- 1Laboratoire Univers et Théorie, Observatoire de Paris, Université PSL, Université Paris Cité, CNRS, Meudon, France
- 2Universidade Federal Do Rio Grande do Sul, Departamento de Astronomia, Porto Alegre, Brazil
- 3Departamento de Física, CFM, Universidade Federal de Santa Catarina, Florianópolis, Brazil
We discuss the role of planetary nebulae and their progeny in galaxy context in terms of ionization of the galaxy interstellar medium. This regards ionized gas outside the disk of spiral galaxies, the diffuse ionized medium in spiral galaxies, and the weak line emission of elliptical galaxies.
1 Introduction
Until recently it was considered that the only stellar ionization sources in galaxies are massive stars. However, low- and intermediate-mass stars (LIMS) are far more numerous (Salpeter, 1955; Kroupa, 2001) and many (if not or most) pass through a stage where they produce ionizing photons evidenced by the spectacular planetary nebula (PN) phenomenon which produces the beautiful images of celestial objects known to all sky lovers. The planetary nebula stage is actually very short on an astronomical timescale–just about a few thousand years (Jacob et al., 2013)—but LIMS by far outnumber the massive stars in galaxies. Besides, after the proper PN stage, when the nebulae have dispersed in the general interstellar medium, their central stars stay hot enough to produce ionizing photons that can travel in the diffuse gas of the galaxies (Marigo et al., 2004).
In the early nineties, Bruzual and Charlot (1993) assembled an evolutionary stellar population synthesis code which was, among many other things, able to quantify the number of ionizing photons emitted by an aging starburst as a function of time, including the photons produced by evolved LIMS. Then Binette et al. (1994) computed the emission-line spectrum that would be produced in the gas surrounding these ‘post-AGB’ stars and compared the results to the–very scarce at that time–data on the weak emission lines observed in elliptical galaxies. They also noted that the ionizing energy distribution of the population of these ‘post-AGB’ stars (better called HOLMES for ‘hot low-mass evolved stars’1) was much harder than that of massive stars. This implies that elliptical galaxies with weak emission lines should show emission-line ratios similar to those of LINERs and lie above the H ii region sequence in the [O iii]/Hβ versus [N ii]/Hα diagram, called the BPT diagram (Baldwin et al., 1981). This led Stasińska et al. (2008) to propose that, in the BPT diagram constructed for galaxies from the Sloan Digital Sky Survey (SDSS; York et al., 2000; Adelman-McCarthy et al., 2007), many of the galaxies situated in the right wing and so far considered to be composed of galaxies with a (weakly) active galactic nucleus (AGN) are actually ionized by the old stellar populations found in these galaxies. They nicknamed them ‘retired galaxies’ (as opposed to ‘active’ with reference to star formation), and suggested that nuclear activity related to a central black hole is not as common as thought before.
In the next section we summarize the paper by Stasińska (2012) which gave an overview of what was known on the topic at that time.
Many processes other than HOLMES have been invoked to explain the line emission properties of the diffuse ionized gas (DIG) observed in galaxies of all types such as shocks (Collins and Rand, 2001), turbulence (Slavin et al., 1993; Binette et al., 2009), magnetic reconnection (Lazarian et al., 2020), dust scattering (Barnes et al., 2015), ionizing photons escaping from H ii regions in the case of late-type galaxies. HOLMES, however, cannot be ignored as they are natural components in any evolved stellar population.
In Section 3 we briefly review the important advances made since 2012 in the observation of the DIG in galaxies. These are mainly due to the increasing use of integral field units in observational astronomy. New models have been computed for the evolution of LIMS that take into account the important progress achieved in stellar physics these last decades. In Section 4 we present these models and compute their effects on evolving stellar populations. We present and discuss a few pending issues in Section 5 and conclude in the last Section.
2 What We Knew Until 2012
2.1 Elliptical and LINER-Like Galaxies
In 2008, the number of galaxies with spectra in the 3900–9500 Å range amounted to nearly one million galaxies, thanks to the SDSS (York et al., 2000; Abazajian et al., 2009). Analysis of these spectra with inverse spectral synthesis tools (such as STARLIGHT by Cid Fernandes et al., 2005) allowed one to decompose the complex stellar populations of these galaxies into simple stellar populations (SSP) of various ages and metallicities. In such a way one could not only determine the galaxies star formation and chemical histories but also dig out weak emission lines from stellar emission. Such a procedure revealed that a large fraction of elliptical galaxies do present emission lines, at a low level. Applying STARLIGHT to the SDSS galaxies, Stasińska et al. (2008) found that galaxies with LINER-like spectra2, have stopped forming stars and contain old stellar populations whose HOLMES can easily produce the emission-line ratios observed in these galaxies. The observed Hα luminosities can be accounted by the HOLMES in about one third of the LINER-like galaxies present in the BPT diagram. In order to distinguish galaxies ionized by HOLMES from galaxies ionized by a weak AGN (LINERs), Cid Fernandes et al. (2011) proposed the WHAN diagram, where the Hα equivalent width, W(Hα), is plotted versus [N ii]/Hα. Using this diagram, five spectral classes can be identified in the entire population of galaxies:
• Pure star forming galaxies: log [N ii]/Hα
• Strong AGNs (i.e., Seyferts): log [N ii]/Hα
• Weak AGNs: log [N ii]/Hα
• Retired galaxies (i.e., fake AGNs): W(Hα)
• Passive galaxies (actually, line-less galaxies): W(Hα) and W ([N ii])
Integral field spectroscopy of LINER-like galaxies has confirmed that in many of them the ionization is indeed due to HOLMES and not to a weak AGN (Annibali et al., 2010; Sarzi et al., 2010).
2.2 The DIG in Spiral Galaxies
In spiral galaxies, the main ionizing source is due to the massive OB stars located in the thin disk and Hα images show many bright H ii regions linked to these stars. However, between these H ii regions one also detects diffuse ionized gas. By studying 109 H i selected galaxies in the SINGS survey, Oey et al. (2007) came to the conclusion that diffuse Hα emission is present in galaxies of all types representing about 60% of the total Hα emission, irrespective of the galaxy Hubble type or total star formation rate. DIG was also detected in edge-on spirals (Dettmar, 1990; Hoopes et al., 1996) even at several kiloparsecs from the galactic plane. While leakage of photons from massive stars out of the H ii regions may contribute to ionize the DIG, it has been shown that this cannot explain the increase of [N ii]/Hα, [S ii]/Hα [O ii]/Hβ and [O iii]/Hβ with galactic height, which require a hardening of the ionizing photons. HOLMES, on the other hand, have a radiation field roughly similar to that of a 105 K star and are thus an obvious candidate for the ionization and heating of the DIG, since they are expected to be plentiful in the old stellar populations of the thick discs and lower haloes of spiral galaxies. Using the edge-on spiral galaxy NGC 891 as a test case, Flores-Fajardo et al. (2011) showed that the estimated content of HOLMES in this galaxy well explains the observed emission-line characteristics of its extraplanar DIG.
