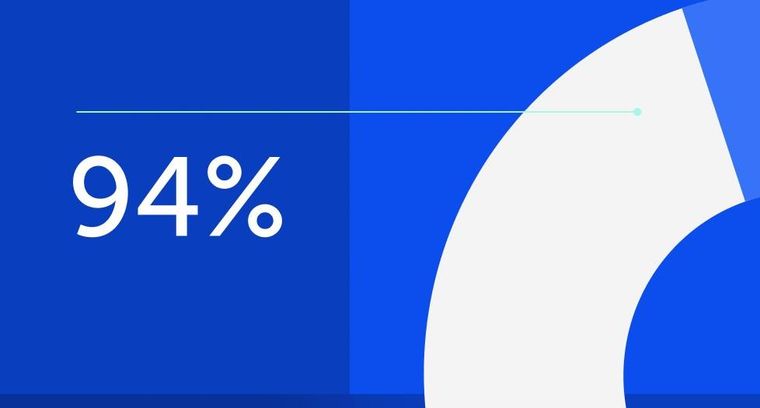
94% of researchers rate our articles as excellent or good
Learn more about the work of our research integrity team to safeguard the quality of each article we publish.
Find out more
HYPOTHESIS AND THEORY article
Front. Sports Act. Living, 03 April 2023
Sec. Exercise Physiology
Volume 5 - 2023 | https://doi.org/10.3389/fspor.2023.1122938
This article is part of the Research TopicEfficacy of Small Muscle Mass Exercise Training to Promote HealthView all 6 articles
The hypothesis is that the Fidget Factor is the innate neurological pulse that propels humans and other species to move to support their health. Fidgets, previously thought to be spontaneous, are neurologically regulated and highly ordered (non-random). Modern societies being chair-based overwhelm Fidget Factor pulses and consequently inflict chair-based living for transportation, labor, and leisure. Despite impulses firing through the nervous system, people sit because environmental design overwhelms the biology. Urbanization and chair-based societies were designed after the industrial revolution to promote productivity; however, the consequence has been opposite. Crushing the natural urge to move—the Fidget Factor—is a public health calamity. Excess sitting is associated with a myriad of detrimental health consequences and impairs productivity. Fidgeting may reduce all-cause mortality associated with excessive sitting. The Fidget Factor offers hope; data demonstrate that workplaces and schools can be designed to promote activity and free people's Fidget Factors. Evidence shows that people are happier, healthier, wealthier, and more successful if their Fidget Factors are freed.
The hypothesis is that the Fidget Factor is an innate and healthful drive for human beings to move. It has long been assumed that the small movements people make are spontaneous and random. The hypothesis addressed in this paper is that these small movements, called fidgets, are neurologically regulated, programmed, and highly ordered. Fidgets trigger body and limb movements and locomotion. While in agricultural times this drive was unfettered, in postindustrial modern environments, chair-based cues are pervasive and suppress people's innate drives to move. Because of suppressed Fidget Factors, people sit excessively. Excess sitting is associated with physical and mental illness and premature death. Solutions exist to reverse sedentariness and allow people's natural Fidget Factors to propagate healthful movement.
Meriam-Webster (1) defines the verb “to fidget” as “to move or act nervously or restlessly” and the noun “fidget” as a “nervous movement.” Fidgets characterize the spectrum of species that range from nematodes to humans. Common to all understandings of fidgets is that they are nervous in origin.
The Fidget Factor is under neurological regulation and integrated in the human energy regulation cycle (2–13). According to one example, Orexin A is a neuropeptide that is produced in caudal hypothalamic regions and projects throughout the neuraxis where it enhances arousal and stimulates the Fidget Factor (4). Orexin is one of several mediators of the Fidget Factor. When orexin is injected into the paraventricular nucleus in rats, it precipitates fidgets in a dose-dependent fashion (14). Paraventricular nucleus injections of an orexin receptor antagonist are associated with decreases in Fidget Factor responses (14). In transgenic mice where orexin-containing neurons are ablated, the phenotype includes inactivity and late-onset obesity, despite the transgenics eating less than non-transgenic littermates (15). Orexin A also stimulates the Fidget Factor codependently with feeding behavior (16) when injected into the lateral hypothalamus. Orexin impacts several hypothalamic nuclei to regulate fidgeting.
Orexin is not the only central mediator of the Fidget Factor; other neuromodulators include cholecystokinin, agouti-related protein, corticotropin-releasing factor, neuromedin U, neuropeptide Y, leptin, the serotonergic system, and ghrelin (12, 13, 17, 18). Several brain loci are involved as well; nucleus accumbens, for example, is considered the neural interface between motivation and movement and controls fidget-like movements (19). Movement is important in multiple facets of life such as feeding, foraging, and fleeing, and so, it is not surprising that the Fidget Factor represents the neurological end product of several central control centers and circuits.
It is not only mammals that have Fidget Factors. Molecular studies in zebrafish show that orexin mediates swimming activity and energy balance (20); worms fidget too (21). The Fidget Factor is ubiquitous in zoology and under intricate neurological control (22).
It has long been assumed that fidgets are spontaneous and random. To examine movements in free-living people, underwear was designed that included multiple sensors embedded in upper and lower undergarments (23); the underwear (Figure 1), thereby, captured all free-living body postures and body movements over 13 axes of motion every half second. The underwear sensing system was used to examine 10,362 free-living walking events and day-time and nighttime postures and movements in 21 people (24). Free-living walking comprised many (−47) short-duration (<15 min), low-velocity (−1 mph) walking bouts. Importantly, there was remarkable within-person consistency for the subvariables of free-living daily activity such as the number of walks a person takes per day, free-living walking velocity, and overall walking time (r2 values ranged 0.6–0.8). This suggests that free-living movement is not random and therefore regulated.
Figure 1. Multiple inclinometers and accelerometers integrated into underwear. Reproduced from Levine et al. (23).
Using modern mathematics, human fidgeting can be analyzed for entropy, which is a measure of randomness (25–30). ApEn determinations of entropy confirm that fidget-like human movements are non-random in infants (27, 29), young adults (25), adults, and in the aged (28, 31). When the orderliness of human movement is disrupted (e.g., jumping back in response to a fast-moving car), a person's physiological disorderliness with respect to movement rapidly self-corrects (32). There is the fitness effect too; the more physically conditioned a person the more orderly their fidgets (33). Human fidgets are highly ordered. The programed orderliness of human movement can be pathologically disrupted by illnesses such as in Parkinson's disease (34, 35) or by direct manipulation, for example, by asking women to walk in heels vs. flat shoes (30). Mechanistically, the rhythmicity of the Fidget Factor is mechanistically linked to the Clock gene, which is central to circadian timing; homozygous Clock mutant mice exhibit temporally disrupted activity patterns (36). The Fidget Factor is, therefore, organized and mechanistically encoded most likely via central modulators. Human movements are neither random nor spontaneous; as George Gershwin wrote, “I got rhythm” (Treasure Girl 1928). People have more rhythm than they realize. The Fidget Factor is an outward manifestation of an inner rhythm to move.
Noting the above and the relevance of fidgets in multiple species and across several genera, we can better define “a fidget” in a biological context. The initial definition of a fidget discussed above was “a nervous movement.” A fidget might be better defined as “a neurologically programmed rhythmic movement of a body part.” Under normal functioning, a fidget might be the spark that predicates a larger orchestrated movement whereby a foot flinch fidget begets a leg extension and precipitates a walk. Under pathological conditions, such as mutation of the HTT gene, a fidget might extend into the choreoathetoid movements that characterize Huntington's Chorea (37).
There are little fidgets (e.g., the tap of a finger) and big fidgets (e.g., the crossing of legs). Both fidgets have numerically different effects on human physiology (Figure 2).
