- 1Institute of Health and Sport Sciences, University of Tsukuba, Tsukuba, Japan
- 2Graduate School of Comprehensive Human Sciences, University of Tsukuba, Tsukuba, Japan
- 3Department of Sport Wellness Sciences, Japan Women's College of Physical Education, Tokyo, Japan
- 4Toyo University, Tokyo, Japan
- 5Morinomiya University of Medical Sciences, Osaka, Japan
- 6Osaka University of Health and Sport Sciences, Osaka, Japan
This study aimed to examine the relationship between the maximal lactate accumulation rate (ċLamax) and sprint performance parameters in male competitive swimmers. Seventeen male competitive swimmers volunteered to perform a 20 m maximal front crawl sprint without pushing off the wall from a floating position. ċLamax was determined by the 20-m sprint time and blood lactate measured before and after the 20 m sprint. For the sprint performance parameter, a 50 m time trial with the front crawl swimming stroke was conducted, and the times taken from 0 to 15 m, 15–25 m, 25–35 m, and 35–45 m were analyzed. A semi-tethered swimming test was conducted to investigate the load-velocity profile of each swimmer. From the load-velocity profile, theoretical maximal velocity (V0), maximal load (L0) and relative maximal load (rL0) were examined. The slope of the load-velocity profile was also determined. According to the results, ċLamax correlated with 50 m front crawl performance (r = −.546, p < .05). Moreover, a higher ċLamax was related to faster 0–35 m section time. Furthermore, ċLamax correlated with L0 (r = .837, p < .01), rL0 (r = .820, p < .01), and load-velocity slope (r = .804, p < .01). ċLamax is a good indicator of 50 m front crawl performance in male swimmers, and higher glycolytic power contributes to the faster time at the beginning of the sprint race. ċLamax could also evaluate the ability of a swimmer to apply force to the water during high-intensity swimming.
1 Introduction
Competitive swimming events include different distances, from 50 m to 1,500 m. The energy required to swim these distances is supplied by the aerobic and anaerobic systems; the relative contributions of each energy system vary depending on the duration of each race (1). Therefore, improvements in both energy systems are essential to enhance swimming performance, and effective monitoring is key to assessing the impact of training and achieving successful performance (2).
Several reliable parameters are available to monitor aerobic capacity in swimmers, including lactate threshold (3, 4), onset of blood lactate accumulation (5), and critical velocity (4, 6). Measurement of maximal accumulated oxygen uptake (MAOD) is widely used to assess anaerobic capacity in sports and is also used in swimming (7–9). However, MAOD is a time-consuming procedure to measure, and it may not be a fully defensible method to determine anaerobic capacity (10). Therefore, alternative practical methods and indices have been explored (11, 12).
The maximal lactate accumulation rate (ċLamax) is also an index to access anaerobic capacity. ċLamax is calculated by dividing the maximum difference in blood lactate (Bla) before and after a maximal sprint by the sprint time, which is reportedly a parameter to evaluate the athlete's glycolytic power (13). Due to its applicability in the training setting, ċLamax regained popularity and was investigated recently in cycling (14, 15), running (16), and rowing exercises (17). In addition, Quittmann et al. (16) investigated ċLamax during 100 m sprint running and reported that ċLamax was significantly correlated with sprint performance parameters, suggesting that it can be used as a sport-specific field test. ċLamax reportedly differs between handcycling and cycling (15) or cycling and running (18) even when investigated in the same individual, so it has been suggested that ċLamax is an extremity and movement-specific parameter.
ċLamax has also been investigated in swimming (19). Sperlich et al. (20) reported that ċLamax increases with high intensity interval training and decreases with high volume training, and that ċLamax is sensitive to detecting the change of anaerobic capacity in swimmers. Studies on the relationship between ċLamax and sprint performance in swimming have yet to reach consensus. Mavroudi et al. (21) reported a significant correlation between 50 m sprint performance and ċLamax which was investigated at that sprint. On the other hand, Kellar and Wahl (22) reported no significant correlation between ċLamax assessed by a 20-s sprint test and the average velocity of a 50 m official race. Thus, it is still being determined whether ċLamax is a genuinely valid measure that can assess anaerobic capacity in swimmers.
Mechanical power is one of the factors that determines maximal swimming velocity (23). Even a semi-tethered swimming test cannot evaluate pure propulsive power, Gonjo et al. (24) reported that the index of the load-velocity profile investigated by semi-tethered swimming can be used to evaluate sprint swimming performance. Their findings revealed that theoretical maximal velocity (V0), maximal load (L0), and load-velocity slope were significantly correlated with the mean velocity of a 50 m front crawl trial. Given the potential of these indices as indicators of sprinting ability, understanding the relationship between ċLamax and these parameters could shed light on whether pure physiological capacity is related to sprinting ability in swimming. If glycolytic power is indeed related to sprint performance parameters, this approach would allow for a more comprehensive understanding of sprint swimming performance, combining both mechanical and physiological aspects.
The purpose of the present study was to investigate the relationship between ċLamax and parameters related to sprint performance in male competitive swimmers. The primary hypothesis of this study was that ċLamax negatively correlates with 50 m race time. Secondly, we hypothesized that ċLamax positively correlates with parameters related to sprint performance (such as V0 and L0) estimated by the load-velocity profile. By addressing these research objectives, we provide valuable insights into the use of ċLamax as an index of anaerobic capacity in competitive swimming.
