawryluk, G. W. J., Bell, M. J., et al. (2017). Guidelines for the Management of Severe Traumatic Brain Injury, Fourth Edition. Neurosurgery. 80 (1), 6–15. doi:10.1227/neu.0000000000001432
Chamoun, R., Suki, D., Gopinath, S. P., Goodman, J. C., and Robertson, C. (2010). Role of Extracellular Glutamate Measured by Cerebral Microdialysis in Severe Traumatic Brain Injury. Jns. 113 (3), 564–570. doi:10.3171/2009.12.Jns09689
Chang, J. J. J., Youn, T. S., Benson, D., Mattick, H., Andrade, N., Harper, C. R., et al. (2009). Physiologic and Functional Outcome Correlates of Brain Tissue Hypoxia in Traumatic Brain Injury*. Crit. Care Med. 37 (1), 283–290. doi:10.1097/CCM.0b013e318192fbd7
Chau, C. Y. C., Craven, C. L., Rubiano, A. M., Adams, H., Tülü, S., Czosnyka, M., et al. (2019). The Evolution of the Role of External Ventricular Drainage in Traumatic Brain Injury. Jcm. 8 (9), 1422. doi:10.3390/jcm8091422
Chau, C. Y. C., Mediratta, S., McKie, M. A., Gregson, B., Tulu, S., Ercole, A., et al. (2020). Optimal Timing of External Ventricular Drainage after Severe Traumatic Brain Injury: A Systematic Review. Jcm. 9 (6), 1996. doi:10.3390/jcm9061996
Chiu, C.-C., Liao, Y.-E., Yang, L.-Y., Wang, J.-Y., Tweedie, D., Karnati, H. K., et al. (2016). Neuroinflammation in Animal Models of Traumatic Brain Injury. J. Neurosci. Methods. 272, 38–49. doi:10.1016/j.jneumeth.2016.06.018
Chodobski, A., Zink, B. J., and Szmydynger-Chodobska, J. (2011). Blood-brain Barrier Pathophysiology in Traumatic Brain Injury. Transl. Stroke Res. 2 (4), 492–516. doi:10.1007/s12975-011-0125-x
Choudhary, A. K., Ishak, R., Zacharia, T. T., and Dias, M. S. (2014). Imaging of Spinal Injury in Abusive Head Trauma: a Retrospective Study. Pediatr. Radiol. 44 (9), 1130–1140. doi:10.1007/s00247-014-2959-3
Control, C. f. D. (2014). Traumatic Brain Injury and Concussion. Atlanta: Centers for Disease Control and Prevention.
Cooper, K. D., Tabaddor, K., Hauser, W. A., Shulman †, K., Feiner, C., and Factor, P. R. (1983). The Epidemiology of Head Injury in the Bronx; Pp. 70-78. Neuroepidemiology. 2, 70–78. doi:10.1159/000110512
Corrigan, J. D., Bogner, J., Mellick, D., Bushnik, T., Dams-O'Connor, K., Hammond, F. M., et al. (2013). Prior History of Traumatic Brain Injury Among Persons in the Traumatic Brain Injury Model Systems National Database. Archives Phys. Med. Rehabilitation. 94 (10), 1940–1950. doi:10.1016/j.apmr.2013.05.018
Corso, P., Finkelstein, E., Miller, T., Fiebelkorn, I., and Zaloshnja, E. (2006). Incidence and Lifetime Costs of Injuries in the United States. Inj. Prev. 12 (4), 212–218. doi:10.1136/ip.2005.010983
Covassin, T., and Elbin, R. J. (2011). The Female Athlete: the Role of Gender in the Assessment and Management of Sport-Related Concussion. Clin. Sports Med. 30 (1), 125–131. x. doi:10.1016/j.csm.2010.08.001
Crompton, E. M., Lubomirova, I., Cotlarciuc, I., Han, T. S., Sharma, S. D., and Sharma, P. (2017). Meta-Analysis of Therapeutic Hypothermia for Traumatic Brain Injury in Adult and Pediatric Patients*. Crit. Care Med. 45 (4), 575–583. doi:10.1097/ccm.0000000000002205
Cuccurullo, S. (2010). “Trauamatic Brain Injury,” in Physical Medicine and Rehabilitation Board Reivew. 2 ed (New York: demosMedical).
da Silva, P. S. L., Reis, M. E., and Aguiar, V. E. (2008). Value of Repeat Cranial Computed Tomography in Pediatric Patients Sustaining Moderate to Severe Traumatic Brain Injury. J. Trauma. 65 (6), 1293–1297. doi:10.1097/TA.0b013e318156866c
Deakers, T. W., Reynolds, G., Stretton, M., and Newth, C. J. L. (1994). Cuffed Endotracheal Tubes in Pediatric Intensive Care. J. Pediatr. 125 (1), 57–62. doi:10.1016/s0022-3476(94)70121-0
Defense, V. A. D. o. (2016). Clinical Practice Guideline for the Management of Concussion-Mild Traumatic Brain Injury. Washington, DC: Veterans Health Administration.