Figure 2. The effect of fidgeting in different postures on human energy expenditure. Data (mean ± SD) are taken from 24 subjects (17 women and 7 men; body weight: 76 ± 21 kg) (38).
The Fidget Factor is centrally regulated and ordered, however, if it is not physiologically meaningful, its significance is less. The impact of the Fidget Factor on human physiology was examined in 16 lean volunteers (23). The subjects ate all their meals at a research center for 10 weeks; all meals were chemically analyzed for caloric content. For the first 2 weeks, each person was fed to establish the calorie intake necessary for weight maintenance to determine how many calories each volunteer required for achieving a steady state. Thereafter, each volunteer was overfed by 1,000 additional kcal per day, i.e., if a subject ate 2,700 kcal/day to maintain a steady state, this was increased to 3,700 kcal/day. In this fashion, each volunteer received an excess 56,000 kcal over 8 weeks.
There was a 7-fold variation in people's susceptibilities to weight gain (Figure 3). Some individuals were remarkably resistant to fat gain with overfeeding because they activated their Fidget Factors; the energy expended through non-exercise movement (39, 40). Increased non-exercise movements with overfeeding expended up to 700 kcal/day above usual energy expenditure. A statistically significant negative correlation (Figure 3) between fat gain and non-exercise movements suggested a mechanistic link, a proposition supported by animal data (41). The Fidget Factor is central in energy homeostasis.
Figure 3. Activation of the Fidget Factor, non-exercise activity thermogenesis (NEAT) in 16 individuals overfed by 1,000 kcal/day for 8 weeks. Body fat was measured using validated dual x-ray absorptiometry (23).
Overfeeding can increase energy expenditure via the Fidget Factor by 700 kcal/day. How is this possible without one going to a gym? Further overfeeding experiments were conducted but with subjects wearing multisensor undergarments (Figure 1). The results (42) showed that people activate their Fidget Factors by increasing locomotion. This is not achieved by exercise but by subconsciously and imperceptibly adjusting the mechanics and energetics of walking.
If overfeeding is important in a person's susceptibility to fat gain, is the Fidget Factor important in obesity? To understand the role of the Fidget Factor in mild obesity, lean and obese office workers were compared using the multisensor system shown in Figure 1. The results were dramatic. Lean people have activated Fidget Factors; they stand and move 2 1/4 h per day more than people with obesity. This appears to reflect a biological predisposition, because people who are active at work are active during their leisure time and people most sedentary at work are those most sedentary at home (24, 42). All subjects in these studies lived in obesogenic chair-based environments. Those with activated Fidget Factors were thin. For others, pervasive environmental cues to sit overrode the physiological impulse to move, resulting in obesity.
If the Fidget Factor changes in response to overfeeding and with obesity, how is it influenced by starvation and weight loss? Regardless of species, Fidget Factors change with acute starvation in a predictable fashion (7, 20, 43). Initially, starvation increases Fidget Factor activities, which is ascribable to foraging behavior. If food remains unavailable, physical activity will then progressively decline. When chronic caloric restriction results in weight loss, people's Fidget Factors reset to a new norm (44–46). Training and fitness levels also impact Fidget Factors in humans (47) and in other species (48–51). The Fidget Factor is clearly central in energy homeostasis.
The Fidget Factor is modulated across the life span (52). In utero (53), “spontaneous” movements are associated with development and limb growth (53). A systematic review of 15 studies showed a significant relationship between the quality of fidgety movements at 8–20 weeks post term and the infants' neurodevelopmental outcome. This association is specific as another systematic review showed, “the presence of abnormalities in the quality of fidgety movements at 12 weeks adjusted age is more predictive of adverse outcomes than abnormal writhing movements” (54). Fidgets in an infant are important for learning to walk and cognitive development (55, 56). Children are more fidgety than adults (48, 57), and then, the Fidget Factor declines with aging (58, 59) which may be important in sarcopenia (60). This pattern of high activity into adulthood and the decline with ageing is mirrored in other mammals (61), flies (62), and worms (21).
Fidgeting is not limited to humans. Multiple behaviors in non-human primates in the wild resemble human fidgeting (63). In primate experiments, cortical activity regulated cytoskeletal-associated protein and brain-derived neurotrophic factor expressions are strongly correlated with fidgeting activity (64). Furthermore, in monkey models of human neurological disease, phenotypes with diminished fidget-like movements mirror the human condition (65). Fidget-like movements occur across genera and are moderated over the life span in fish, flies, and worms (20, 21, 62). Interestingly, worms show a similar diminishment in “fidgets” (spontaneous movements) over the life span compared with humans, becoming near motionless just prior to death.
The Fidget Factor, controlled by multiple neurological loci, has an important role across the life span of many species that are central for growth and energy homeostasis.
There is an intersect between environment and biology. The Fidget Factors of some people appear to be insufficient to override obesogenic chair-based environments; such individuals sit too much and develop obesity. People whose Fidget Factors override environmental cues to sit remain mobile, active, and thin. How quantitatively important are these environmental drives on the Fidget Factor?
Since the Industrial Revolution, most of the world's population has relocated from agricultural communities to urban centers (66–69). Urbanization was initially meant to support factory production, but this developed into chair-based offices. To what extent has this massive demographic shift impacted the Fidget Factor?
Movement sensing undergarments (Figure 1) were used to compare agricultural and urban populations in Jamaica (70). The agricultural community included field workers, teachers, dancers, hairdressers, and educators. They were compared with weight-matched controls working in offices in the capital, Kingston. Ambulation was 60% greater in agricultural Jamaicans than in urban dwellers. Agricultural Jamaicans sat 4 h/day less than Americans with obesity (336 ± 68 min/day vs. 562 ± 78; P < 0.001). This illustrates the amount of excess sitting that resulted in response to urbanization—4 h more. Genetic vulnerability may help explain why some people respond more to environmental cues to sit than others (71). Nonetheless, environment is a key driver of the Fidget Factor. If people were liberated from their chairs, they could move for 4 h more every day (72, 73).
Agricultural workers sit between bouts of physical work and for leisure (73); the default posture is to work standing and exothermically and walk for transportation. In modern chair-based societies, sitting is the default posture and cars are used for transportation. For many people who live in chair-based societies, their Fidget Factors are suppressed; if these are released, people can healthily tolerate 4 h less sitting per day.
In contrast, there is ample empirical evidence from the affective computing literature that knowledge workers engage in extensive hand fidgeting during long and intense bouts of cognitive work (74). Furthermore, when the fidgeting cycle is disrupted during pathological conditions such as Huntington's Chorea (37), there is cognitive decline. It is fascinating how the state of anxiety is linked to fidgeting; in extreme anxiety, under adrenergic drive, tremulousness and elevated energy expenditure occur in concert (75). Environments impact biology: fidgetiness is a marker of heightened anxiety.
In a similar way that environment can impact a person's inmate fidgetiness, so does culture (76–79). Cultural group differences influence a person's likelihood to fidget; people from different cultures fidget differently in response to anxiety (77) and even when deliberately deceiving others (76).
While people have innate neurological drives to fidget and move, environment and culture can quash these drives, but at what cost?
Chair-based living and the environmental cues associated with it override peoples' natural tendencies to move—their Fidget factors are suppressed (80). This would not be problematic except that excessive sitting is harmful to health (81–83). Excess sitting is associated with metabolic, musculoskeletal problems, malignancy, and mortality (84–88); examples include cardiovascular disease, obesity (89), type two diabetes (90), hyperlipidemia, cardiovascular disease (91), hypertension, lower back pain (92), carpal tunnel syndrome, venous stasis (93), low mood, and a greater risk of malignancy (94–99).