3 Recent Observations of the DIG
3.1 DIG With IFS Observations
The recent explosion of studies using integral field spectroscopy (IFS) has provided a wealth of new observational data allowing one to study the DIG in galaxies of all types and orientations at different spatial resolutions, and to better quantify the effect of HOLMES. For example analyzing a sample of 32 early-type galaxies (ETG) from the CALIFA survey (Sánchez et al., 2016), Gomes et al. (2016) found that in half of them the radial distribution of diagnostic line ratios is of the LINER-type in both their nuclear and extranuclear zones and that the distribution of W(Hα) is compatible with the HOLMES hypothesis (the other half of the galaxies being devoid of gas in the central zones). Using data from the entire CALIFA survey, Lacerda et al. (2018) showed that W(Hα) is a better discriminant of the DIG than the commonly used surface-brighness in Hα which gives flawed indications in the bulges of late-type galaxies. They also showed that the DIG contribution to the total Hα luminosity varies in a systematic way along the Hubble sequence, increasing from late-to early-types.
Gomes et al. (2016) found that about half of their ETGs are dominated by rotation, and many of those show decoupling between gas and stellar kinematics. Other studies have looked at the kinematics of the extraplanar DIG in edge-on galaxies, e.g., Levy et al. (2019), who have investigated a sample of 25 edge-on galaxies observed by CALIFA and in CO with CARMA, and Rautio et al. (2022), whose sample consists of 5 edge-on galaxies observed with MUSE and deep narrowband Hα imaging. Both works showed that, for many of their galaxies, the Hα rotation velocity decreases above the midplane. They also measured the ionized gas velocity lag as a function of the galactocentric radius; Levy et al. (2019) found the extraplanar DIG to be linked to star formation activity in the disk, while Rautio et al. (2022) concluded that their results point to a complex external and/or internal origin for the ionized gas.
3.2 Other Options Than HOLMES
Among additional sources to ionize the DIG, Woods and Gilfanov (2014) suggested accreting, steadily nuclear-burning white dwarfs with effective temperatures of several 105 K that produce super-soft X-ray sources. To test this hypothesis, Johansson et al. (2014, 2016) stacked the spectra of 11 500 gas-rich retired galaxies from the SDSS in several age ranges, allowing them to measure the intensities of the weak He ii λ4686 and [O i] λ6300 lines. These lines provide additional diagnostics to the usual lines used in the BPT diagram, allowing one to estimate the relative populations of hot ionizing sources–accreting white dwarfs or HOLMES–that can produce the observed intensities in these weak lines. Johansson et al. (2014, 2016) concluded that in the presence of the expected population of HOLMES, accreting white dwarfs provide a negligible contribution to the ionization of the DIG.
3.3 Effect of DIG on Abundances From Emission Lines in Galaxies
The chemical composition of late-type galaxies is obtained from the lines emitted by their H ii regions. A review of the methods for abundance determinations in H ii regions can be found in Stasińska (2009). The method considered as the most accurate is the so-called ‘temperature-based’ or ‘direct’ method, in which the electron temperature is derived directly from a temperature-sensitive observed line ratio such as [O iii] λ4363/5,007, followed by the computation of ionic abundances from the observed emission line intensities. In most cases, however, it is not possible to apply this technique because the lines allowing the measurement of the temperature are not observed. One must use statistical methods, the so-called ‘strong line methods’ which rely on empirical relations between intensity ratios of strong lines and the oxygen abundances in H ii regions. These relations are based either on the observations of H ii regions where the weak auroral lines have been detected, or on photoionization models for H ii regions. Thus those calibrations do not take into account the contribution from the DIG to the emission-line spectra in star-forming galaxies. Since collisional-to-recombination line ratios such as [N ii] λ6584/Hα and [O ii] λ3727/Hβ are usually enhanced in the DIG, strong line methods may yield biased oxygen abundances.
Zhang et al. (2017), using a sample of 365 face-on star-forming galaxies from Mapping Nearby Galaxies at APO (MaNGA, Bundy et al., 2015), have characterized DIG regions as those having small values of Hα surface brightness. They concluded that strong emission line calibrations based on [N ii] λ6584/Hα, [O iii] λ5007/[N ii] λ6584, ([O ii] λ3727+[O iii] λ5007)/Hβ and a combination of [N ii] λ6584/[S ii] λ6716, λ6731 and [N ii] λ6584/Hα are unreliable, due to their broad distribution of O/H residuals as a function of Hα surface brightness. They considered that a calibration based on [N ii] λ6584/[O ii] λ3727 is relatively safe. They also compared emission line ratios with a set of photoionization models, and concluded that the radiation escape from OB stars alone is unable to model those line ratios and the addition of ionization by HOLMES is needed. The mixing of leaking radiation from H ii regions and HOLMES to model the DIG emission is further explored by Belfiore et al. (2022) at higher resolution for a sample of 19 nearby galaxies from the PHANGS–MUSE survey (Emsellem et al., 2022). They found that models with leakage only are able to account for most of the Hα emission, but a harder ionizing spectra from HOLMES is required to explain the behaviour of the line ratios such as [O iii] λ5007/Hβ, [N ii] λ6584/Hα, [S ii] λ6716, λ6731/Hα and [O i] λ6300/Hα.
Vale Asari et al. (2019) have proposed corrections for emission line luminosities due to the emission from the DIG based on a sample of 1,409 face-on star-forming galaxies from MaNGA. They have identified the DIG as contaminating spaxels of low WHα, following Lacerda et al. (2018). Those corrections were then applied to a sample of
4 New Models for HOLMES and Stellar Population Models Including Them
4.1 Stellar evolution
All the discussions regarding the effect of HOLMES on the ionization interstellar gas in galaxies rely on stellar evolution models of the 80–90s (Schoenberner, 1981; Vassiliadis and Wood, 1993; Bloecker, 1995), even for the most recent papers (with the exception of Byler et al., 2019). However, a significant update of evolutionary models for LIMS up to the white dwarf stage has been performed by Kitsikis and Weiss (2007), Weiss and Ferguson (2009), and, most importantly by Miller Bertolami (2016). While the Miller Bertolami’s models are now widely used for planetary nebulae studies, they have not been incorporated so far in stellar population studies. In the next section, we explain how we incorporated them and how the resulting stellar population models differ from the previous ones.