Dellazizzo, L., Demers, S.-P., Charbonney, E., Williams, V., Serri, K., Albert, M., et al. (2019). Minimal PaO2 Threshold after Traumatic Brain Injury and Clinical Utility of a Novel Brain Oxygenation Ratio. J. Neurosurg. 131, 1639–1647. doi:10.3171/2018.5.Jns18651
Dewan, M. C., Mummareddy, N., Wellons, J. C., and Bonfield, C. M. (2016). Epidemiology of Global Pediatric Traumatic Brain Injury: Qualitative Review. World Neurosurg. 91, 497–509.e491. doi:10.1016/j.wneu.2016.03.045
Dick, R. W. (2009). Is There a Gender Difference in Concussion Incidence and Outcomes? Br. J. Sports Med. 43 (Suppl. 1), i46–i50. doi:10.1136/bjsm.2009.058172
Dinsmore, J. (2013). Traumatic Brain Injury: an Evidence-Based Review of Management. Continuing Educ. Anaesth. Crit. Care & Pain. 13 (6), 189–195. doi:10.1093/bjaceaccp/mkt010
Diringer, M. N., Yundt, K., Videen, T. O., Adams, R. E., Zazulia, A. R., Deibert, E., et al. (2000). No Reduction in Cerebral Metabolism as a Result of Early Moderate Hyperventilation Following Severe Traumatic Brain Injury. J. Neurosurg. 92 (1), 7–13. doi:10.3171/jns.2000.92.1.0007
Dixon, K. J. (2017). Pathophysiology of Traumatic Brain Injury. Phys. Med. Rehabilitation Clin. N. Am. 28 (2), 215–225. doi:10.1016/j.pmr.2016.12.001
Eapen, B. C., and Cifu, D. X. (2018). Rehabilitation after Traumatic Brain Injury. Elsevier Health Sciences.
Eisenberg, H. M., Frankowski, R. F., Contant, C. F., Marshall, L. F., Walker, M. D., and _, _. (1988). High-dose Barbiturate Control of Elevated Intracranial Pressure in Patients with Severe Head Injury. J. Neurosurg. 69 (1), 15–23. doi:10.3171/jns.1988.69.1.0015
Elovic, B., and Galang, C. (2010). “Traumatic Brain Injury,” in Physical Medicine and Rehabilitation Board Review (New York: Demos Medical Publishing). SJ. 2.
Esparza, J., M-Portillo, J., Sarabia, M., Yuste, J. A., Roger, R., and Lamas, E. (1985). Outcome in Children with Severe Head Injuries. Child's Nerv. Syst. 1 (2), 109–114. doi:10.1007/bf00706691
Farling, P. A., Johnston, J. R., and Coppel, D. L. (1989). Propofol Infusion for Sedation of Patients with Head Injury in Intensive Care. Anaesthesia. 44 (3), 222–226. doi:10.1111/j.1365-2044.1989.tb11228.x
Figaji, A. A., Fieggen, A. G., Argent, A., and Peter, J. C. (2006). Surgical Treatment for "brain Compartment Syndrome" in Children with Severe Head Injury. S Afr. Med. J. 96 (9 Pt 2), 969–975.
Figaji, A. A. (2017). Anatomical and Physiological Differences between Children and Adults Relevant to Traumatic Brain Injury and the Implications for Clinical Assessment and Care. Front. Neurol. 8, 685. doi:10.3389/fneur.2017.00685
Figaji, A. A., Fieggen, A. G., Argent, A. C., Le Roux, P. D., and Peter, J. C. (2008). Intracranial Pressure and Cerebral Oxygenation Changes after Decompressive Craniectomy in Children with Severe Traumatic Brain Injury. Acta Neurochir. Suppl. 102, 77–80. doi:10.1007/978-3-211-85578-2_15
Figaji, A. A., Fieggen, A. G., and Peter, J. C. (2003). Early Decompressive Craniotomy in Children with Severe Traumatic Brain Injury. Child's Nerv. Syst. 19 (9), 666–673. doi:10.1007/s00381-003-0804-3
Figg, R. E., Stouffer, C. W., Vander Kolk, W. E., and Connors, R. H. (2006). Clinical Efficacy of Serial Computed Tomographic Scanning in Pediatric Severe Traumatic Brain Injury. Ped Surg. Int. 22 (3), 215–218. doi:10.1007/s00383-005-1560-0
Fitch, M. T., and Silver, J. (2008). CNS Injury, Glial Scars, and Inflammation: Inhibitory Extracellular Matrices and Regeneration Failure. Exp. Neurol. 209 (2), 294–301. doi:10.1016/j.expneurol.2007.05.014
Flower, O., and Hellings, S. (2012). Sedation in Traumatic Brain Injury. Emerg. Med. Int. 2012, 1–11. doi:10.1155/2012/637171
Frugier, T., Morganti-Kossmann, M. C., O'Reilly, D., and McLean, C. A. (2010). In Situ detection of Inflammatory Mediators in Post Mortem Human Brain Tissue after Traumatic Injury. J. Neurotrauma. 27 (3), 497–507. doi:10.1089/neu.2009.1120
Gerstenbrand, F., and Lücking, C. H. (1970). Die Akuten Traumatischen Hirnstammschäden. Arch. Psychiat. Nervenkr. 213, 264–281. doi:10.1007/bf00342662
Gibbs, J. M. (1972). The Effect of Intravenous Ketamine on Cerebrospinal Fluid Pressure. Br. J. Anaesth. 44 (12), 1298–1302. doi:10.1093/bja/44.12.1298