Why is excess sitting so harmful? According to one example (100), healthy volunteers attended a research center and carried out normal chair-based work and leisure activities. Blood glucose was monitored continuously throughout the experiment. Breakfast, lunch, and dinner were provided and meal-related changes in blood glucose were recorded after each meal. For these subjects, their meal-associated glucose responses (incremental glucose area under the curve) were 9.6 mmol/L/270 min. The same volunteers repeated the same chair-based protocol with the duplicate meals except that after each meal, the subjects took a 30-min stroll at 2 km/h. Their meal-associated glucose responses were halved (4.5 mmol/L/270 min; P = 0.002), a finding replicated by others (101–103). Noting that meal-associated glucose responses predict the development of type 2 diabetes (104, 105), this experiment helps explain why chair-suppressed Fidget Factors are associated with type 2 diabetes (90, 106).
Similar experiments show the harmful effect of sedentariness on lipid metabolism (88) and that slow walking raises lipoprotein lipase activity beneficially by approximately 8-fold (107, 108). Furthermore, sedentariness is associated with proinflammatory markers, depressed sympathetic activity (109), and elevated insulin-like growth factors (110), which, in turn, are associated with malignancy (111). It is interesting to note from Morishima's carefully conducted studies of bilateral popliteal artery flow–mediated dilation that prolonged sitting-induced leg endothelial dysfunction may be prevented by fidgeting (112).
Anatomic and ergonomic analyses explain why musculoskeletal problems such as back pain and other mechanical disorders are linked to excess sitting (113, 114). Prolonged sitting is associated with spine flexion, low back disorders, disc stress, and pain (115). Back muscles are activated when a person stands, and similarly, the trunk muscles represent a counterbalance (116, 117). The musculoskeletal system is hurt when a person sits for hours without break.
It is not well understood as to why cognitive skills and mental health issues, especially depressed mood, are linked to excess sitting (118), but they are. Multiple prospective studies show that walking helps in preventing depression (119). An active body begets a happy mind!
Excess sitting has substantial detrimental health consequences and is associated with at least 34 different chronic diseases and conditions (113). The Fidget Factor is a pulsatile neurological impulse to move. Millions of people are sedentary because their natural Fidget Factors are suppressed by chair-based environments.
Almost no fidgeting intervention studied exist, epidemiological data suggest that fidgeting is associated with lower mortality risk (120). Analyzing data from the UK Women's Cohort Study, Hagger-Johnson et al. conclude, “Fidgeting may reduce the risk of all-cause mortality associated with excessive sitting time” (85).
The health consequences of sedentary living are calamitous, and people perish prematurely. If people were able to respond naturally to their innate Fidget Factors and move more, would they be healthier?
Scalable studies have taken place in workplaces and schools and demonstrate that Fidget Factor–permissive environments can be designed to reverse sedentariness and enable people to move (121). Such interventions are not straightforward because chair-based cues are pervasive at work and during leisure (122). Consequently, environmental redesign is only one part of the solution. Behavioral change strategies (123) are critical (Table 1) to help people reverse sedentariness, move (124), and “liberate” their Fidget Factors.
Table 1. Evidence-based behavioral change techniques that are effective for improving physical activity in healthy sedentary adults (124).
Workplaces and schools impose a group dynamic that is important in supporting individual change. Group behaviors are well studied in animals (125) but less in humans (126, 127). Although group behavioral dynamics are not well understood, workplace productivity is evaluated using group-based criteria such as a company's profitability. Similarly, schools are compared against whole-school performance criteria. Fidget Factor–permissive environments facilitate a healthier group dynamic, physically, mentally, and productivity wise.
The economic value of Fidget Factor–permissive environments has been established. Returns on investments have been measured in workplace and school interventions and have been found to be positive (128–131) and associated with improved health behaviors, decreased absenteeism, and better mental health (132–134). Importantly, employee wellbeing has become included in a company's Environmental, Social, and Governance (ESG) metrics, which are the standards socially conscious investors apply to screen investments. Workplace wellbeing measures are likely to expand.
Taken en masse, workplace interventions are associated with improved physical and mental health (135) and improved employee wellbeing and productivity (86, 136–141). Similarly in school children, active learning at the expense of chair-based learning is associated with improved education and health (142–145). These programs pay for themselves (146, 147) and the costs reflect the sum of healthcare cost savings, decreased absenteeism, and improved productivity/education. People are happier, healthier, wealthier, and more successful if their Fidget Factors are freed.
Fidget Factor, the rhythmic impulse to move, is programmed from deep within the brain stem. These impulses precipitate movements that range from barely perceptible fidgets to larger motions. Modern chair-based societies override people's innate Fidget Factors; people living in agricultural societies move 4 h a day more than overweight people in modern offices, suggesting that people who are not restricted by chairs naturally move several hours per day more than chair-based urban office workers (68, 69). Consequently, when people's Fidget Factors are suppressed, excess sitting is pervasive, and the physical and mental health consequences are dire. People die early from sedentariness. Fidget Factor–permissive environments enable people to be healthier, happier, smarter, and more productive (148–151). Solutions exist, but they need to be deployed.
The author confirms being the sole contributor of this work and has approved it for publication.
The author declares that the research was conducted in the absence of any commercial or financial relationships that could be construed as a potential conflict of interest.
All claims expressed in this article are solely those of the authors and do not necessarily represent those of their affiliated organizations, or those of the publisher, the editors and the reviewers. Any product that may be evaluated in this article, or claim that may be made by its manufacturer, is not guaranteed or endorsed by the publisher.
1. Merriam-Webster. “Fidget.” [Online]. Merriam-Webster. Available at: https://www.merriam-webster.com/dictionary/fidget (Accessed February 21, 2023).