The models by Miller Bertolami include the improvements that have been carried out in stellar physics since the mid 90s: modern opacities, nuclear reaction rates, equations of state, conductive opacities, neutrino emission rates, and additional mixing processes. As a result, the post-AGB models are at least 3–10 times faster than the models of similar mass in the older grids, and 0.1–0.3 dex more luminous than the older ones at similar remnant masses. As noted by Miller Bertolami, the shorter timescales are in agreement with the results obtained for a restricted mass range by Kitsikis (2008) and Weiss and Ferguson (2009) using a completely independent code but also with updated physics.
The contribution of HOLMES to the ionizing flux is illustrated in Figures 1, 2, where we show the evolution of the spectra of HOLMES with solar metallicity and initial masses of 0.98 M⊙ (Figure 1) and 3.1 M⊙ (Figure 2). The spectra shown in these figures were taken from the stellar libraries by Hainich et al. (2019) and Rauch (2003), as indicated in the inner panels, and rescaled to have the total luminosity given by the Miller Bertolami (2016) models. The hardening of the ionizing photons during the hottest HOLMES phases can be clearly seen.
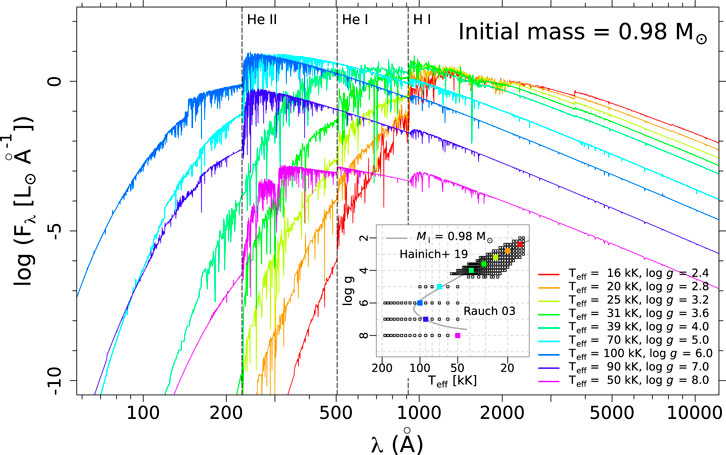
FIGURE 1. Evolution of the spectrum of a star with initial mass of 0.98 M⊙ and solar metalicity. The inner panel shows the HOLMES evolutionary sequence by Miller Bertolami (2016) (grey line) and the parameters (effective temperature and surface gravity) of stars in the Rauch (2003) and Hainich et al. (2019) libraries of stellar spectra (open squares). The coloured squares indicate the parameters of the stars for which spectra are shown in the main panel. The star takes 3.4 Myr to evolve from the first (red) to the last (purple) phase shown in this Figure.
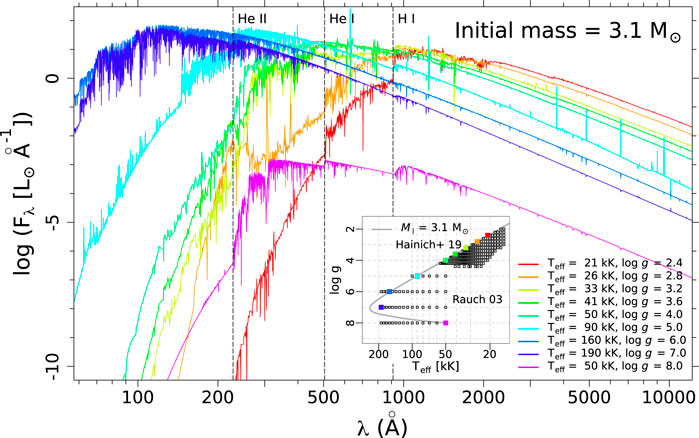
FIGURE 2. The same as Figure 1, but for a star with initial mass of 3.1 M⊙. The time elapsed between the first (red) and the last (purple) phase is 1.6 Myr.
Given the big changes in the predicted evolution of HOLMES, it is natural to ask how they affect the computed output of ionizing photons produced by aging stellar populations.
4.2 Simple Stellar Populations With the New Stellar Tracks
To incorporate the new evolutionary sequences by Miller Bertolami (2016) into stellar population models, we interpolated the tracks to generate the isochrones and created the model spectra of single-age, single metallicity HOLMES populations. To interpolate the evolutionary models, we calculated the curvature of the evolutionary sequences, applied the dynamic time warping technique–implemented in the R package dtw (Giorgino, 2009)–to find the optimal alignment between the tracks, and performed the interpolation. We used stellar spectra from the Hainich et al. (2019) and Rauch (2003) spectral libraries and adopted a Chabrier initial mass function (Chabrier, 2003) with lower and upper mass limits of 0.08 and 100 M⊙, respectively.
In the left panel of Figure 3, we show the H i ionizing photon rates, qH I, of individual stars along isochrones of HOLMES populations with different ages (0.2, 1.0, and 12 Gyr). HOLMES hotter than 25 ,000 K and brighter than 2000 L⊙ have ionizing photon fluxes qH I > 1047 s−1 and are present in populations of all ages. The hardness of the ionizing photons of individual stars, quantified by the ratio between the He i and H i ionizing photon rates (qHe I/qH I), is presented in the right panel of Figure 3. HOLMES with effective temperatures greater than ∼70 ,000 K have qHe I/qH I > 0.5 and are also present in populations of all ages. On the other hand, it is clear from these figures that more massive HOLMES in younger stellar populations can reach higher values of qH I and qHe I/qH I than low-mass HOLMES. The maximum H i ionizing photon rates of HOLMES in a 0.2-Gyr population is
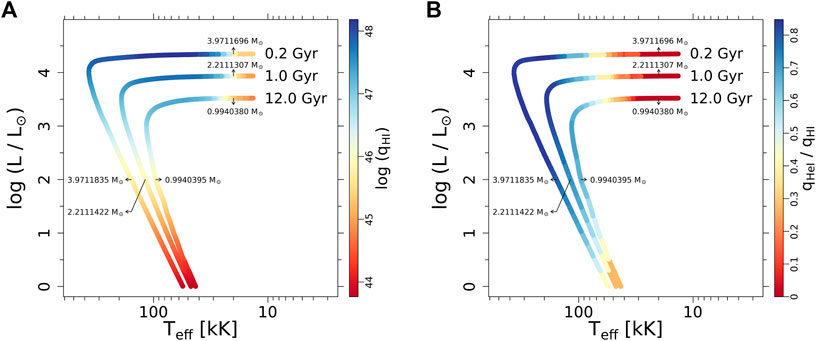
FIGURE 3. Isochrones in the luminosity vs. effective temperature plane for stellar populations with 0.2, 1.0 and 12 Gyr and solar metallicity. The points along the isochrones are colour-coded according to their logqH I (A) and qHe I/qH I ratio (B), where qH I and qHe I are the H i and He i ionizing photon rates in units of s−1. The masses of stars in two different points along each isochrone are indicated; the mass difference between these points is on the order of 10–5 and 10–6 M⊙ for the populations with 0.2 and 12 Gyr, respectively.