2 Materials and methods
2.1 Participants
Seventeen well-trained male competitive swimmers participated in this study. The personal characteristics of the swimmers are shown in Table 1. Four swimmers specialized in short-distance front crawl, five in middle-distance front crawl, five in butterfly, two in backstroke, and one in individual medley. All swimmers (who were from the same university swimming team) had engaged in competitive swimming for over 6 years and competed at national-level championships. The World Aquatics point scoring of the swimmers’ personal best records of their major event was 751.8 ± 68.1 point. The participants were made fully aware of the risks, benefits, and stress factors of the study and gave their written consent to participate. In addition, the participants were instructed to refrain from caffeine intake within 12 h, and water was only allowed within 2 h of testing. This study received the formal approval of the Research Ethics Committee of the authors’ institution (No. 024-23) in compliance with the Declaration of Helsinki.
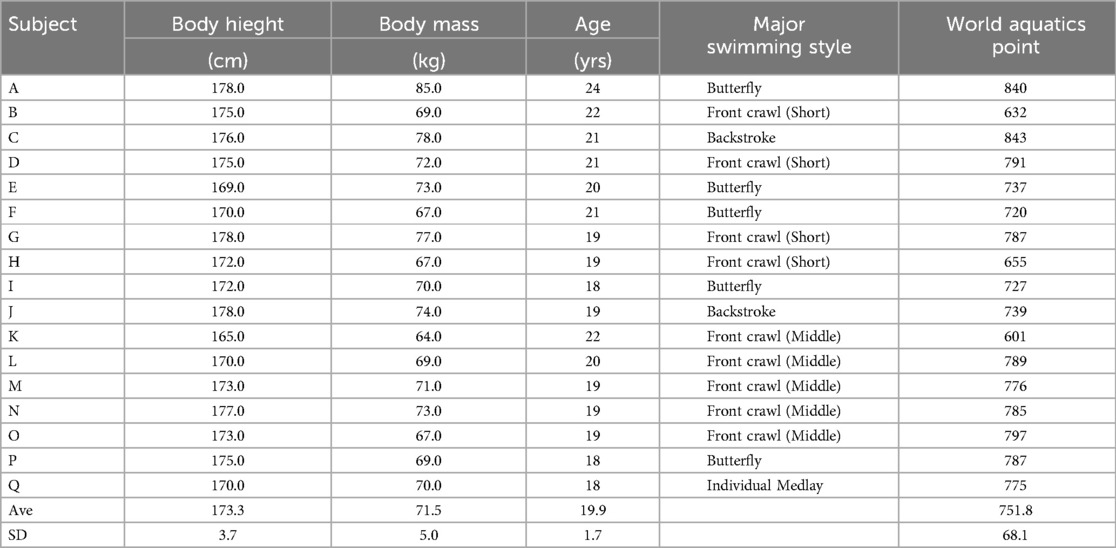
Table 1. Participants’ physical characteristics, major swimming style and performance level. The World Aquatics point scoring of the swimmers’ personal best records of their major event is demonstrated.
2.2 Experimental procedure
All experiments were scheduled during the general and preparation period of the training season (2) and conducted in an indoor 50 m pool. Participants were instructed to conduct a self-selected warm-up mimicking their competition routine. This was followed by 5 min of passive rest while seated. The duration of the rest period was in accordance with previous research (15, 17, 18). After the passive rest, the participants performed a 20 m all-out front crawl sprint swim without pushing off the wall to evaluate ċLamax. In this trial, the participants were instructed to accelerate as fast as possible from a floating position at the 5 m point until they passed the 25 m point. Thereafter, a 50 m time trial and semi-tethered swimming test with a front crawl were conducted to investigate the sprint performance parameters. All experiments were separately conducted with minimum interval of 16 h and performed within one week.
2.3 ċLamax investigation
Olbrecht (19) recommends that ċLamax should be obtained and measured during a maximal sprint exercise of 10–15 s. In swimming, Mavroudi et al. (21) reported that ċLamax values obtained in a 25 m all-out swim were significantly higher than those obtained in 35 m and 50 m all-out swims, shorter duration is appropriate for investigating ċLamax. Instead of a 25 m all-out swim, we adopted a 20 m sprint swim of front crawl starting from a floating position at the 5 m mark. As it is reported that ċLamax is a limb and movement specific parameter (15, 18), this procedure eliminates the movement of the block start or wall push and the underwater phase and make it possible to execute the sprint exercise with front crawl swimming movement only. Our preliminary investigation confirmed that the duration of this swim sprint can last approximately 11 s, which falls within the range recommended by Olbrecht (19). ċLamax was determined using Equation 1 (13, 19, 25):
where Lamax is the highest Bla after the 20 m sprint, Lapre is the Bla measured during the passive rest before the sprint, tsprint is the time to complete the 20 m sprint, and talac is the estimated time when energy is delivered by the alactic system.