2. Bunney PE, Zink AN, Holm AA, Billington CJ, Kotz CM. Orexin activation counteracts decreases in nonexercise activity thermogenesis (NEAT) caused by high-fat diet. Physiol Behav. (2017) 176:139–48. doi: 10.1016/j.physbeh.2017.03.040
3. Gac L, Butterick TA, Duffy CM, Teske JA, Perez-Leighton CE. Role of the non-opioid dynorphin peptide des-Tyr-dynorphin [DYN-A(2–17)] in food intake and physical activity, and its interaction with orexin-A. Peptides. (2016) 76:14–8. doi: 10.1016/j.peptides.2015.12.001
4. Kotz CM, Wang C, Teske JA, Thorpe AJ, Novak CM, Kiwaki K, et al. Orexin A mediation of time spent moving in rats: neural mechanisms. Neuroscience. (2006) 142:29–36. doi: 10.1016/j.neuroscience.2006.05.028
5. Zink AN, Bunney PE, Holm AA, Billington CJ, Kotz CM. Neuromodulation of orexin neurons reduces diet-induced adiposity. Int J Obes. (2018) 42:737–45. doi: 10.1038/ijo.2017.276
6. Olver TD, McDonald MW, Klakotskaia D, Richardson RA, Jasperse JL, Melling CWJ, et al. A chronic physical activity treatment in obese rats normalizes the contributions of ET-1 and NO to insulin-mediated posterior cerebral artery vasodilation. J Appl Physiol. (2017) 122:1040–50. doi: 10.1152/japplphysiol.00811.2016
7. Nixon JP, Kotz CM, Novak CM, Billington CJ, Teske JA. Neuropeptides controlling energy balance: orexins and neuromedins. Handb Exp Pharmacol. (2012) 209:77–109. doi: 10.1007/978-3-642-24716-3_4
8. Perez-Leighton CE, Boland K, Billington CJ, Kotz CM. High and low activity rats: elevated intrinsic physical activity drives resistance to diet-induced obesity in non-bred rats. Obesity. (2013) 21:353–60. doi: 10.1002/oby.20045
9. Teske JA, Billington CJ, Kotz CM. Mechanisms underlying obesity resistance associated with high spontaneous physical activity. Neuroscience. (2014) 256:91–100. doi: 10.1016/j.neuroscience.2013.10.028
10. Leak RK, Garbett KA, Dettmer AM, Zhang Z, Mirnics K, Cameron JL. Physical activity is linked to ceruloplasmin in the striatum of intact but not MPTP-treated primates. Cell Tissue Res. (2012) 350:401–7. doi: 10.1007/s00441-012-1488-3
11. Garland T Jr, Schutz H, Chappell MA, Keeney BK, Meek TH, Copes LE, et al. The biological control of voluntary exercise, spontaneous physical activity and daily energy expenditure in relation to obesity: human and rodent perspectives. J Exp Biol. (2011) 214:206–29. doi: 10.1242/jeb.048397
12. Teske JA, Billington CJ, Kotz CM. Neuropeptidergic mediators of spontaneous physical activity and non-exercise activity thermogenesis. Neuroendocrinology. (2008) 87:71–90. doi: 10.1159/000110802
13. Mavanji V, Pomonis B, Kotz CM. Orexin, serotonin, and energy balance. WIRES Mech Dis. (2022) 14:e1536. doi: 10.1002/wsbm.1536
14. Kiwaki K, Kotz CM, Wang C, Lanningham-Foster L, Levine JA. Orexin A (hypocretin 1) injected into hypothalamic paraventricular nucleus and spontaneous physical activity in rats. Am J Physiol Endocrinol Metab. (2004) 286:E551–9. doi: 10.1152/ajpendo.00126.2003
15. Hara J, Beuckmann CT, Nambu T, Willie JT, Chemelli RM, Sinton CM, et al. Genetic ablation of orexin neurons in mice results in narcolepsy, hypophagia, and obesity. Neuron. (2001) 30:345–54. doi: 10.1016/S0896-6273(01)00293-8
16. Kotz CM, Teske JA, Levine JA, Wang C. Feeding and activity induced by orexin A in the lateral hypothalamus in rats. Regul Pept. (2002) 104:27–32. doi: 10.1016/S0167-0115(01)00346-9
17. Trayhurn P, Bing C. Appetite and energy balance signals from adipocytes. Philos Trans R Soc Lond B Biol Sci. (2006) 361:1237–49. doi: 10.1098/rstb.2006.1859
18. Williams G, Harrold JA, Cutler DJ. The hypothalamus and the regulation of energy homeostasis: lifting the lid on a black box. Proc Nutr Soc. (2000) 59:385–96. doi: 10.1017/S0029665100000434
19. Shay DA, Welly RJ, Givan SA, Bivens N, Kanaley J, Marshall BL, et al. Changes in nucleus accumbens gene expression accompany sex-specific suppression of spontaneous physical activity in aromatase knockout mice. Horm Behav. (2020) 121:104719. doi: 10.1016/j.yhbeh.2020.104719
20. Novak CM, Jiang X, Wang C, Teske JA, Kotz CM, Levine JA. Caloric restriction and physical activity in zebrafish (Danio rerio). Neurosci Lett. (2005) 383:99–104. doi: 10.1016/j.neulet.2005.03.048
21. Kirkwood TB, Finch CE. Ageing: the old worm turns more slowly. Nature. (2002) 419:794–5. doi: 10.1038/419794a
22. Kotz CM. Integration of feeding and spontaneous physical activity: role for orexin. Physiol Behav. (2006) 88:294–301. doi: 10.1016/j.physbeh.2006.05.031
23. Levine JA, Lanningham-Foster LM, McCrady SK, Krizan AC, Olson LR, Kane PH, et al. Interindividual variation in posture allocation: possible role in human obesity. Science. (2005) 307:584–6. doi: 10.1126/science.1106561
24. McCrady SK, Levine JA. Sedentariness at work: how much do we really sit? Obesity. (2009) 17:2103–5. doi: 10.1038/oby.2009.117
25. Yentes JM, Denton W, McCamley J, Raffalt PC, Schmid KK. Effect of parameter selection on entropy calculation for long walking trials. Gait Posture. (2018) 60:128–34. doi: 10.1016/j.gaitpost.2017.11.023
26. Morrison S, Hong SL, Newell KM. Inverse relations in the patterns of muscle and center of pressure dynamics during standing still and movement postures. Exp Brain Res. (2007) 181:347–58. doi: 10.1007/s00221-007-0928-x
27. Smith BA, Teulier C, Sansom J, Stergiou N, Ulrich BD. Approximate entropy values demonstrate impaired neuromotor control of spontaneous leg activity in infants with myelomeningocele. Pediatr Phys Ther. (2011) 23:241–7. doi: 10.1097/PEP.0b013e3182289ae4
28. Yu SH, Huang CY. Improving posture-motor dual-task with a supraposture-focus strategy in young and elderly adults. PLoS One. (2017) 12:e0170687. doi: 10.1371/journal.pone.0170687
29. Dusing SC, Kyvelidou A, Mercer VS, Stergiou N. Infants born preterm exhibit different patterns of center-of-pressure movement than infants born at full term. Phys Ther. (2009) 89:1354–62. doi: 10.2522/ptj.20080361
30. Alkjær T, Raffalt P, Petersen NC, Simonsen EB. Movement behavior of high-heeled walking: how does the nervous system control the ankle joint during an unstable walking condition? PLoS One. (2012) 7:e37390. doi: 10.1371/journal.pone.0037390
31. Karmakar CK, Khandoker AH, Begg RK, Palaniswami M, Taylor S. Understanding ageing effects by approximate entropy analysis of gait variability. Annu Int Conf IEEE Eng Med Biol Soc. (2007) 2007:1965–8. doi: 10.1109/IEMBS.2007.4352703
32. Thomas KS, Vanlunen BL, Morrison S. Changes in postural sway as a function of prolonged walking. Eur J Appl Physiol. (2013) 113:497–508. doi: 10.1007/s00421-012-2456-z
33. Solís-Montufar EE, Gálvez-Coyt G, Muñoz-Diosdado A. Entropy analysis of RR-time series from stress tests. Front Physiol. (2020) 11:981. doi: 10.3389/fphys.2020.00981
34. Huang CY, Chen YA, Hwang IS, Wu RM. Improving dual-task control with a posture-second strategy in early-stage Parkinson disease. Arch Phys Med Rehabil. (2018) 99:1540–6.e2. doi: 10.1016/j.apmr.2018.02.013
35. Gates P, Discenzo FM, Kim JH, Lemke Z, Meggitt J, Ridgel AL. Analysis of movement entropy during community dance programs for people with Parkinson’s Disease and older adults: a cohort study. Int J Environ Res Public Health. (2022) 19:1–6. doi: 10.3390/ijerph19020655
36. Turek FW, Joshu C, Kohsaka A, Lin E, Ivanova G, Mcdearmon E, et al. Obesity and metabolic syndrome in circadian Clock mutant mice. Science. (2005) 308:1043–5. doi: 10.1126/science.1108750
37. Zeitler B, Froelich S, Marlen K, Shivak DA, Yu Q, Li D, et al. Allele-selective transcriptional repression of mutant HTT for the treatment of Huntington’s disease. Nat Med. (2019) 25:1131–42. doi: 10.1038/s41591-019-0478-3
38. Levine JA, Schleusner SJ, Jensen MD. Energy expenditure of nonexercise activity. Am J Clin Nutr. (2000) 72:1451–4. doi: 10.1093/ajcn/72.6.1451