The figures discussed above give us an idea of how HOLMES with different initial masses contribute to the ionizing flux of stellar populations with different ages. However, we need to take into account that HOLMES contributing to the ionizing flux in younger stellar populations are less numerous and pass through the HOLMES phase faster than the less-massive HOLMES found in older stellar systems. As a result, the flux of H i ionizing photons remains approximately constant with time after 100 Myr, as shown in the top panel of Figure 4. In this figure, we also show the evolution of the He i and He ii ionizing photon rates relative to the flux of H i ionizing photons, and compare our predictions with those of other models from the literature. After 100 Myr, qHe I/qH I slightly decreases with time, going from qHe I/qH I = 0.62 to 0.50 between 0.2 and 12 Gyr. A similar decrease is also observed for the previous models. On the other hand, the flux He ii ionizing photon decreases by a factor of almost 9 from 0.2 to 12 Gyr, making qHe II/qH I decrease from approximately 0.05 to 0.007.
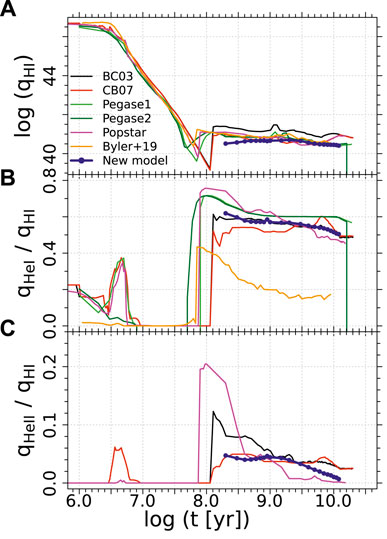
FIGURE 4. Evolution of the H i ionizing photon rates in units of
Finally, we compare our results with predictions from the recent model by Byler et al. (2019), who computed self-consistent stellar population models using evolutionary tracks from MIST (Choi et al., 2016; Dotter, 2016). In the MIST models, the HOLMES phase is faster and brighter than in the old generation of models, in agreement with the models calculated by Miller Bertolami (2016). The Byler et al. predictions for the evolution of H i ionizing photon rates and qHeI/qHI ratios are shown in Figure 4. While there their predictions for the qH I evolution is similar to that of other models, it can be seen that their models lead to lower He i ionizing photo rates when compared to other models, including ours. The source of this difference will be investigated in a future study.
5 Pending Problems
5.1 Origin of the Gas Ionized by HOLMES
Since all old stellar populations contain HOLMES in similar proportions, why is it that about half of the elliptical galaxies in the SDSS do not show emission lines at all (Stasińska et al., 2015)?
It should be noted that the mass of gas needed to produce the emission line luminosities in ‘liny’3 retired galaxies is smaller by orders of magnitudes than the total mass lost by winds in AGB and post-AGB stars in these galaxies: Following Herpich et al. (2018) the mass of gas needed to produce the Hα luminosity in liny retired galaxies is given by
where both L (Hα) and Mg are in solar units, and ne is the average electron density in cm−3. For a density of 100 cm−3 as found by Johansson et al. (2016) from stacked spectra of retired galaxies, this gives Mg ∼ 105 M⊙. This corresponds to the mass of gas injected into the ISM by stellar mass loss during only a few million years (Belfiore et al., 2017) in these retired galaxies.
But is it really the gas coming from stellar winds that produces the line emission in liny retired galaxies? Herpich et al. (2018) found that in the liny galaxies, the [N ii]/[O ii] sequence as a function of galaxy mass prolongates exactly the [N ii]/[O ii] sequence of star-forming galaxies, indicating that the emitting gas is not enriched in nitrogen in a way similar to what is seen in PNe and thus must have an external origin.
An additional argument for an external origin of the emitting gas in liny retired galaxies is that gas arising from stellar winds is expected to have the same kinematic signatures as that of the parent stars, which is at odds with the observations (Belfiore et al., 2017).
Herpich et al. (2018) compared in detail the properties of lineless and liny retired galaxies. Starting from a clean sample of SDSS ellipticals with good signal-to-noise spectra, they built a sample of 59,662 liny retired galaxies and 96,844 lineless retired galaxies (defined by W(Hα) smaller that 0.5 Å). To meaningfully compare the galaxy properties in the two samples, they pair-matched the two samples by total stellar mass, half-light radius in the r band and redshift (the latter to avoid aperture effects due to the fixed diameter of the SDSS fiber). No differences were seen in the total output of ionizing photons expected from HOLMES from a stellar population analysis. The difference in mean stellar ages is at the limit of significance. But there is a significant difference in the dust attenuation estimated from the stellar continuum, being higher for liny galaxies. Mid-infrared data from the WISE explorer (Wright et al., 2010) in the W2 and W3 bands indicate that the luminosity in the W3 band is greater in liny galaxies, pointing to the presence of polycyclic aromatic hydrocarbon (PAH) 11.2 and 12.7 micron features, which are greatly enhanced in the presence of the hard UV field able to excite the PAH grains (Draine and Li, 2007). Also data from the GALEX survey (Morrissey et al., 2007) show a slightly higher flux in the near-UV for liny galaxies. All this seems to indicate some low-level recent star formation. Since, as mentioned above, the gas producing the emission lines is not enriched in nitrogen, this new generation of stars must have been made of gas having an external origin. It probably comes from accretion from the haloes of the galaxies, or from residual streams of metal-rich gas coming from a merger in the recent past. Thus, the ionization source and the origin of the gas producing the emission lines are disconnected!
One still unanswered question is what happens to the gas ejected by the stellar winds. A priori one would expect it to be present in all the retired galaxies. Calculations show that gas from stellar-mass loss (Parriott and Bregman, 2008) and PNe (Bregman and Parriott, 2009) in early-type galaxies is quickly heated to very high temperatures and it is not clear whether the amount of remaining warm gas is sufficient to explain the observed Hα luminosities.
