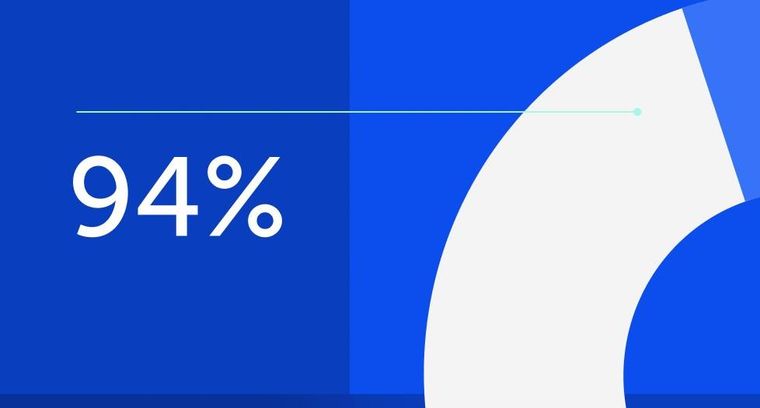
94% of researchers rate our articles as excellent or good
Learn more about the work of our research integrity team to safeguard the quality of each article we publish.
Find out more
ORIGINAL RESEARCH article
Front. Astron. Space Sci., 09 February 2022
Sec. Astrobiology
Volume 9 - 2022 | https://doi.org/10.3389/fspas.2022.794932
This article is part of the Research TopicMars analogs: Environment, Habitability and BiodiversityView all 11 articles
The biological system uses L-amino acids (L-AAs) to construct peptides. The selection of AA chirality in the prebiotic Earth still remains a mystery. Here we report the intrinsic mechanism of AA chiral selection in the formation of prebiotic proline-containing cyclic dipeptide (DKPs). The DKPs were synthesized by equal amounts of L-AA and D-AA with L-Pro that exhibited a clear preference for D-AA as opposed to L-AA. And surprisingly, the L-AA was preferred over D-AA in the mirror-image system with D-Pro. These results indicate that prebiotic DKPs synthesis has a certain selectivity for AA chirality preceded the biological enzymatic catalysis.
Life on Earth has a unique characteristic of chiral selection, e.g., L-amino acids (L-AAs) in proteins and D-nucleosides in nucleic acids (Bondy and Harrington, 1979; Bonner, 2000; Tamura, 2008). It is widely known that the chirality of biomolecules is catalyzed by bio-enzymes. The process of enzymatic catalyzed chirality is extremely precise and complex and cannot be realized at the beginning of life. However, the question of prebiotic chiral selection in biomolecule remains an abstruse problem. For the chemical origin of life, the simpler forms must have driven the prebiotic chiral selection process. Something as simple as intrinsic reactivity may have driven the prebiotic evolution process (Joshi et al., 2000; Rikken and Raupach, 2000; Hazen and Sholl, 2003; Ying et al., 2018a; Guasti, 2019; Yachmenev et al., 2019; Ye et al., 2019).
Cyclic dipeptides (DKPs), as one kind of the simplest peptide derivatives, have been found to have chiral catalysis properties (Prasad, 1995; Poullennec et al., 2002; Borthwick, 2012; Manchineella et al., 2013). DKPs might have chiral catalytic characteristics previous to the appearance of the bio-enzymes within the prebiotic chemical evolution. Consequently, it is reasonable to consider that DKPs ought to have played a crucial function in this process (Huber et al., 2003; Ying et al., 2018b). The synthesis of DKPs has been implemented under different conditions (Botti et al., 1996; Trauger et al., 2000; Lambert et al., 2001; Jainta et al., 2008; Nonappa et al., 2011; Borthwick, 2012), but there are limited reports involving plausible prebiotic chemical inventory, i.e., aqueous environment (Parker et al., 2014; Ying et al., 2018b; Guo et al., 2021). P3m may have existed in the prebiotic environment. Yamagata found volcanic production of polyphosphates including P3m (Yamagata et al., 1991). Rabinowitz demonstrated that some amino acids, such as Gly and Ala, could be condensed to dipeptides in aqueous solutions with maximum yield by treatment of P3m which may play an important role in the prebiotic synthesis of peptides (Rabinowitz et al., 1969).
