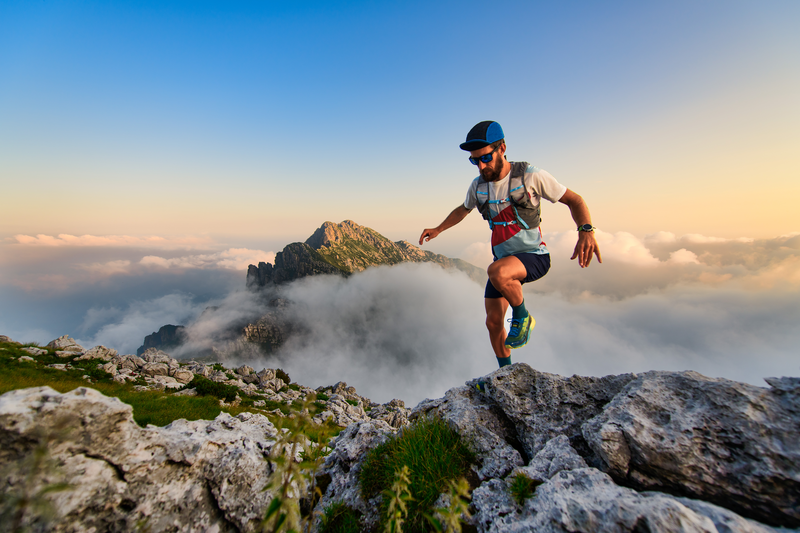
94% of researchers rate our articles as excellent or good
Learn more about the work of our research integrity team to safeguard the quality of each article we publish.
Find out more
ORIGINAL RESEARCH article
Front. Water , 28 October 2024
Sec. Environmental Water Quality
Volume 6 - 2024 | https://doi.org/10.3389/frwa.2024.1474499
Agriculture-dependent developing Southeast Asian countries need to assess residual pesticides in receiving water from agricultural runoff as one of the basis for the establishment of baseline data. Monitoring residual pesticides in surface water is challenging due to pesticides’ irregular/intermittent emission and low solubility in water. To address the challenge of pesticide assessment, passive samplers composed of silicon rubber sheets (SR) and speedisk (SD) were used to capture hydrophobic and hydrophilic organic pesticides and other organic pollutants, respectively. Samplers were submerged uninterruptedly for at least 30 days in three selected sites in Can Tho River, Mekong Delta, Vietnam, and Cagayan de Oro River Basin, Philippines, for 2 years. Passive samplers (SR spiked with 232 pesticides) captured 83 and 69 residual pesticides at concentrations of ng/L levels in Vietnam and the Philippines, respectively, which is unattainable through conventional grab sampling methods. Trace concentrations of banned compounds such as OCPs, PCBs, and PAHs were also detected at pg./L levels. The success of this alternative methodology can be attributed to the combination of passive samplers tailored for nonpolar (water-insoluble) and polar (water-soluble) organic contaminants coupled with sensitive analytical instruments, including GCMSMS and LCMSMS.
Agriculture is the economic backbone of the developing countries in Southeast Asia. The Association of Southeast Asian Nations, ASEAN, envisioned economic prosperity in its member nations through the created integrated economy that allows the free movement of goods and services, skilled labor, and capital among member nations (Ishikawa, 2021). ASEAN’s major concern to its member nations is food security due to their agricultural dependency and growing population. As of 2022, ASEAN has a combined population among its 10 member nations of approximately 673 million (Statista, 2024). ASEAN’s vision paved the way for the economic growth of the region by supporting livelihood and the protection of social stability (Sundram, 2023).
The ASEAN integration created an economic shift from an agrarian economy to a highly commercialized agriculture. The shift is strengthened by policy initiatives and played an important role in the economic growth of largely agriculture-dependent countries (Hurni et al., 2017). For the last decade, the vision brought economic growth through agricultural expansion in the Southeast Asia region.
Among ASEAN member nations, Vietnam shows impressive economic growth through agriculture expansion. The dramatic economic growth experienced by Vietnam has been attributed to a series of economic reforms known as “doi moi” and its membership in ASEAN in 1995 (Emmers and Le Thu, 2021; Van Lam, 2019). It showed transformation in the agricultural sectors that benefitted the marginalized and reduced poverty. The latest decrease was attributed to the improvement of income of the Vietnamese farmers in the highland by encouraging them to grow profitable agro-industrial crops (The World Bank, 2018).
In the Philippines, the agribusiness shift, which transpired from the ASEAN integrated economic goal implemented in early 2000, converted approximately two (2) million hectares of forest and agricultural lands, or the so-called “idle lands,” to agribusiness areas within 5 years from 2005 to 2010 (Pulhin and Ramirez, 2013). The agribusiness shift was under the directives of the 2004–2010 Philippine Medium Term Development Plan, adopted in the 2011–2016 Philippine Development Plan (PDP), further enhanced by PDP 2016–2022, and now the PDP 2023–2028, with the current administration, further enhanced as the government’s strategy to improve the economy and alleviate poverty (Briones et al., 2023; Custodio and Sombilla, 2020).
In both countries, Vietnam and the Philippines, aside from agricultural expansion, intensive agriculture using pesticides is practiced (Tran et al., 2018). According to the latest data, Vietnam has a total land area of 331,690 sq. km; 39.4% is agricultural land, and 47% is forest area. Vietnam’s agricultural value added in USD is 51.40B, with a crop production index of 109.6 index points. But has high fertilizer usage of 427.6 kg per hectare of arable land (TheGlobalEconomy.com, 2024b). The Philippines has a total land area of 343,450 sq. km, of which 42.5% is agricultural land with only 24.2% forest area. The agricultural value added in USD is 41.08B, with a crop production index of 104.0 index points. The fertilizer usage is 232.0 kg per hectare of arable land, which is almost 50% less than Vietnam’s (TheGlobalEconomy.com, 2024a).
In agriculture, the benefits of pesticides are well recognized and understood, as they are developed with strict guidelines for very reasonable purposes. However, if necessary, agricultural intensification uses more pesticides to increase productivity per land area. An increase in the chemical application will affect the crops and soil quality, which will then affect the water quality of the receiving water through agricultural runoff. Pesticide application in farms contaminates air, soil, water, and other organisms. This study assesses the residual pesticide contamination on the receiving surface water brought about by agricultural runoff.