Goodman, J. C., Van, M., Gopinath, S. P., and Robertson, C. S. (2008). Pro-inflammatory and Pro-apoptotic Elements of the Neuroinflammatory Response Are Activated in Traumatic Brain Injury. Acta Neurochir. Suppl. 102, 437–439. doi:10.1007/978-3-211-85578-2_85
Greene, N. H., Kernic, M. A., Vavilala, M. S., and Rivara, F. P. (2014). Variation in Pediatric Traumatic Brain Injury Outcomes in the United States. Archives Phys. Med. Rehabilitation. 95 (6), 1148–1155. doi:10.1016/j.apmr.2014.02.020
Hardcastle, N., Benzon, H. A., and Vavilala, M. S. (2014). Update on the 2012 Guidelines for the Management of Pediatric Traumatic Brain Injury - Information for the Anesthesiologist. Paediatr. Anaesth. 24 (7), 703–710. doi:10.1111/pan.12415
Hawryluk, G. W. J., and Manley, G. T. (2015). Classification of Traumatic Brain Injury. Handb. Clin. Neurology,Traumatic Brain Inj. Part I., 15–21. Elsevier. doi:10.1016/b978-0-444-52892-6.00002-7
Henry, M. K., French, B., Feudtner, C., Zonfrillo, M. R., Lindberg, D. M., Anderst, J. D., et al. (2018). Cervical Spine Imaging and Injuries in Young Children with Non-motor Vehicle Crash-Associated Traumatic Brain Injury. Pediatr. Emerg. Care. (1), e1–e6. Publish Ahead of Print. doi:10.1097/pec.0000000000001455
Hicks, R. R., Baldwin, S. A., and Scheff, S. W. (1997). Serum Extravasation and Cytoskeletal Alterations Following Traumatic Brain Injury in Rats. Mol. Chem. Neuropathology. 32 (1-3), 1–16. doi:10.1007/bf02815164
Howells, T., Elf, K., Jones, P. A., Ronne-Engström, E., Piper, I., Nilsson, P., et al. (2005). Pressure Reactivity as a Guide in the Treatment of Cerebral Perfusion Pressure in Patients with Brain Trauma. J. Neurosurg. 102 (2), 311–317. doi:10.3171/jns.2005.102.2.0311
Hutchison, J. S., Ward, R. E., Lacroix, J., Hébert, P. C., Barnes, M. A., Bohn, D. J., et al. (2008). Hypothermia Therapy after Traumatic Brain Injury in Children. N. Engl. J. Med. 358 (23), 2447–2456. doi:10.1056/NEJMoa0706930
Hwang, S. Y., Ong, J. W., Ng, Z. M., Foo, C. Y., Chua, S. Z., Sri, D., et al. (2019). Long-term Outcomes in Children with Moderate to Severe Traumatic Brain Injury: a Single-Centre Retrospective Study. Brain Inj. 33 (11), 1420–1424. doi:10.1080/02699052.2019.1641625
Ilie, G., Mann, R. E., Boak, A., Adlaf, E. M., Hamilton, H., Asbridge, M., et al. (2016). Cross-sectional Examination of the Association of Co-occurring Alcohol Misuse and Traumatic Brain Injury on Mental Health and Conduct Problems in Adolescents in Ontario, Canada. BMJ Open. 6 (11), e011824. doi:10.1136/bmjopen-2016-011824
Ista, E., van Dijk, M., Gamel, C., Tibboel, D., and de Hoog, M. (2007). Withdrawal Symptoms in Children after Long-Term Administration of Sedatives And/or Analgesics: a Literature Review. "Assessment Remains Troublesome". Intensive Care Med. 33 (8), 1396–1406. doi:10.1007/s00134-007-0696-x
Jagannathan, J., Okonkwo, D. O., Dumont, A. S., Ahmed, H., Bahari, A., Prevedello, D. M., et al. (2007). Outcome Following Decompressive Craniectomy in Children with Severe Traumatic Brain Injury: a 10-year Single-Center Experience with Long-Term Follow up. J. Neurosurg. Pediatr. 106 (4 Suppl. l), 268–275. doi:10.3171/ped.2007.106.4.268
Jagannathan, J., Okonkwo, D. O., Yeoh, H. K., Dumont, A. S., Saulle, D., Haizlip, J., et al. (2008). Long-term Outcomes and Prognostic Factors in Pediatric Patients with Severe Traumatic Brain Injury and Elevated Intracranial Pressure. Ped. 2 (4), 240–249. doi:10.3171/ped.2008.2.10.240
Johnston, K. C., Bruno, A., Pauls, Q., Hall, C. E., Barrett, K. M., Barsan, W., et al. (2019). Intensive vs Standard Treatment of Hyperglycemia and Functional Outcome in Patients with Acute Ischemic Stroke. Jama. 322 (4), 326–335. doi:10.1001/jama.2019.9346
Joseph, B., Pandit, V., Meyer, D., Butvidas, L., Kulvatunyou, N., Khalil, M., et al. (2014). The Significance of Platelet Count in Traumatic Brain Injury Patients on Antiplatelet Therapy. J. Trauma Acute Care Surg. 77 (3), 417–421. doi:10.1097/ta.0000000000000372
Kamal, H. M., Sammou, H., Mardini, A. A., and Zaitoni, A. (2011). Fall of Platelet Count in Children with Traumatic Brain Injury: Is it of Value? Chin. J. Traumatol. 14 (6), 336–342.