Bla was measured using a portable analyzer (Lactate Pro2, LT-1730, Arkray, Kyoto, Japan). Considering the reliability and accuracy of the measurement device (26), each Bla was examined using two analyzers, and then the average value was used for analysis. Immediately after completing the 20 m sprint swim, participants were seated on the pool deck. An examiner started a stopwatch at the moment swimmer completed the 20 m sprint. Blood was sampled from the fingertips at precise one-minute intervals. The sampling and measurement process continued until the mean Bla value was lower than the previous measurement, indicating that the peak had been reached and passed. The timing of the blood sampling was consistent for all participants, ensuring standardized data collection across the study. The highest value was taken as Lamax, and the time to reach Lamax after the sprint (tLamax) was also examined. Two digital cameras (GC-IJ20B; Sports Sensing, Fukuoka, Japan) were set at 5 m and 25 m points to capture the timing of the passage of an LED marker attached to the participant's head through 5 m and 25 m marks of the pool. Their passing times and tsprint were analyzed using 2-D motion analysis software (Frame Dias V, Q'sfix, Tokyo, Japan). Camera synchronization was achieved through an LED system (LED synchronizer, Q'sfix). talac is the period at the beginning of exercise for which no lactate production is assumed. In cycling exercise, talac can be determined as the time when power output decreased by 3.5% from peak power output directly measured during the maximal sprint (27, 28). However, as it is difficult to measure the power output during swimming exercise, a fixed standard value of 3 s was used for talac in this study according to previous research (13, 29). Eleven participants were retested with the same procedure within 4 days to confirm the reliability of the measurement data.
2.4 Sprint performance parameters
2.4.1 50 m maximal trial
The 50 m time trial required participants to swim front crawl with maximum effort for the sprint performance parameter. After a self-selected warm-up on land and in water, the trial was performed starting from the starting block. The maximal trial was conducted by each swimmer at the center lane of the pool to avoid pacing strategies. The 50 m time trial was recorded by panning one digital video camera (60 fps, GC-IJ20B; Sports Sensing) from the second floor of the indoor pool. The timing when the swimmer's head passed the 15, 25, 35, and 45 m point, which was marked on both side of the lane ropes, and the timing swimmer's hand touched the wall after the starting signal was investigated by feeding the video recording frame by frame at a frame rate of 60 fps. This procedure was repeated three times by the same researcher, and the total time to complete 50 m swim (t50) and the section time of 0–15 m, 15–25 m, 25–35 m, and 35–45 m distances were analyzed.
2.4.2 Load-velocity profile
To obtain the load-velocity profile of each participant, a semi-tethered swimming test was performed using a portable robotic resistance device “1080 sprint” (1080 Motion, Lidingö, Sweden). The device was positioned 0.5 m above the water surface on the starting side of the pool (Figure 1). Participants were instructed to wear a swim belt for resisted sprint training around their pelvis (Long Belt Slider S11875, StrechCordz, Bloomington, IL, USA), which was connected to the cord of the resistance device. Each sprint began with a push-off start in the water, and the participants were instructed to start swimming before reaching the 5 m mark. After the warm-up, participants had to perform five 25 m front crawl sprints with maximal effort. There was a rest interval of more than 6 min between each sprint. The instantaneous time-swim velocity data were collected at 333 Hz. The mean velocity during the three stroke cycles between 10 and 20 m section was analyzed using the 1080 sprint software. The velocity measurement cord was not aligned with the swimming direction because the measurement device was placed 0.5 m above the water surface. As a result, the measured velocity was adjusted to the horizontal velocity based on Gonjo et al. (30).
The load-velocity profile of the sprint swim was calculated. The added loads for each sprint were 1, 3, 5, 7, and 9 kg (investigated in ascending order). Olstad et al. (31) reported that 9 kg load imposed more than a 50% velocity reduction compared to a 1 kg load trial in swimmers. This aligns with the suggestion by Cross et al. (32) that a 50% velocity decrease is appropriate for multiple trial sprint testing with external loads. Based on these findings, Gonjo et al. (24) proposed that an absolute load with a maximum of 9 kg is adequate for a semi-tethered swimming test. While Olstad et al. (31) indicated that using five trials (i.e., 1, 3, 5, 7, and 9 kg) in semi-tethered front crawl swimming does not significantly change the outcomes of load-velocity profiling compared to three trials (1, 5, and 9 kg), we opted the five-load protocol to obtain a more precise load-velocity relationship. By examining the linear regression line of the five load-velocity plots (33), the theoretical maximal velocity (V0) and maximal load (L0) were investigated. L0 was also expressed as a percentage of body weight (rL0). The slope of the load-velocity relationship was also determined (31). The load-velocity slope indicates the resistive force and is reportedly correlated with active drag in front crawl swimming. A steeper slope indicates lower active drag (34). Figure 2 shows an example of the load-velocity profile measured for the sprint performance parameters.
2.5 Statistical analysis
All data are reported as mean ± SD and 95% confidence intervals was calculated. Normal distribution of variables was assessed using the Shapiro–Wilk test. The reliability of ċLamax (measured twice in 11 swimmers) was analyzed using the intra-class correlation coefficient (ICC). ICC was classified as “excellent” (ICC ≥ 0.90), “good” (0.90 > ICC ≥ 0.75), “moderate” (0.75 > ICC ≥ 0.50), or “poor” (ICC < 0.50) (35). The relationships between ċLamax and sprint performance parameters were investigated using Pearson's correlation coefficient for normally distributed data and Spearman's rank correlation coefficient for non-normally distributed data. Correlation threshold values of 0.1, 0.3, 0.5, 0.7, and 0.9 were interpreted as small, moderate, large, very large, and extremely large correlations, respectively (36). The power of the correlation test was also computed. The analysis was performed with SPSS software (version 29, IBM, Tokyo, Japan), and the statistical significance level was set at 5%.