39. Graziano PA, Garcia AM, Landis TD. To fidget or not to fidget, that is the question: a systematic classroom evaluation of fidget spinners among young children with ADHD. J Atten Disord. (2020) 24:163–71. doi: 10.1177/1087054718770009
40. Ravussin E. A NEAT way to control weight? Science. (2005) 307:530–1. doi: 10.1126/science.1108597
41. Kotz C, Nixon J, Butterick T, Perez-Leighton C, Teske J, Billington C. Brain orexin promotes obesity resistance. Ann N Y Acad Sci. (2012) 1264:72–86. doi: 10.1111/j.1749-6632.2012.06585.x
42. Levine JA, McCrady SK, Lanningham-Foster LM, Kane PH, Foster RC, Manohar CU. The role of free-living daily walking in human weight gain and obesity. Diabetes. (2008) 57:548–54. doi: 10.2337/db07-0815
43. Novak CM, Escande C, Burghardt PR, Zhang M, Barbosa MT, Chini EN, et al. Spontaneous activity, economy of activity, and resistance to diet-induced obesity in rats bred for high intrinsic aerobic capacity. Horm Behav. (2010) 58:355–67. doi: 10.1016/j.yhbeh.2010.03.013
44. Teske JA, Kotz CM. Effect of acute and chronic caloric restriction and metabolic glucoprivation on spontaneous physical activity in obesity-prone and obesity-resistant rats. Am J Physiol Regul Integr Comp Physiol. (2009) 297:R176–84. doi: 10.1152/ajpregu.90866.2008
45. Martin CK, Heilbronn LK, De Jonge L, Delany JP, Volaufova J, Anton SD, et al. Effect of calorie restriction on resting metabolic rate and spontaneous physical activity. Obesity. (2007) 15:2964–73. doi: 10.1038/oby.2007.354
46. Parashar V, Rogina B. Dsir2 mediates the increased spontaneous physical activity in flies on calorie restriction. Aging. (2009) 1:529–41. doi: 10.18632/aging.100061
47. Yamada Y, Kemnitz JW, Weindruch R, Anderson RM, Schoeller DA, Colman RJ. Caloric restriction and healthy life span: frail phenotype of nonhuman primates in the Wisconsin National Primate Research Center Caloric Restriction study. J Gerontol A Biol Sci Med Sci. (2018) 73:273–8. doi: 10.1093/gerona/glx059
48. Eiholzer U, Meinhardt U, Petrò R, Witassek F, Gutzwiller F, Gasser T. High-intensity training increases spontaneous physical activity in children: a randomized controlled study. J Pediatr. (2010) 156:242–6. doi: 10.1016/j.jpeds.2009.08.039
49. Schmitz KH, Jensen MD, Kugler KC, Jeffery RW, Leon AS. Strength training for obesity prevention in midlife women. Int J Obes Relat Metab Disord. (2003) 27:326–33. doi: 10.1038/sj.ijo.0802198
50. Banz WJ, Maher MA, Thompson WG, Bassett DR, Moore W, Ashraf M, et al. Effects of resistance versus aerobic training on coronary artery disease risk factors. Exp Biol Med. (2003) 228:434–40. doi: 10.1177/153537020322800414
51. Meijer EP, Westerterp KR, Verstappen FT. Effect of exercise training on total daily physical activity in elderly humans. Eur J Appl Physiol Occup Physiol. (1999) 80:16–21. doi: 10.1007/s004210050552
52. Johannsen DL, Ravussin E. Spontaneous physical activity: relationship between fidgeting and body weight control. Curr Opin Endocrinol Diabetes Obes. (2008) 15:409–15. doi: 10.1097/MED.0b013e32830b10bb
53. Liu Y, Xuan R, He Y, Ren F, Gu Y. Computation of fetal kicking in various fetal health examinations: a systematic review. Int J Environ Res Public Health. (2022) 19(7):4366. doi: 10.3390/ijerph19074366
54. Darsaklis V, Snider LM, Majnemer A, Mazer B. Predictive validity of Prechtl’s method on the qualitative assessment of general movements: a systematic review of the evidence. Dev Med Child Neurol. (2011) 53:896–906. doi: 10.1111/j.1469-8749.2011.04017.x
55. Mowbray R, Cowie D. Mind your step: learning to walk in complex environments. Exp Brain Res. (2020) 238:1455–65. doi: 10.1007/s00221-020-05821-y
56. Wright CM, Duquesnay PJ, Anzman-Frasca S, Chomitz VR, Chui K, Economos CD, et al. Study protocol: the Fueling Learning through Exercise (FLEX) study—a randomized controlled trial of the impact of school-based physical activity programs on children’s physical activity, cognitive function, and academic achievement. BMC Public Health. (2016) 16:1078. doi: 10.1186/s12889-016-3719-0
57. Boldemann C, Blennow M, Dal H, Mårtensson F, Raustorp A, Yuen K, et al. Impact of preschool environment upon children’s physical activity and sun exposure. Prev Med. (2006) 42:301–8. doi: 10.1016/j.ypmed.2005.12.006
58. Harris AM, Lanningham-Foster LM, McCrady SK, Levine JA. Nonexercise movement in elderly compared with young people. Am J Physiol Endocrinol Metab. (2007) 292:E1207–12. doi: 10.1152/ajpendo.00509.2006
59. Westerterp KR. Daily physical activity and ageing. Curr Opin Clin Nutr Metab Care. (2000) 3:485–8. doi: 10.1097/00075197-200011000-00011
60. Trounce I, Byrne E, Marzuki S. Decline in skeletal muscle mitochondrial respiratory chain function: possible factor in ageing. Lancet. (1989) 1:637–9. doi: 10.1016/S0140-6736(89)92143-0
61. Skalicky M, Bubna-Littitz H, Viidik A. Influence of physical exercise on aging rats: i. Life-long exercise preserves patterns of spontaneous activity. Mech Ageing Dev. (1996) 87:127–39. doi: 10.1016/0047-6374(96)01707-1
62. Woods JK, Ziafazeli T, Rogina B. The effects of reduced rpd3 levels on fly physiology. Nutr Healthy Aging. (2017) 4:169–79. doi: 10.3233/NHA-160016
63. Whiten A, Goodall J, Mcgrew WC, Nishida T, Reynolds V, Sugiyama Y, et al. Cultures in chimpanzees. Nature. (1999) 399:682–5. doi: 10.1038/21415
64. Mitchell AC, Aldridge G, Kohler S, Stanton G, Sullivan E, Garbett K, et al. Molecular correlates of spontaneous activity in non-human primates. J Neural Transm. (2010) 117:1353–8. doi: 10.1007/s00702-010-0483-7
65. Sherman LS, Su W, Johnson AL, Peterson SM, Cullin C, Lavinder T, et al. A novel non-human primate model of Pelizaeus–Merzbacher disease. Neurobiol Dis. (2021) 158:105465. doi: 10.1016/j.nbd.2021.105465
66. Yamauchi T, Umezaki M, Ohtsuka R. Influence of urbanisation on physical activity and dietary changes in Huli-speaking population: a comparative study of village dwellers and migrants in urban settlements. Br J Nutr. (2001) 85:65–73. doi: 10.1079/BJN2000208
67. Barr AL, Partap U, Young EH, Agoudavi K, Balde N, Kagaruki GB, et al. Sociodemographic inequities associated with participation in leisure-time physical activity in sub-Saharan Africa: an individual participant data meta-analysis. BMC Public Health. (2020) 20:927. doi: 10.1186/s12889-020-08987-w
68. Shanahan DF, Franco L, Lin BB, Gaston KJ, Fuller RA. The benefits of natural environments for physical activity. Sports Med. (2016) 46:989–95. doi: 10.1007/s40279-016-0502-4
69. Lam TM, Vaartjes I, Grobbee DE, Karssenberg D, Lakerveld J. Associations between the built environment and obesity: an umbrella review. Int J Health Geogr. (2021) 20:7. doi: 10.1186/s12942-021-00260-6
70. Levine JA, McCrady SK, Boyne S, Smith J, Cargill K, Forrester T. Non-exercise physical activity in agricultural and urban people. Urban Stud. (2011) 48:2417–27. doi: 10.1177/0042098010379273
71. Schnurr TM, Stallknecht BM, Sørensen TIA, Kilpeläinen TO, Hansen T. Evidence for shared genetics between physical activity, sedentary behaviour and adiposity-related traits. Obes Rev. (2021) 22:e13182. doi: 10.1111/obr.13182
72. Levine JA, Weisell R, Chevassus S, Martinez CD, Burlingame B, Coward WA. The work burden of women. Science. (2001) 294:812. doi: 10.1126/science.1064627
73. Singh J, Prentice AM, Diaz E, Coward WA, Ashford J, Sawyer M, et al. Energy expenditure of Gambian women during peak agricultural activity measured by the doubly-labelled water method. Br J Nutr. (1989) 62:315–29. doi: 10.1079/BJN19890033
74. Akleman E, Hasan MT, Pavlidis I. Under the spell of deadlines. Extended Abstracts of the 2021 CHI Conference on Human Factors in Computing Systems. (2021). p. 1–12.