Kan, P., Amini, A., Hansen, K., White, G. L., Brockmeyer, D. L., Walker, M. L., et al. (2006). Outcomes after Decompressive Craniectomy for Severe Traumatic Brain Injury in Children. J. Neurosurg. Pediatr. 105 (5 Suppl. l), 337–342. doi:10.3171/ped.2006.105.5.337
Keenan, H. T., and Bratton, S. L. (2006). Epidemiology and Outcomes of Pediatric Traumatic Brain Injury. Dev. Neurosci. 28 (4-5), 256–263. doi:10.1159/000094152
Ketharanathan, N., Yamamoto, Y., Rohlwink, U., Wildschut, E. D., Hunfeld, M., De Lange, E. C. M., et al. (2017). Analgosedation in Paediatric Severe Traumatic Brain Injury (TBI): Practice, Pitfalls and Possibilities. Childs Nerv. Syst. 33 (10), 1703–1710. doi:10.1007/s00381-017-3520-0
Khine, H. H., Corddry, D. H., Kettrick, R. G., Martin, T. M., McCloskey, J. J., Rose, J. B., et al. (1997). Comparison of Cuffed and Uncuffed Endotracheal Tubes in Young Children during General Anesthesia. Anesthesiology. 86 (3), 627–631. discussion 627A. doi:10.1097/00000542-199703000-00015
Kim, H. B., Kim, D. K., Kwak, Y. H., Shin, S. D., Song, K. J., Lee, S. C., et al. (2012). Epidemiology of Traumatic Head Injury in Korean Children. J. Korean Med. Sci. 27 (4), 437–442. doi:10.3346/jkms.2012.27.4.437
Kleinman, M. E., Chameides, L., Schexnayder, S. M., Samson, R. A., Hazinski, M. F., Atkins, D. L., et al. (2010). Part 14: Pediatric Advanced Life Support. Circulation. 122 (18 Suppl. 3), S876–S908. doi:10.1161/circulationaha.110.971101
Kochanek, P. M., Carney, N., Adelson, P. D., Ashwal, S., Bell, M. J., Bratton, S., et al. (2012). Guidelines for the Acute Medical Management of Severe Traumatic Brain Injury in Infants, Children, and Adolescents-Second Edition. Pediatr. Crit. Care Med. 13 (Suppl. 1), S1–S2. doi:10.1097/PCC.0b013e31823f435c
Kochanek, P. M., Tasker, R. C., Bell, M. J., Adelson, P. D., Carney, N., Vavilala, M. S., et al. (2019a). Management of Pediatric Severe Traumatic Brain Injury. Pediatr. Crit. Care Med. 20 (3), 269–279. doi:10.1097/pcc.0000000000001737
Kochanek, P. M., Tasker, R. C., Carney, N., Totten, A. M., Adelson, P. D., Selden, N. R., et al. (2019b). Guidelines for the Management of Pediatric Severe Traumatic Brain Injury, Third Edition: Update of the Brain Trauma Foundation Guidelines, Executive Summary. Neurosurg. 84 (6), 1169–1178. doi:10.1093/neuros/nyz051
Kochanek, P. M. T. R. C. B., Michael, J., and David, A. P. (2019c). “Management of Pediatric Severe Traumatic Brain Injury: 2019 Consensus and Guidelines-Based Algorithm for First and Second Tier Therapies,” in Pediatric Critical Care Medicine: Wolters Kluwer Health. Editors A. J. Houtrow, K. P. Murphy, and M. A. McMahon (Springer Publishing Company).
Koenig, M. A., Bryan, M., Lewin, J. L., Mirski, M. A., Geocadin, R. G., and Stevens, R. D. (2008). Reversal of Transtentorial Herniation with Hypertonic Saline. Neurology. 70 (13), 1023–1029. doi:10.1212/01.wnl.0000304042.05557.60
Kossmann, T., Stahel, P. F., Lenzlinger, P. M., Redl, H., Dubs, R. W., Trentz, O., et al. (1997). Interleukin-8 Released into the Cerebrospinal Fluid after Brain Injury Is Associated with Blood-Brain Barrier Dysfunction and Nerve Growth Factor Production. J. Cereb. Blood Flow. Metab. 17 (3), 280–289. doi:10.1097/00004647-199703000-00005
Lamade, A. M., Anthonymuthu, T. S., Hier, Z. E., Gao, Y., Kagan, V. E., and Bayır, H. (2020). Mitochondrial Damage & Lipid Signaling in Traumatic Brain Injury. Exp. Neurol. 329, 113307. doi:10.1016/j.expneurol.2020.113307
Langlois, J. A., and Thomas, K. E.NationalCenters for Disease Control and Prevention (2004). Traumatic Brain Injury in the United States: Emergency Department Visits, Hospitalizations, and Deaths. Atlanta: Centers for Disease Control and Prevention, National Center for Injury Prevention and Control. N.C.f.I.P.a.C.