5.2 The Effect of Binaries
The works mentioned so far consider only single stars. However it is known that about 60% of LIMS evolve in binary systems (e.g., Kamath, 2019). Evolution in binary systems can be affected by mass transfer due Roche-lobe overflow which results in a change of the mass distribution and lifetimes of the stars (Han et al., 2010). It can even prevent certain stars from reaching states predicted in single-star evolution. For example some stars may never become red giants due to early removal of their hydrogen envelopes (Stanway and Eldridge, 2018; Xiao et al., 2018). On the other hand, the population of certain stellar types can be greatly increased, such as that of hot subdwarfs which are produced mostly in binary interactions and naturally explain the so-called UV upturn observed in elliptical galaxies (Han et al., 2007).
But what about HOLMES? Because binary evolution can result in mass transfer between stars or even in the production of merged stars (see Figure 1 in Han, 2003) the mass distribution of PN progenitors will be different from the one that would be found in a population of single stars.
Binary population synthesis codes have flourished these past 20 years (see references in Han et al., 2020), mostly to investigate exotic objects but also to investigate the global effects that stellar populations with binaries may have on the properties of galaxies (e.g., Stanway and Eldridge, 2020). However, to our knowledge, the problem of ionizing photons produced by HOLMES in binary scenarios has not been addressed so far.
Is it expected to be important? Estimates of the fraction of binaries among central stars of planetary nebulae could give some clue. Unfortunately such estimates are not accurate and vary among authors from about 20 to 70% with huge error bars (see Boffin and Jones, 2019). It is likely that, unless the population of PNe with binary central stars is dominant, their integrated effect on the Lyman continuum radiation produced by HOLMES in galaxies will not greatly differ from the predictions from single star population syntheses, but this point requires further investigation.
6 Conclusion
As this whole book demonstrates4, PNe, in addition to being interesting objects in their own right, play a more general role in Astronomy. For example, PNe and their progenies (and progenitors) have a collective effect on certain properties of galaxies. The role of LIMS on the chemical evolution of galaxies has been known for many decades. The effect of HOLMES in the production of emission lines in early-type galaxies has been demonstrated only recently and has been the subject of this short paper, where we presented the present-day knowledge on this topic. It led to the concept of ‘retired galaxies’, i.e., galaxies that stopped forming stars and are ionized by their old stellar populations. This concept has gained more and more supporters and has changed the astronomers’ view on the demography of AGNs in the local Universe (Stasińska et al., 2015), since many of the galaxies formerly thought to contain a weakly active nucleus are actually simply ionized by HOLMES.
Data Availability Statement
The data that support the findings of this study are available from the corresponding author upon reasonable request.
Author Contributions
GS, MT, and NVA contributed to conception and design of the study. MT performed the computations presented in Section 4. GS wrote the first draft of the manuscript. MT and NVA wrote sections of the manuscript. All authors contributed to manuscript revision, and have read and approved the submitted version.
Funding
GS acknowledges the support from the CAPES CSF–PVE project 88881.068116/2014-01. MT thanks the support of CNPq (process #307675/2018-1) and the program L’Oréal UNESCO ABC Para Mulheres na Ciência. NV acknowledges support of FAPESC, CNPq, and the Royal Society–Newton Advanced Fellowship award (NAF∖R1∖180403). The publication fees were supported by UNAM, PAPIIT IN105020.
Conflict of Interest
The authors declare that the research was conducted in the absence of any commercial or financial relationships that could be construed as a potential conflict of interest.
Publisher’s Note
All claims expressed in this article are solely those of the authors and do not necessarily represent those of their affiliated organizations, or those of the publisher, the editors and the reviewers. Any product that may be evaluated in this article, or claim that may be made by its manufacturer, is not guaranteed or endorsed by the publisher.
Footnotes
1Since 2010, we prefer to use the term HOLMES rather than ‘post-AGB’ to avoid confusion with ‘proto planetary nebulae’ which are also commonly referenced as ‘post-AGB’ in the PN community. In addition, the term HOLMES does not exclude stars which may provide ionizing photons without having reached the tip of the AGB.
2LINER was a term coined by Heckman (1980) to designate ‘low ionization nuclear emission regions’ in galaxies. In the BPT diagram, objects with LINER-like spectra lie above the H ii region sequence, and to the lower left of the Seyfert branch, see e.g., Kauffmann et al. (2003).
3The term ‘liny’ was introduced by Herpich et al. (2018) to distinguish retired galaxies showing emission lines from those where no emission lines could be detected after a careful subtraction of the stellar continuum using inverse spectral synthesis tools such as STARLIGHT.
4See also the proceedings of the conference ‘Planetary Nebulae as Astronomical Tools’(Szczerba et al., 2005).
References
Abazajian, K. N., Adelman-McCarthy, J. K., Agüeros, M. A., Allam, S. S., Allende Prieto, C., An, D., et al. (2009). The Seventh Data Release of the Sloan Digital Sky Survey. Astrophysical J. Suppl. Ser. 182, 543–558. doi:10.1088/0067-0049/182/2/543
Adelman-McCarthy, J. K., Agüeros, M. A., Allam, S. S., Anderson, K. S. J., Anderson, S. F., Annis, J., et al. (2007). The Fifth Data Release of the Sloan Digital Sky Survey. Astrophysical J. Suppl. Ser. 172, 634–644. doi:10.1086/518864
Annibali, F., Bressan, A., Rampazzo, R., Zeilinger, W. W., Vega, O., and Panuzzo, P. (2010). Nearby Early-type Galaxies with Ionized Gas. Astronomy Astrophysics 519, A40. doi:10.1051/0004-6361/200913774
Baldwin, J. A., Phillips, M. M., and Terlevich, R. (1981). Classification Parameters for the Emission-Line Spectra of Extragalactic Objects. Astronomical Soc. Pac. Publ. 93, 5–19. doi:10.1086/130766
Barnes, J. E., Wood, K., Hill, A. S., and Haffner, L. M. (2015). Models of Diffuse Hα in the Interstellar Medium: the Relative Contributions from In Situ Ionization and Dust Scattering. Mon. Notices R. Astronomical Soc. 447, 559–566. doi:10.1093/mnras/stu2454
Belfiore, F., Maiolino, R., Maraston, C., Emsellem, E., Bershady, M. A., Masters, K. L., et al. (2017). SDSS-IV MaNGA - the Spatially Resolved Transition from Star Formation to Quiescence. Mon. Not. R. Astron. Soc. 466, 2570–2589. doi:10.1093/mnras/stw3211
Belfiore, F., Santoro, F., Groves, B., Schinnerer, E., Kreckel, K., Glover, S. C. O., et al. (2022). A Tale of Two DIGs: The Relative Role of H II Regions and Low-Mass Hot Evolved Stars in Powering the Diffuse Ionised Gas (DIG) in PHANGS-MUSE Galaxies. Astronomy Astrophysics 659, A26. doi:10.1051/0004-6361/202141859
Binette, L., Drissen, L., Úbeda, L., Raga, A. C., Robert, C., and Krongold, Y. (2009). The Broad Hα, [O III] Line Wings in Stellar Supercluster A of NGC 2363 and the Turbulent Mixing Layer Hypothesis. Astronomy Astrophysics 500, 817–826. doi:10.1051/0004-6361/200811132
Binette, L., Magris, C. G., Stasińska, G., and Bruzual, A. G. (1994). Photoionization in Elliptical Galaxies by Old Stars. Astronomy Astrophysics 292, 13–19.