We recently reported the formation of DKPs from free AAs with the presence of trimetaphosphate (P3m) in aqueous solution (Ying et al., 2018b). Meanwhile, we found proline-containing DKPs (cyclo-Pro-AAs) can spontaneously be acquired from linear dipeptides under aqueous environment. Notably, the yields of DKPs had been suffering from the sequence of linear dipeptides and whether the reaction contains P3m (Guo et al., 2021). These findings are very useful to understand the formation of DKPs and provide some guidelines for properly know-how the function of DKPs in the process of prebiotic chemistry.
In our assay, cyclo-Pro-AAs were detected in L-Pro reaction with L-AAs (Ying et al., 2018b). How the effect of Pro and AAs chirality on the formation of cyclo-Pro-AAs in plausible prebiotic situations has not been mentioned. In other words, it was unclear whether the chirality of Pro and another AA (components of cyclo-Pro-AA) have effect on the corresponding DKPs synthesis. In this paper, we experimentally explored the amino acid chiral selection in the process of prebiotic DKPs synthesis.
A sealed tube was charged with L-Pro or D-Pro (.05 mmol, .5 equiv.), L-AA or D-AA (.1 mmol, 1 equiv.) and P3m (.1 mmol, 1 equiv.) in water (1 ml) under regular air compositions. The pH of reaction mixtures were adjusted to 11.7 with 10 M NaOH. Then, the samples were carried out in an incubator at 35°C for 6 days. After cooling at room temperature, the mixture were filtered with the .22 μm filter for HPLC-MS analysis.
L-proline (L-Pro), L-alanine (L-Ala), L- arginine (L-Arg), L-leucine (L-Leu), L- Methionine (L-Met), D-proline (D-Pro), D-alanine (D-Ala), D- arginine (D-Arg), D-leucine (D-Leu) and D- methionine (D-Met) were purchased from MACKLIN (China). Trimetaphosphate (P3m) was purchased from Sigma Aldrich (United States). NaOH was obtained from Energy Chemical (China). Analytical reagents methanol (High Performance Liquid Chromatography (HPLC) grade) was purchased from SpectrumChemical. HPLC grade formic acid was purchased from Sigma Aldrich (United States). Ultrapure water (Millipore, 18.2 MΩ) was used to prepare the aqueous solutions and the mobile phase.
For Cyclo-L-Pro-AAs, .1 mmol L-/D-AA was mixed with .05 mmol L-Pro in 1 ml trimetaphosphate (P3m, .1 mmol) aqueous solutions, respectively. The pH of the mixtures was adjusted to 11.7 with 10 M NaOH. Then, the samples were carried out at 35°C for 6 days.
For Cyclo-D-Pro-AAs, .1 mmol L-/D-AA was mixed with .05 mmol D-Pro in 1 ml P3m aqueous solutions, respectively. The pH of mixtures was adjusted to 11.7 with 10 M NaOH. Then, the samples were carried out at 35°C for 6 days.
MS was performed using Bruker micrOTOF-Q Ⅱmass spectrometer in positive-ion mode. The MS main spectrometer parameters were as follows: capillary voltage: 4,500 V; nebulizer pressure: 2 bar; dry gas: 8 L min−1; dry temperature: 200°C. Mass spectra were recorded in the mass range from m/z 50 to m/z 1,000. The 1/10 eluate sample was introduced into electrospray ionization-MS (ESI-MS) through a splitting three-way pipe. In order to detect the reaction product online, we set up the divert valve of the MS spectrometer parameters as follows: 1) when the divert valve was set to waste position in the first 3 min, so that the HPLC flows directly to waste tank; 2) and then, when the divert valve was switched to source position, the valve can be used for HPLC flows immediately to MS ion source.
The HPLC was performed on Agilent HPLC systems series 1,260 Infinity. The mobile phase A was .1% formic acid and mobile phase B was methanol. The gradient elution program was used: 5%–30% B: 0–15 min; 30%–70% B: 15–20 min; 70% B: 20–22 min; 70%–5% B: 22–24 min; 5% B: 24–29 min. The column was kept at ambient temperature. The HPLC flow rate was .8 ml min−1 with TC- C18 column (4.6 mm × 150 mm, 5 μm, Agilent).