Lewis, S. R., Evans, D. J., Butler, A. R., Schofield-Robinson, O. J., and Alderson, P. (2017). Hypothermia for Traumatic Brain Injury. Cochrane Database Syst. Rev. 2017 (9), Cd001048. doi:10.1002/14651858.CD001048.pub5
Lincoln, A. E., Caswell, S. V., Almquist, J. L., Dunn, R. E., Norris, J. B., and Hinton, R. Y. (2011). Trends in Concussion Incidence in High School Sports. Am. J. Sports Med. 39 (5), 958–963. doi:10.1177/0363546510392326
Liu, Y.-l., Xu, Z.-m., Yang, G.-y., Yang, D.-x., Ding, J., Chen, H., et al. (2017). Sesamin Alleviates Blood-Brain Barrier Disruption in Mice with Experimental Traumatic Brain Injury. Acta Pharmacol. Sin. 38 (11), 1445–1455. doi:10.1038/aps.2017.103
Lotocki, G., de Rivero Vaccari, J. P., Perez, E. R., Sanchez-Molano, J., Furones-Alonso, O., Bramlett, H. M., et al. (2009). Alterations in Blood-Brain Barrier Permeability to Large and Small Molecules and Leukocyte Accumulation after Traumatic Brain Injury: Effects of Post-traumatic Hypothermia. J. Neurotrauma. 26 (7), 1123–1134. doi:10.1089/neu.2008.0802
Majdan, M., Mauritz, W., Rusnak, M., Brazinova, A., Rehorcikova, V., and Leitgeb, J. (2014). Long-term Trends and Patterns of Fatal Traumatic Brain Injuries in the Pediatric and Adolescent Population of austria in 1980-2012: Analysis of 33 Years. J. Neurotrauma. 31 (11), 1046–1055. doi:10.1089/neu.2013.3200
Marar, M., McIlvain, N. M., Fields, S. K., and Comstock, R. D. (2012). Epidemiology of Concussions Among United States High School Athletes in 20 Sports. Am. J. Sports Med. 40 (4), 747–755. doi:10.1177/0363546511435626
Marehbian, J., Muehlschlegel, S., Edlow, B. L., Hinson, H. E., and Hwang, D. Y. (2017). Medical Management of the Severe Traumatic Brain Injury Patient. Neurocrit Care. 27 (3), 430–446. doi:10.1007/s12028-017-0408-5
Michaud, L. J., Rivara, F. P., Grady, M. S., and Reay, D. T. (1992). Predictors of Survival and Severity of Disability after Severe Brain Injury in Children. Neurosurgery. 31 (2), 254–264. doi:10.1227/00006123-199208000-0001010.1097/00006123-199208000-00010
Mohseni-Bod, H., Drake, J., and Kukreti, V. (2014). Management of Raised Intracranial Pressure in Children with Traumatic Brain Injury. J. Pediatr. Neurosci. 9 (3), 207–215. doi:10.4103/1817-1745.147572
Myburgh, J., Cooper, D. J., Finfer, S., Bellomo, R., Myburgh, J., Cooper, D. J., et al. (2007). Saline or Albumin for Fluid Resuscitation in Patients with Traumatic Brain Injury. N. Engl. J. Med. 357 (9), 874–884. doi:10.1056/NEJMoa067514
Nangunoori, R., Maloney-Wilensky, E., Stiefel, M., Park, S., Andrew Kofke, W., Levine, J. M., et al. (2012). Brain Tissue Oxygen-Based Therapy and Outcome after Severe Traumatic Brain Injury: a Systematic Literature Review. Neurocrit Care. 17 (1), 131–138. doi:10.1007/s12028-011-9621-9
Ng, S. Y., and Lee, A. Y. W. (2019). Traumatic Brain Injuries: Pathophysiology and Potential Therapeutic Targets. Front. Cell. Neurosci. 13, 528. doi:10.3389/fncel.2019.00528
Oh, A., Sawvel, M., Heaner, D., Bhatia, A., Reisner, A., Tubbs, R. S., et al. (2017). Changes in Use of Cervical Spine Magnetic Resonance Imaging for Pediatric Patients with Nonaccidental Trauma. J. Neurosurg. Pediatr. 20 (3), 271–277. doi:10.3171/2017.2.Peds16644
Okonkwo, D. O., Yue, J. K., Puccio, A. M., Panczykowski, D. M., Inoue, T., McMahon, P. J., et al. (2013). GFAP-BDP as an Acute Diagnostic Marker in Traumatic Brain Injury: Results from the Prospective Transforming Research and Clinical Knowledge in Traumatic Brain Injury Study. J. Neurotrauma. 30 (17), 1490–1497. doi:10.1089/neu.2013.2883
Pearn, M. L., Niesman, I. R., Egawa, J., Sawada, A., Almenar-Queralt, A., Shah, S. B., et al. (2017). Pathophysiology Associated with Traumatic Brain Injury: Current Treatments and Potential Novel Therapeutics. Cell. Mol. Neurobiol. 37 (4), 571–585. doi:10.1007/s10571-016-0400-1
Peterson, A. (2021). Traumatic Brain Injury-Related Hospitalizations and Deaths by Age Group, Sex, and Mechanism of Injury. Atlanta, GA: CDC.