3 Results
The measured parameters are shown in Table 2. The average time of the 20 m sprint for the ċLamax measurement was 11.5 ± 0.4 s, Lapre was 1.6 ± 0.4 mmol/L, Lamax was 6.8 ± 1.2 mmol/L, tLamax was 1.8 ± 0.8 min, and ċLamax was 0.63 ± 0.14 mmol/L/s. ICC of the twice-measured ċLamax was 0.913 (95% confidence interval: 0.724–0.976, p < .01). ċLamax showed significant correlation with t50 (r = −.546, p < .05) and the section time of 0–15 m (r = −.627, p < .01), 15–25 m (r = −.618, p < .01), 25–35 m (r = −.604, p < .05) of the trial. L0 (r = .837, p < .01), rL0 (r = .820, p < .01), and the slope (r = .804, p < .01) obtained from the load-velocity profile also significantly correlated with ċLamax.
4 Discussion
The present study explored the relationship between ċLamax and factors associated with sprint performance in male competitive swimmers. The main findings of this study were that ċLamax is significantly correlated with 50 m front crawl performance in male swimmers and that a higher glycolytic power contributes to a faster time at the beginning of the sprint race. We were also able to clarify that ċLamax is significantly related to the theoretical maximal load a swimmer can pull with front crawl swimming.
The time taken for the 20 m sprint to measure ċLamax was 11.5 ± 0.4 s, which falls within the recommended range of 10–15 s for this parameter (19). In a study by Langley et al. (37), it was found that the 10-s sprint duration produced the highest ċLamax value when compared to the 15-s and 30-s sprints in cycling tests. Mavroudi et al. (21) also reported higher ċLamax values when investigating shorter sprints in swimming. Additionally, Quittmann et al. (16) suggested that investigating ċLamax based on a fixed distance instead of a fixed duration protocol may be more appropriate in field-based settings if the duration variation is minimal. Overall, the 20 m sprint utilized in this study was appropriate and applicable for assessing a swimmer's highest glycolytic power. The ċLamax value calculated in this study was 0.63 ± 0.14 mmol/L/s, which is similar to the values reported by Sperlich et al. (20) and Mavroudi et al. (21) of 0.64 ± 0.29 and 0.75 ± 0.18 mmol/L/s, respectively. However, in research with young female swimmers, Kellar and Wahl (22) found a lower value of 0.38 ± 0.11 mmol/L/s in their research with young female swimmers. It is worth noting that although ċLamax was assessed using different methods in these studies, it seems that the value is lower in females compared to males, possibly due to differences in muscle mass (38), which could affect lactate production during exercise (39). Generally, female swimmers tend to have longer completion times for a 20 m sprint compared to male swimmers. Additionally, if lactate production is lower in females, ċLamax values will tend to be lower due to the formula used to calculate this parameter (Equation 1). These factors suggest that the relationship between ċLamax and performance may be gender-specific.
The reliability of the ċLamax was “excellent” when measured twice within 4 days (ICC = 0.913). This ICC value was slightly higher than those reported in previous investigations on cycling [ICC = 0.904, (40)], running [ICC = 0.907, (16)], and rowing [ICC = 0.85, (17)]. The accuracy and reliability of the Bla measurement device used in this study were evaluated as good according to the methods of Bonaventura et al. (26). It is worth noting that given the small volume of sampled blood (0.3 µl), we took the average Bla value assessed by the two devices to ensure a stable value. The measurement procedure used in this study clarified that ċLamax could be investigated with high reliability.
Mavroudi et al. (21) studied eight male and four female swimmers and found that a 50 m sprint trial significantly correlated with ċLamax measured during that swim; however, this result may be affected by including both genders. In the present study of male-only measurements and a 20 m sprint, we also found a significant correlation between ċLamax and t50. On the other hand, Kellar and Wahl (22) investigated the relationship between ċLamax and 50 m swim race performance but found no significant relationship. The differences in the measurement protocol may cause different results. Kellar and Wahl (22) investigated ċLamax from a 20-s sprint test in which the duration is longer than the highest value reportedly observed. The sprint duration should be shorter to assess ċLamax related to 50 m performance. Moreover, our measurement setting was defined by the fact that the short sprint was executed from a floating position. This execution eliminates the movement of the block start or wall push and the underwater phase, which is completely different from the regular swimming motion. Thus, this indicated that accurately assessing ċLamax could be achieved by excluding factors other than swimming motion, allowing for the practical evaluation of glycolytic power during swimming. As a result, a 20 m sprint swim solely relying on the swimming motion is an effective way to measure ċLamax, making it an important parameter to monitor during training.
When examining the relationship between ċLamax and each section time of the 50 m trial, large correlations were observed at the 0–15 m, 15–25 m, and 25–35 m section times. The contribution ratio of aerobic and anaerobic metabolism is reportedly 30:70 in a 50 m race (1). Therefore, a higher glycolytic power is related to a faster time up to 35 m of the race, while aerobic capacity may be related to the later performance. Therefore, the present study confirmed that ċLamax is a good performance indicator in the first half of a 50 m front crawl swim, suggesting that glycolytic power is crucial for 50 m sprint performance, and swimmers aiming to start fast in a 50 m race should focus on improving ċLamax. Research has shown varied effects of different training modalities on ċLamax. Sperlich et al. (20) reported an increase in ċLamax following high-intensity interval training in junior swimmers. Similarly, Nitzsche et al. (41) observed an increase in ċLamax with resistance training in male strength-trained volunteers. However, contrasting results were found by Hommel et al. (14), who reported a decrease in ċLamax after a six-week sprint interval cycling training program. These conflicting findings underscore the need for further research on training interventions specifically aimed at improving glycolytic power in swimmers.