CD were performed on a 1700 JASCO circular dichroism spectrometer. The samples for the CD measurements were prepared in 1 mm cuvette. The CD instrument parameters were as follows: scanning speed: 50 nm min−1; bandwidth: 1 nm; sheath gas flow rate: 3 L min−1; data pitch: .1 nm. CD were registered in the scan range from 190 to 260 nm.
Initially, we investigated whether the chirality of amino acid (AA, one of components of cyclo-Pro-AA) would affect the formation of cyclo-Pro-AAs. The synthesis of chiral cyclo-Pro-Leu using equal amounts of D-Leu and L-Leu with L-Pro and trimetaphosphate (P3m) was carried out at an initial pH 11.7 at 35°C for 6 days, respectively. The pH was not adjusted during the whole process. The reaction was quenched with 4 M HCl solution. All the experimental data reported here were obtained after repeating the experiments three times. The resulting reactions were monitored by HPLC-high resolution mass spectrometry (HPLC-HRMS).
The reaction of D-Leu with L-Pro showed an extracted ion chromatogram (EIC) of m/z 211.1441 with retention times, respectively, of 19.8 and 20.2 min, which correspond to cyclo-Pro-Leu molecular ions [M + H]+ (Figures 1A,B). This result indicated that the cyclo-Pro-Leu has two isomers. There are two reasons for this phenomenon. One is the racemization of D-Leu or L-Pro during the reaction. Another possibility is that enantioselection occurred during the cyclo-L-Pro-D-Leu formation. To explain this confusion, we then investigated whether the chiral amino acids used in the experiment were racemized under the above reaction conditions (that is an initial pH 11.7 at 35°C for 6 days). The results showed that these chiral amino acids did not undergo chiral racemization (Figure 2). So, the two isomers may be the result of the condensations reaction itself, in which two different stereoisomer products were formed from the reaction of L-Pro and D-Leu. Therefore, the yield of cyclo-L-Pro-D-Leu is the sum of these two peaks of 19.8 min and 20.2 min (Figures 1A,B). Similarly, in Figure 1C, the yield of cyclo-L-Pro-L-Leu is the sum of peak 19.8 min and peak 20.2 min (Figures 1A,C).
FIGURE 1. Formation of cyclo-L-Pro-Leu from a reaction mixture of L-Pro, L/D-Leu and P3m in an alkaline aqueous solution (pH = 11.7). The calculated value of cyclo-Pro-Leu [M + H]+ is 211.1441. (A) Reaction scheme. (B) EIC-MS spectrum of the cyclo-L-Pro-L-Leu from the L-Pro reaction with L-Leu. (C) EIC-MS profile of the cyclo-L-Pro-D-Leu from the L-Pro reaction with D-Leu.
FIGURE 2. CD spectra of four chiral Leu. Blue line: the CD spectrum is derived from freshly prepared L-Leu aqueous solutions. Purple line: the CD spectrum of L-Leu in an initial pH 11.7 at 35°C for 6 days Black line: the CD spectrum is derived from freshly prepared D-Leu aqueous solutions. Red line: the CD spectrum of D-Leu in an initial pH 11.7 at 35°C for 6 d. The results showed that L-Leu or D-Leu did not undergo chiral racemization in the experiment reaction conditions.
These results indicated that both L-Leu and D-Leu with L-Pro can form corresponding chiral cyclo-Pro-Leu under alkaline aqueous solution, respectively. And remarkably, it showed that the formation of cyclo-L-Pro-D-Leu was preferred over that of cyclo-L-Pro-L-Leu by a ratio of about 6.61: 1, i.e., D-Leu was preferred over L-Leu in the presence of L-Pro (Figures 3, 4). Moreover, when the chirality of Pro was changed into the D-configuration, the results showed that the yield of cyclo-D-Pro-L-Leu from L-Leu was higher than that cyclo-D-Pro-D-Leu from D-Leu, which was improved to 6.42-fold (Figure 4; Supplementary Figures S2–S3). As in our previous study, cyclo-Pro-Pro was the primary product of Pro reaction with other amino acids in the P3m alkaline solution, while cyclo-Pro-Leu (heterocyclic dipeptides) were relatively rare (Ying et al., 2018b). The formation of cyclo-Pro-Pro did not involve chiral selection, so the cyclo-Pro-Pro was not considered in this study.