The residual pesticide contamination in the surface water validates the presence of contamination of the pesticides farmers extensively use. Assessing residual pesticides in water resources is challenging due to the very low solubility of ppb (parts per billion) concentrations in water (10−9) or even lower. One major problem in residual pesticide assessment is the fluctuating and irregular emission of pesticides in surface water. Hence, using the traditional grab water sampling method to obtain water samples taken in a specified time can give a wide range of values that often give unreliable results.
To address the problem of monitoring residual pesticides in water, it has been substituted by monitoring pesticides on sediments and tissues. These substitution methods have drawbacks. Despite direct water exposure, the tissue samples failed to consider other chemical pollutants, low bioaccumulation, avoidance, metabolism, and organism death (Alvarez, 2013). Aside from the low solubility of pesticides, sporadic emission is also a significant problem in which the commonly used water sampling method is limited. Passive samplers (PS) are an alternative sampling technique that enables the accumulation of pollutants in the samplers for more reliable monitoring of residual pesticides in water (Valenzuela et al., 2020).
The sampling method must be addressed to attain reliable results. Thus, this study uses passive samplers (PS) instead of the traditional water grab sampling method. Passive sampling methods were first developed in the early 70s for air pollutants and were used to determine occupational exposure to volatile organic chemicals in the air (Carmichael et al., 2003). Byrne and Aylott (1980) made the first patented PS in water, which used a nonpolar solvent in a nonporous membrane. Early PS was developed to effectively measure traces of POPs in water using a semipermeable membrane device, SPMD and is still currently used (Booij et al., 1998; Gonçalves et al., 2021).
After introducing SPMD, sampling devices for nonpolar organic contaminants have been developed. One is the polar organic integrated sampler (POCIS), tested in hydrophilic compounds like atrazine, diazinon, and 17-α-ethynylestradiol, which were not readily measured in the other devices (Kolpin et al., 2002). Several passive samplers have been developed, including an Empore disk, a Chemcatcher, a membrane-enclosed silicon collector, and a polyoxymethylene sheet (Becker et al., 2021; Gravell et al., 2020; Odetayo et al., 2020).
Some may argue that residual pesticide assessment using PS involves considerable investments in analytical instruments, which could be challenging in developing countries. But this is also true for the grab water sampling method. Generally, the PS method is cost-effective, consistent, practical, and suitable for routine monitoring (Deltares, 2016; Górecki and Namieśnik, 2002; Regan et al., 2018). It can detect low concentrations of organic compounds in pg./L to ng/L, which is difficult to attain in the grab sampling method (Hawthorne et al., 2011; Moschet et al., 2014).
For Vietnam and the Philippines, pesticides are used for practical purposes, such as preserving crops and controlling infestations for continuous production to cater to the client’s needs. What is overlooked is that when a pesticide application is mismanaged or overused, it has the potential to cause harm to humans and the environment. These residual pesticides in farms are potential runoff pollutants in surface water that can affect communities living along rivers dependent on these contaminated surface waters for domestic use. Worst, if residual pesticides are measured in communities where these pesticides were never applied.
Thus, in this study, the assessment of residual pesticides of two river systems in two developing Southeast Asian countries, namely, Can Tho River, Vietnam, and Cagayan de Oro River Basin (CdO), Philippines, the samplers developed by Deltares for more than a decade will be used (Deltares, 2019a). This set of PS gives good estimates of residual organic pesticides for the following reasons: it can detect and measure fluctuating emission of organic pollutants; it can detect more organic substances on water samples taken at the same time compared to the grab sampling method (Booij and Smedes, 2010; Deltares, 2019a; Smedes and Booij, 2012).
Moreover, the PS used in this study is one of the few deployed in the abovementioned advanced sampling devices to analyze and evaluate the residual pesticides that are unintentionally disposed of and transported through surface runoff from upstream farms to receiving waters. The water sampling using PS will be conducted uninterruptedly for at least 30 days for 2 consecutive years. In addition to the residual pesticides, traces of persistent organic pollutants (POPs) banned two decades ago will also be assessed. These POPs include organochlorine pesticides (OCP), polychlorinated biphenyls (PCB), and polycyclic aromatic hydrocarbons (PAH).
This study conducted residual pesticide assessment in nanogram/liter (ng/L) and other organic pollutants in picogram/liter (pg/L) in rivers using passive samplers in two developing countries in Southeast Asia that are agriculture-dependent. The chosen rivers are strategically located; the upstream are primarily farms, and the downstream areas are urban communities experiencing flooding. The samplers are silicon rubber (SR) and speedisk (SD). SR is designed for nonpolar organic compounds (insoluble in water), and SD for polar organic compounds (water-soluble). Before water sampling, samples were prepared in Deltares, TNO Laboratories, Utrecht, The Netherlands. At least after 30 days of uninterrupted sampling, the samplers were dismantled and shipped back to Deltares for analysis using GCMSMS and LCMSMS. The pesticide assessments were conducted for 2 consecutive years in Vietnam and the Philippines.
Can Tho is located in the southern part of the country, in the Mekong Delta. It is internationally known for its floating markets. Daily, small to medium-scale farmers travel by boats and sell their crops on these floating markets to business people onshore, either by themselves or through go-betweens. Can Tho River is essential and the economic center for Can Tho City in terms of agricultural trading, transport, and tourism. Can Tho have 158 rivers and canals with deteriorating water quality (Leloup et al., 2013; Salingay et al., 2017).
The specific sampling sites in Can Tho River, Vietnam where the assessment of residual pesticide were conducted. Three sampling sites are shown in Figure 1. Thi Tran, Phong Điền (DMS: 10000′54.8″N 105038′55.8″E,) designated as S1, and Nhon Nghia Phong Điền (DMS: 9059′07.9″N 105041′26.6″E), designated as S2, are located in the rural district of Phong Dien, Can Tho. S1 and S2 are located before and after the Phong Dien floating market. An Binh (DMS: 10000′25.3″N 105044′49.3″E) designated as S3, is located in the urban district of Nien Kieu, before the An Binh public market and across the famous “Cai Rang” floating market.
Figure 1. Three sampling sites in Song Can River, Vietnam, Mekong Delta were: S1, Thi Trang, Phong Dien; S2, Nhon Nghia, Phong Dien, and S3, An Binh, Ninh Kieu.