Bloecker, T. (1995). Stellar Evolution of Low- and Intermediate-Mass Stars. II. Post-AGB Evolution. Astronomy Astrophysics 299, 755.
Boffin, H. M. J., and Jones, D. (2019). “The Importance of Binaries in the Formation and Evolution of Planetary Nebulae,” in Springer Briefs in Astronomy. doi:10.1007/978-3-030-25059-1
Bregman, J. N., and Parriott, J. R. (2009). Mass Loss from Planetary Nebulae in Elliptical Galaxies. Astrophysical J. 699, 923–932. doi:10.1088/0004-637X/699/2/923
Bruzual, G., and Charlot, S. (1993). Spectral Evolution of Stellar Populations Using Isochrone Synthesis. ApJ 405, 538–553. doi:10.1086/172385
Bundy, K., Bershady, M. A., Law, D. R., Yan, R., Drory, N., MacDonald, N., et al. (2015). Overview of the SDSS-IV MaNGA Survey: Mapping Nearby Galaxies at Apache Point Observatory. Astrophysical J. 798, 7. doi:10.1088/0004-637X/798/1/7
Byler, N., Dalcanton, J. J., Conroy, C., Johnson, B. D., Choi, J., Dotter, A., et al. (2019). Self-consistent Predictions for LIER-like Emission Lines from Post-AGB Stars. Astronomical J. 158, 2. doi:10.3847/1538-3881/ab1b70
Chabrier, G. (2003). Galactic Stellar and Substellar Initial Mass Function. Publ. Astron Soc. Pac 115, 763–795. doi:10.1086/376392
Choi, J., Dotter, A., Conroy, C., Cantiello, M., Paxton, B., and Johnson, B. D. (2016). Mesa Isochrones and Stellar Tracks (MIST). I. Solar-Scaled Models. Astrophysical J. 823, 102. doi:10.3847/0004-637x/823/2/102
Cid Fernandes, R., Stasińska, G., Mateus, A., and Vale Asari, N. (2011). A Comprehensive Classification of Galaxies in the Sloan Digital Sky Survey: How to Tell True from Fake AGN? Mon. Notices R. Astronomical Soc. 413, 1687–1699. doi:10.1111/j.1365-2966.2011.18244.x
Collins, J. A., and Rand, R. J. (2001). Ionization Sources and Physical Conditions in the Diffuse Ionized Gas Halos of Four Edge‐On Galaxies. Astrophysical J. 551, 57–71. doi:10.1086/320072
Dettmar, R. J. (1990). The Distribution of the Diffuse Ionized Interstellar Medium Perpendicular to the Disk of the Edge-On Galaxy NGC891. Astronomy Astrophysics 232, L15.
Dotter, A. (2016). MESA Isochrones and Stellar Tracks (MIST) 0: Methods for the Construction of Stellar Isochrones. Astrophysical J. Suppl. Ser. 222, 8. doi:10.3847/0067-0049/222/1/8
Draine, B. T., and Li, A. (2007). Infrared Emission from Interstellar Dust. IV. The Silicate‐Graphite‐PAH Model in the Post‐SpitzerEra. Astrophysical J. 657, 810–837. doi:10.1086/511055
Emsellem, E., Schinnerer, E., Santoro, F., Belfiore, F., Pessa, I., McElroy, R., et al. (2022). The PHANGS-MUSE survey. Astronomy Astrophysics 659, A191. doi:10.1051/0004-6361/202141727
Fernandes, R. C., Mateus, A., Sodré, L., Stasińska, G., and Gomes, J. M. (2005). Semi-empirical analysis of Sloan Digital Sky Survey galaxies - I. Spectral synthesis method. Mon. Notices R. Astronomical Soc. 358, 363–378. doi:10.1111/j.1365-2966.2005.08752.x
Fioc, M., and Rocca-Volmerange, B. (1997). PEGASE: a UV to NIR spectral evolution model of galaxies. Application to the calibration of bright galaxy counts. Astronomy Astrophysics 326, 950–962.
Flores-Fajardo, N., Morisset, C., Stasińska, G., and Binette, L. (2011). Ionization of the diffuse gas in galaxies: hot low-mass evolved stars at work. Mon. Notices R. Astronomical Soc. 415, 2182–2192. doi:10.1111/j.1365-2966.2011.18848.x
Giorgino, T. (2009). Computing and Visualizing Dynamic Time Warping Alignments inR: ThedtwPackage. J. Stat. Soft. 31, 1–24. doi:10.18637/jss.v031.i07
Gomes, J. M., Papaderos, P., Kehrig, C., Vílchez, J. M., Lehnert, M. D., Sánchez, S. F., et al. (2016). Warm ionized gas in CALIFA early-type galaxies. Astronomy Astrophysics 588, A68. doi:10.1051/0004-6361/201525976
Hainich, R., Ramachandran, V., Shenar, T., Sander, A. A. C., Todt, H., Gruner, D., et al. (2019). PoWR grids of non-LTE model atmospheres for OB-type stars of various metallicities. Astronomy Astrophysics 621, A85. doi:10.1051/0004-6361/201833787
Han, Z.-W., Ge, H.-W., Chen, X.-F., and Chen, H.-L. (2020). Binary Population Synthesis. Res. Astron. Astrophys. 20, 161. doi:10.1088/1674-4527/20/10/161
Han, Z. (2003). “Binary Evolution – Problems and Applications,” in The Proceedings of the IAU 8th Asian-Pacific Regional Meeting, Volume 1. Vol. 289 of Astronomical Society of the Pacific Conference Series. Editors S. Ikeuchi, J. Hearnshaw, and T. Hanawa, 413–420.