FIGURE 3. HPLC spectra analysis of cyclo-Pro-Leu products. (A): HPLC profile for the reaction products cyclo-L-Pro-L-Leu of L-Leu with L-Pro and P3m in aqueous solution. (B): HPLC spectrum of products cyclo-L-Pro-D-Leu from the reaction system with D-Leu, L-Pro and P3m. The peaks in the gridlines represent corresponding products cyclo-Pro-Leu. The peak with retention time of 11.9 min was cyclo-Pro-Pro.
FIGURE 4. The relative intensity of UV- absorption of the cyclo-Pro-AAs at 210 nm in these reactions. (A) Bar graph representation of the reaction L-Pro with four L-/D-AAs, respectively. (B) Bar graph representation of the reaction D-Pro with four L-/D-AAs, respectively.
Moreover, analogous experiments were also performed with the other three L-/D-AAs (Arg, Met and Ala) revealing similar selectivity for the cyclo-L-Pro-AAs and cyclo-D-Pro-AAs species. That was L-Pro preferentially reacted with the D-AA to form cyclo-L-Pro-AA while D-Pro chose L-AA more than D-AA to form cyclo-D-Pro-AA (Figure 4; Supplementary Figures S1, S4–S15). It was further shown that the yield of heterochiral cyclo-Pro-AA was higher than that of homochiral cyclo-Pro-AA (Figure 5; Supplementary Figure S16).
FIGURE 5. Effect of chirality of Pro and Leu on the cyclo-Pro-Leu formation. These reactions were carried out under standard conditions.
In the case of Ala, the reaction with L-/D-Pro showed EIC (m/z 169.0972) of cyclo-Pro-Ala yielding one peak with the retention time of 8.6 min (Supplementary Figures S12–S15). The unusual behavior of Ala was attributed to the small polarity difference between two stereoisomers of cyclo-Pro-Ala, which results in their retention times being almost identical in the HPLC-MS.
Taken together, our data showed that chiral selection of amino acids can occur during the process of AAs reaction with Pro generating cyclo-Pro-AAs. These results revealed that the formation of cyclo-Pro-AAs for the reaction of L-/D-AA and Pro exhibit an intrinsic chiral selection at the molecular level. Figure 4 shows that the chiral selectively was about 1.65–6.61 fold for each case, respectively. Alata argues that cyclic dipeptide was a planar structure when it was formed by amino acids with small side chains (e.g., glycine). When the side chain groups of amino acids are larger, a planar structure is produced only when the amino acids have opposite chirality which had smaller formation energy (Alata et al., 2017). It is coincided with our results. In other words, heterochiral cyclic dipeptides are formed more than homochiral cyclic dipeptides. Pro is thought to have existed in the prebiotic environment and was one of the first amino acids to be biologically encoded (Zaia et al., 2008). Cis-trans isomerization reactions usually limit the rate of peptide and protein folding (Wedemeyer et al., 2002). Pro can kink the helix, terminate the helix, and form amide hydrogen without the helix (Counterman and Clemmer, 2004). We speculate that Pro may have provided a special contribution to the evolution of peptides due to its aforementioned properties. Studying the formation and structure of Pro-containing DKP may be a key step in prebiotic peptides. According to previous studies, some characteristic environmental factors could achieve chiral enrichment of amino acids from the initial racemic mixing in the early Earth (Bondy and Harrington, 1979; Hazen et al., 2001; Wedyan and Preston, 2005; Breslow and Cheng, 2009; Fraser et al., 2011a; Fraser et al., 2011b). During the long-term evolution of the Earth, some environmental conditions led to selective enrichment of L-AA under a prebiotic environment (Bondy and Harrington, 1979; Breslow and Cheng, 2009). In the above mentioned case, D-AAs are more likely to participate in the formation of cyclic dipeptides in the presence of L-Pro of enantio-enrichment, resulting in more L-AAs to take part in other reactions (e.g., linear dipeptides synthesis). Although this chiral selection is under weak pressure, it could lead to an overpowering preference to use L-amino acids in the biological system over the long period of the prebiotic evolution process.