The research was conducted in the northern part of Northern Mindanao, Philippines, where agriculture is the primary source of the economy and where agricultural expansion and intensification are currently implemented. One of the region’s significant water resources is Mt. Kitanglad Mountain Range, which is approximately 3,000 m above sea level and a catchment of various rivers. The research area is one of the central river systems of Mt. Kitanglad watershed, CDO River Basin, which drains toward the CdO River in CdO City downstream.
The three sampling sites in the CDO River Basin were in Lapinigan Creek, Bubunawan River Junction (DMS: 8018′46.1″N 124041′54.8″E) designated as S1; Maasin, CdO River midstream (DMS: 8023′17.9″N 124036′48.8″E) designated as S2 and Taguanaw-Lumbia Diversion; CdO River downstream (DMS: 8025′11.9″N 124037′46.7″E) designated as S3. The detailed location of the sampling sites is shown in Figure 2. S1 and S2 are located along agricultural areas (mostly annual crops), while S3 is located along wooden grassland and residential areas.
Figure 2. Pesticide assessments were conducted in the following rivers: S1 Bubunawan River, Libona; S2 CdO River (midstream), Maasin and S3 CdO River (downstream), Taguanaw.
The PS in this study consists of silicone rubber (SR) and speedisk sampler (SD) to measure nonpolar (insoluble in water) and polar organic (water-souble) compounds, respectively. SR and SD have been developed for more than a decade by Deltares, a research institute in the Netherlands (Deltares, 2019b). SR and SD are expected to give reasonable estimates of ultra-trace organic pollutants in water, even at low concentrations of pico-nanogram per liter.
Silicone rubber before deployment were spiked with performance reference compounds (PRC). The complete list of PRC is shown in Supplementary Table 1. PRC will be used to calculate the freely dissolved concentrations in water and estimate the sampled volume. Unlike SR, SD is an adsorption material enclosed by a rigid propylene cage and will not be spiked by PRC. The established release model on the pre-spiked SR will be used to calculate the freely dissolved concentrations in water and estimate the sampled volume. Concentrations of freely dissolved pyrene and fluoranthene play essential roles in measuring pesticides in SD. These two compounds show comparable behavior in SR and SD (Rusina et al., 2010).
Before water sampling, SR was pre-cleaned for 100 h using ethyl acetate solvent in a soxhlet apparatus. The ethyl acetate was removed by soaking the SR in methanol twice for eight (8) h of approximately 4 mL of methanol per sheet. The SR samplers were spiked with PRC. Unlike SR, SD, as an adsorption sampler, was not pre-spiked with PRC. Samplers SR and SD were stored in glass containers until the actual sampling.
The deployment of PS started approximately 60 days after the rainy season and was completed almost at the end. In the deployment, PS comprised six (6) sheets of SR and three (3) pieces of SD. These were attached firmly in an iron post and submerged below the water surface at approximately 1.5–2.0 m for at least 30 days uninterrupted for the 2 consecutive years of monitoring. After sampling, the PS was dismantled, as shown in Figures 3, 4. The set of PS was kept in its original glass containers and stored at −20°C before actual analyses.
Figure 3. The upper left image shows the passive samplers used, which were composed of six silicon rubbers (SR) and three speedisks (SD) arranged and individually tied with tie wires and arranged in a plastic wire with brick block for added weight and support. The set was submerged in river water uninterruptedly for at least 30 days in three sampling sites: images in lower left is VS1, upper right is VS2, and lower right is VS3.
Figure 4. Passive samplers composed of six silicone rubbers (SR) and three speedisks (SD) arranged in an iron stand as shown in the upper left picture. The iron stand with the samplers was submerged in river water uninterrupted for at least 30 days as shown in lower left figure. The three middle pictures are the sampling sites; upper is PS1, the middle is PS2, and the lower is PS3. Right picture the removal of iron in preparation for pesticide analyses.
The preparation of samplers before water sampling and the extraction and chemical analysis of contaminants were done in the EMSA laboratory of TNO in Utrecht, the Netherlands. Both samplers, SR and SD, were extracted by acetonitrile through dialysis and, successively, after mild evaporation, transferred into hexane for analysis. The separation, identification, and quantification of pesticides were done by HPLC, GC–MS MS, and LC–MS MS.
The release model of Rusina et al. (2010) was used to calculate the freely dissolved concentrations in water and estimate the sampled volume on the pre-spiked PRC on SR before deployment. The freely dissolved concentrations and the respective sampled volumes were calculated by integrating the freely dissolved concentrations of fluoranthene and pyrene from SR with the measured concentration in SD. These two compounds exhibit comparable behaviors in both samplers.
The analyte’s aqueous concentrations were calculated using the formulas developed by Smedes and Booij (2012). The water sampling rates were calculated by the retained PRC fractions, ratios of the amount of PRC at the beginning and end of the exposure. The PRC data were pre-screened before calculating the sampling rates. The detection limits from the extract may depend on the number of other compounds present. PRC was further examined for possible interferences. It can only be processed further if the PRC peak shows no interferences.
The PRC fractions (f) can be calculated by Equation 1:
Where Nt and N0 are, respectively, the dosed amount in the reference SR and the amount after exposure; Rs is the equivalent water sampling rate (d-1), t is the exposure time (d), m is the mass of the SR (kg) and Kpw (kg−1) is SR-water partition coefficient. The Kpw can be obtained from Smedes et al. (2009). The degree of Rs can be controlled by the passage of the water boundary layer as Rusina et al. (2010) prove that Rs decreases with increasing molar mass (M) as shown in Equation 2.
where B is a proportionality constant that is proportional to the sampler’s surface area. Thus Equation 3 becomes:
The absorbed organic compounds were calculated by Equations 4, 5 as given by Booij et al. (2007):
Then, using the proportionality constant B, Cw can be calculated by combining Equations 2, 5 to have a general Equation 6 that incorporates the linear uptake, transition, and equilibrium stages.
The Kpw values were necessary for PRCs and targeted organic compounds that approach equilibrium. Kpw values can be obtained from Smedes et al. (2009).
Both the SR and SD samplers were capable of detecting multiple pesticides. While certain pesticides were consistently measured throughout the 2-year monitoring period, others were only detected in the first year and not in the second year, and vice versa. The residual pesticide concentrations are detailed in Tables 1, 2, while values for other organic contaminants can be found in Tables 3, 4. It is important to note that “not detected (nd)” values do not necessarily indicate the absence of a particular pesticide. If a pesticide was detected at least once in a sampling site, the lack of detection in subsequent samples may be attributed to extremely low concentrations, making quantification unfeasible, or potentially due to farmers not utilizing such pesticides during the sampling period.