Han, Z., Chen, X., Zhang, F., and Podsiadlowski, P. (2010). Evolution of binary stars and its implications for evolutionary population synthesis. Proc. IAU 5, 44–47. doi:10.1017/S1743921310002504
Han, Z., Podsiadlowski, P., and Lynas-Gray, A. E. (2007). A binary model for the UV-upturn of elliptical galaxies. Mon. Notices R. Astronomical Soc. 380, 1098–1118. doi:10.1111/j.1365-2966.2007.12151.x
Heckman, T. M. (1980). An optical and radio survey of the nuclei of bright galaxies - Stellar populations and normal H II regions. Astronomy Astrophysics 87, 142–151.
Herpich, F., Stasińska, G., Mateus, A., Vale Asari, N., and Cid Fernandes, R. (2018). Why Do many early-type galaxies lack emission lines? I. Fossil clues. Mon. Notices R. Astronomical Soc. 481, 1774–1785. doi:10.1093/mnras/sty2391
Hoopes, C. G., Walterbos, R. A. M., and Greenwalt, B. E. (1996). Diffuse Ionized Gas in Three Sculptor Group Galaxies. Astronomical J. 112, 1429. doi:10.1086/118111
Jacob, R., Schönberner, D., and Steffen, M. (2013). The evolution of planetary nebulae. Astronomy Astrophysics 558, A78. doi:10.1051/0004-6361/201321532
Johansson, J., Woods, T. E., Gilfanov, M., Sarzi, M., Chen, Y.-M., and Oh, K. (2014). Diffuse gas in galaxies sheds new light on the origin of Type Ia supernovae. Mon. Notices R. Astronomical Soc. 442, 1079–1089. doi:10.1093/mnras/stu907
Johansson, J., Woods, T. E., Gilfanov, M., Sarzi, M., Chen, Y.-M., and Oh, K. (2016). Diffuse gas in retired galaxies: nebular emission templates and constraints on the sources of ionization. Mon. Not. R. Astron. Soc. 461, 4505–4516. doi:10.1093/mnras/stw1668
Kamath, D. (2019). Post-RGB and Post-AGB stars as tracers of binary evolution. Proc. IAU 14, 209–219. doi:10.1017/s1743921318006154
Kauffmann, G., Heckman, T. M., Tremonti, C., Brinchmann, J., Charlot, S., White, S. D. M., et al. (2003). The host galaxies of active galactic nuclei. Mon. Notices R. Astronomical Soc. 346, 1055–1077. doi:10.1111/j.1365-2966.2003.07154.x
Kitsikis, A. (2008). Theoretical AGB and Post-AGB Models for Synthetic Population Studies. Ph.D. thesis (Munich, Germany: Ludwig-Maximilians-Universität München).
Kitsikis, A., and Weiss, A. (2007). “AGB and Post-AGB Stellar Evolution: Theoretical Models for Synthetic Population Studies,” in Why Galaxies Care about AGB Stars: Their Importance as Actors and Probes. Vol. 378 of Astronomical Society of the Pacific Conference Series. Editors F. Kerschbaum, C. Charbonnel, and R. F. Wing, 99.
Kroupa, P. (2001). On the variation of the initial mass function. Mon. Notices R. Astronomical Soc. 322, 231–246. doi:10.1046/j.1365-8711.2001.04022.x
Lacerda, E. A. D., Cid Fernandes, R., Couto, G. S., Stasińska, G., García-Benito, R., Vale Asari, N., et al. (2018). Diffuse ionized gas in galaxies across the Hubble sequence at the CALIFA resolution. Mon. Notices R. Astronomical Soc. 474, 3727–3739. doi:10.1093/mnras/stx3022
Lazarian, A., Eyink, G. L., Jafari, A., Kowal, G., Li, H., Xu, S., et al. (2020). 3D turbulent reconnection: Theory, tests, and astrophysical implications. Phys. Plasmas 27, 012305. doi:10.1063/1.5110603
Levy, R. C., Bolatto, A. D., Sánchez, S. F., Blitz, L., Colombo, D., Kalinova, V., et al. (2019). The EDGE-CALIFA Survey: Evidence for Pervasive Extraplanar Diffuse Ionized Gas in Nearby Edge-on Galaxies. Astrophysical J. 882, 84. doi:10.3847/1538-4357/ab2ed4
Lilly, S. J., Carollo, C. M., Pipino, A., Renzini, A., and Peng, Y. (2013). Gas Regulation of Galaxies: The Evolution of the Cosmic Specific Star Formation Rate, the Metallicity-Mass-Star-formation Rate Relation, and the Stellar Content of Halos. Astrophysical J. 772, 119. doi:10.1088/0004-637x/772/2/119
Marigo, P., Girardi, L., Weiss, A., Groenewegen, M. A. T., and Chiosi, C. (2004). Evolution of planetary nebulae. Astronomy Astrophysics 423, 995–1015. doi:10.1051/0004-6361:20040234
Miller Bertolami, M. M. (2016). New models for the evolution of post-asymptotic giant branch stars and central stars of planetary nebulae. Astronomy Astrophysics 588, A25. doi:10.1051/0004-6361/201526577
Mollá, M., García-Vargas, M. L., and Bressan, A. (2009). PopStar I: evolutionary synthesis model description. Mon. Notices R. Astronomical Soc. 398, 451–470. doi:10.1111/j.1365-2966.2009.15160.x
Morrissey, P., Conrow, T., Barlow, T. A., Small, T., Seibert, M., Wyder, T. K., et al. (2007). The Calibration and Data Products of GALEX. Astrophys. J. Suppl. S 173, 682–697. doi:10.1086/520512
Oey, M. S., Meurer, G. R., Yelda, S., Furst, E. J., Caballero‐Nieves, S. M., Hanish, D. J., et al. (2007). The Survey for Ionization in Neutral Gas Galaxies. III. Diffuse, Warm Ionized Medium and Escape of Ionizing Radiation. Astrophysical J. 661, 801–814. doi:10.1086/517867
Parriott, J. R., and Bregman, J. N. (2008). Mass Loss from Evolved Stars in Elliptical Galaxies. Astrophysical J. 681, 1215–1232. doi:10.1086/588033
Rauch, T. (2003). A grid of synthetic ionizing spectra for very hot compact stars from NLTE model atmospheres. Astronomy Astrophysics 403, 709–714. doi:10.1051/0004-6361:20030412
Rautio, R. P. V., Watkins, A. E., Comerón, S., Salo, H., Díaz-García, S., and Janz, J. (2022). The multifarious ionization sources and disturbed kinematics of extraplanar gas in five low-mass galaxies. Astronomy Astrophysics 659, A153. doi:10.1051/0004-6361/202142440
Salpeter, E. E. (1955). The Luminosity Function and Stellar Evolution. Astrophysical J. 121, 161. doi:10.1086/145971
Sánchez, S. F., García-Benito, R., Zibetti, S., Walcher, C. J., Husemann, B., Mendoza, M. A., et al. (2016). CALIFA, the Calar Alto Legacy Integral Field Area survey. Astronomy Astrophysics 594, A36. doi:10.1051/0004-6361/201628661
Sarzi, M., Shields, J. C., Schawinski, K., Jeong, H., Shapiro, K., Bacon, R., et al. (2010). The SAURON project - XVI. On the sources of ionization for the gas in elliptical and lenticular galaxies. Mon. Notices R. Astronomical Soc. 402, 2187–2210. doi:10.1111/j.1365-2966.2009.16039.x
Schoenberner, D. (1981). Late stages of stellar evolution : central stars of planetary nebulae. Astronomy Astrophysics 103, 119–130.