Pinaud, M., Lelausque, J.-N., Chetanneau, A., Fauchoux, N., Ménégalli, D., and Souron, R. (1990). Effects of Propofol on Cerebral Hemodynamics and Metabolism in Patients with Brain Trauma. Anesthesiology. 73 (3), 404–409. doi:10.1097/00000542-199009000-00007
Podolsky-Gondim, G. G., Furlanetti, L. L., Viana, D. C., Ballestero, M. F. M., and de Oliveira, R. S. (2018). The Role of Coagulopathy on Clinical Outcome Following Traumatic Brain Injury in Children: Analysis of 66 Consecutive Cases in a Single Center Institution. Childs Nerv. Syst. 34 (12), 2455–2461. doi:10.1007/s00381-018-3989-1
Puccio, A. M., Fischer, M. R., Jankowitz, B. T., Yonas, H., Darby, J. M., and Okonkwo, D. O. (2009). Induced Normothermia Attenuates Intracranial Hypertension and Reduces Fever Burden after Severe Traumatic Brain Injury. Neurocrit Care. 11 (1), 82–87. doi:10.1007/s12028-009-9213-0
Qureshi, A. I., Suarez, J. I., Bhardwaj, A., Mirski, M., Schnitzer, M. S., Hanley, D. F., et al. (1998). Use of Hypertonic (3%) Saline/acetate Infusion in the Treatment of Cerebral Edema: Effect on Intracranial Pressure and Lateral Displacement of the Brain. Crit. Care Med. 26 (3), 440–446. doi:10.1097/00003246-199803000-00011
Qureshi, A. I., Geocadin, R. G., Suarez, J. I., and Ulatowski, J. A. (2000). Long-term Outcome after Medical Reversal of Transtentorial Herniation in Patients with Supratentorial Mass Lesions. Crit. Care Med. 28 (5), 1556–1564. doi:10.1097/00003246-200005000-00049
Rajajee, V., Riggs, B., and Seder, D. B. (2017). Emergency Neurological Life Support: Airway, Ventilation, and Sedation. Neurocrit Care. 27 (Suppl. 1), 4–28. doi:10.1007/s12028-017-0451-2
Rancan, M., Otto, V. I., Hans, V. H. J., Gerlach, I., Jork, R., Trentz, O., et al. (2001). Upregulation of ICAM-1 and MCP-1 but Not of MIP-2 and Sensorimotor Deficit in Response to Traumatic Axonal Injury in Rats. J. Neurosci. Res. 63 (5), 438–446. doi:10.1002/1097-4547(20010301)63:5<438::aid-jnr1039>3.0.co;2-p
Rangel-Castillo, L., Gopinath, S., and Robertson, C. S. (2008). Management of Intracranial Hypertension. Neurol. Clin. 26 (2), 521–541. x. doi:10.1016/j.ncl.2008.02.003
Rice, S. A., Blackman, J. A., Braun, S., Linn, R. T., Granger, C. V., and Wagner, D. P. (2005). Rehabilitation of Children with Traumatic Brain Injury: Descriptive Analysis of a Nationwide Sample Using the WeeFIM. Archives Phys. Med. Rehabilitation. 86 (4), 834–836. doi:10.1016/j.apmr.2004.11.006
Rosenthal, J. A., Foraker, R. E., Collins, C. L., and Comstock, R. D. (2014). National High School Athlete Concussion Rates from 2005-2006 to 2011-2012. Am. J. Sports Med. 42 (7), 1710–1715. doi:10.1177/0363546514530091
Rutigliano, D., Egnor, M. R., Priebe, C. J., McCormack, J. E., Strong, N., Scriven, R. J., et al. (2006). Decompressive Craniectomy in Pediatric Patients with Traumatic Brain Injury with Intractable Elevated Intracranial Pressure. J. Pediatr. Surg. 41 (1), 83–87. discussion 83-87. doi:10.1016/j.jpedsurg.2005.10.010
Saatman, K. E., Duhaime, A.-C., Bullock, R., Maas, A. I. R., Valadka, A., and Manley, G. T. (2008). Classification of Traumatic Brain Injury for Targeted Therapies. J. Neurotrauma. 25 (7), 719–738. doi:10.1089/neu.2008.0586
Samant, U. B., Mack, C. D., Koepsell, T., Rivara, F. P., and Vavilala, M. S. (2008). Time of Hypotension and Discharge Outcome in Children with Severe Traumatic Brain Injury. J. Neurotrauma. 25 (5), 495–502. doi:10.1089/neu.2007.0491
Scherzer, D., Leder, M., and Tobias, J. D. (2012). Pro-con Debate: Etomidate or Ketamine for Rapid Sequence Intubation in Pediatric Patients. J. Pediatr. Pharmacol. Ther. 17 (2), 142–149. doi:10.5863/1551-6776-17.2.142
Schizodimos, T., Soulountsi, V., Iasonidou, C., and Kapravelos, N. (2020). An Overview of Management of Intracranial Hypertension in the Intensive Care Unit. J. Anesth. 34 (5), 741–757. doi:10.1007/s00540-020-02795-7
Schneier, A. J., Shields, B. J., Hostetler, S. G., Xiang, H., and Smith, G. A. (2006). Incidence of Pediatric Traumatic Brain Injury and Associated Hospital Resource Utilization in the United States. Pediatrics. 118 (2), 483–492. doi:10.1542/peds.2005-2588
Schroeppel, T. J., Sharpe, J. P., Melendez, C. I., Jepson, B., Dunn, R., Paige Clement, L., et al. (2020). Long-Term Analysis of Functional Outcomes in Traumatic Brain Injury Patients. Am. Surg. 86 (9), 1124–1128. doi:10.1177/0003134820943648
Semple, B. D., Bye, N., Rancan, M., Ziebell, J. M., and Morganti-Kossmann, M. C. (2010). Role of CCL2 (MCP-1) in Traumatic Brain Injury (TBI): Evidence from Severe TBI Patients and CCL2−/− Mice. J. Cereb. Blood Flow. Metab. 30 (4), 769–782. doi:10.1038/jcbfm.2009.262