Table 1. Residual pesticide concentrations in Can Tho River, Vietnam in nanogram per liter (ng/L) per sampling site for 2 years.
Table 2. Residual pesticide concentrations in the Cagayan de Oro River Basin, Philippines in nanogram per liter (ng/L) per sampling site for 2 years.
Table 3. Trace concentrations of OCP, PCB, and PAH measured in picogram per liter (10−12 g/L) in per sampling site in Can Tho River, Vietnam.
Table 4. Trace concentrations of OCP, PCB, and PAH measured in picogram per liter (10−12 g/L) in per sampling site in Cagayan de Oro River Basin, Philippines.
There were 83 organic residual pesticides measured in nanogram per liter concentrations. Based on the type of pesticides measured, Vietnamese farmers used various insecticides (47%) as compared to fungicides (31%), herbicides (18%), repellents, and rodenticides (4%). The methodology used in this study is not the general procedure set by the Vietnamese government. The established governmental standards relied on the conventional grab water sampling technique, whereas this study employed passive samplers in conjunction with more sensitive analytical instruments. Consequently, the generic threshold level of 10 ng/L was adopted in this study. As shown in Table 1, 83 organic pesticides were measured and monitored for 2 years. Out of 83 pesticides, 27 exceeded the applied threshold level of 10 ng/L for at least one sampling site for the duration of the study. These 27 prevalent pesticides are highlighted in Table 1.
The 27 pesticides that exceeded the threshold level are the following: 2,4-D, 2-aminoacetophenone, acetamiprid, atrazine, atrazine-desethyl, azoxystrobin, carbendazim, chlorpyrifos-ethyl, chlorantraniliprole, clopyralid, DEET, dicamba, dimethoate, dimethomorph, dinoterb, diuron, dodine, ethoprophos, fipronil, imidacloprid, metalaxyl, paclobutrazol, propiconazole, tebuconazole, terbutryn, terbutalazine, and triadimenol.
For the trend of these prevalent pesticides. Most of these 27 pesticides were measured in both samplers and of higher values obtained in SR than in SD. Except in the following cases: 2,4-D was only measured in SD, 2-aminoacetophenone had high contamination of 1,920 ng/L in SR of VS2 in the second year of monitoring, clopyralid was only measured in SR of VS2 in year 2, dimethoate in SR of VS1 in year 1, imidacloprid was measured only in SD in year 1 but measured in all SR and SD in year 2.
Some of the 83 pesticides were measured only in a specific monitoring year. There were 22 pesticides measured only on the first year and not detected on the second year. These are the following: Bifenox, brodifacoum A, brodifacoum B, Cinerin II, cypermethrin (sum), daminozide, dichlofluanide, dichlorvos, dimethoaat, dimethomorph A, dimethomorph B, etoxazole, Fenamifos, fluxapyroxad, isoprocarb, methiocarb, milbemectin A4, oxamyl, parathion-ethyl, pyridaben, tolclofos-methyl, and trifloxystrobin acid. And the following 11 pesticides measured only on the second monitoring year: Chlortoluron, clopyralid, clothianidin, cyfluthrin (sum), dicamba, dinoterb, fenarimol, hexythiazox, imazalil, penconazool, and pencycuron.
For the 2 years monitoring, there were pesticides measured only at a specific sampling site and at a particular sampling period. In the first year, isoprocarb and pyridaben were measured only in VS1, daminozide only in VS2, and Bifenox, brodifacoum A and B, carbaryl, demothoate, etoxazole, and parathion-ethyl only in VS3. For the second year, chlortoluron, clopyralid, dicamba, and imazalil were measured in VS 2 only, and carbaryl, and penconazole were measured only in VS3.
In general, the values recorded at VS 1 (upstream) exhibited higher concentrations compared to the two other sampling sites. However, the values obtained across all three sites were predominantly similar, with minor discrepancies observed, except in specific instances. For instance, 2-aminoacetophenone, atrazine-desethyl, and DEET exhibited elevated concentrations in VS 2 (midstream), particularly during the second year of monitoring. Additionally, dicamba and dodine were solely detected in VS 2 during the second year of monitoring. Dimethoate, conversely, was exclusively measured at VS 3 during the first year of observation. These notable exceptions, characterized by significantly higher concentrations at particular sampling sites, highlight localized pollutant occurrences within those specific areas.
There were 69 residual organic pesticides measured in nanograms per liter (ng L−1) as shown in Table 2. Based on type of pesticides measured, just like the Vietnamese, Filipino farmers used various insecticides (45%) as compared to fungicides (33.3%), herbicides (17.4%), repellents, and rodenticides (4.3%).
The river systems under study were classified by the concerned government agency as Class A. This means, these water sources require conventional treatment to meet the Philippines National Standards for Drinking Water (DENR, 2016). Just like Vietnam, the government set standards use the traditional grab water sampling method with different analytical instruments as compared to this study.
Out of 69 pesticides, 13 exceeded the applied threshold level of 10 ng/L for at least one sampling site for the duration of the study. These pesticides are boscalid, carbendazim, DEET, dichlorvos, dimethomorph, diuron, epoxiconazole, fenamiphos, metalaxyl, monuron, propiconazole, pyrimethanil, and simazine.
The pattern observed with these predominant pesticides differs from that observed in Vietnam. Specifically, boscalid, diuron, fenamiphos, metalaxyl, and pyrimethanil were consistently detected in both SR and SD samplers throughout the 2-year monitoring period. Carbendazim was similarly measured across all sampling sites, although not in both types of samplers. Dichlorvos, however, registered a concentration of only 11 ng/L in the SR sampler of PS1 during the first year of monitoring. Dimethomorph B was solely detected in the first year, while epoxiconazole was exclusively observed in the second year. Monuron was exclusively measured in both samplers at PS1 over the 2-year monitoring period, while simazine was solely detected in the SR sampler during the first year of observation.
In the 2-year pesticide monitoring 22 out of the 69 pesticides were measured only in the first year. These were 2,4-D, 2-aminoacetophenon, bifenox, bifenthrin, bitertanol (sum), brodifacoum A and B, carbofuran, Cinerin II, cypermethrin (sum), dichlofluanide, dimethomorph A and B, dimethomorph B, endosulfan-alfa, fluazifop-butyl, fluxapyroxad, methomyl, milbemectin A4, simazine, Terbutryn, terbutylazine, and tolclofos-methyl. There were 14 pesticides measured only on the second year of monitoring. These are the following: acequinocyl, carbaryl, cyfluthrin (sum), dinoterb, DMST, dodine, fenarimol, imidacloprid, Jasmolin I, paclobutrazol, parathion-ethyl, permethrin-trans, piperonylbutoxide, and pyridaben. And 33 pesticides were measured on both years.