75. Greene BHC, Lalonde DH, Seal SKF. Incidence of the “Adrenaline rush” and vasovagal response with local anesthetic injection. Plast Reconstr Surg Glob Open. (2021) 9:e3659. doi: 10.1097/GOX.0000000000003659
76. Van Der Zee S, Poppe R, Taylor PJ, Anderson R. To freeze or not to freeze: a culture-sensitive motion capture approach to detecting deceit. PLoS One. (2019) 14:e0215000. doi: 10.1371/journal.pone.0215000
77. Kim Y, Williams AI, Liu C, Zhou Q. Dynamic associations between emotion expressions and strategy use in Chinese American and Mexican American preschoolers. Emotion. (2023) 23(2):460–72. doi: 10.1037/emo0001100
78. Okazaki S, Liu JF, Longworth SL, Minn JY. Asian American-white American differences in expressions of social anxiety: a replication and extension. Cultur Divers Ethnic Minor Psychol. (2002) 8:234–47. doi: 10.1037/1099-9809.8.3.234
79. Dang HM, Nguyen H, Weiss B. Incremental validity of the Child Behavior Checklist (CBCL) and the Strengths and Difficulties Questionnaire (SDQ) in Vietnam. Asian J Psychiatr. (2017) 29:96–100. doi: 10.1016/j.ajp.2017.04.023
80. Levine JA. Nonexercise activity thermogenesis—liberating the life-force. J Intern Med. (2007) 262:273–87. doi: 10.1111/j.1365-2796.2007.01842.x
81. Ekelund U, Steene-Johannessen J, Brown WJ, Fagerland MW, Owen N, Powell KE, et al. Does physical activity attenuate, or even eliminate, the detrimental association of sitting time with mortality? A harmonised meta-analysis of data from more than 1 million men and women. Lancet. (2016) 388:1302–10. doi: 10.1016/S0140-6736(16)30370-1
82. Thorp AA, Owen N, Neuhaus M, Dunstan DW. Sedentary behaviors and subsequent health outcomes in adults a systematic review of longitudinal studies, 1996–2011. Am J Prev Med. (2011) 41:207–15. doi: 10.1016/j.amepre.2011.05.004
83. Manini TM, Everhart JE, Patel KV, Schoeller DA, Colbert LH, Visser M, et al. Daily activity energy expenditure and mortality among older adults. JAMA. (2006) 296:171–9. doi: 10.1001/jama.296.2.171
84. McManus AM, Ainslie PN, Green DJ, Simair RG, Smith K, Lewis N. Impact of prolonged sitting on vascular function in young girls. Exp Physiol. (2015) 100:1379–87. doi: 10.1113/EP085355
85. Hagger-Johnson G, Gow AJ, Burley V, Greenwood D, Cade JE. Sitting time, fidgeting, and all-cause mortality in the UK Women’s Cohort Study. Am J Prev Med. (2016) 50:154–60. doi: 10.1016/j.amepre.2015.06.025
86. Bailey DP. Sedentary behaviour in the workplace: prevalence, health implications and interventions. Br Med Bull. (2021) 137:42–50. doi: 10.1093/bmb/ldaa039
87. Dempsey PC, Owen N, Yates TE, Kingwell BA, Dunstan DW. Sitting less and moving more: improved glycaemic control for type 2 diabetes prevention and management. Curr Diab Rep. (2016) 16:114. doi: 10.1007/s11892-016-0797-4
88. Füzéki E, Engeroff T, Banzer W. Health benefits of light-intensity physical activity: a systematic review of accelerometer data of the National Health and Nutrition Examination Survey (NHANES). Sports Med. (2017) 47:1769–93. doi: 10.1007/s40279-017-0724-0
89. Zurlo F, Ferraro RT, Fontvielle AM, Rising R, Bogardus C, Ravussin E. Spontaneous physical activity and obesity: cross-sectional and longitudinal studies in Pima Indians. Am J Physiol. (1992) 263:E296–300. doi: 10.1152/ajpendo.1992.263.2.E296
90. Solomon TP, Thyfault JP. Type 2 diabetes sits in a chair. Diabetes Obes Metab. (2013) 15:987–92. doi: 10.1111/dom.12105
91. Stewart RAH, Held C, Hadziosmanovic N, Armstrong PW, Cannon CP, Granger CB, et al. Physical activity and mortality in patients with stable coronary heart disease. J Am Coll Cardiol. (2017) 70:1689–700. doi: 10.1016/j.jacc.2017.08.017
92. Citko A, Górski S, Marcinowicz L, Górska A. Sedentary lifestyle and nonspecific low back pain in medical personnel in North-East Poland. Biomed Res Int. (2018) 2018:1965807. doi: 10.1155/2018/1965807
93. Padilla J, Fadel PJ. Prolonged sitting leg vasculopathy: contributing factors and clinical implications. Am J Physiol Heart Circ Physiol. (2017) 313:H722–8. doi: 10.1152/ajpheart.00326.2017
94. Schnohr P, Gronbaek M, Petersen L, Hein HO, Sorensen TI. Physical activity in leisure-time and risk of cancer: 14-year follow-up of 28,000 Danish men and women. Scand J Public Health. (2005) 33:244–9. doi: 10.1080/14034940510005752
95. Steindorf K, Jedrychowski W, Schmidt M, Popiela T, Penar A, Galas A, et al. Case-control study of lifetime occupational and recreational physical activity and risks of colon and rectal cancer. Eur J Cancer Prev. (2005) 14:363–71. doi: 10.1097/00008469-200508000-00009
96. Tardon A, Lee WJ, Delgado-Rodriguez M, Dosemeci M, Albanes D, Hoover R, et al. Leisure-time physical activity and lung cancer: a meta-analysis. Cancer Causes Control. (2005) 16:389–97. doi: 10.1007/s10552-004-5026-9
97. Macera CA. Past recreational physical activity and risk of breast cancer. Clin J Sport Med. (2005) 15:115–6. doi: 10.1097/01.jsm.0000151868.60437.b9
98. Patel AV, Rodriguez C, Jacobs EJ, Solomon L, Thun MJ, Calle EE. Recreational physical activity and risk of prostate cancer in a large cohort of U.S. men. Cancer Epidemiol Biomarkers Prev. (2005) 14:275–9. doi: 10.1158/1055-9965.275.14.1
99. Patel AV, Callel EE, Bernstein L, Wu AH, Thun MJ. Recreational physical activity and risk of postmenopausal breast cancer in a large cohort of US women. Cancer Causes Control. (2003) 14:519–29. doi: 10.1023/A:1024895613663
100. Manohar C, Levine JA, Nandy DK, Saad A, Dalla Man C, McCrady-Spitzer SK, et al. The effect of walking on postprandial glycemic excursion in patients with type 1 diabetes and healthy people. Diabetes Care. (2012) 35:2493–9. doi: 10.2337/dc11-2381
101. Li Z, Hu Y, Yan R, Li H, Zhang D, Li F, et al. Twenty minute moderate-intensity post-dinner exercise reduces the postprandial glucose response in Chinese patients with type 2 diabetes. Med Sci Monit. (2018) 24:7170–7. doi: 10.12659/MSM.910827
102. Dunstan DW, Kingwell BA, Larsen R, Healy GN, Cerin E, Hamilton MT, et al. Breaking up prolonged sitting reduces postprandial glucose and insulin responses. Diabetes Care. (2012) 35:976–83. doi: 10.2337/dc11-1931
103. Zderic TW, Hamilton MT. Physical inactivity amplifies the sensitivity of skeletal muscle to the lipid-induced downregulation of lipoprotein lipase activity. J Appl Physiol. (2006) 100:249–57. doi: 10.1152/japplphysiol.00925.2005