Shapira, Y., Setton, D., Artru, A. A., and Shohami, E. (1993). Blood-brain Barrier Permeability, Cerebral Edema, and Neurologic Function after Closed Head Injury in Rats. Anesth. Analgesia. 77 (1), 141–148. doi:10.1213/00000539-199307000-00028
Sharma, D., and Vavilala, M. S. (2012). Perioperative Management of Adult Traumatic Brain Injury. Anesthesiol. Clin. 30 (2), 333–346. doi:10.1016/j.anclin.2012.04.003
Simon, D. W., McGeachy, M. J., Bayır, H., Clark, R. S. B., Loane, D. J., and Kochanek, P. M. (2017). The Far-Reaching Scope of Neuroinflammation after Traumatic Brain Injury. Nat. Rev. Neurol. 13 (3), 171–191. doi:10.1038/nrneurol.2017.13
Slomski, A. (2019). Tranexamic Acid Improves Outcomes in TBI. Jama. 322 (24), 2375. doi:10.1001/jama.2019.20077
Stanley, R. M., Bonsu, B. K., Zhao, W., Ehrlich, P. F., Rogers, A. J., and Xiang, H. (2012). US Estimates of Hospitalized Children with Severe Traumatic Brain Injury: Implications for Clinical Trials. Pediatrics. 129 (1), e24–e30. doi:10.1542/peds.2011-2074
Stevens, R. D., Shoykhet, M., and Cadena, R. (2015). Emergency Neurological Life Support: Intracranial Hypertension and Herniation. Neurocrit Care. 23 (Suppl. 2Suppl 2), 76–82. doi:10.1007/s12028-015-0168-z
Stocchetti, N., Maas, A. I. R., Chieregato, A., and van der Plas, A. A. (2005). Hyperventilation in Head Injury. Chest. 127 (5), 1812–1827. doi:10.1378/chest.127.5.1812
Stocchetti, N., and Zanier, E. R. (2016). Chronic Impact of Traumatic Brain Injury on Outcome and Quality of Life: a Narrative Review. Crit. Care. 20 (1), 148. doi:10.1186/s13054-016-1318-1
Suárez, E. P., González, A. S., Díaz, C. P., Salido, A. G., de Azagra Garde, A. M., and Flores, J. C. (2011). Decompressive Craniectomy in 14 Children with Severe Head Injury: Clinical Results with Long-Term Follow-Up and Review of the Literature. J. Trauma. 71 (1), 133–140. doi:10.1097/TA.0b013e318211071f
Sulhan, S., Lyon, K. A., Shapiro, L. A., and Huang, J. H. (2020). Neuroinflammation and Blood-Brain Barrier Disruption Following Traumatic Brain Injury: Pathophysiology and Potential Therapeutic Targets. J. Neurosci. Res. 98 (1), 19–28. doi:10.1002/jnr.24331
Suttipongkaset, P., Chaikittisilpa, N., Vavilala, M. S., Lele, A. V., Watanitanon, A., Chandee, T., et al. (2018). Blood Pressure Thresholds and Mortality in Pediatric Traumatic Brain Injury. Pediatrics. 142 (2). doi:10.1542/peds.2018-0594
Szabó, I., and Zoratti, M. (1991). The Giant Channel of the Inner Mitochondrial Membrane Is Inhibited by Cyclosporin A. J. Biol. Chem. 266 (6), 3376–3379.
Tabish, A., Lone, N. A., Afzal, W. M., and Salam, A. (2006). The Incidence and Severity of Injury in Children Hospitalised for Traumatic Brain Injury in Kashmir. Injury. 37 (5), 410–415. doi:10.1016/j.injury.2006.01.039
Tabori, U., Kornecki, A., Sofer, S., Constantini, S., Paret, G., Beck, R., et al. (2000). Repeat Computed Tomographic Scan within 24-48 Hours of Admission in Children with Moderate and Severe Head Trauma. Crit. Care Med. 28 (3), 840–844. doi:10.1097/00003246-200003000-00038
Taylor, A., Butt, W., Rosenfeld, J., Shann, F., Ditchfield, M., Lewis, E., et al. (2001). A Randomized Trial of Very Early Decompressive Craniectomy in Children with Traumatic Brain Injury and Sustained Intracranial Hypertension. Child's Nerv. Syst. 17 (3), 154–162. doi:10.1007/s003810000410
Teasdale, G., and Jennett, B. (1976). Assessment and Prognosis of Coma after Head Injury. Acta Neurochir. 34 (1-4), 45–55. doi:10.1007/bf01405862
Teasdale, G., and Jennett, B. (1974). Assessment of Coma and Impaired Consciousness. Lancet. 304 (7872), 81–84. doi:10.1016/s0140-6736(74)91639-0
Temkin, N. R., Dikmen, S. S., Wilensky, A. J., Keihm, J., Chabal, S., and Winn, H. R. (1990). A Randomized, Double-Blind Study of Phenytoin for the Prevention of Post-traumatic Seizures. N. Engl. J. Med. 323 (8), 497–502. doi:10.1056/nejm199008233230801
Thomas, B. W., Mejia, V. A., Maxwell, R. A., Dart, B. W., Smith, P. W., Gallagher, M. R., et al. (2010). Scheduled Repeat CT Scanning for Traumatic Brain Injury Remains Important in Assessing Head Injury Progression. J. Am. Coll. Surg. 210 (5), 824–830. doi:10.1016/j.jamcollsurg.2009.12.039
Tieves, K., and Sharp, N. (2015). Pediatric Head Trauma. J. Pediatr. Intensive Care. 04 (1), 047–054. doi:10.1055/s-0035-1554989
Tiret, L., Hausherr, E., Thicoipe, M., Garros, B., Maurette, P., Castel, J.-P., et al. (1990). The Epidemiology of Head Trauma in Aquitaine (France), 1986: a Community-Based Study of Hospital Admissions and Deaths. Int. J. Epidemiol. 19 (1), 133–140. doi:10.1093/ije/19.1.133
Tobias, J. D. (2000). Tolerance, Withdrawal, and Physical Dependency after Long-Term Sedation and Analgesia of Children in the Pediatric Intensive Care Unit. Crit. Care Med. 28 (6), 2122–2132. doi:10.1097/00003246-200006000-00079