There were pesticides measured at specific sampling site and at particular sampling period. 2-aminoacetophenon, bifenthrin, carbofuran, cinnerin II, clothianidin, fluxapyroxad, methomyl, and Terbutryn were only measured at PS1 of year 1 monitoring. Befinox and bitertanol (sum) were only measured at PS2 of year 1 and terbutylazine was only measured at PS3 of year 1.
Aside from the residual pesticides, there were trace concentrations in picogram per liter of OCP, PCB, and PAH, as shown in Tables 3, 4 for Vietnam and the Philippines, respectively. OCPs are synthetic pesticides widely used as insecticides from the 1940s to the 1960s due to their effectiveness. However, due to their high toxicity, slow degradation rate, and propensity for bioaccumulation in the environment, these compounds were prohibited in the early 2000s. OCPs have strong bonds between chlorine and carbon components and are lipophilic. Their insolubility in water facilitates their dispersion through agricultural runoff, allowing them to persist not only in aquatic environments but in soil and other living organisms, including humans, for extended periods. If OCP is applied in a particular field, exposure can be through inhalation or ingestion of contaminated food, fish, dairy products, and other foods with high fat. Since OCPs do not break down easily in fatty tissues, they bioaccumulate or build up in living organisms and are passed on to the next eater.
Polycyclic aromatic hydrocarbon comprises multiple aromatic rings, with naphthalene as the simplest organic compound with two rings. It is uncharged, planar, and nonpolar (insoluble in water). Most PAH is found in coal and oil deposits and is also produced by incomplete combustion or high-pressure processes of organic matter. PAH have relatively high molecular masses, soluble in organic solvents, and are relatively insoluble in water. At room temperature, PAH are solids of low volatility, and most can be photo-oxidized and degraded into simpler organic compounds.
Polychlorinated biphenyls is a chlorinated organic compound used for industrial and commercial applications. It is produced industrially by chlorinating biphenyl. It is lipophilic and does not degrade easily, tending to bioaccumulate in the fatty tissue of living organisms, including humans.
Organochlorine pesticides, PAH, and PCB or commonly called as POPs are organic contaminants that were banned in the Stockholm Convention in the early 2000s due to their persistence to exist and bioaccumulates in the environmental need to be measured, even in trace contaminations for baseline data and risk assessment.
Trace concentrations of OCP, PCB, and PAH were measured in all sampling sites in the 2-year monitoring period, except a few OCPs that were not detected in the second year, namely, aldrin, chlordane-cis, endosulfan II, endosulfan sulfate, HCH-alpha, -delta, and -gamma. The measured traces are shown in Table 3.
The OCP, PCB and PAH trends differ from the residual pesticides. Generally, for OCP and PCB, the traces measured decrease from the first to the second year of monitoring. With values higher in VS2 than in VS1 or VS3 in the first year, but in the second year, VS3 traces were higher than in VS2 or VS1.
Some of these compounds in traces no longer exist in their original active ingredient. These POPs were either degraded or oxidized by factors existing in the environment. The initial active ingredient and its metabolites can co-exist and persist simultaneously in the environment, as shown in the cases of most OCPs measured.
Trace concentrations of OCP, PCB and PAH were measured in all sampling sites at various trends in the 2-year monitoring period, except in a few cases in the second year where HCH compounds were not detected. The OCP contaminations in pg./L in the Cagayan de Oro River Basin, Philippines, are shown in Table 4. For contamination trends, OCPs in the 2-year monitoring are generally higher concentrations in PS1 than PS2 or PS3, except in the second year where chlordane-cis, endosulfan-beta, hexachlorobenzene, hexachloride-1,3-butadiene, and pentachlorobenzene were higher at PS2.
Trace concentrations of OCPs, PCBs, and PAHs were measured in all sampling sites at various trends in the 2-year monitoring period, except in a few cases. In the second year, HCH compounds were undetected, and all PCBs were undetected in PS1 except PCB 28.
For contamination trends, OCPs in the 2-year monitoring are generally higher concentrations in PS1 than PS2 or PS3, except in the second year where chlordane-cis, endosulfan-beta, hexachlorobenzene, hexachloride-1,3-butadiene and pentachlorobenzene were higher at PS2. Generally, for PCBs and PAHs traces, PS3 had higher concentrations than PS1 or PS2 in the first year, but in the second year, PS2 had higher concentrations than PS1 or PS3.
In Vietnam, 2,4-D was measured in only in SD samplers for the 2-year monitoring. It was above the applied threshold level of 10 ng/L only at VS1 and VS2 of year 1. Recently, 2,4-D was banned in Vietnam as proven one of the components of Agent Orange (PAN Asia Pacific, 2017). Atrazine and atrazine-desethyl were measured in all sampling sites for 2 years. Their values were higher in SR than in SD samplers. Atrazine and atrazine-desethyl were proven to be endocrine disruptors that were banned in the European Union.
Carbendazim, dimethoate, ethoprophos, fipronil, propiconazole, and triadimenol have values above the applied threshold level in at least one sampler at a specific sampling site. These compounds are classified as “possible human carcinogen,” except ethoprophos, which is classified as “likely to be carcinogenic to humans” by USEPA (2018).
The values of 27 prevalent pesticides per sampling site within the year of monitoring were statistically compared. The pesticide values on the same sampling sites monitored for 2 years were also statistically compared. Results show no significant differences between the values of these prevalent pesticides from VS1 to VS3 measured within the year for the 2-year monitoring period. No significant differences existed between the pesticides measured from the same sampling stations for a 2-year monitoring period. The ANOVA two-factor with replication result is shown in Supplementary Table 2.
In the Philippines, some pesticides measured were banned or restricted in the EU and the US due to their adverse effect on human health. Carbendazim was banned in the EU (PPDB, 2019); dichlorvos was classified as a probable human carcinogen and was proposed in 1995 by US EPA to cancel its usage (PubChem, 2019a); diuron is known/likely to be a human carcinogen (Huovinen et al., 2015); monuron in the US is not registered for use but manufactured for export (PubChem, 2019d); fenamiphos was listed restricted in the Philippines since 1989 but needed for banana and pineapple plantations (Fertilizer and Pesticide Authority, 2023); pyrimethanil was categorized as a possible human carcinogen (PubChem, 2019b); and simazine was reclassified as not genotoxic in 2005 after being classified as a carcinogen in 1989 (PubChem, 2019c; USEPA/Office of Pesticides Programs, 2006).