104. Augustin LSA, Kendall CWC, Jenkins DJA, Willett WC, Astrup A, Barclay AW, et al. Glycemic index, glycemic load and glycemic response: an International Scientific Consensus Summit from the International Carbohydrate Quality Consortium (ICQC). Nutr Metab Cardiovasc Dis. (2015) 25:795–815. doi: 10.1016/j.numecd.2015.05.005
105. Stephenson EJ, Smiles W, Hawley JA. The relationship between exercise, nutrition and type 2 diabetes. Med Sport Sci. (2014) 60:1–10. doi: 10.1159/000357331
106. Hamilton MT, Hamilton DG, Zderic TW. Sedentary behavior as a mediator of type 2 diabetes. Med Sport Sci. (2014) 60:11–26. doi: 10.1159/000357332
107. Bey L, Hamilton MT. Suppression of skeletal muscle lipoprotein lipase activity during physical inactivity: a molecular reason to maintain daily low-intensity activity. J Physiol. (2003) 551:673–82. doi: 10.1113/jphysiol.2003.045591
108. Hamilton MT, Hamilton DG, Zderic TW. Exercise physiology versus inactivity physiology: an essential concept for understanding lipoprotein lipase regulation. Exerc Sport Sci Rev. (2004) 32:161–6. doi: 10.1097/00003677-200410000-00007
109. Spraul M, Ravussin E, Fontvieille AM, Rising R, Larson DE, Anderson EA. Reduced sympathetic nervous activity. A potential mechanism predisposing to body weight gain. J Clin Invest. (1993) 92:1730–5. doi: 10.1172/JCI116760
110. Poehlman ET, Copeland KC. Influence of physical activity on insulin-like growth factor-I in healthy younger and older men. J Clin Endocrinol Metab. (1990) 71:1468–73. doi: 10.1210/jcem-71-6-1468
111. Cao J, Yan W, Ma X, Huang H, Yan H. Insulin-like growth factor 2 mRNA-binding protein 2-a potential link between type 2 diabetes mellitus and cancer. J Clin Endocrinol Metab. (2021) 106:2807–18. doi: 10.1210/clinem/dgab391
112. Morishima T, Restaino RM, Walsh LK, Kanaley JA, Fadel PJ, Padilla J. Prolonged sitting-induced leg endothelial dysfunction is prevented by fidgeting. Am J Physiol Heart Circ Physiol. (2016) 311:H177–82. doi: 10.1152/ajpheart.00297.2016
113. Daneshmandi H, Choobineh A, Ghaem H, Karimi M. Adverse effects of prolonged sitting behavior on the general health of office workers. J Lifestyle Med. (2017) 7:69–75. doi: 10.15280/jlm.2017.7.2.69
114. Pesola AJ, Pekkonen M, Finni T. Why is excessive sitting a health risk? Duodecim. (2016) 132:1964–71. PMID: 29190048
115. McGill SM, Fenwick CM. Using a pneumatic support to correct sitting posture for prolonged periods: a study using airline seats. Ergonomics. (2009) 52:1162–8. doi: 10.1080/00140130902936067
116. Callaghan JP, McGill SM. Low back joint loading and kinematics during standing and unsupported sitting. Ergonomics. (2001) 44:280–94. doi: 10.1080/00140130118276
117. Motmans RR, Tomlow S, Vissers D. Trunk muscle activity in different modes of carrying schoolbags. Ergonomics. (2006) 49:127–38. doi: 10.1080/00140130500435066
118. Chandrasekaran B, Pesola AJ, Rao CR, Arumugam A. Does breaking up prolonged sitting improve cognitive functions in sedentary adults? A mapping review and hypothesis formulation on the potential physiological mechanisms. BMC Musculoskelet Disord. (2021) 22:274. doi: 10.1186/s12891-021-04136-5
119. Mammen G, Faulkner G. Physical activity and the prevention of depression: a systematic review of prospective studies. Am J Prev Med. (2013) 45:649–57. doi: 10.1016/j.amepre.2013.08.001
120. Pavey TG, Pulsford R. Fidgeting is associated with lower mortality risk. Evid Based Med. (2016) 21:109. doi: 10.1136/ebmed-2016-110410
121. Reis RS, Salvo D, Ogilvie D, Lambert EV, Goenka S, Brownson RC. Scaling up physical activity interventions worldwide: stepping up to larger and smarter approaches to get people moving. Lancet. (2016) 388:1337–48. doi: 10.1016/S0140-6736(16)30728-0
122. Levine JA. Lethal sitting: homo sedentarius seeks answers. Physiology. (2014) 29:300–1. doi: 10.1152/physiol.00034.2014
123. Winter SJ, Sheats JL, King AC. The use of behavior change techniques and theory in technologies for cardiovascular disease prevention and treatment in adults: a comprehensive review. Prog Cardiovasc Dis. (2016) 58(6):605–12. doi: 10.1016/j.pcad.2016.02.005
124. Howlett N, Trivedi D, Troop NA, Chater AM. Are physical activity interventions for healthy inactive adults effective in promoting behavior change and maintenance, and which behavior change techniques are effective? A systematic review and meta-analysis. Transl Behav Med. (2019) 9:147–57. doi: 10.1093/tbm/iby010
125. Soonberg M, Kaart T, Arney DR. How does changing the feeding bin affect cows’ behaviour? J Dairy Res. (2019) 86:43–7. doi: 10.1017/S0022029919000050
126. Ahmed QA, Memish ZA. From the “Madding Crowd” to mass gatherings-religion, sport, culture and public health. Travel Med Infect Dis. (2019) 28:91–7. doi: 10.1016/j.tmaid.2018.06.001
127. Stönner C, Williams J. European football: goals change crowd air chemistry. Nature. (2016) 535:355. doi: 10.1038/535355a
128. Ben-Ner A, Hamann DJ, Koepp G, Manohar CU, Levine J. Treadmill workstations: the effects of walking while working on physical activity and work performance. PLoS One. (2014) 9:e88620. doi: 10.1371/journal.pone.0088620
129. Koepp GA, Manohar CU, McCrady-Spitzer SK, Ben-Ner A, Flint-Paulson D, Runge CF, et al. Treadmill desks: a 1-year prospective trial. Obesity. (2013) 2013:705–11. doi: 10.1002/oby.20121
130. Koepp GA, Snedden BJ, Flynn L, Puccinelli D, Huntsman B, Levine JA. Feasibility analysis of standing desks for sixth graders. Infant Child Adolesc Nutr. (2012) 4:89–92. doi: 10.1177/1941406412439414
131. Livingstone AG, Spears R, Manstead AS. The language of change? Characterizations of in-group social position, threat, and the deployment of distinctive group attributes. Br J Soc Psychol. (2009) 48:295–311. doi: 10.1348/014466608X329533
132. de Oliveira C, Cho E, Kavelaars R, Jamieson M, Bao B, Rehm J. Economic analyses of mental health and substance use interventions in the workplace: a systematic literature review and narrative synthesis. Lancet Psychiatry. (2020) 7:893–910. doi: 10.1016/S2215-0366(20)30145-0
133. Song Z, Baicker K. Effect of a workplace wellness program on employee health and economic outcomes: a randomized clinical trial. JAMA. (2019) 321:1491–501. doi: 10.1001/jama.2019.3307