ċLamax correlated with L0 and rL0, indicating the connection between glycolytic power and the ability to exert force in the water. As Maglischo (2) highlighted, the limiting factors of a 50 m race performance are the stroke technique, the rate of anaerobic metabolism, and the amount of creatine phosphate in the working muscle fibers of a swimmer. Therefore, ċLamax could evaluate the first two factors, making it a valuable parameter for monitoring the swimmer's sprint performance capacity throughout the training season. Coaches and athletes can use ċLamax and aerobic capacity parameter to plan and prescribe training for performance improvement and to avoid nonfunctional overreaching (19, 29). Our study also proposed a practical procedure to evaluate ċLamax in swimming.
A very large correlation was found between ċLamax and L0, rL0, and the slope calculated from the load-velocity profile. L0 represents the theoretical maximal load that swimmers can exert on the water (31). As active drag is approximately proportional to the cube of swimming velocity (42), swimmers need to overcome a large amount of drag during high-velocity swimming. Consequently, a high L0 is one of the essential abilities for sprinters. rL0 correlates significantly with 50 m front crawl performance (24). Therefore, the present results indicate that the pure capacity to produce power at a higher rate is related to the higher capacity to apply force to the water, which could be crucial for achieving high sprinting performance. Moreover, the significant correlation between ċLamax and the load–velocity slope may be affected by the significant relationship between ċLamax and L0. Based on our findings, V0 did not correlate with ċLamax. As V0 represents the theoretical maximal velocity, it may be closely associated with the contribution of the alactic energy system. Furthermore, Gonjo et al. (24) suggested that V0 should not be used to predict the absolute free-swimming speed during a race due to the observed systematic bias between the average swimming velocity of the 50 m front crawl trial and V0, which may have affected our results.
Beyond evaluating an athlete's glycolytic power, ċLamax has potential applications in predicting individual pacing strategies. Quittmann et al. (43) suggested that this parameter could be used to predict pacing strategies in 5,000 m running, a concept that may be applicable to sprint swimming races. Swimmers with high ċLamax values are likely to start races quickly and apply greater force to the water. For these athletes, coaches should focus on strategies that allow for a fast start while minimizing effort, to prevent fatigue at the end of the race. Conversely, swimmers with lower ċLamax values should avoid an intense burst immediately after the breakout. Instead, they should focus on gradually increasing their effort towards the end of the race. By tailoring race strategies to individual ċLamax profiles, coaches and swimmers can optimize performance and energy distribution throughout the race. Comparing the relationship between ċLamax and race performance across multiple competitions may provide valuable insights into whether success or failure in a race is primarily due to physiological or technical factors. This approach could help coaches and athletes differentiate between improvements in anaerobic capacity and enhancements in swimming technique or race strategy. Furthermore, Morais et al. (44) reported that swimmer with faster critical speed was more likely to perform better in middle- or long-distance events than in sprints. By examining critical speed together, it may provide more detailed information to coaches and swimmers including evaluation of aerobic capacity. Longitudinal studies are warranted to fully clarify the practical applicability of ċLamax in real-world competitive settings. Such research could reveal how this parameter changes over time with training and how these changes correlate with performance improvements. Additionally, it could help establish ċLamax as a reliable tool for long-term athlete monitoring and performance prediction.
A limitation of the present study is that a constant value of 3 s was used for talac to calculate ċLamax. Yang et al. (45) reported that insights into the inter-individual differences in energy and glycolytic metabolism would be altered by using different talac values. An alternative procedure to assess talac is being explored (46). The actual time of talac likely varies among participants, which may influence the precision of ċLamax calculations. If novel approaches to measure power output during swimming can be developed, it would enable a more accurate evaluation of ċLamax. Such advancements in measurement techniques could potentially affect the results of the present study and provide more precise insights into the relationship between ċLamax and swimming performance. However, it still seems complicated to establish a rational method to assess talac during swimming exercise; using a constant value may remain a practical procedure to investigate ċLamax in this sport. Second, Affonso et al. (47) reported an extremely high Bla level of 12.1–17.4 mmol/L after a 5-s short sprint in an elite front crawl swimmer (World Aquatics point = 985). The high ċLamax in this swimmer suggests that higher glycolytic power may be essential for achieving high sprint performance. However, it should be noted that this relationship may have been observed due to the swimmers having identical mechanical efficiencies. Toussaint (48) examined the propelling efficiency of well-trained competitive swimmers and triathletes, reporting values of 61 ± 6% for swimmers and 44 ± 3% for triathletes. This suggests that, even if two swimmers exert the same glycolytic power, a swimmer with lower mechanical efficiency will be slower. It is essential to consider the implications of our study when applied to swimmers at different performance levels. Several methods exist for investigating propulsive efficiency during sprint swimming, including the MAD (Measuring Active Drag) system (48) and pressure distribution analysis (49). By combining these propulsive efficiency evaluations with ċLamax assessments, it would be possible to develop a more comprehensive profile of a swimmer's characteristics. This integrated approach could provide valuable information for planning future training strategies. Specifically, it would allow coaches and athletes to determine whether to focus on improving stroke mechanics or enhancing anaerobic power, based on individual strengths and weaknesses identified through these complementary assessments. Such a multifaceted evaluation strategy could lead to more targeted and effective training interventions, potentially optimizing performance improvements in sprint swimming.