The values of 13 prevalent pesticides per sampling site within the year of monitoring were statistically compared, and so with the pesticide values on the same sampling sites monitored for 2 years. Results show significant differences between the values of these prevalent pesticides from PS1 to PS2 to PS3 measured within the year for the 2-year monitoring period. Significant differences existed between the pesticides measured from the same sampling stations for a 2-year monitoring period. The ANOVA two-factor with replication result is shown in Supplementary Table 3.
The statistical results can be further explained by the nature of the two river systems under study. The water flow of Can Tho River, Vietnam, comes from one river system. Meanwhile, the CDO River Basin, Philippines, considered in this study, consists of two river systems that flow toward PS3 downstream.
OCPs, PCBs, and PAHs were measured in trace concentrations in both countries, even banned or restricted by both governments. This shows that after two decades, these organic compounds have persisted in the environment for years. Stockholm Convention of 2004 initially banned or restricted most of these organic compounds (Lallas, 2001) while some compounds were under review and eventually added to the Rotterdam Convention in 2016 (United Nations, 2016). These compounds have been proven to persist, bioaccumulate in the food chain, and adversely affect the environment and humans.
While the residual pesticides measured in this study fall within the tolerable levels of contaminants in river water as established by governmental standards, their presence cannot be disregarded when considering the goal of ensuring sustainable access to clean water for all, as well as ensuring the safety of water for future generations. Prevention and mitigation should be in place to avoid potential health damage. Instances of irreversible consequences, such as the case of over 4,000 workers/farmers in banana plantations in Davao, Philippines, exposed to the pesticide dibromochloropropane (DBCP), serve as stark reminders. Their prolonged exposure to DBCP left 80% of the complainants infertile, with some even testing with zero sperm counts (Chen, 2012; Gomez, 1997). It is an example of the chronic effect of prolonged exposure to pesticides by farm workers.
The extensive presence of multiple residual pesticide contaminations in surface water, spanning rural communities and urban areas downstream, stresses the pervasive impact of agricultural runoff on water quality along these water bodies. The expansion and intensification of agriculture in Vietnam and the Philippines show that agricultural runoff contaminates surface water particularly during the rainy season, when agricultural runoff is expected to rapidly increase.
Despite the residual pesticide levels being within “acceptable” limits according to the government guidelines, the imperative for regular monitoring and evaluation remains paramount. It is crucial for concerned government agencies to establish baseline data to preempt pesticide-related disputes. The inefficacy of current pesticide monitoring methodologies impedes efficient implementation in both countries.
To address this challenge, adoption of an alternative methodology is imperative. Passive samplers, as demonstrated in this study, offers a promising way forward. Passive samplers captured 83 and 69 residual pesticides at concentrations of ng/L in Vietnam and the Philippines, respectively, a result which is unattainable through conventional grab sampling methods. Additionally, trace concentrations of banned compounds such as OCPs, PCBs, and PAHs were detected at pg./L levels. This success can be attributed to the combination of passive samplers tailored for both nonpolar (water-insoluble) and polar (water-soluble) organic contaminants, coupled with sensitive analytical instruments including GCMSMS and LCMSMS.
In light of the demonstrated effectiveness of passive samplers in detecting a wide array of residual pesticides, it is recommended to adopt these innovative sampling techniques into routine water quality monitoring programs in developing countries that are agriculture dependent. This would provide a more comprehensive and robust assessment of these pesticide contamination levels, enabling to substantiate our findings and provide early warnings for the enforcement of effective mitigation strategies. For example, given the detection of trace concentrations of banned compounds such as OCPs, PCBs, and PAHs at pg./L levels, there is a pressing need for stringent regulation and enforcement measures to stop the use and release of these hazardous substances.
Additionally, efforts should be directed toward enhancing pesticide education and training programs for farmers, emphasizing the importance of adopting sustainable agricultural practices and alternative pest management strategies to reduce reliance on chemical pesticides. Collaborative initiatives between government agencies, research institutions, and agricultural stakeholders can facilitate knowledge exchange and capacity-building efforts aimed at promoting environmentally friendly farming practices.
Overall, the adoption of passive sampling techniques coupled with regulatory measures and educational interventions will be required to mitigate pesticide contamination in surface waters and safeguarding environmental and public health of the two countries of this study and beyond. Continued research and investment in innovative monitoring technologies and sustainable agricultural practices seem to be crucial for achieving long-term water quality goals and ensuring the well-being of both ecosystems and communities reliant on these vital water resources.
The original contributions presented in the study are included in the article/supplementary material, further inquiries can be directed to the corresponding author.
MS: Conceptualization, Funding acquisition, Investigation, Methodology, Project administration, Resources, Supervision, Validation, Visualization, Writing – original draft, Writing – review & editing. CZ: Conceptualization, Funding acquisition, Investigation, Project administration, Resources, Supervision, Validation, Visualization, Writing – review & editing. AP: Conceptualization, Investigation, Project administration, Resources, Supervision, Validation, Visualization, Writing – review & editing.
The author(s) declare that financial support was received for the research, authorship, and/or publication of this article. The Netherlands Fellowship Program and Schlumberger Foundation Faculty for the Future funded this work under project number 106275.
Technical support was extended by Deltares, TNO Laboratories, Utrecht, The Netherlands, and the Department of Environment and Natural Resources of Can Tho, Vietnam.
The authors declare that the research was conducted in the absence of any commercial or financial relationships that could be construed as a potential conflict of interest.
All claims expressed in this article are solely those of the authors and do not necessarily represent those of their affiliated organizations, or those of the publisher, the editors and the reviewers. Any product that may be evaluated in this article, or claim that may be made by its manufacturer, is not guaranteed or endorsed by the publisher.