134. Van Dongen JM, Coffeng JK, Van Wier MF, Boot CRL, Hendriksen IJM, Van Mechelen W, et al. The cost-effectiveness and return-on-investment of a combined social and physical environmental intervention in office employees. Health Educ Res. (2017) 32:384–98. doi: 10.1093/her/cyx055
135. Puig-Ribera A, Martínez-Lemos I, Giné-Garriga M, González-Suárez ÁM, Bort-Roig J, Fortuño J, et al. Self-reported sitting time and physical activity: interactive associations with mental well-being and productivity in office employees. BMC Public Health. (2015) 15:72. doi: 10.1186/s12889-015-1447-5
136. Parry SP, Coenen P, Shrestha N, O’Sullivan PB, Maher CG, Straker LM. Workplace interventions for increasing standing or walking for decreasing musculoskeletal symptoms in sedentary workers. Cochrane Database Syst Rev. (2019) 2019(11):CD012487. doi: 10.1002/14651858.CD012487.pub2
137. Kruse NT, Hughes WE, Benzo RM, Carr LJ, Casey DP. Workplace strategies to prevent sitting-induced endothelial dysfunction. Med Sci Sports Exerc. (2018) 50:801–8. doi: 10.1249/MSS.0000000000001484
138. Mendez-Hernandez P, Dosamantes-Carrasco D, Siani C, Flores YN, Arredondo A, Lumbreras-Delgado I, et al. A workplace physical activity program at a public university in Mexico can reduce medical costs associated with type 2 diabetes and hypertension. Salud Publica Mex (2012) 54:20–7. doi: 10.1590/S0036-36342012000100004
139. Thomas L, Williams M. Promoting physical activity in the workplace: using pedometers to increase daily activity levels. Health Promot J Austr. (2006) 17:97–102. doi: 10.1071/HE06097
140. Rosenkranz SK, Mailey EL, Umansky E, Rosenkranz RR, Ablah E. Workplace sedentary behavior and productivity: a cross-sectional study. Int J Environ Res Public Health. (2020) 17(18):6535. doi: 10.3390/ijerph17186535
141. Nooijen CFJ, Blom V, Ekblom Ö, Ekblom MM, Kallings LV. Improving office workers’ mental health and cognition: a 3-arm cluster randomized controlled trial targeting physical activity and sedentary behavior in multi-component interventions. BMC Public Health. (2019) 19:266. doi: 10.1186/s12889-019-6589-4
142. Van Sluijs EMF, Ekelund U, Crochemore-Silva I, Guthold R, Ha A, Lubans D, et al. Physical activity behaviours in adolescence: current evidence and opportunities for intervention. Lancet. (2021) 398:429–42. doi: 10.1016/S0140-6736(21)01259-9
143. Swartz AM, Tokarek NR, Lisdahl K, Maeda H, Strath SJ, Cho CC. Do stand-biased desks in the classroom change school-time activity and sedentary behavior? Int J Environ Res Public Health. (2019) 16(6):933. doi: 10.3390/ijerph16060933
144. Arundell L, Fletcher E, Salmon J, Veitch J, Hinkley T. A systematic review of the prevalence of sedentary behavior during the after-school period among children aged 5–18 years. Int J Behav Nutr Phys Act. (2016) 13:93. doi: 10.1186/s12966-016-0419-1
145. Barbosa SC, Coledam DH, Stabelini Neto A, Elias RG, Oliveira AR. School environment, sedentary behavior and physical activity in preschool children. Rev Paul Pediatr. (2016) 34:301–8. doi: 10.1016/j.rpped.2016.01.001
146. Loitz CC, Potter RJ, Walker JL, Mcleod NC, Johnston NJ. The effectiveness of workplace interventions to increase physical activity and decrease sedentary behaviour in adults: protocol for a systematic review. Syst Rev. (2015) 4:178. doi: 10.1186/s13643-015-0166-4
147. Nguyen P, Le LK, Ananthapavan J, Gao L, Dunstan DW, Moodie M. Economics of sedentary behaviour: a systematic review of cost of illness, cost-effectiveness, and return on investment studies. Prev Med. (2022) 156:106964. doi: 10.1016/j.ypmed.2022.106964
148. Lin YT, Chen M, Ho CC, Lee TS. Relationships among leisure physical activity, sedentary lifestyle, physical fitness, and happiness in adults 65 years or older in Taiwan. Int J Environ Res Public Health. (2020) 17(14):5235. doi: 10.3390/ijerph17145235
149. Pengpid S, Peltzer K. Sedentary behaviour, physical activity and life satisfaction, happiness and perceived health status in university students from 24 countries. Int J Environ Res Public Health. (2019) 16(12):2084. doi: 10.3390/ijerph16122084
150. Yasunaga A, Koohsari MJ, Shibata A, Ishii K, Miyawaki R, Araki K, et al. Sedentary behavior and happiness: the mediation effects of social capital. Innov Aging. (2021) 5:igab044. doi: 10.1093/geroni/igab044
151. Rodriguez-Ayllon M, Cadenas-Sánchez C, Estévez-López F, Muñoz NE, Mora-Gonzalez J, Migueles JH, et al. Role of physical activity and sedentary behavior in the mental health of preschoolers, children and adolescents: a systematic review and meta-analysis. Sports Med. (2019) 49:1383–410. doi: 10.1007/s40279-019-01099-5
Keywords: physical activity, fidget, obesity, diabetes, neural circuits, school–environment relations
Citation: Levine JA (2023) The Fidget Factor and the obesity paradox. How small movements have big impact. Front. Sports Act. Living 5:1122938. doi: 10.3389/fspor.2023.1122938
Received: 13 December 2022; Accepted: 24 February 2023;
Published: 3 April 2023.
Edited by:
Ryan Broxterman, The University of Utah, United StatesReviewed by:
Ioannis Pavlidis, University of Houston, United States© 2023 Levine. This is an open-access article distributed under the terms of the Creative Commons Attribution License (CC BY). The use, distribution or reproduction in other forums is permitted, provided the original author(s) and the copyright owner(s) are credited and that the original publication in this journal is cited, in accordance with accepted academic practice. No use, distribution or reproduction is permitted which does not comply with these terms.
*Correspondence: James A. Levine SmFtZXMubGV2aW5lQGlwc2VuLmNvbQ==
Specialty Section: This article was submitted to Exercise Physiology, a section of the journal Frontiers in Sports and Active Living
Disclaimer: All claims expressed in this article are solely those of the authors and do not necessarily represent those of their affiliated organizations, or those of the publisher, the editors and the reviewers. Any product that may be evaluated in this article or claim that may be made by its manufacturer is not guaranteed or endorsed by the publisher.
Research integrity at Frontiers
Learn more about the work of our research integrity team to safeguard the quality of each article we publish.