5 Conclusion
The testing procedure of ċLamax used in the present study was found to be suitable for training settings and to have high reliability. The study clarified that ċLamax is a parameter associated with the 50 m front crawl performance in male swimmers, and a higher glycolytic power leads to faster times at the beginning of a sprint race. It also indicated that ċLamax can assess a swimmer's ability to apply force to the water during high-intensity swimming. Therefore, ċLamax is a good indicator of front crawl sprint performance for well-trained male swimmers.
Data availability statement
The original contributions presented in the study are included in the article/Supplementary Material, further inquiries can be directed to the corresponding author.
Ethics statement
The studies involving humans were approved by Human Subjects Committee of the University of Tsukuba. The studies were conducted in accordance with the local legislation and institutional requirements. The participants provided their written informed consent to participate in this study.
Author contributions
YS: Conceptualization, Funding acquisition, Methodology, Supervision, Writing – original draft, Writing – review & editing, Data curation. AS: Formal Analysis, Investigation, Methodology, Visualization, Writing – original draft, Writing – review & editing. JK: Formal Analysis, Investigation, Methodology, Validation, Writing – original draft, Writing – review & editing. KH: Formal Analysis, Investigation, Methodology, Validation, Writing – original draft, Writing – review & editing. YN: Formal Analysis, Investigation, Methodology, Validation, Writing – original draft, Writing – review & editing. TT: Investigation, Methodology, Validation, Writing – original draft, Writing – review & editing. NH: Conceptualization, Resources, Writing – original draft, Writing – review & editing. AN: Investigation, Methodology, Validation, Writing – original draft, Writing – review & editing, Formal Analysis. MI: Investigation, Methodology, Validation, Writing – original draft, Writing – review & editing.
Funding
The author(s) declare financial support was received for the research, authorship, and/or publication of this article. This research was supported by a grant 2023(I)5 from Advanced Research Initiative for Human High Performance (ARIHHP), University of Tsukuba and JSPS KAKENHI Grant Number JP23K10683.
Conflict of interest
The authors declare that the research was conducted in the absence of any commercial or financial relationships that could be construed as a potential conflict of interest.
Publisher's note
All claims expressed in this article are solely those of the authors and do not necessarily represent those of their affiliated organizations, or those of the publisher, the editors and the reviewers. Any product that may be evaluated in this article, or claim that may be made by its manufacturer, is not guaranteed or endorsed by the publisher.
References
1. Ogita F. Energetics in competitive swimming and application for training. Portuguese J Sport Sci. (2006) 6(Suppl.2):117–21.
3. Pyne DB, Lee H, Swanwick KM. Monitoring the lactate threshold in world-ranked swimmers. Med Sci Sports Exer. (2001) 33:291–7. doi: 10.1097/00005768-200102000-00019
4. Toubekis AG, Tsami AP, Tokmakidis SP. Critical velocity and lactate threshold in young swimmers. Int J Sports Med. (2006) 27:117–23. doi: 10.1055/s-2005-83748716475057
5. Wakayoshi K, Yoshida T, Ikuta Y, Mutoh Y, Miyashita M. Adaptations to six months of aerobic swim training. Changes in velocity, stroke rate, stroke length and blood lactate. Int J Sports Med. (1993) 14:368–72. doi: 10.1055/s-2007-10211948244602
6. Wakayoshi K, Yoshida T, Udo M, Kasai T, Moritani T, Mutoh Y, et al. A simple method for determining critical speed as swimming fatigue threshold in competitive swimming. Int J Sports Med. (1992) 13:367–71. doi: 10.1055/s-2007-10212821521952
7. Ogita F, Hara M, Tabata I. Anaerobic capacity and maximal oxygen uptake during arm stroke, leg kicking and whole body swimming. Acta Physiol Scand. (1996) 157:435–41. doi: 10.1046/j.1365-201X.1996.490237000.x8869726
8. Reis VM, Marinho DA, Policarpo FB, Carneiro AL, Baldari C, Silva AJ. Examining the accumulated oxygen deficit method in front crawl swimming. Int J Sports Med. (2010) 31:421–7. doi: 10.1055/s-0030-124828620301045
9. Kalva-Filho CA, Araújo MY, Silva A, Gobatto CA, Zagatto AM, Gobbi RB, et al. Determination of VO2-intensity relationship and MAOD in tethered swimming. Int J Sports Med. (2016) 37:687–93. doi: 10.1055/s-0035-155969627176891
10. Noordhof DA, de Koning JJ, Foster C. The maximal accumulated oxygen deficit method: a valid and reliable measure of anaerobic capacity? Sports Med. (2010) 40:285–302. doi: 10.2165/11530390-000000000-0000020364874
11. Smolka L, Ochmann B. A novel method of anaerobic performance assessment in swimming. J Strength Cond Res. (2013) 27:533–9. doi: 10.1519/JSC.0b013e31825489b222465990
12. Kalva-Filho CA, Zagatto AM, da Silva ASR, Castanho MY, Gobbi RB, Gobatto CA, et al. Relationships among the tethered 3-min all-out test, MAOD and swimming performance. Int J Sports Med. (2017) 38:353–8. doi: 10.1055/s-0043-10191428486734