In conclusion, reactions of L-/D-amino acids (AAs) with Pro can shape the corresponding chiral cyclo-Pro-AAs in the alkaline aqueous solution. Furthermore, the chiral selectivity of AAs, during the cyclo-Pro-AAs formation, has been found at the molecular level. In other words, cyclo-Pro-AAs can be synthesized by L-Pro with a clear preference for D-AA over L-AA. Vice-versa, L-AA was preferred over D-AA in the presence of D-Pro. These findings are very helpful to understand the chiral selection of AA in the prebiotic chemical evolution. Therefore, we believe that the fixed chirality of AA in biosystem peptides is not necessarily determined in a certain process, but may be caused by the confluence of multiple processes.
The original contributions presented in the study are included in the article/Supplementary Material, further inquiries can be directed to the corresponding authors.
YZ conceived the research. JY designed the experiments. YG jointly performed key research and experiments. YZ and YL performed HPLC-MS. YZ, JY, and GZ wrote the paper.
This work was supported by the National Natural Science Foundation of China (Nos. 42003062, 91856126 and 21778042), Scientific Research Grant of Ningbo University (No. 215-432000282), Ningbo Top Talent Project (No. 215-432094250) and Scientific Research Foundation of Key Laboratory of Marine Biogenetic Resources, Third Institute of Oceanography, Ministry of Natural Resources (No. HY202104).
The authors declare that the research was conducted in the absence of any commercial or financial relationships that could be construed as a potential conflict of interest.
All claims expressed in this article are solely those of the authors and do not necessarily represent those of their affiliated organizations, or those of the publisher, the editors and the reviewers. Any product that may be evaluated in this article, or claim that may be made by its manufacturer, is not guaranteed or endorsed by the publisher.
We thank Songsen Fu (Ningbo University) and Yile Wu (Ningbo University) for many constructive comments on this manuscript.
The Supplementary Material for this article can be found online at: https://www.frontiersin.org/articles/10.3389/fspas.2022.794932/full#supplementary-material
Alata, I., Pérez-Mellor, A., Ben Nasr, F., Scuderi, D., Steinmetz, V., Gobert, F., et al. (2017). Does the Residues Chirality Modify the Conformation of a Cyclo-Dipeptide? Vibrational Spectroscopy of Protonated Cyclo-Diphenylalanine in the Gas Phase. J. Phys. Chem. A. 121, 7130–7138. doi:10.1021/acs.jpca.7b06159
Bondy, S. C., and Harrington, M. E. (1979). L Amino Acids and D-Glucose Bind Stereospecifically to a Colloidal clay. Science 203, 1243–1244. doi:10.1126/science.424749
Bonner, W. A. (2000). Parity Violation and the Evolution of Biomolecular Homochirality. Chirality 12, 114–126. doi:10.1002/(sici)1520-636x(2000)12:3<114:aid-chir3>3.0.co;2-n
Borthwick, A. D. (2012). 2,5-Diketopiperazines: Synthesis, Reactions, Medicinal Chemistry, and Bioactive Natural Products. Chem. Rev. 112, 3641–3716. doi:10.1021/cr200398y
Botti, P., Pallin, T. D., and Tam, J. P. (1996). Cyclic Peptides from Linear Unprotected Peptide Precursors through Thiazolidine Formation. J. Am. Chem. Soc. 118, 10018–10024. doi:10.1021/ja954278g
Breslow, R., and Cheng, Z.-L. (2009). On the Origin of Terrestrial Homochirality for Nucleosides and Amino Acids. Proc. Natl. Acad. Sci. 106, 9144–9146. doi:10.1073/pnas.0904350106