The Supplementary material for this article can be found online at: https://www.frontiersin.org/articles/10.3389/frwa.2024.1474499/full#supplementary-material
Alvarez, D. A. (2013). Development of semipermeable membrane devices (SPMD s) and polar organic chemical integrative samplers (POCIS) for environmental monitoring. Environ. Toxicol. Chem. 32, 2179–2181. doi: 10.1002/etc.2339
Becker, B., Kochleus, C., Spira, D., Möhlenkamp, C., Bachtin, J., Meinecke, S., et al. (2021). Passive sampler phases for pesticides: evaluation of AttractSPE™ SDB-RPS and HLB versus Empore™ SDB-RPS. Environ. Sci. Pollut. Res. 28, 11697–11707. doi: 10.1007/s11356-020-12109-9
Booij, K., Sleiderink, H. M., and Smedes, F. (1998). Calibrating the uptake kinetics of semipermeable membrane devices using exposure standards. Environ. Toxicol. Chem. 17, 1236–1245. doi: 10.1002/etc.5620170707
Booij, K., and Smedes, F. (2010). An improved method for estimating in situ sampling rates of nonpolar passive samplers. Environ. Sci. Technol. 44, 6789–6794. doi: 10.1021/es101321v
Booij, K., Vrana, B., and Huckins, J. N. (2007). Theory, modelling and calibration of passive samplers used in water monitoring: Chapter 7.
Briones, R. M., Galang, I. M. R., and Latigar, J. S. (2023). Transforming Philippine Agri-Food Systems with Digital Technology: Extent, Prospects, and Inclusiveness.
Byrne, B. A., and Ayott, R. I. (1980). Concentrator for Removing Organic Materials from Aqueous System. Bitish Patent. 1566253.
Carmichael, G. R., Ferm, M., Thongboonchoo, N., Woo, J.-H., Chan, L. Y., Murano, K., et al. (2003). Measurements of sulfur dioxide, ozone and ammonia concentrations in Asia, Africa, and South America using passive samplers. Atmos. Environ. 37, 1293–1308. doi: 10.1016/S1352-2310(02)01009-9
Chen, M. (2012). Filipino Banana Workers Frustrated in Battle Over Dole's Pesticides. Philippines: In These Times.
Custodio, K. Q., and Sombilla, M. A. (2020). Rural transformation in the Philippines: a development Agenda1. Policy 2, 1–8.
Deltares (2016). The added value of passive sampling in the monitoring of organic pollutants. Available online at: https://publications.deltares.nl/EP3524.pdf
Deltares. (2019a). Passive sampling for the effective monitoring of pollutants in water. (Accessed April 03, 2024)
Deltares (2019b). Water quality monitoring of plant protection substances. The application of passive sampling.
DENR (2016). Water quality guidelines and general effluent standards of 2016, DENR administrative order no. 2016–08. Phlippines.
Emmers, R., and Le Thu, H. (2021). Vietnam and the search for security leadership in ASEAN. Asian Secur. 17, 64–78. doi: 10.1080/14799855.2020.1769068
Fertilizer and Pesticide Authority. (2023). Banned and restricted pesticides. Available online at: https://fpa.da.gov.ph/NW/index.php/information-resources/data/banned-and-restricted-pesticides# (Accessed April 02, 2024).
Gonçalves, A. D., Martins, T. G., and Cassella, R. J. (2021). Passive sampling of toluene (and benzene) in indoor air using a semipermeable membrane device. Ecotoxicol. Environ. Saf. 208:111707. doi: 10.1016/j.ecoenv.2020.111707
Górecki, T., and Namieśnik, J. (2002). Passive sampling. TrAC Trends Anal. Chem. 21, 276–291. doi: 10.1016/S0165-9936(02)00407-7
Gravell, A., Fones, G. R., Greenwood, R., and Mills, G. A. (2020). Detection of pharmaceuticals in wastewater effluents—a comparison of the performance of Chemcatcher® and polar organic compound integrative sampler. Environ. Sci. Pollut. Res. 27, 27995–28005. doi: 10.1007/s11356-020-09077-5
Hawthorne, S. B., Jonker, M. T., van der Heijden, S. A., Grabanski, C. B., Azzolina, N. A., and Miller, D. J. (2011). Measuring picogram per liter concentrations of freely dissolved parent and alkyl PAHs (PAH-34), using passive sampling with polyoxymethylene. Anal. Chem. 83, 6754–6761. doi: 10.1021/ac201411v
Huovinen, M., Loikkanen, J., Naarala, J., and Vähäkangas, K. (2015). Toxicity of diuron in human cancer cells. Toxicol. in Vitro 29, 1577–1586. doi: 10.1016/j.tiv.2015.06.013
Hurni, K., Schneider, A., Heinimann, A., Nong, D. H., and Fox, J. (2017). Mapping the expansion of boom crops in mainland Southeast Asia using dense time stacks of Landsat data. Remote Sens. 9:320. doi: 10.3390/rs9040320
Ishikawa, K. (2021). The ASEAN economic community and ASEAN economic integration. J. Contemp. East Asia Stud. 10, 24–41. doi: 10.1080/24761028.2021.1891702
Kolpin, D. W., Furlong, E. T., Meyer, M. T., Thurman, E. M., Zaugg, S. D., Barber, L. B., et al. (2002). Pharmaceuticals, hormones, and other organic wastewater contaminants in U.S. streams, 1999−2000: a National Reconnaissance. Environ. Sci. Technol. 36, 1202–1211. doi: 10.1021/es011055j
Lallas, P. L. (2001). The Stockholm convention on persistent organic pollutants. Am. J. Int. Law 95, 692–708. doi: 10.2307/2668517
Leloup, V., Razafindrazay, L. Z., Dzung, N. H., and Vinh, K. Q. (2013). Can Tho (Vietnam) Comphrehensive resilience planning for integrated flood risk management (SCE QA project no. 12060).
Moschet, C., Vermeirssen, E. L., Seiz, R., Pfefferli, H., and Hollender, J. (2014). Picogram per liter detections of pyrethroids and organophosphates in surface waters using passive sampling. Water Res. 66, 411–422. doi: 10.1016/j.watres.2014.08.032
Odetayo, A. A., Reible, D. D., Acevedo-Mackey, D., Price, C., and Thai, L. (2020). Development of polyoxymethylene passive sampler for assessing air concentrations of PCBs at a confined disposal facility (CDF). Environ. Pollut. 265:114720. doi: 10.1016/j.envpol.2020.114720
PAN Asia Pacific (2017). PAN Vietnam welcomes the ban of Paraquat and 2.4-D. PAN Asia Pacific, Vietnam.
PPDB (2019). Carbendazim (Ref:BAS 346F). University of Hertfordshire. Available online at: https://sitem.herts.ac.uk/aeru/ppdb/en/Reports/116.htm (Accessed January 15, 2024).