Tuettenberg, J., Czabanka, M., Horn, P., Woitzik, J., Barth, M., Thomé, C., et al. (2009). Clinical Evaluation of the Safety and Efficacy of Lumbar Cerebrospinal Fluid Drainage for the Treatment of Refractory Increased Intracranial Pressure. Jns. 110 (6), 1200–1208. doi:10.3171/2008.10.Jns08293
Valadka, A. B., Gopinath, S. P., Contant, C. F., Uzura, M., and Robertson, C. S. (1998). Relationship of Brain Tissue PO2 to Outcome after Severe Head Injury. Crit. Care Med. 26 (9), 1576–1581. doi:10.1097/00003246-199809000-00029
van den Brink, W. A., van Santbrink, H., Steyerberg, E. W., Avezaat, C. J. J., Suazo, J. A. C., Hogesteeger, C., et al. (2000). Brain Oxygen Tension in Severe Head Injury. Neurosurgery. 46 (4), 868–878. discussion 876-868. doi:10.1097/00006123-200004000-0001810.1227/00006123-200004000-00018
van Erp, I. A., Gaitanidis, A., El Moheb, M., Kaafarani, H. M. A., Saillant, N., Duhaime, A.-C., et al. (2021). Low-molecular-weight Heparin versus Unfractionated Heparin in Pediatric Traumatic Brain Injury. J. Neurosurg. Pediatr. 27 (4), 469–474. doi:10.3171/2020.9.Peds20615
van Santbrink, H., Maas, A. I. R., and Avezaat, C. J. J. (1996). Continuous Monitoring of Partial Pressure of Brain Tissue Oxygen in Patients with Severe Head Injury. Neurosurgery. 38 (1), 21–31. doi:10.1097/00006123-199601000-00007
Vella, M. A., Crandall, M. L., and Patel, M. B. (2017). Acute Management of Traumatic Brain Injury. Surg. Clin. N. Am. 97 (5), 1015–1030. doi:10.1016/j.suc.2017.06.003
Wagner, A. (2016). “Traumatic Brain Injury,” in Braddom’s Physical Medicine and Rehabilitation. Editor C. Cifu. 5 ed (Philadelphia: Elsevier).
Wang, M. C., Linnau, K. F., Tirschwell, D. L., and Hollingworth, W. (2006). Utility of Repeat Head Computed Tomography after Blunt Head Trauma: a Systematic Review. J. Trauma Inj. Infect. Crit. Care. 61 (1), 226–233. doi:10.1097/01.ta.0000197385.18452.89
Ware, M. L., Nemani, V. M., Meeker, M., Lee, C., Morabito, D. J., and Manley, G. T. (2005). Effects of 23.4% Sodium Chloride Solution in Reducing Intracranial Pressure in Patients with Traumatic Brain Injury: a Preliminary Study. Neurosurgery. 57 (4), 727–736. discussion 727-736. doi:10.1227/01.NEU.0000175726.08903.0A
Wenderoth, B. R., Kaneda, E. T., Amini, A., Amini, R., and Patanwala, A. E. (2013). Morphine versus Fentanyl for Pain Due to Traumatic Injury in the Emergency Department. J. Trauma Nurs. 20 (1), 10–15. doi:10.1097/JTN.0b013e31828660b5
Williams, G. S. B., Boyman, L., Chikando, A. C., Khairallah, R. J., and Lederer, W. J. (2013). Mitochondrial Calcium Uptake. Proc. Natl. Acad. Sci. U.S.A. 110 (26), 10479–10486. doi:10.1073/pnas.1300410110
Wintermark, M., Sanelli, P. C., Anzai, Y., Tsiouris, A. J., Whitlow, C. T., Druzgal, T. J., et al. (2015). Imaging Evidence and Recommendations for Traumatic Brain Injury: Conventional Neuroimaging Techniques. J. Am. Coll. Radiology. 12 (2), e1–e14. doi:10.1016/j.jacr.2014.10.014
Wolf, J. A., Stys, P. K., Lusardi, T., Meaney, D., and Smith, D. H. (2001). Traumatic Axonal Injury Induces Calcium Influx Modulated by Tetrodotoxin-Sensitive Sodium Channels. J. Neurosci. 21 (6), 1923–1930. doi:10.1523/jneurosci.21-06-01923.2001
Wyte, S. R., Shapiro, H. M., Turner, P., and Harris, A. B. (1972). Ketamine-induced Intracranial Hypertension. Anesthesiology. 36 (2), 174–176. doi:10.1097/00000542-197202000-00021
Yen, H. L., and Wong, J. T. (2007). Rehabilitation for Traumatic Brain Injury in Children and Adolescents. Ann. Acad. Med. Singap. 36 (1), 62–66.
Keywords: traumatic brain injury, pediatrics, molecular pathogenesis, clinical management, algorithm
Citation: Lui A, Kumar KK and Grant GA (2022) Management of Severe Traumatic Brain Injury in Pediatric Patients. Front. Toxicol 4:910972. doi: 10.3389/ftox.2022.910972
Received: 01 April 2022; Accepted: 10 May 2022;
Published: 24 June 2022.
Edited by:
Stefan Liebau, University of Tübingen, GermanyReviewed by:
Lindsay Ferguson, University of California, Los Angeles, United StatesApostolos Zarros, Pharmacological Research Observatory, United Kingdom
Copyright © 2022 Lui, Kumar and Grant. This is an open-access article distributed under the terms of the Creative Commons Attribution License (CC BY). The use, distribution or reproduction in other forums is permitted, provided the original author(s) and the copyright owner(s) are credited and that the original publication in this journal is cited, in accordance with accepted academic practice. No use, distribution or reproduction is permitted which does not comply with these terms.
*Correspondence: Gerald A. Grant, Z2VyYWxkLmdyYW50QGR1a2UuZWR1