Counterman, A. E., and Clemmer, D. E. (2004). Anhydrous Polyproline Helices and Globules. J. Phys. Chem. B 108, 4885–4898. doi:10.1021/jp036454a
Fernández Guasti, M. (2019). Chirality, Helicity and the Rotational Content of Electromagnetic fields. Phys. Lett. A 383, 3180–3186. doi:10.1016/j.physleta.2019.06.002
Fraser, D. G., Fitz, D., Jakschitz, T., and Rode, B. M. (2011a). Selective Adsorption and Chiral Amplification of Amino Acids in Vermiculite clay-implications for the Origin of Biochirality. Phys. Chem. Chem. Phys. 13, 831–838. doi:10.1039/c0cp01388a
Fraser, D. G., Greenwell, H. C., Skipper, N. T., Smalley, M. V., Wilkinson, M. A., Demé, B., et al. (2011b). Chiral Interactions of Histidine in a Hydrated Vermiculite clay. Phys. Chem. Chem. Phys. 13, 825–830. doi:10.1039/c0cp01387k
Guo, Y., Ying, J., Sun, D., Zhang, Y., Zheng, M., Ding, R., et al. (2021). Cyclic Dipeptides Formation from Linear Dipeptides under Potentially Prebiotic Earth Conditions. Front. Chem. 9, 398. doi:10.3389/fchem.2021.675821
Hazen, R. M., Filley, T. R., and Goodfriend, G. A. (2001). Selective Adsorption of L- and D-Amino Acids on Calcite: Implications for Biochemical Homochirality. Pnas 98, 5487–5490. doi:10.1073/pnas.101085998
Hazen, R. M., and Sholl, D. S. (2003). Chiral Selection on Inorganic Crystalline Surfaces. Nat. Mater 2, 367–374. doi:10.1038/nmat879
Huber, C., Eisenreich, W., Hecht, S., and Wächtershäuser, G. (2003). A Possible Primordial Peptide Cycle. Science 301, 938–940. doi:10.1126/science.1086501
Jainta, M., Nieger, M., and Bräse, S. (2008). Microwave-Assisted Stereoselective One-Pot Synthesis of Symmetrical and Unsymmetrical 2,5-Diketopiperazines from Unprotected Amino Acids. Eur. J. Org. Chem. 2008, 5418–5424. doi:10.1002/ejoc.200800605
Joshi, P. C., Ferris, J. P., and Pitsch, S. (2000). Homochiral Selection in the Montmorillonite-Catalyzed and Uncatalyzed Prebiotic Synthesis of RNA. Chem. Commun., 2497–2498. doi:10.1039/b007444f
Lambert, J. N., Mitchell, J. P., and Roberts, K. D. (2001). The Synthesis of Cyclic Peptides. J. Chem. Soc. Perkin Trans. 1, 471–484. doi:10.1039/b001942i
Manchineella, S., Prathyusha, V., Priyakumar, U. D., and Govindaraju, T. (2013). Solvent‐Induced Helical Assembly and Reversible Chiroptical Switching of Chiral Cyclic‐Dipeptide‐Functionalized Naphthalenediimides. Chem. Eur. J. 19, 16615–16624. doi:10.1002/chem.201303123
Nonappa, N., Ahonen, K., Lahtinen, M., and Kolehmainen, E. (2011). Cyclic Dipeptides: Catalyst/promoter-free, Rapid and Environmentally Benign Cyclization of Free Amino Acids. Green. Chem. 13, 1203–1209. doi:10.1039/c1gc15043j
Parker, E. T., Zhou, M., Burton, A. S., Glavin, D. P., Dworkin, J. P., Krishnamurthy, R., et al. (2014). A Plausible Simultaneous Synthesis of Amino Acids and Simple Peptides on the Primordial Earth. Angew. Chem. Int. Ed. 53, 8132–8136. doi:10.1002/anie.201403683
Poullennec, K. G., Kelly, A. T., and Romo, D. (2002). Highly Diastereoselective Desymmetrizations of Cyclo(Pro,Pro): An Enantioselective Strategy toward Phakellstatin and Phakellin. Org. Lett. 4, 2645–2648. doi:10.1021/ol026140q
Prasad, C. (1995). Bioactive Cyclic Dipeptides. Peptides 16, 151–164. doi:10.1016/0196-9781(94)00017-z
Rabinowitz, J., Flores, J., Krebsbach, R., and Rogers, G. (1969). Peptide Formation in the Presence of Linear or Cyclic Polyphosphates. Nature 224, 795–796. doi:10.1038/224795a0
Rikken, G. L. J. A., and Raupach, E. (2000). Enantioselective Magnetochiral Photochemistry. Nature 405, 932–935. doi:10.1038/35016043