Slavin, J. D., Shull, J. M., and Begelman, M. C. (1993). Turbulent Mixing Layers in the Interstellar Medium of Galaxies. Astrophysical J. 407, 83. doi:10.1086/172494
Stanway, E. R., and Eldridge, J. J. (2020). Interpreting galaxy properties with improved modelling. Proc. IAU 15, 84–97. doi:10.1017/S1743921319009050
Stanway, E. R., and Eldridge, J. J. (2018). Re-evaluating old stellar populations. Mon. Notices R. Astronomical Soc. 479, 75–93. doi:10.1093/mnras/sty1353
Stasińska, G., Costa-Duarte, M. V., Vale Asari, N., Cid Fernandes, R., and Sodré, L. (2015). Retired galaxies: Not to be forgotten in the quest of the star formation - AGN connection. Mon. Notices R. Astronomical Soc. 449, 559–573. doi:10.1093/mnras/stv078
Stasińska, G. (2012). The ionization of galaxies by their planetary nebulae. IAU Symp. 283, 239–242. doi:10.1017/S1743921312011027
Stasińska, G., Vale Asari, N., Cid Fernandes, R., Gomes, J. M., Schlickmann, M., Mateus, A., et al. (2008). Can retired galaxies mimic active galaxies? Clues from the Sloan Digital Sky Survey. Mon. Notices R. Astronomical Soc. 391, L29–L33. doi:10.1111/j.1745-3933.2008.00550.x
Stasińska, G. (2009). “What can emission lines tell us?,” in The Emission-Line Universe: XVIII Canary Islands Winter School of Astrophysics. Editor J. Cepa (Cambridge: Cambridge University Press), 1.
R. Szczerba, G. Stasińska, and S. K. Gorny (Editors) (2005). International Conference on Planetary Nebulae as Astronomical Tools. Vol. 804 of American Institute of Physics Conference Series.
Vale Asari, N., Couto, G. S., Cid Fernandes, R., Stasińska, G., de Amorim, A. L., Ruschel-Dutra, D., et al. (2019). Diffuse ionized gas and its effects on nebular metallicity estimates of star-forming galaxies. Mon. Notices R. Astronomical Soc. 489, 4721–4733. doi:10.1093/mnras/stz2470
Vassiliadis, E., and Wood, P. R. (1993). Evolution of Low- and Intermediate-Mass Stars to the End of the Asymptotic Giant Branch with Mass Loss. Astrophysical J. 413, 641. doi:10.1086/173033
Weiss, A., and Ferguson, J. W. (2009). New asymptotic giant branch models for a range of metallicities. Astronomy Astrophysics 508, 1343–1358. doi:10.1051/0004-6361/200912043
Woods, T. E., and Gilfanov, M. (2014). Emission-line diagnostics to constrain high-temperature populations in early-type galaxies. Mon. Notices R. Astronomical Soc. 439, 2351–2363. doi:10.1093/mnras/stu072
Wright, E. L., Eisenhardt, P. R. M., Mainzer, A. K., Ressler, M. E., Cutri, R. M., Jarrett, T., et al. (2010). The Wide-field Infrared Survey Explorer (WISE): Mission Description and Initial On-orbit Performance. Astronomical J. 140, 1868–1881. doi:10.1088/0004-6256/140/6/1868
Xiao, L., Stanway, E. R., and Eldridge, J. J. (2018). Emission-line diagnostics of nearby H II regions including interacting binary populations. Mon. Notices R. Astronomical Soc. 477, 904–934. doi:10.1093/mnras/sty646
York, D. G., Adelman, J., Anderson, J., John, E., Anderson, S. F., Annis, J., et al. (2000). The Sloan Digital Sky Survey: Technical Summary. Astronomical J. 120, 1579–1587. doi:10.1086/301513
Zhang, K., Yan, R., Bundy, K., Bershady, M., Haffner, L. M., Walterbos, R., et al. (2017). SDSS-IV MaNGA: the impact of diffuse ionized gas on emission-line ratios, interpretation of diagnostic diagrams and gas metallicity measurements. Mon. Not. R. Astron. Soc. 466, 3217–3243. doi:10.1093/mnras/stw3308
Keywords: planetary nebulae, galaxies, ionization, interstellar medium, stellar population
Citation: Stasińska G, Trevisan M and Vale Asari N (2022) Planetary Nebulae and the Ionization of the Interstellar Medium in Galaxies. Front. Astron. Space Sci. 9:913485. doi: 10.3389/fspas.2022.913485
Received: 05 April 2022; Accepted: 20 June 2022;
Published: 18 July 2022.
Edited by:
Marcelo Miguel Miller Bertolami, CONICET Instituto de Astrofísica de La Plata, ArgentinaReviewed by:
Gustavo Bruzual, National Autonomous University of Mexico, MexicoPaula Coelho, University of São Paulo, Brazil
Copyright © 2022 Stasińska, Trevisan and Vale Asari. This is an open-access article distributed under the terms of the Creative Commons Attribution License (CC BY). The use, distribution or reproduction in other forums is permitted, provided the original author(s) and the copyright owner(s) are credited and that the original publication in this journal is cited, in accordance with accepted academic practice. No use, distribution or reproduction is permitted which does not comply with these terms.
*Correspondence: Grażyna Stasińska, Z3JhenluYS5zdGFzaW5za2FAb2JzcG0uZnI=