13. Heck H, Schulz H, Bartmus U. Diagnostics of anaerobic power and capacity. Eur J Sport Sci. (2003) 3:1–23. doi: 10.1080/17461390300073302
14. Hommel J, Öhmichen S, Rudolph UM, Hauser T, Schulz H. Effects of six-week sprint interval or endurance training on calculated power in maximal lactate steady state. Biol Sport. (2019) 36:47–54. doi: 10.5114/biolsport.2018.7890630899139
15. Quittmann OJ, Abel T, Vafa R, Mester J, Schwarz YM, Strüder HK. Maximal lactate accumulation rate and post-exercise lactate kinetics in handcycling and cycling. Eur J Sport Sci. (2021) 21:539–51. doi: 10.1080/17461391.2020.175642032290796
16. Quittmann OJ, Appelhans D, Abel T, Strüder HK. Evaluation of a sport-specific field test to determine maximal lactate accumulation rate and sprint performance parameters in running. J Sci Med Sport. (2020) 23:27–34. doi: 10.1016/j.jsams.2019.08.01331477377
17. Held S, Rappelt L, Brockherde J, Donath L. Reliability of the maximal lactate accumulation rate in rowers. Int J Sports Med. (2024) 45:238–44. doi: 10.1055/a-2206-495938109898
18. Quittmann OJ, Schwarz YM, Mester J, Foitschik T, Abel T, Strüder HK. Maximal lactate accumulation rate in all-out exercise differs between cycling and running. Int J Sports Med. (2021) 42:314–22. doi: 10.1055/a-1273-758933137832
19. Olbrecht J. Lactate production and metabolism in swimming. In: Seifert L, Chalet D, Mujika I, editors. World Book of Swimming: From Science to Performance. New York, NY: Nova Science Publishers, Inc. (2011). p. 255–75.
20. Sperlich B, Zinner C, Heilemann I, Kjendlie PL, Holmberg HC, Mester J. High-intensity interval training improves VO2peak, maximal lactate accumulation, time trial and competition performance in 9–11-year-old swimmers. Eur J Appl Physiol. (2010) 110:1029–36. doi: 10.1007/s00421-010-1586-420683609
21. Mavroudi M, Kabasakalis A, Petridou A, Mougios V. Blood lactate and maximal lactate accumulation rate at three sprint swimming distances in highly trained and elite swimmers. Sports. (2023) 11:87. doi: 10.3390/sports1104008737104161
22. Keller S, Wahl P. Relationship between metabolic and mechanical determinants and performance in young female squad swimmers. In: Witt M, editor. Proceeding of the XIVth International Symposium on Biomechanics and Medicine in Swimming; Leipzig: evoletics Media (2023). p. 273.
23. Gatta G, Cortesi M, Swaine I, Zamparo P. Mechanical power, thrust power and propelling efficiency: relationships with elite sprint swimming performance. J Sports Sci. (2018) 36:506–12. doi: 10.1080/02640414.2017.132221428471718
24. Gonjo T, Njøs N, Eriksrud O, Olstad BH. The relationship between selected load-velocity profile parameters and 50 m front crawl swimming performance. Front Physiol. (2021) 12:625411. doi: 10.3389/fphys.2021.62541133679439
25. Manunzio C, Mester J, Kaiser W, Wahl P. Training intensity distribution and changes in performance and physiology of a 2nd place finisher team of the race across America over a 6 month preparation period. Front Physiol. (2016) 7:642. doi: 10.3389/fphys.2016.0064228082909
26. Bonaventura JM, Sharpe K, Knight E, Fuller KL, Tanner RK, Gore CJ. Reliability and accuracy of six hand-held blood lactate analysers. J Sports Sci Med. (2015) 14:203–14.25729309
27. Hauser T, Adam J, Schulz H. Comparison of calculated and experimental power in maximal lactate-steady state during cycling. Theor Biol Med Model. (2014) 11:25. doi: 10.1186/1742-4682-11-2524886168
28. Quittmann OJ, Abel T, Zeller S, Foitschik T, Strüder HK. Lactate kinetics in handcycling under various exercise modalities and their relationship to performance measures in able-bodied participants. Eur J Appl Physiol. (2018) 118:1493–505. doi: 10.1007/s00421-018-3879-y29725756
29. Quittmann OJ, Lenatz B, Bartsch P, Lenatz F, Foitschik T, Abel T. Case report: training monitoring and performance development of a triathlete with spinal cord injury and chronic myeloid leukemia during a paralympic cycle. Front Rehabilit Sci. (2022) 3:867089. doi: 10.3389/fresc.2022.867089
30. Gonjo T, Eriksrud O, Papoutsis F, Olstad BH. Relationships between a load-velocity profile and sprint performance in butterfly swimming. Int J Sports Med. (2020) 41:461–7. doi: 10.1055/a-1103-211432059244
31. Olstad BH, Gonjo T, Njøs N, Abächerli K, Eriksrud O. Reliability of load-velocity profiling in front crawl swimming. Front Physiol. (2020) 11:574306. doi: 10.3389/fphys.2020.57430633071829
32. Cross MR, Samozino P, Brown SR, Morin JB. A comparison between the force-velocity relationships of unloaded and sled-resisted sprinting: single vs. multiple trial methods. Eur J Appl Physiol. (2018) 118:563–71. doi: 10.1007/s00421-017-3796-529302753
33. Dominguez-Castells R, Arellano R. Effect of different loads on stroke and coordination parameters during freestyle semi-tethered swimming. J Hum Kinet. (2012) 32:33–41. doi: 10.2478/v10078-012-0021-923486657
34. Gonjo T, Ljødal I, Karsson R, Olstad BH. Load-velocity slope can be an indicator of the active drag in front crawl swimming. ISBS Conference Proceedings. (2022) 40:19–23.