PubChem. (2019a). PubChem Database, Dichlorvos, CID 3039. National Center for Biotechnology Information, U.S. National Library of Medicine. Available online at: https://pubchem.ncbi.nlm.nih.gov/compound/dichlorvos (Accessed January 15, 2024).
PubChem (2019b). PubChem Database, Pyrimethanil, CID 91650. National Center for Biotechnology Information, U.S. National Library of Medicine. Available online at: https://pubchem.ncbi.nlm.nih.gov/compound/Pyrimethanil (Accessed January 15, 2024).
PubChem (2019c). PubChem Database, Simazine, CID 5216. National Center for Biotechnology Information, U.S. National Library of Medicine. Available online at: https://pubchem.ncbi.nlm.nih.gov/compound/simazine
PubChem (2019d). PubChem, Monuron, CID 8800. Available online at: https://pubchem.ncbi.nlm.nih.gov/compound/monuron from National Center for Biotechnology Information, U.S. National Library of Medicine. Available online at: https://pubchem.ncbi.nlm.nih.gov/compound/monuron
Pulhin, J. M., and Ramirez, M. A. M. (2013). National updates on agribusiness large scale land acquisitions in Southeast Asia brief# 4 of 8: Republic of the Philippines. Agribusiness large-scale land acquisitions and human rights in Southeast Asia: Updates from Indonesia, Thailand, Philippines, Malaysia, Cambodia, Timor-Leste and Burma.
Regan, F., Jones, L., Ronan, J., Crowley, D., McGovern, E., and McHugh, B. (2018). Role of passive sampling in screening and monitoring of new and emerging chemicals. Environmental Protection Agency, Iceland.
Rusina, T. P., Smedes, F., Koblizkova, M., and Klanova, J. (2010). Calibration of silicone rubber passive samplers: experimental and modeled relations between sampling rate and compound properties. Environ. Sci. Technol. 44, 362–367. doi: 10.1021/es900938r
Salingay, M. L. B., Pathirana, A., Rijke, J., Steen, P. V. D., Zevenbergen, C., Quan, N. H., et al. (2017). Microbiological assessment of surface waters and health awareness of four vulnerable communities in can Tho City, Vietman. J. Eng. Appl. Sci. 12, 2644–2650. doi: 10.3923/jeasci.2017.2644.2650
Smedes, F., and Booij, K. (2012). Guidelines for passive sampling of hydrophobic contaminants in water using silicone rubber samplers: International Council for the Exploration of the sea.
Smedes, F., Geertsma, R. W., Zande, T., and Booij, K. (2009). Polymer−water partition coefficients of hydrophobic compounds for passive sampling: application of Cosolvent models for validation. Environ. Sci. Technol. 43, 7047–7054. doi: 10.1021/es9009376
Statista (2024). Total population of the ASEAN countries from 2019 to 2029. Available online at: https://www.statista.com/statistics/796222/total-population-of-the-asean-countries/ (Accessed July 26, 2024).
Sundram, P. (2023). Food security in ASEAN: progress, challenges and future. Front. Sustain. Food Syst. 7:1260619. doi: 10.3389/fsufs.2023.1260619
The World Bank. (2018). “Vietnam continues to reduce poverty, according to WB report” n According to climbing the ladder: Poverty reduction and shared prosperity in Vietnam. Hanoi, Vietnam: The World Bank.
TheGlobalEconomy.com (2024a). Philippines: Agricultural land. Available online at: https://www.theglobaleconomy.com/Philippines/agricultural_land/ (Accessed July 27, 2024).
TheGlobalEconomy.com (2024b). Vietnam: Agricultural land. Available online at: https://www.theglobaleconomy.com/Vietnam/agricultural_land/ (Accessed July 27, 2024).
Tran, D. D., van Halsema, G., Hellegers, P. J., Ludwig, F., and Wyatt, A. (2018). Questioning triple rice intensification on the Vietnamese Mekong delta floodplains: an environmental and economic analysis of current land-use trends and alternatives. J. Environ. Manag. 217, 429–441. doi: 10.1016/j.jenvman.2018.03.116
United Nations (2016). Rotterdam Convention. Sustainable Development Goals, Knowledge Platform. Available online at: https://sustainabledevelopment.un.org/index.php?page=view&type=30022&nr=132&menu=3170 (Accessed January 15, 2024).
USEPA (2018). Chemicals evaluated for carcinogenic, Potentail annual Cancer report 2018. USA: US Environmental Protection Agency. Available online at: http://www.epa.gov/pesticide-reevaluation (Accessed March 25, 2024).
Valenzuela, E. F., Menezes, H. C., and Cardeal, Z. L. (2020). Passive and grab sampling methods to assess pesticide residues in water. Environ. Chem. Lett. 18, 1019–1048. doi: 10.1007/s10311-020-00998-8
Keywords: Mekong delta, CDO River Basin, residual pesticides, passive samplers, emerging pollutants
Citation: Salingay MLB, Zevenbergen C and Pathirana A (2024) Assessment of residual pesticides and other organic pollutants using passive samplers in Can Tho River, Vietnam, and Cagayan de Oro River Basin, Philippines. Front. Water. 6:1474499. doi: 10.3389/frwa.2024.1474499
Received: 01 August 2024; Accepted: 02 October 2024;
Published: 28 October 2024.
Edited by:
Tatenda Dalu, University of Mpumalanga, South AfricaReviewed by:
Sangar Khan, Zhejiang University, ChinaCopyright © 2024 Salingay, Zevenbergen and Pathirana. This is an open-access article distributed under the terms of the Creative Commons Attribution License (CC BY). The use, distribution or reproduction in other forums is permitted, provided the original author(s) and the copyright owner(s) are credited and that the original publication in this journal is cited, in accordance with accepted academic practice. No use, distribution or reproduction is permitted which does not comply with these terms.
*Correspondence: Maria Luisa Baiño Salingay, bS5sLnNhbGluZ2F5QHR1ZGVsZnQubmw=
Disclaimer: All claims expressed in this article are solely those of the authors and do not necessarily represent those of their affiliated organizations, or those of the publisher, the editors and the reviewers. Any product that may be evaluated in this article or claim that may be made by its manufacturer is not guaranteed or endorsed by the publisher.
Research integrity at Frontiers
Learn more about the work of our research integrity team to safeguard the quality of each article we publish.