Tamura, K. (2008). Origin of Amino Acid Homochirality: Relationship with the RNA World and Origin of tRNA Aminoacylation. Biosystems 92, 91–98. doi:10.1016/j.biosystems.2007.12.005
Trauger, J. W., Kohli, R. M., Mootz, H. D., Marahiel, M. A., and Walsh, C. T. (2000). Peptide Cyclization Catalysed by the Thioesterase Domain of Tyrocidine Synthetase. Nature 407, 215–218. doi:10.1038/35025116
Wedemeyer, W. J., Welker, E., and Scheraga, H. A. (2002). Proline Cis−Trans Isomerization and Protein Folding. Biochemistry 41, 14637–14644. doi:10.1021/bi020574b
Wedyan, M., and Preston, M. R. (2005). Isomer-selective Adsorption of Amino Acids by Components of Natural Sediments. Environ. Sci. Technol. 39, 2115–2119. doi:10.1021/es040474o
Yachmenev, A., Onvlee, J., Zak, E., Owens, A., and Küpper, J. (2019). Field-Induced Diastereomers for Chiral Separation. Phys. Rev. Lett. 123, 243202. doi:10.1103/PhysRevLett.123.243202
Yamagata, Y., Watanabe, H., Saitoh, M., and Namba, T. (1991). Volcanic Production of Polyphosphates and its Relevance to Prebiotic Evolution. Nature 352, 516–519. doi:10.1038/352516a0
Ye, X., Cui, J., Li, B., Li, N., Wang, R., Yan, Z., et al. (2019). Enantiomer-selective Magnetization of Conglomerates for Quantitative Chiral Separation. Nat. Commun. 10, 1964. doi:10.1038/s41467-019-09997-y
Ying, J., Fu, S., Li, X., Feng, L., Xu, P., Liu, Y., et al. (2018a). A Plausible Model Correlates Prebiotic Peptide Synthesis with the Primordial Genetic Code. Chem. Commun. 54, 8598–8601. doi:10.1039/c8cc04767g
Ying, J., Lin, R., Xu, P., Wu, Y., Liu, Y., and Zhao, Y. (2018b). Prebiotic Formation of Cyclic Dipeptides under Potentially Early Earth Conditions. Sci. Rep. 8, 936. doi:10.1038/s41598-018-19335-9
Keywords: chiral selection, amino acid, cyclic dipeptide, prebiotic chemistry, origin of life
Citation: Guo Y, Zhang Y, Ying J, Liu Y, Zhang G and Zhao Y (2022) Selection of Amino Acid Chirality Induced by Cyclic Dipeptide Synthesis in Plausible Prebiotic Conditions. Front. Astron. Space Sci. 9:794932. doi: 10.3389/fspas.2022.794932
Received: 14 October 2021; Accepted: 21 January 2022;
Published: 09 February 2022.
Edited by:
Yiliang Li, The University of Hong Kong, Hong Kong SAR, ChinaReviewed by:
Albert Rimola, Universitat Autònoma de Barcelona, SpainCopyright © 2022 Guo, Zhang, Ying, Liu, Zhang and Zhao. This is an open-access article distributed under the terms of the Creative Commons Attribution License (CC BY). The use, distribution or reproduction in other forums is permitted, provided the original author(s) and the copyright owner(s) are credited and that the original publication in this journal is cited, in accordance with accepted academic practice. No use, distribution or reproduction is permitted which does not comply with these terms.
*Correspondence: Jianxi Ying, eWluZ2ppYW54aUBuYnUuZWR1LmNu; Gaiyun Zhang, emhneXVuQHRpby5vcmcuY24=; Yufen Zhao, emhhb3l1ZmVuQG5idS5lZHUuY24=
Disclaimer: All claims expressed in this article are solely those of the authors and do not necessarily represent those of their affiliated organizations, or those of the publisher, the editors and the reviewers. Any product that may be evaluated in this article or claim that may be made by its manufacturer is not guaranteed or endorsed by the publisher.
Research integrity at Frontiers
Learn more about the work of our research integrity team to safeguard the quality of each article we publish.