35. Koo TK, Li MY. A guideline of selecting and reporting interclass correlation coefficients for reliability research. J Chiropr Med. (2016) 15:155–63. doi: 10.1016/j.jcm.2016.02.01227330520
36. Hopkins WG, Marshall SW, Batterham AM, Hanin J. Progressive statistics for studies in sports medicine and exercise science. Med Sci Sports Exerc. (2009) 41:3–13. doi: 10.1249/MSS.0b013e31818cb27819092709
37. Langley JO, Porter MS. VLamax: determining the optimal test duration for maximal lactate formation rate during all-out sprint cycle ergometry. Eur J Appl Physiol Ahead of Print. (2024) 124:3149–50. doi: 10.1007/s00421-024-05507-1
38. Dopsaj M, Zuoziene IJ, Milić R, Cherepov E, Erlikh V, Masiulis N, et al. Body composition in international sprint swimmers: are there any relations with performance? Int J Environ Res Public Health. (2020) 17:9464. doi: 10.3390/ijerph1724946433348744
39. Jensen-Urstad M, Svedenhag J, Sahlin K. Effect of muscle mass on lactate formation during exercise in humans. Eur J Appl Physiol Occup Physiol. (1994) 69:189–95. doi: 10.1007/BF010947878001528
40. Adam J, Öhmichen M, Öhmichen E, Rother J, Müller UM, Hauser T, et al. Reliability of the calculated maximal lactate steady state in amateur cyclists. Biol Sport. (2015) 32:97–102. doi: 10.5604/20831862.113431126028808
41. Nitzsche N, Lenz JC, Voronoi P, Schulz H. Adaption of maximal glycolysis rate after resistance exercise with different volume load. Sports Med Int Open. (2020) 4:E39–44. doi: 10.1055/a-1146-423632377562
42. Narita K, Nakashima M, Takagi H. Developing a methodology for estimating the drag in front-crawl swimming at various velocities. J Biomech. (2017) 54:123–8. doi: 10.1016/j.jbiomech.2017.01.03728249682
43. Quittmann OJ, Foitschik T, Vafa R, Freitag FJ, Sparmann N, Nolte S, et al. Is maximal lactate accumulation rate promising for improving 5000-m prediction in running? Int J Sports Med. (2023) 44:268–79. doi: 10.1055/a-1958-387636529130
44. Morais JE, Barbosa TM, Bragada JA, Ramirez-Campillo R, Marinho DA. Interaction of kinematic, kinetic, and energetic predictors of young swimmers’ speed. Int J Sports Physiol Perform. (2023) 18:833–9. doi: 10.1123/ijspp.2022-043037268299
45. Yang WH, Park SY, Kim T, Jeon HJ, Heine O, Gehlert S. A modified formula using energy system contributions to calculate pure maximal rate of lactate accumulation during a maximal sprint cycling test. Front Physiol. (2023) 14:1147321. doi: 10.3389/fphys.2023.114732137123252
46. Dunst AK, Hesse C, Feldmann A, Holmberg HC. A novel approach to determining the alactic time span in connection with assessment of the maximal rate of lactate accumulation in elite track cyclists. Int J Sports Physiol Perform. (2023) 18:157–63. doi: 10.1123/ijspp.2021-046436596309
47. Affonso HO, Silva AS, Fernandes RJ. Can blood lactate concentrations rise significantly after very short duration swimming bouts? Ann Sports Med Res. (2019) 6:1139.
48. Toussaint HM. Differences in propelling efficiency between competitive and triathlon swimmers. Med Sci Sports Exerc. (1990) 22:409–15.2381311
Keywords: anaerobic capacity, glycolytic power, blood lactate, load-velocity profile, front crawl swimming
Citation: Sengoku Y, Shinno A, Kim J, Homoto K, Nakazono Y, Tsunokawa T, Hirai N, Nobue A and Ishikawa M (2024) The relationship between maximal lactate accumulation rate and sprint performance parameters in male competitive swimmers. Front. Sports Act. Living 6:1483659. doi: 10.3389/fspor.2024.1483659
Received: 20 August 2024; Accepted: 8 October 2024;
Published: 22 October 2024.
Edited by:
Rodrigo Zacca, University of Porto, PortugalReviewed by:
Flávio De Souza Castro, Federal University of Rio Grande do Sul, BrazilGavriil George Arsoniadis, National and Kapodistrian University of Athens, Greece
Jorge E. Morais, Polytechnic Institute of Bragança (IPB), Portugal
Copyright: © 2024 Sengoku, Shinno, Kim, Homoto, Nakazono, Tsunokawa, Hirai, Nobue and Ishikawa. This is an open-access article distributed under the terms of the Creative Commons Attribution License (CC BY). The use, distribution or reproduction in other forums is permitted, provided the original author(s) and the copyright owner(s) are credited and that the original publication in this journal is cited, in accordance with accepted academic practice. No use, distribution or reproduction is permitted which does not comply with these terms.
*Correspondence: Yasuo Sengoku, c2VuZ29rdS55YXN1by5nZUB1LnRzdWt1YmEuYWMuanA=